Abstract
Free full text

Amphiregulin enhances regulatory T cell suppressive function via the epidermal growth factor receptor
Summary
Epidermal growth factor receptor (EGFR) is known to be critically involved in tissue development and homeostasis as well as in the pathogenesis of cancer. Here we showed that Foxp3+ regulatory T (Treg) cells express EGFR under inflammatory conditions. Stimulation with the EGF-like growth factor Amphiregulin (AREG) markedly enhanced Treg cell function in vitro, and in a colitis and tumor vaccination model we showed that AREG was critical for efficient Treg cell function in vivo. In addition, mast cell-derived AREG fully restored optimal Treg cell function. These findings reveal EGFR as a component in the regulation of local immune responses and establish a link between mast cells and Treg cells. Targeting of this immune regulatory mechanism may contribute to the therapeutic successes of EGFR-targeting treatments in cancer patients.
Introduction
Stimulation of the intrinsic tyrosine kinase activity of the epidermal growth factor receptor (EGFR) induces a complex cascade of phosphorylation and activation events that determine cell-fate decisions, such as proliferation or differentiation, and the development of tumors (Schlessinger, 2000; Avraham and Yarden, 2011). The EGFR is well-established to be ubiquitously expressed but, in general, is thought to be absent on the hematopoietic cell linage. This assumption has ignored sporadic demonstrations of EGFR expression on leukocyte populations, such as monocytes (Chan et al., 2009) or plasma cells (Mahtouk et al., 2005). In addition to EGFR, its ligands have also been shown to be not only produced by epithelial cells but also by activated leukocyte and lymphocyte populations. For example, the EGF-like growth factor Amphiregulin (AREG) is expressed by activated Th2 cells (Zaiss et al., 2006), mast cells (Wang et al., 2005; Okumura et al., 2005), eosinophils (Matsumoto et al., 2009) and basophils (Qi et al., 2010). These data suggest that the EGFR signalling pathway could play a more substantial role within the immune system than appreciated, so far.
Hints for such an assumption can also be found from the wide spread use of EGFR targeted treatments in cancer patients. Targeting the EGFR has become an established approach in tumor treatment and such treatments improve the overall and the progression-free survival of patients suffering from different types of cancer, such as colorectal (Cunningham et al., 2004) and non-small-cell lung cancer (NSCLC) (Bonner et al., 2006). The observed clinical successes of this treatment remain poorly understood, but are assumed to be predominantly mediated by interference with survival and growth signals needed by the tumor. This concept is supported by a number of findings, such as, the demonstration of tumor adaption within a patient in response to treatment (Montagut et al., 2012), suggesting strong evolutionary pressure mediated by treatment on the tumor. Nevertheless, other studies indicate that a substantial part of the clinical responses observed following EGFR targeting treatments may not only be mediated by direct effects on the tumor, but also by treatment-induced immune responses (Ferris et al., 2010). For example, Garrido et al. demonstrated in a Lewis lung cell tumor model that the effect of an EGFR blocking antibody treatment was virtually entirely mediated by treatment-induced T cell responses (Garrido et al., 2007). Such involvement of treatment-induced immune responses may also explain a number of somewhat enigmatic clinical reports, such as objective clinical responses to EGFR targeted treatments from patients with tumors lacking detectable EGFR expression (Chung et al., 2005). Most interestingly, it has been reported that the expression of AREG constitute the best known predictor for a low therapy efficacy in cancer patients with a tumor expressing a non-mutated form of K-Ras (Tinhofer et al., 2011; Jacobs et al., 2009; Khambata-Ford et al., 2007; Ishikawa et al., 2005).
AREG is known to be a type II cytokine (Zaiss et al., 2006), for which it is established that one of their main function is the control of inflammation (Chen et al., 2012). In light of these findings, we wanted to determine whether AREG may contribute to the regulation of immune responses. This study showed that regulatory T (Treg) cells expressed EGFR under inflammatory conditions and that AREG was of pivotal importance to ensure optimal Treg cell mediated immune regulation. Thus, our data reveal a novel mechanism by which Treg cell function is regulated at the site of inflammation.
RESULTS
Amphiregulin gene-deficient mice display immune regulatory dysfunction
We previously identified the EGF-like growth factor Amphiregulin (AREG) as a type II cytokine (Zaiss et al., 2006). While characterizing AREG gene-deficient (Areg−/−) mice, we noticed an immune regulatory dysfunction in this mouse strain. This was evident, for example, from enhanced frequencies of antigen (Ag)-specific CD4+ T cells in Areg−/− compared with wild-type (WT) C57BL/6 mice after Listeria monocytogenes infection (data not shown), or from the development of dermatitis after bone marrow (BM) transplantation of Areg−/− BM into WT recipient C57BL/6 mice (Figure 1A & B). Because FoxP3 expressing Treg cells are of crucial importance both for dampening CD4+ T cell responses and immune tolerization after BM transplantation (Cobbold et al., 2006), we postulated that Treg cell function could be impaired in the absence of AREG. Flow-cytometry analyses, however, showed similar frequencies of FoxP3 expressing Treg cells in the secondary lymphoid organs of Areg−/− and WT mice (Figure 1C). Also in the skin-draining, inguinal LN of BM chimeric C57BL/6 WT mice that had received Areg−/− BM, normal frequencies of FoxP3 expressing Treg cells were detected (Figure S1A). These data indicate that neither insufficient numbers nor lack of homing of Treg cells to the site of inflammation could explain the lack of immune regulation observed in Areg−/− mice.
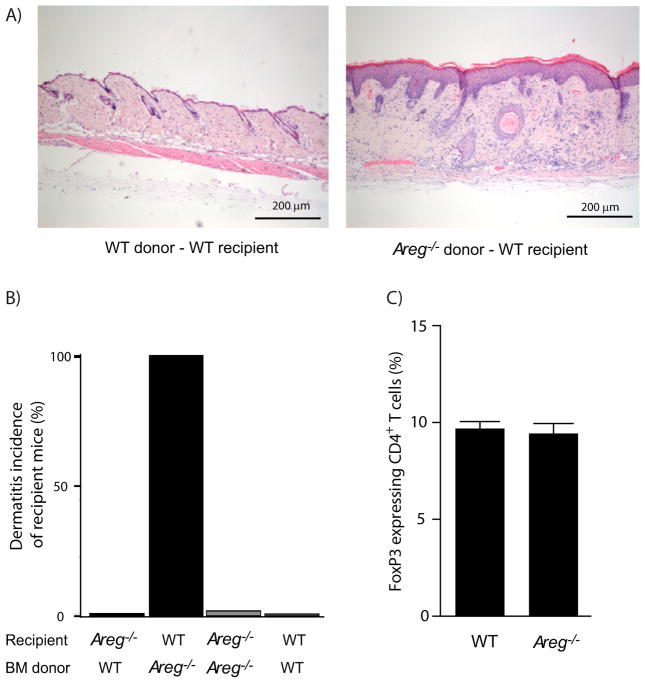
A & B) BL6-SJL (CD45.1) or Areg−/− mice were irradiated with 10 Gy X-rays and reconstituted with 107 BM cells derived from BL6-SJL or Areg−/− mice, incidence of dermatitis of recipient mice six weeks after BM transfer is shown (n= 4 mice per group, bars represent means +SEM).
C) Lymphocytes derived from mesenteric lymph nodes of WT or Areg−/− mice were stained for CD4 and intracellular for FoxP3; frequency of FoxP3 expressing cells is shown (n=4 mice per group, bars represent means +SEM). For additional information, see Figure S1.
Regulatory T cells express the EGFR
To determine whether AREG might have a direct effect on Treg cells, we measured EGFR expression on T cells ex vivo. To this end, we sorted Treg cells from FoxP3-GFP transgenic mice based on GFP expression, as well as Treg cells derived from peripheral blood mononuclear cells (PBMCs) of healthy donors based on high expression of CD25 and the presence or absence of CD127. Analysis by quantitative reverse transcription linked polymerase chain reaction (Q-PCR) showed clearly detectable amounts of EGFR mRNA in Treg cells derived from either species (Figure 2A – C). In human PBMCs, very low EGFR mRNA expression was detectable also in conventional CD4+ T cells, but not in CD8+ T cells (Figure 2B).
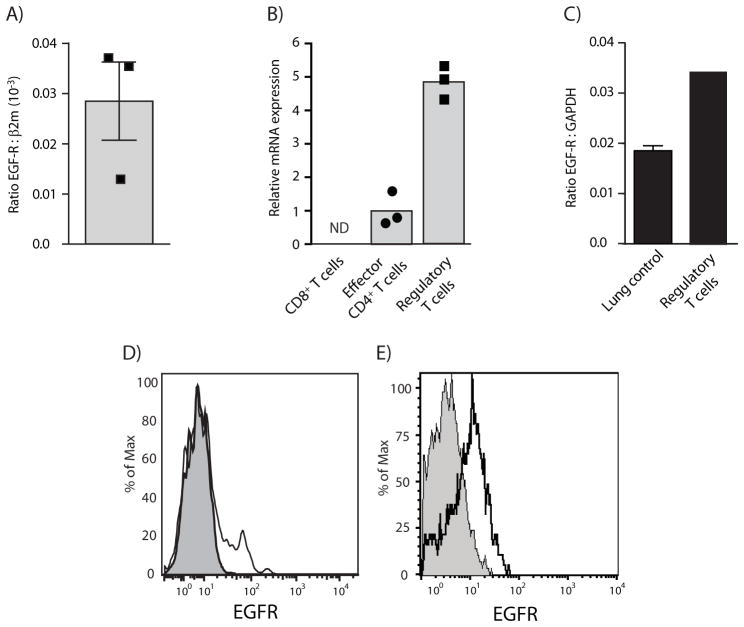
A & B) T cells from the blood of healthy volunteers were sorted by flow cytometry based on CD4 and CD8 expression. Effector T cells and Treg cells were further separated based on non-overlapping expression markers, i.e. CD127hi versus CD127lo and CD25lo versus CD25hi, respectively. EGFR expression was determined by quantitative RT-PCR. A) Depicts absolute expression in comparison to β2m expression and B) depicts relative EGFR expression of different T cell populations to each other. (n=3 volunteers per group, bars represent means +SEM). ND = non detectible (no signal for EGFR)
C) Treg cells derived from the spleen of FoxP3-GFP mice were sorted by flow cytometry based on GFP expression. EGFR mRNA expression was determined by quantitative RT-PCR (Experiment performed in triplicates, bar represents means +/− SEM).
D) PBMC of healthy volunteers were blocked with an excess of unspecific nanobodies and then stained for CD4, EGFR using an EGFR-specific biotinylated nanobody. Thereafter, cells were stained intracellularly for Helios and FoxP3 and SA-PE. Black line shows EGFR staining; filled line represents background SA-PE only staining.
E) EGFR expression on tumor infiltrating Treg cells derived from B16 melanoma of WT C57BL/6 or Egfrflox/flox × Cd4cre mice was analyzed by flow-cytometry. Cells were stained for CD4, EGFR and intracellular for FoxP3. Full lines show the staining of Treg cells derived from WT mice, filled lines the staining of Treg cells derived from Egfrflox/flox × Cd4cre mice.
For additional information, see Figure S2.
To verify EGFR expression on Treg cells by flow cytometry analysis, we used a biotinylated nanobody specific for a shared region of the mouse and human EGFR. About 15% of the FoxP3 and Helios expressing CD4+ T cells in the peripheral blood of healthy volunteers expressed the EGFR (Figure 2D, for gating strategies see Figure S2A) and very low amounts of EGFR were detectable on FoxP3 expressing CD4+ T cells in the spleen of healthy mice (data not shown). B16 melanoma residential Treg cells, however, expressed well detectable amounts of the EGFR (Figure 2E, for gating strategies see Figure S2B). The staining was specific and absent on FoxP3 expressing CD4+ T cells derived from B16 melanoma of Egfrflox/flox× Cd4-cre mice (Figure 2E). Because in humans, almost all EGFR expressing Treg cells were FoxP3hi and CD45RA− (Figure S2C), a subtype of Treg cells that Sakaguchi and colleagues described as activated Treg cells (Miyara et al., 2009), and because human Treg cells gained EGFR expression upon in vitro activation (data not shown), we concluded that Treg cells express the EGFR upon activation.
Amphiregulin enhances regulatory T-cell function
The EGFR and the T cell receptor (TCR) share a common signal transduction pathway, the ERK-MAP-kinase module, and AREG treatment substantially increased ERK activation in differentiated induced Treg cells (Figure 3A). In contrast to in effector T cells, where upon TCR engagement the MAP kinase pathway in a binary manner is briefly activated and then rapidly turned off (Altan-Bonnet and Germain, 2005), this pathway in Treg cells is activated for an extended period of time (Tsang et al., 2006). This situation closely correlated with the MAP kinase signal transduction pathway downstream of the EGFR. Most EGFR ligands, such as EGF or TGFα, induce a strong but transient signal. Such a signal initiates ubiquitination via the E3-ligase Clb, which then induces rapid internalization and degradation of the EGFR and thus a transient desensitization. AREG ligation on the other hand induces a sustained, tonic signal through the MAP kinase signal transduction pathway, which does not induce internalization and degradation of the EGFR (Stern et al., 2008). Thus, we hypothesized that an AREG-induced signal may support and sustain MAP kinase activation in Treg cells, thereby enhancing their regulatory function.
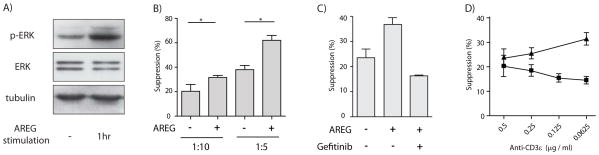
A) iTregs were differentiated form umbilical cord blood CD4+ T cells in the presence of TGF-β. After 5 days of differentiation, 0.5×107 were either treated with 100 ng/ml AREG or left untreated and ERK activation determined by protein blot with p-ERK specific antiserum.
B) PBMCs derived from healthy volunteers were stimulated with membrane-bound anti-CD3 and CD25+CD127− CD4+ Treg cells were added to suppress proliferation of CFSE-labeled CD4+ T cells, in the presence or absence of at least 100 ng/ml recombinant AREG and (C) in the presence or absence of 100 ng/ml Gefitinib. Proliferation was defined as the percentage of cells that had undergone at least one division. (Experiment performed in triplicates; bars represent means +SEM).
D) Mouse FoxP3-GFP CD4+ T cells were cultured for four days together with CFSE labeled CD45.1 expressing splenocytes at a ratio of 1:4 in the presence or absence of 100 ng/ml rec. AREG. T cells were activated with different amounts of soluble anti-CD3, and CFSE dilution within the CD45.1 expressing CD8+ T cell population was analyzed by flow-cytometry. Proliferation was defined as the percentage of cells that had undergone at least one division. Triangles, inhibition in the presence of AREG; squares, inhibition in the absence of AREG. (Experiments performed in triplicates; points are means +/−SEM)
For additional information, see Figure S3.
To address this hypothesis, we determined the effect of AREG on modulating Treg cell function in in vitro suppression assays. As shown in Figure 3B and Figure S3A, the presence of AREG during the assay significantly enhanced the suppressive capacity of Treg cells. Importantly, AREG had no influence on the overall proliferation or survival of Treg cells and did not directly influence the proliferation of effector cells (Figure S3B & C). As a control for the specificity of AREG, we performed in vitro suppression assays in the presence of the EGFR specific tyrosine kinase inhibitor Gefitinib, which entirely eliminated the AREG mediated effect (Figure 3C).
The effect of AREG on the suppressive activity of Treg cells became more pronounced the more the activating anti-CD3ε was diluted (Figure 3D). While the dilution of the antibody had no appreciable direct effect on the proliferation of the effector T cells (data not shown), the suppressive capacity of Treg cells substantially declined in the absence but not in the presence of AREG.
Based on these data we concluded that AREG directly enhances the suppressive capacity of Treg cells in vitro, most likely by enhancing and sustaining the TCR induced signal through the MAP kinase signaling pathway.
Amphiregulin is essential for efficient regulatory T-cell function in vivo
To determine the physiological relevance of AREG expression on Treg cell function in vivo, we tested the suppressive capacity of Treg cells in a T cell transfer colitis model, the gold standard to determine Treg cell function in vivo (Powrie et al., 1994). To this end, we transferred naïve CD4+ T cells in the presence or absence of Treg cells into lymphopenic RAG1-deficient (Rag1−/−) or Areg−/−Rag1−/− mice. Colitis development was determined six weeks after transfer according to a histological score established by Berg et al. (Berg et al., 1996). As shown in Figure 4A, transfer of a fixed number of naïve CD4+ T cells together with increasing amounts of Treg cells into either Rag1−/− or Areg−/−Rag1−/− mice decreased the severity of disease in a dose-dependent manner in both Rag1−/− and Areg−/−Rag1−/− mice. However, transferred Treg cells were significantly less suppressive in Areg−/−Rag1−/− than in Rag1−/− mice (Fig 4A). Since the total number of Treg cells as well as the frequency of Treg cells within the T cell population recovered from the mesenteric lymph nodes and spleen correlated more with the state of inflammation rather than with the genetic background of the animal (data not shown), we concluded that also in vivo AREG does not influence the proliferation or survival of transferred T cells but directly enhances the suppressive capacity of Treg cells.
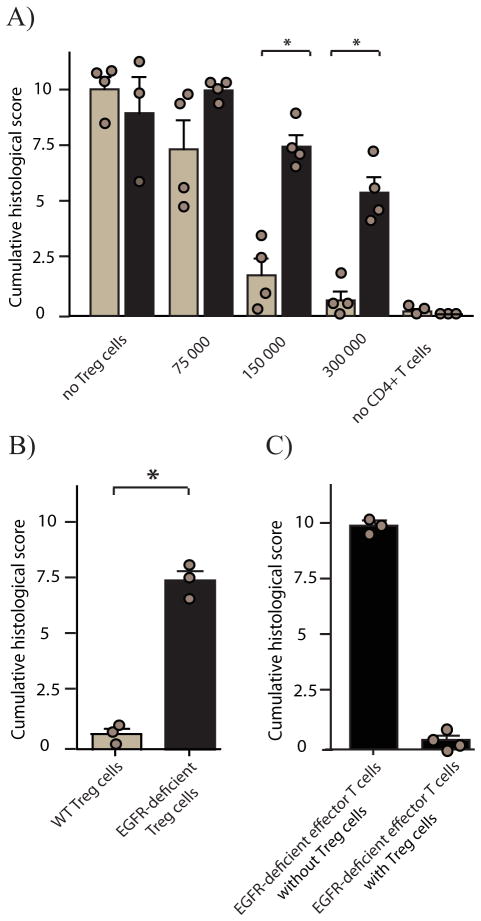
Rag1−/− (light bars) or Areg−/−Rag1−/− (dark bars) mice received 400 000 flow cytometry-sorted naive CD4+ T cells together with (A) increasing numbers of FoxP-GFP expressing Treg cells or (B) Rag1−/− mice received 400 000 naive CD4+ T cells together with 200 000 CD25+ CD4+ T cells derived from either WT (light bar) or Egfrflox/flox × Cd4-cre (dark bar) mice or C) Rag1−/− mice received 400 000 naive CD4+ T cells derived from Egfrflox/flox 0215 Cd4-cre mice in the presence or absence of 200 000 CD25+ CD4+ T cells derived from WT mice. Development of colitis was measured six weeks later by histological score. Bars represent means +SEM; results for individual mice are shown as circles.
For additional information and representative H&E staining of tissue samples derived from the different mouse groups, see Figure S4.
To verify that the AREG-mediated effect was directly mediated via Treg cell-expressed EGFR, we crossed Egfrflox/flox mice onto a Cd4-cre background and transferred sorted Treg cells based on CD25 expression derived from WT and from Egfrflox/flox × Cd4-cre mice into Rag1−/− mice. Both CD25 expressing populations contained similar frequencies of FoxP3 expressing cells (Figure S4G), however, as shown in Figure 4B, EGFR gene-deficient Treg cells were significantly less capable of suppressing the development of colitis than Treg cells derived from WT mice. EGFR gene-deficient naïve CD4+ T cells, however, were fully able to induce colitis upon transfer into Rag1−/− mice and Treg cells derived from WT mice could efficiently suppress their effector function (Figure 4C). These data show that EGFR mediated signaling does not affect the functionality nor the regulation of effector T cells, while at the same time Treg cells are directly dependent on AREG-induced signals for optimal functioning in vivo.
Amphiregulin protects B16 tumors against tumor immunization
EGFR targeting treatment methodologies are well established in the clinic for the treatment of tumors. Specific side-effects, such as skin rashes, have been observed following treatment that could suggest treatment-associated immune dysregulation. At the same time, has it been shown that Treg cells can constitute an important escape mechanism by which tumors protect themselves against tumor-specific immune responses (Zou, 2006). To test the hypothesis that the EGFR targeting treatments could affect Treg function, we performed tumor immunization experiments in the presence and absence of an EGFR blocking antibody and Gefitinib, an EGFR specific tyrosine kinase inhibitor (TKI). We used the B16 melanoma model, for which the pivotal role of Treg cells in the generation of a tumor intrinsic immuno-suppressive environment is well established (Sutmuller et al., 2001). So can tumor immunization-induced rejection of transplanted B16-F10 tumors be achieved in WT C57BL/6 mice, if Tregs are depleted prior to tumor transfer and immunization (Sutmuller et al., 2001). Moreover, B16-F10 tumors lack EGFR expression (Figure 5A). To test the relevance of AREG-EGFR interaction in Treg functioning in this tumor model, we immunized B16 transplanted mice with TRP2180–188 tumor epitope-pulsed in vitro differentiated bone marrow derived dendritic cells (BM-DC), 5 and 7 days after tumor transplantation. Concomitant to immunization, mice were treated with EGFR blocking nanobodies every second day or, as a control (Matsushita et al., 2008), once with a low dose of cyclophosphamide (Figure 5B). As described before (Sutmuller et al., 2001; Matsushita et al., 2008), immunization alone had no effect on tumor growth in C57BL/6 mice. Also nanobody or cyclosphosphamide treatment each by itself exerted no substantial influence on tumor growth. The combination of immunization with nanobody treatment, however, significantly enhanced the efficacy of the peptide-pulsed BM-DC immunization (Figure 5B). A similar enhanced efficacy of peptide-pulsed BM-DC immunization was obtained following concomitant treatment with the EGFR-specific tyrosine kinase inhibitor Gefitinib (Figure 5C), although slightly less pronounced than observed by EGFR blocking nanobody treatment. This slightly lower efficacy is explained most likely by the short serum half-life of Gefitinib of only approximately six hrs, due to rapid excretion through the kidney.
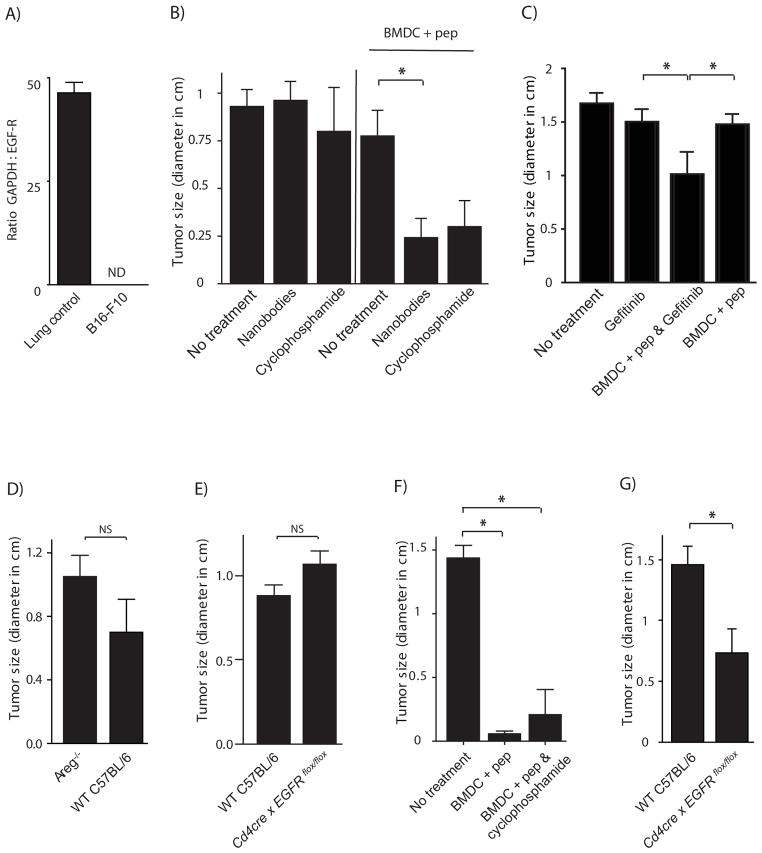
A) mRNA from B16-F10 tumor cells and control lung tissue was purified and quantitative RT-PCR was performed. Bar is mean of ratio GAPDH : EGFR expression +SEM. ND = non-detectable (no signal for EGFR).
B – C) 1×104 B16-F10 cells were injected s.c. into the left flank of WT C57BL/6. On day 5 and 7 after tumor cell transfer, mice were immunized with BM-DC loaded with an immunogenic B16 melanoma epitope (TRP2180–188) or left un-immunized. One mouse group received in addition 300 μg purified EGFR blocking nanobodies (B), or 200 μg Gefitinib (C), every other day for one week starting from day 5.
D–G) 1×104 B16-F10 cells were injected s.c. into WT C57BL/6 or AREG−/− (D & F) mice or Egfrflox/flox × Cd4-cre mice (E & G). On day 5 and 7 after tumor cell transfer, mice were immunized with BM-DC loaded with an immunogenic B16 melanoma epitope (TRP2180–188) (F & G) or left un-immunized (D & E). Tumor size was determined on days 21 till 23 after tumor transfer. (n = 3 – 7 mice per group; bars represent mean +SEM)
Taken together, our data show that EGFR targeted treatments can facilitate the rejection of a transplanted tumor that does not express the EGFR, when applied concomitant to CD8+ T-cell inducing anti-tumor immunization. These data indicate that EGFR mediated signals are of critical importance for Treg mediated establishment of a tumor intrinsic immune suppressive environment.
To establish that the observed effects of EGFR blocking treatment indeed are AREG dependent and mediated via Treg cell expressed EGFR, we transferred B16-F10 melanoma cells into WT C57BL/6, Areg−/− or Egfrflox/flox × Cd4-cre mice and immunized them with TRP2180–188 tumor epitope-pulsed BM-DC, 5 and 7 days after tumor transplantation. B16 tumors grew with similar characteristics in the different mouse strains (Figure 5D & E) (although consistently somewhat faster in AREG deficient than in WT mice, as shown in Figure 5D); however, unlike WT mice, Areg−/− (Figure 5F) and Egfrflox/flox × Cd4-cre (Figure 5G) mice efficiently rejected transplanted B16-F10 tumors upon immunization. Because BM-DC immunization induced similar anti-TRP2180–188-specifc immune responses in the different mouse strains (data not shown) and we were not able to detect EGFR expression in CD8 T cells (Figure 2B), we concluded that AREG-mediated enhancement of Treg cell function is of critical importance for the establishment of a tumor intrinsic immune suppressive environment in B16 tumors that prevents successful tumor rejection upon immunization in mice.
Mast cell-derived Amphiregulin enhances regulatory T-cell function
Next we investigated the physiologically relevant source of AREG that enhances Treg cell function in vivo. Since Treg cells and mast cells have been shown to cooperate with each other (Lu et al., 2006; Hershko et al., 2011) and AREG has been shown to be the single most up-regulated growth factor upon mast cell activation (Wang et al., 2005; Okumura et al., 2005; and Figure S5), we tested whether mast cells could be a source of AREG in our models. To this end, we used mast cell deficient c-kitw-sh/w-sh mice (Grimbaldeston et al., 2005), which were reconstituted with in vitro differentiated BM-derived mast cells (BM-MC) prior to B16 transplantation and DC immunization. As shown in Figure 6A, c-kitw-sh/w-sh control mice that did not receive in vitro differentiated BM-MC or mice that had been reconstituted with BM-MC differentiated from BM from Areg−/− mice, efficiently controlled tumor growth following peptide-specific immunization. In contrast, immunized mice reconstituted with BM-MC differentiated from BM derived from WT C57BL/6 mice failed to control B16 growth. Since tumor growth was similar in all un-immunized c-kitw-sh/w-sh mouse groups (Figure 6B) and similar numbers of mast cells were detected in the peri-tumoral region surrounding blood vessels (Figure 6C) in all reconstituted c-kitw-sh/w-sh mice, these data indicate that in the absence of AREG expressing mast cells, Treg cell function was impaired.
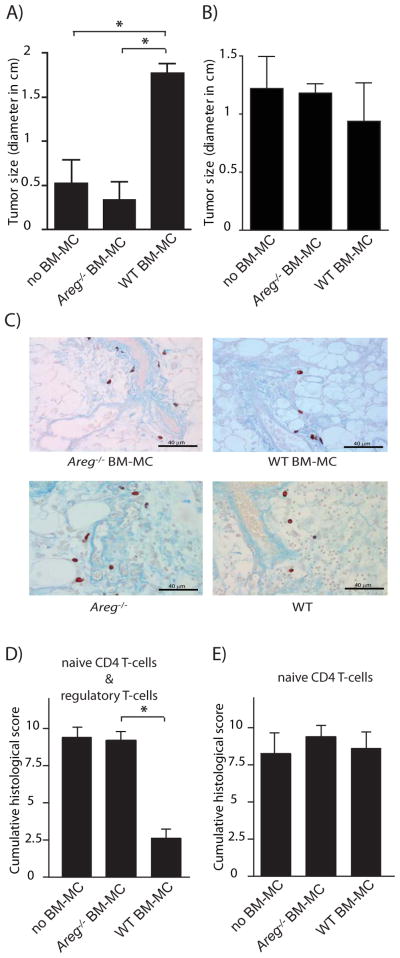
A&B) 1×104 B16-F10 cells were injected s.c. into c-kitw-sh/w-sh mice reconstituted with BM-MC differentiated from BM derived from WT C57BL/6 or Areg−/− mice, or were left un-reconstituted. On day 5 and 7 after tumor cell transfer, mice were immunized with BM-DC loaded with an immunogenic B16 melanoma epitope (TRP2180–188) (A) or left un-immunized (B). Tumor size was determined 23 days after tumor transfer. n = 5–7 mice per group. Bars represent means +SEM.
C) c-kitw-sh/w-sh mice were reconstituted with either WT or Areg−/− BM-MC. Three weeks after re-constitution 10 000 B16-F10 cells were injected. 21 days after tumor cell transfer, tumors were harvested and stained for mast cells using Csaba staining.
D&E) c-kitw-sh/w-shRag1−/− mice were reconstituted with BM-MC derived from either WT C57BL/6 or Areg−/− mice, or were left un-reconstituted. 200 000 FoxP3/GFP CD4+ T cells were co-transferred with 400 000 naive CD4+ T cells and colitis scores were determined six weeks after transfer. (n = 3–7 mice per group. Bars represent means +SEM). For additional information, see Figure S6.
Experiments performed in the T cell transfer induced colitis model (Figure 6D & E) and the BM transfer induced dermatitis model (Figure S1B) confirmed the critical role of mast cell-derived AREG in enhancing Treg cell function. Colitis scores in c-kitw-sh/w-shRAG1−/− mice that had been reconstituted with BM-MC differentiated from BM of WT mice prior to transfer were six weeks after co-transfer of naïve CD4+ T cells and Treg cells significantly lower than in mice that had not received BM-MC or had been reconstituted with BM-MC differentiated from BM of Areg−/− mice (Figure 6D). Transfer of naïve CD4+ T cells only, led to similar severe forms of colitis in all c-kitw-sh/w-shRAG1−/− mouse groups (Figure 6E), indicating that neither mast cells nor mast cell-derived AREG were necessary for the functioning or differentiation of colitogenic effector T cells. Also co-transfer of BM-MC differentiated from BM derived from WT C57BL/6, but not of BM-MC differentiated from BM derived from Areg−/− mice prevented the development of dermatitis of WT C57BL/6 BM-chimeric mice that had received Areg−/− BM (Figure S1B).
These data, obtained in mast cell reconstituted mice indicate that leukocyte-, in this case mast cell-derived, AREG can be of crucial importance for efficient Treg functioning in vivo.
DISCUSSION
The pivotal role of Treg cells in immune regulation, i.e. in fine tuning protective immune responses and minimizing harmful pathology, has become widely appreciated. Despite this appreciation, many aspects of the highly complex process of how Treg cells are activated and, upon entry of inflamed tissues, are regulated, remain incompletely understood. Different factors and cell types have been shown to play a prominent role in this process, and a number of factors have been identified that influence the functionality of Tregs at the site of inflammation. So far, mainly factors that diminish Treg cell function, such as IL6 or TLR mediated signals, have been described. Importantly, however, experiments have demonstrated that Treg cells gain optimal suppressive capacity only by migrating through the site of inflammation (Zhang et al., 2009). This finding suggests that at the site of inflammation also factors that enhance Treg cell function must be present. In line with this thought, we here demonstrate that AREG-induced and Treg cell expressed EGFR mediated signals are of pivotal importance for the optimal functioning of Treg cells in vivo. In particular the observations that AREG is the single most up-regulated growth factor upon activation of human mast cells (Wang et al., 2005) and that mast cell-derived AREG can be of critical importance for optimal Treg function shed a new light on the interaction between mast cells and Tregs. Both Tregs and mast cells rapidly infiltrate sites of inflammation and mutual regulation has repeatedly been described (reviewed in Mekori and Hershko, 2012). So far, however, mainly factors such as the cytokines IL-9 (Lu et al., 2006) and IL-2 (Hershko et al., 2011) have been described to be involved in this cross talk between mast cells and Tregs. The main function of these cytokines, however, is to support survival and proliferation of mast cells (Townsend et al., 2000) and Treg cells (Burchill et al., 2007), respectively. Thus, interference with either of these factors has strong influences on the overall number of either type of cells. The finding presented here, that mast cell-derived AREG can directly enhances Treg cell function, adds a new qualitative component to this interaction.
In the situation of developing tumors, both mast cells and Treg cells are known to accumulate in the peri-tumoral region (Ju et al., 2009) and we found that a particularly high percentage of Treg cells in the ectopic lymphoid structures at the periphery of tumors expressed the EGFR. Accumulation of mast cells around tumors has repeatedly been associated with poor prognosis for patients suffering from a number of tumors, such as for example prostate cancer (Johansson et al., 2011), melanoma (Ribatti et al., 2003), breast cancer (Amini et al., 2007) or colon-carcinoma (Lachter et al., 1995). Mast cells have been shown to support tumor growth in a number of different ways such as, for example, by enhancing the vascularization of the tumor, in particular in the early phase of tumor development (Murdoch et al., 2008). Nevertheless, a contribution of mast cells to the tumor intrinsic immune suppressive environment is well established as well (Huang et al., 2008). Our data described here that in particular mast cell-derived AREG substantially contributes to the immune suppressive environment within tumors, suggest a novel molecular mechanism by which mast cells may support this Treg cell mediated tumor intrinsic immuno suppressive environment.
In line with this hypothesis, it is tempting to speculate that the immune regulatory function of the EGFR may contribute to the clinical successes of EGFR targeting medications. Tumor treatments often have multiple, sequential effects. Starting with the direct killing of a portion of the tumor cells, the thereby released tumor antigens can prime anti-tumor immune responses de novo or re-activate dormant, anergic responses (Apetoh et al., 2007). Our findings suggest that EGFR-targeting treatments may, in addition, also allow treatment-induced anti-tumor immune responses to be more effective, as the treatment may also interfere with the suppressive capacity of tumor-intrinsic Treg cells that otherwise could dampen the induced anti-tumor immune response.
Our findings may explain also why EGFR targeting treatments in some tumors, such as colorectal carcinoma (Cunningham et al., 2004), function primarily as an adjuvant to concomitant chemotherapy (CT). CT not only induces cell death that results in release of tumor antigens that can prime or re-activate anti-tumor T cell responses and diminishes the overall tumor load temporarily and, thus, the therewith associated immune suppressive environment (Zitvogel et al., 2008), but most importantly, CT also induces a transient lymphopenia in which dormant and/or anergic tumor-specific T cell responses can be re-activated (Schietinger et al., 2012). Thus, in patients undergoing CT treatment a similar situation is created as in our T cell transfer induced colitis model in Rag1−/− mice. Most interestingly, also in many other settings the enhancing effects of transient lymphopenia on the induction of auto-immune responses have been observed (Krupica et al., 2006; Guerau-de-Arellano et al., 2009) and in particular in such lymphopenic situations optimal Treg cell function has been shown to be of pivotal importance for maintenance of immune tolerance and tissue homeostasis (Le Campion et al., 2009). Thus, it is easy to envision that blocking of the EGFR on Treg cells in combination with CT can enhance the efficacy of tumor treatments - which closely correlates with what is observed in the clinic (Cunningham et al., 2004). The exact mechanisms by which EGFR targeted treatments mediate their objective clinical responses in cancer patients has remained poorly understood and further targeted research to determine the precise role of the immune system in the clinical successes of EGFR targeted tumor treatments is needed. Nevertheless, our data showing a direct effect of the EGFR on Treg cell regulation may reveal one unexpected effect of such treatments. A much wider usage of EGFR targeting treatments to enhance anti-tumor immune responses concomitant to other tumor treatments may therefore appear warranted.
Thus, taken together, we here reveal an EGFR mediated mechanism that contributes to the regulation of local immune responses. While factors that diminish Treg cell function at the site of inflammation have been known for many years, we here describe a factor that enhances Treg function. Optimal immune regulation probably will follow from the balance between the two types of factors. It is easy to envision that either type of factor could have a distinct relevance at different time points of inflammation. During the acute phase, factors that diminish Treg cell function most likely prevail, however, when the inflammation has cooled down, mast cell-derived AREG could play a more prominent role, and thereby prevent excessive tissue damage and the development of chronic inflammations.
Material and Methods
Mice
C-kitw-sh/w-sh and FoxP3-GFP transgenic mice were purchased from Jackson Laboratory, CD4-cre mice were purchased from Taconics and Egfrflox/flox mice (Natarajan et al., 2007) were provided by M. Sibilia, University of Vienna. Areg−/−, which had been backcrossed for at least 14 times onto a C57BL/6 background, B6.SJL (CD45.1) and Rag1−/− mice were bred in house under specific pathogen-free conditions. Mice were used between 7–17 wks of age. All animal experiments were approved by the Committee on Animal Experiments of the University of Utrecht.
Nanobody isolation
As EGFR blocking antibodies target the human EGFR only and do not cross-react with the murine EGFR, we first screened antibodies derived from Llama Immunoglobulin libraries for their capacity to interact with mouse EGFR. Besides conventional immunoglobulins, llamas express single-domain, heavy chain antibodies devoid of the light chain, known as nanobodies. We isolated three nanobody-producing clones that targeted a between the mouse and the human EGFR conserved stretch, among which RR359 blocked EGFR activation (Figure S6). To enhance the nanobody half-life in the serum of treated mice, a bivalent, bispecific nanobody construct was prepared by cloning an anti-mouse albumin nanobody at the C-terminus of RR359, separated by a 15(G4S) linker; resulting in a serum half-life of this bi-head nanobody of approximately 48 hrs (data not shown). Bivalent RR359 was expressed in S. cerevisiae shake flask cultures and purified via C-His tag affinity using 1 ml HisTrap column. For FACS staining the nanobody was dialyzed to PBS with 5 ml HiTrap Desalting column (both GE Healthcare) and biotinylated using biotin (amido hexanoic acid 3-sulpho-N-hydroxy succinimide ester, Roche); staining was performed in the presence of Fc-block and / or unspecific, unlabeled nanobodies.
B16 melanoma vaccination
10 000 B16-F10 melanoma cells were transferred subcutaneously into the lower left flank of mice. Mice were then either immunized with TRP2180–188 peptide-loaded BM-DC on day 5 and 7 after transfer or left untreated. On day six after tumor transfer, mice were either i.p. injected every other day with 10 mg / kg bodyweight Gefitinib or with 300 μg purified EGFR blocking nanobodies. Assuming a half-life of about 48 hrs, the level of nanobodies per mouse was kept above 100 μg for one week. A control group received once on day 5 after transfer, an i.p. injection of 50 mg/kg body weight cyclophosphamide dissolved in 100 μl PBS to inactivate Tregs. 21 days after injection the tumor size was determined. Up to a diameter of approximately 0.8 cm tumors normally grew perfectly round. At bigger sizes, space restrainments formed more oval shaped tumors; of these the average of width versus length was calculated.
Induced colitis mouse model
Rag1−/− mice were injected with 4×105 CD4+CD45RBhigh cells to induce colitis. If not stated differently in the figure legend, 2×105 Tregs isolated from Foxp3-GFP mice were co-transferred and six weeks later the mice were sacrificed and colons scored by two independent experts in a blinded fashion according to Berg et al. (Berg et al., 1996), in brief: Grade 0: no infiltration of mononuclear cells. Grade 1: few foci of mononuclear cells, only slight depletion of goblet cells. Grade 2: many foci of mononuclear cells, infiltration in the lamina propria, however, not yet in the submucosa; diminished numbers of goblet cells. Grade 3: strong infiltration, also in the submucosa; epithelial hyperplasia; number of goblet cells strongly diminished. Grade 4: transmural infiltration of mononucleated cells; strong epithelial hyperplasia, goblet cell depletion. Overall histological score per mouse is the sum of individual scores for the different segments of the colon (c. ascendens, c. transversum, c. descendens).
Differentiation and reconstitution of bone marrow derived mast cells
Bone marrow-derived mast cells (BM-MCs) were differentiated in vitro from bone marrow cells that were cultured for 3 wks in the presence of pokeweed mitogen-stimulated spleen cell-conditioned medium as a source for IL-3. Non-adherent cells were passed once a week into new medium and purity of BM-MC population was determined by flow cytometry. Cultures contained a uniform cell population to over 94% positive for c-Kit and FcεRIα. Mast cell-deficient c-kitw-sh/w-sh mice with an age of at least 8 weeks were i.v. injected and received 5 × 106 cultured BM-MC cells three weeks prior to start of the experiments. Mast cell reconstitution of the inflamed area was determined by Csaba staining and toluidine blue.
In vitro suppression assay Human
CD4+CD25highCD127low T cells isolated from human PBMC were co-cultured with PBMC labeled with 2μM CFSE in anti-CD3 (clone OKT3) coated 96-wells. Cells were cultured for four days in RPMI medium supplemented with 10% FCS, penicillin & streptomycin, and 2-mercaptoethanol, in the presence or absence of 100 ng/ml recombinant Amphiregulin, purified from the supernatant of COS7 cells that had been transiently been transfected with AREG expressing vectors or purchased from R&D Systems. Proliferation of CD4+ and CD8+ cells was determined by measuring CFSE dilution using the FACS CANTO (BD Biosciences). Proliferation was defined as the percentage of cells that have undergone at least one division.
Mouse
FACS-sorted Tregs were added to CFSE labeled CD45.1 expressing splenocytes at different ratios. Cells were cultured in IMDM supplemented with 10% FCS, glutamax, penicillin & streptomycin, and 2-mercaptoethanol for four days, in the presence or absence of 100 ng/ml recombinant AREG. T cells were activated with different amounts of soluble anti-CD3 (clone 145-2C11; BD Pharmingen). To determine suppression of proliferation, CFSE dilution within the CD45.1 expressing T cell populations was analyzed by FACS. Proliferation was defined as the percentage of cells that have undergone at least one division.
Statistics
We performed data analysis using statistical software (Prizm 4.0, GraphPad Software). Comparisons between two groups were performed using two-tailed Mann-Whitney test. P-values <0.05 were considered significant.
Acknowledgments
The authors’ research was supported by a grant from the University of Utrecht to DZ, Wellcome Trust grant WT 085733MA and NIH Grant AI064576 to AS; and a Seed Grant from the Infectious Diseases and Immunology Center Utrecht (IICU) to AS and PC. DZ performed the mouse experiments, JvL and CB performed the experiments involving human material. AGo, PvBeH and RR isolated and expressed the EGFR blocking nanobody, AGr help with the histological analysis and MS contributed the Egfrflox/flox mouse strain. DZ and AS conceived the experimental approach, directed the experiments and interpret the data. DZ, PC and AS wrote the manuscript.
Footnotes
Publisher's Disclaimer: This is a PDF file of an unedited manuscript that has been accepted for publication. As a service to our customers we are providing this early version of the manuscript. The manuscript will undergo copyediting, typesetting, and review of the resulting proof before it is published in its final citable form. Please note that during the production process errors may be discovered which could affect the content, and all legal disclaimers that apply to the journal pertain.
References
- Altan-Bonnet G, Germain RN. Modeling T cell antigen discrimination based on feedback control of digital ERK responses. PLoS Biol. 2005;3:e356. [Abstract] [Google Scholar]
- Amini RM, Aaltonen K, Nevanlinna H, Carvalho R, Salonen L, Heikkila P, Blomqvist C. Mast cells and eosinophils in invasive breast carcinoma. BMC Cancer. 2007;7:165. [Europe PMC free article] [Abstract] [Google Scholar]
- Apetoh L, Ghiringhelli F, Tesniere A, Obeid M, Ortiz C, Criollo A, Mignot G, Maiuri MC, Ullrich E, Saulnier P, et al. Toll-like receptor 4-dependent contribution of the immune system to anticancer chemotherapy and radiotherapy. Nat Med. 2007;13:1050–1059. [Abstract] [Google Scholar]
- Avraham R, Yarden Y. Feedback regulation of EGFR signalling: decision making by early and delayed loops. Nat Rev Mol Cell Biol. 2011;12:104–117. [Abstract] [Google Scholar]
- Berg DJ, Davidson N, Kuhn R, Muller W, Menon S, Holland G, Thompson-Snipes L, Leach MW, Rennick D. Enterocolitis and colon cancer in interleukin-10-deficient mice are associated with aberrant cytokine production and CD4(+) TH1-like responses. J Clin Invest. 1996;98:1010–1020. [Europe PMC free article] [Abstract] [Google Scholar]
- Bonner JA, Harari PM, Giralt J, Azarnia N, Shin DM, Cohen RB, Jones CU, Sur R, Raben D, Jassem J, et al. Radiotherapy plus cetuximab for squamous-cell carcinoma of the head and neck. N Engl J Med. 2006;354:567–578. [Abstract] [Google Scholar]
- Burchill MA, Yang J, Vang KB, Farrar MA. Interleukin-2 receptor signaling in regulatory T cell development and homeostasis. Immunol Lett. 2007;114:1–8. [Europe PMC free article] [Abstract] [Google Scholar]
- Chan G, Nogalski MT, Yurochko AD. Activation of EGFR on monocytes is required for human cytomegalovirus entry and mediates cellular motility. Proc Natl Acad Sci U S A. 2009;106:22369–22374. [Europe PMC free article] [Abstract] [Google Scholar]
- Chen F, Liu Z, Wu W, Rozo C, Bowdridge S, Millman A, Van Rooijen N, Urban JF, Jr, Wynn TA, Gause WC. An essential role for T(H)2-type responses in limiting acute tissue damage during experimental helminth infection. Nat Med. 2012;18:260–266. [Europe PMC free article] [Abstract] [Google Scholar]
- Chung KY, Shia J, Kemeny NE, Shah M, Schwartz GK, Tse A, Hamilton A, Pan D, Schrag D, Schwartz L, et al. Cetuximab shows activity in colorectal cancer patients with tumors that do not express the epidermal growth factor receptor by immunohistochemistry. J Clin Oncol. 2005;23:1803–1810. [Abstract] [Google Scholar]
- Cobbold SP, Adams E, Graca L, Daley S, Yates S, Paterson A, Robertson NJ, Nolan KF, Fairchild PJ, Waldmann H. Immune privilege induced by regulatory T cells in transplantation tolerance. Immunol Rev. 2006;213:239–255. [Abstract] [Google Scholar]
- Cunningham D, Humblet Y, Siena S, Khayat D, Bleiberg H, Santoro A, Bets D, Mueser M, Harstrick A, Verslype C, Chau I, Van Cutsem E. Cetuximab monotherapy and cetuximab plus irinotecan in irinotecan-refractory metastatic colorectal cancer. N Engl J Med. 2004;351:337–45. [Abstract] [Google Scholar]
- Ferris RL, Jaffee EM, Ferrone S. Tumor antigen-targeted, monoclonal antibody-based immunotherapy: clinical response, cellular immunity, and immunoescape. J Clin Oncol. 2010;28:4390–4399. [Europe PMC free article] [Abstract] [Google Scholar]
- Garrido G, Lorenzano P, Sanchez B, Beausoleil I, Alonso DF, Perez R, Fernandez LE. T cells are crucial for the anti-metastatic effect of anti-epidermal growth factor receptor antibodies. Cancer Immunol Immunother. 2007;56:1701–1710. [Abstract] [Google Scholar]
- Grimbaldeston MA, Chen CC, Piliponsky AM, Tsai M, Tam SY, Galli SJ. Mast cell-deficient W-sash c-kit mutant Kit W-sh/W-sh mice as a model for investigating mast cell biology in vivo. Am J Pathol. 2005;167:835–848. [Europe PMC free article] [Abstract] [Google Scholar]
- Guerau-de-Arellano M, Martinic M, Benoist C, Mathis D. Neonatal tolerance revisited: a perinatal window for Aire control of autoimmunity. J Exp Med. 2009;206:1245–1252. [Europe PMC free article] [Abstract] [Google Scholar]
- Hershko AY, Suzuki R, Charles N, Alvarez-Errico D, Sargent JL, Laurence A, Rivera J. Mast cell interleukin–2 production contributes to suppression of chronic allergic dermatitis. Immunity. 2011;35:562–571. [Europe PMC free article] [Abstract] [Google Scholar]
- Huang B, Lei Z, Zhang GM, Li D, Song C, Li B, Liu Y, Yuan Y, Unkeless J, Xiong H, Feng ZH. SCF-mediated mast cell infiltration and activation exacerbate the inflammation and immunosuppression in tumor microenvironment. Blood. 2008;112:1269–1279. [Europe PMC free article] [Abstract] [Google Scholar]
- Ishikawa N, Daigo Y, Takano A, Taniwaki M, Kato T, Hayama S, Murakami H, Takeshima Y, Inai K, Nishimura H, et al. Increases of amphiregulin and transforming growth factor-alpha in serum as predictors of poor response to gefitinib among patients with advanced non-small cell lung cancers. Cancer Res. 2005;65:9176–9184. [Abstract] [Google Scholar]
- Jacobs B, De Roock W, Piessevaux H, Van Oirbeek R, Biesmans B, De Schutter J, Fieuws S, Vandesompele J, Peeters M, Van Laethem JL, et al. Amphiregulin and epiregulin mRNA expression in primary tumors predicts outcome in metastatic colorectal cancer treated with cetuximab. J Clin Oncol. 2009;27:5068–5074. [Abstract] [Google Scholar]
- Johansson A, Rudolfsson S, Hammarsten P, Halin S, Pietras K, Jones J, Stattin P, Egevad L, Granfors T, Wikstrom P, Bergh A. Mast cells are novel independent prognostic markers in prostate cancer and represent a target for therapy. Am J Pathol. 2011;177:1031–1041. [Europe PMC free article] [Abstract] [Google Scholar]
- Ju MJ, Qiu SJ, Gao Q, Fan J, Cai MY, Li YW, Tang ZY. Combination of peritumoral mast cells and T-regulatory cells predicts prognosis of hepatocellular carcinoma. Cancer Sci. 2009;100:1267–1274. [Abstract] [Google Scholar]
- Khambata-Ford S, Garrett CR, Meropol NJ, Basik M, Harbison CT, Wu S, Wong TW, Huang X, Takimoto CH, Godwin AK, et al. Expression of epiregulin and amphiregulin and K-ras mutation status predict disease control in metastatic colorectal cancer patients treated with cetuximab. J Clin Oncol. 2007;25:3230–3237. [Abstract] [Google Scholar]
- Krupica T, Jr, Fry TJ, Mackall CL. Autoimmunity during lymphopenia: a two-hit model. Clin Immunol. 2006;120:121–128. [Abstract] [Google Scholar]
- Lachter J, Stein M, Lichtig C, Eidelman S, Munichor M. Mast cells in colorectal neoplasias and premalignant disorders. Dis Colon Rectum. 1995;38:290–293. [Abstract] [Google Scholar]
- Le Campion A, Gagnerault MC, Auffray C, Becourt C, Poitrasson-Riviere M, Lallemand E, Bienvenu B, Martin B, Lepault F, Lucas B. Lymphopenia-induced spontaneous T-cell proliferation as a cofactor for autoimmune disease development. Blood. 2009;114:1784–1793. [Abstract] [Google Scholar]
- Lu LF, Lind EF, Gondek DC, Bennett KA, Gleeson MW, Pino-Lagos K, Scott ZA, Coyle AJ, Reed JL, Van Snick J, et al. Mast cells are essential intermediaries in regulatory T-cell tolerance. Nature. 2006;442:997–1002. [Abstract] [Google Scholar]
- Mahtouk K, Hose D, Reme T, De Vos J, Jourdan M, Moreaux J, Fiol G, Raab M, Jourdan E, Grau V, et al. Expression of EGF-family receptors and amphiregulin in multiple myeloma. Amphiregulin is a growth factor for myeloma cells. Oncogene. 2005;24:3512–3524. [Europe PMC free article] [Abstract] [Google Scholar]
- Matsumoto K, Fukuda S, Nakamura Y, Saito H. Amphiregulin production by human eosinophils. Int Arch Allergy Immunol. 2009;149(Suppl 1):39–44. [Abstract] [Google Scholar]
- Matsushita N, Pilon-Thomas SA, Martin LM, Riker AI. Comparative methodologies of regulatory T cell depletion in a murine melanoma model. J Immunol Methods. 2008;333:167–179. [Europe PMC free article] [Abstract] [Google Scholar]
- Mekori YA, Hershko AY. T cell-mediated modulation of mast cell function: heterotypic adhesion-induced stimulatory or inhibitory effects. Frontiers in Inflammation. 2012;3 10.3389/fimmu.2012.00006. [Europe PMC free article] [Abstract] [CrossRef] [Google Scholar]
- Miyara M, Yoshioka Y, Kitoh A, Shima T, Wing K, Niwa A, Parizot C, Taflin C, Heike T, Valeyre D, et al. Functional delineation and differentiation dynamics of human CD4+ T cells expressing the FoxP3 transcription factor. Immunity. 2009;30:899–911. [Abstract] [Google Scholar]
- Montagut C, Dalmases A, Bellosillo B, Crespo M, Pairet S, Iglesias M, Salido M, Gallen M, Marsters S, Tsai SP, et al. Identification of a mutation in the extracellular domain of the Epidermal Growth Factor Receptor conferring cetuximab resistance in colorectal cancer. Nat Med. 2012;18:221–223. [Abstract] [Google Scholar]
- Murdoch C, Muthana M, Coffelt SB, Lewis CE. The role of myeloid cells in the promotion of tumour angiogenesis. Nat Rev Cancer. 2008;8:618–631. [Abstract] [Google Scholar]
- Natarajan A, Wagner B, Sibilia M. The EGF receptor is required for efficient liver regeneration. Proc Natl Acad Sci U S A. 2007;104:17081–17086. [Europe PMC free article] [Abstract] [Google Scholar]
- Okumura S, Sagara H, Fukuda T, Saito H, Okayama Y. FcepsilonRI-mediated amphiregulin production by human mast cells increases mucin gene expression in epithelial cells. J Allergy Clin Immunol. 2005;115:272–279. [Abstract] [Google Scholar]
- Powrie F, Leach MW, Mauze S, Menon S, Caddle LB, Coffman RL. Inhibition of Th1 responses prevents inflammatory bowel disease in scid mice reconstituted with CD45RBhi CD4+ T cells. Immunity. 1994;1:553–562. [Abstract] [Google Scholar]
- Qi Y, Operario DJ, Oberholzer CM, Kobie JJ, Looney RJ, Georas SN, Mosmann TR. Human basophils express amphiregulin in response to T cell-derived IL-3. J Allergy Clin Immunol. 2010;126:1260–1266. e1264. [Europe PMC free article] [Abstract] [Google Scholar]
- Ribatti D, Ennas MG, Vacca A, Ferreli F, Nico B, Orru S, Sirigu P. Tumor vascularity and tryptase-positive mast cells correlate with a poor prognosis in melanoma. Eur J Clin Invest. 2003;33:420–425. [Abstract] [Google Scholar]
- Schietinger A, Delrow JJ, Basom RS, Blattman JN, Greenberg PD. Rescued tolerant CD8 T cells are preprogrammed to reestablish the tolerant state. Science. 2012;335:723–727. [Europe PMC free article] [Abstract] [Google Scholar]
- Schlessinger J. Cell signaling by receptor tyrosine kinases. Cell. 2000;103:211–225. [Abstract] [Google Scholar]
- Stern KA, Place TL, Lill NL. EGF and amphiregulin differentially regulate Cbl recruitment to endosomes and EGF receptor fate. Biochem J. 2008;410:585–594. [Europe PMC free article] [Abstract] [Google Scholar]
- Sutmuller RP, van Duivenvoorde LM, van Elsas A, Schumacher TN, Wildenberg ME, Allison JP, Toes RE, Offringa R, Melief CJ. Synergism of cytotoxic T lymphocyte-associated antigen 4 blockade and depletion of CD25(+) regulatory T cells in antitumor therapy reveals alternative pathways for suppression of autoreactive cytotoxic T lymphocyte responses. J Exp Med. 2001;194:823–832. [Europe PMC free article] [Abstract] [Google Scholar]
- Tinhofer I, Klinghammer K, Weichert W, Knodler M, Stenzinger A, Gauler T, Budach V, Keilholz U. Expression of amphiregulin and EGFRvIII affect outcome of patients with squamous cell carcinoma of the head and neck receiving cetuximab-docetaxel treatment. Clin Cancer Res. 2011;17:5197–5204. [Abstract] [Google Scholar]
- Townsend JM, Fallon GP, Matthews JD, Smith P, Jolin EH, McKenzie NA. IL-9-deficient mice establish fundamental roles for IL-9 in pulmonary mastocytosis and goblet cell hyperplasia but not T cell development. Immunity. 2000;13:573–583. [Abstract] [Google Scholar]
- Tsang JY, Camara NO, Eren E, Schneider H, Rudd C, Lombardi G, Lechler R. Altered proximal T cell receptor (TCR) signaling in human CD4+CD25+ regulatory T cells. J Leukoc Biol. 2006;80:145–151. [Abstract] [Google Scholar]
- Wang SW, Oh CK, Cho SH, Hu G, Martin R, Demissie-Sanders S, Li K, Moyle M, Yao Z. Amphiregulin expression in human mast cells and its effect on the primary human lung fibroblasts. J Allergy Clin Immunol. 2005;115:287–294. [Abstract] [Google Scholar]
- Zaiss DM, Yang L, Shah PR, Kobie JJ, Urban JF, Mosmann TR. Amphiregulin, a TH2 cytokine enhancing resistance to nematodes. Science. 2006;314:1746. [Abstract] [Google Scholar]
- Zhang N, Schroppel B, Lal G, Jakubzick C, Mao X, Chen D, Yin N, Jessberger R, Ochando JC, Ding Y, Bromberg JS. Regulatory T cells sequentially migrate from inflamed tissues to draining lymph nodes to suppress the alloimmune response. Immunity. 2009;30:458–469. [Europe PMC free article] [Abstract] [Google Scholar]
- Zitvogel L, Apetoh L, Ghiringhelli F, Kroemer G. Immunological aspects of cancer chemotherapy. Nat Rev Immunol. 2008;8:59–73. [Abstract] [Google Scholar]
- Zou W. Regulatory T cells, tumour immunity and immunotherapy. Nat Rev Immunol. 2006;6:295–307. [Abstract] [Google Scholar]
Full text links
Read article at publisher's site: https://doi.org/10.1016/j.immuni.2012.09.023
Read article for free, from open access legal sources, via Unpaywall:
https://europepmc.org/articles/pmc3582723?pdf=render
Citations & impact
Impact metrics
Citations of article over time
Alternative metrics
Article citations
Group 2 Innate Lymphoid Cells in Allergic Rhinitis.
J Inflamm Res, 17:8599-8610, 09 Nov 2024
Cited by: 0 articles | PMID: 39539728 | PMCID: PMC11559184
Review Free full text in Europe PMC
Amphiregulin promotes activated regulatory T cell-suppressive function via the AREG/EGFR pathway in laryngeal squamous cell carcinoma.
Head Face Med, 20(1):62, 26 Oct 2024
Cited by: 0 articles | PMID: 39456084 | PMCID: PMC11515249
AREG Upregulation in Cancer Cells via Direct Interaction with Cancer-Associated Fibroblasts Promotes Esophageal Squamous Cell Carcinoma Progression Through EGFR-Erk/p38 MAPK Signaling.
Cells, 13(20):1733, 19 Oct 2024
Cited by: 0 articles | PMID: 39451251 | PMCID: PMC11506648
Mini-Review: Tregs as a Tool for Therapy-Obvious and Non-Obvious Challenges and Solutions.
Cells, 13(20):1680, 11 Oct 2024
Cited by: 0 articles | PMID: 39451198 | PMCID: PMC11506333
Review Free full text in Europe PMC
Bridging Chronic Inflammation and Digestive Cancer: The Critical Role of Innate Lymphoid Cells in Tumor Microenvironments.
Int J Biol Sci, 20(12):4799-4818, 09 Sep 2024
Cited by: 0 articles | PMID: 39309440 | PMCID: PMC11414386
Review Free full text in Europe PMC
Go to all (247) article citations
Data
Data behind the article
This data has been text mined from the article, or deposited into data resources.
BioStudies: supplemental material and supporting data
Similar Articles
To arrive at the top five similar articles we use a word-weighted algorithm to compare words from the Title and Abstract of each citation.
Amphiregulin Confers Regulatory T Cell Suppressive Function and Tumor Invasion via the EGFR/GSK-3β/Foxp3 Axis.
J Biol Chem, 291(40):21085-21095, 18 Jul 2016
Cited by: 82 articles | PMID: 27432879 | PMCID: PMC5076518
Basophil-derived amphiregulin is essential for UVB irradiation-induced immune suppression.
J Invest Dermatol, 135(1):222-228, 04 Jul 2014
Cited by: 28 articles | PMID: 25089660
Membrane-bound Dickkopf-1 in Foxp3+ regulatory T cells suppresses T-cell-mediated autoimmune colitis.
Immunology, 152(2):265-275, 27 Jun 2017
Cited by: 14 articles | PMID: 28556921 | PMCID: PMC5588763
Amphiregulin as a novel target for breast cancer therapy.
J Mammary Gland Biol Neoplasia, 13(2):171-179, 25 Apr 2008
Cited by: 29 articles | PMID: 18437539
Review
Funding
Funders who supported this work.
NIAID NIH HHS (2)
Grant ID: AI064576
Grant ID: R01 AI064576
Wellcome Trust (1)
Grant ID: WT 085733MA