Abstract
Free full text

Plasma Membrane Estrogen Receptors
Abstract
It is now firmly established that estrogen and all sex steroid receptors exist in discrete cellular pools outside the nucleus. Estrogen receptors (ER) have been localized to the plasma membrane where both ERα and ERβ function in a wide variety of cells and organs. ERs have also been found in discrete cytoplasmic organelles including mitochondria and the endoplasmic reticulum. In ligand-dependent fashion, each ER pool contributes to the overall, integrated effects of estrogens producing biological outcomes. This review highlights the recent work establishing new roles and targets of membrane ER signaling. Such actions include prevention of vascular injury or cardiac hypertrophy, sexual behavior and pain perception mediated through the central nervous system, osteoblast survival, and fluid resorption in the colon.
Overview
Sex steroid and other steroid receptor superfamily members exist in discrete cellular locations in the cell, including the nucleus and extra-nuclear compartments [1]. The most completely studied of the extra-nuclear steroid receptors are the plasma membrane estrogen receptors (ER). Rapid estrogen by signaling from the plasma membrane was first identified forty years ago in Clara Szego's laboratory [2,3]. Many investigators have subsequently contributed to this area and have defined key aspects of the nature and functions of extra-nuclear ER. Both ERα and ERβ, the two ER isoforms, localize in many cells to the plasma membrane and to cytoplasmic organelles including mitochondria and the endoplasmic reticulum. Little is known about the functions of mitochondrial ER [4] and virtually nothing is established concerning endoplasmic reticulum ER. Both in-vitro and in-vivo models have defined the structure/function aspects of extra-nuclear ER, and the conclusions derived from this work have been extended to other members of the extra-nuclear steroid receptor family [1]. Ongoing work continues to define unanticipated and novel functions of these receptors for steroid biology.
Plasma membrane ER
Nature of membrane ER
The identity of membrane ER has been quite controversial but has more recently been defined. It is now clear that cells derived from combined ERα/ERβ knockout mice lack all estrogen binding proteins, including at the plasma membrane [5]. These cells also lack the ability to respond to 17-β-estradiol (E2) in rapidly activating signaling to downstream kinase cascades that contribute to the cell biological effects of the steroid hormone. Estrogen action for gene transcription is also lost in ERα/ERβ null cells, in part due to the combined lack of membrane and nuclear ERα [6]. The loss of steroid receptor-induced transcription compromises the normal development of the mammary gland and reproductive tracts in female mammals.
To determine the nature of endogenous E2-binding proteins, an unbiased isolation of membrane ER from a breast cancer cell line was accomplished by affinity chromatography [5]. The estrogen-binding protein was identified by mass spectrometry as the classical ERα, identical to the nuclear ERα from the same cells. These findings are congruent to results from a variety of additional cell-based approaches, including siRNA knockdown of classical ERα and ERβ [5] and earlier studies using anti-sense oligonucleotides and immunohistochemical identification of ER at the membrane [7].
One purported membrane or endoplasmic reticulum ER is the orphan G-protein coupled receptor 30 (GPR30) [8,9]. This receptor was initially reported to respond to E2 or the ER antagonist, ICI182780, with both binding and signal transduction (e.g.-cAMP generation and calcium stimulation). More recent work has addressed this concept definitively. Four different GPR30 knockout mice have been created and show little phenotype despite extensive evaluation (reviewed in [10]). Importantly, none of these models demonstrate any reproductive or mammary gland disruption of structure or function [10]. This is in marked contrast to the ERα knockout female mouse that exhibits infertility, extreme atrophy of the uterus, hemorrhagic cysts in the ovary, and rudimentary development of the mammary glands [11]. ERα knockout mice are obese, and lack the hypothalamic/pituitary feedback regulation of LH secretion seen in wild type mice. In contrast, GPR30 KO mice exhibit comparable functions in all these respects to wild type mice. In recent cell based studies, work has not shown that E2 binds to GPR30, and a GPR30 chemical agonist, G1, has not been found to simulate estrogen actions in mammary gland or reproductive organs [12]. In some cells types, there is evidence that GPR30 might collaborate with membrane- localized ERα as part of a large complex of proteins at the membrane (signalsome), transmitting membrane ERα-generated signals to downstream kinase cascades [13]. Any further work in this area should use in-vivo ER and GPR30 KO models to establish functions of estrogen that are not mediated by ERα and ERβ, but are lost from GPR30 deletion.
Another putative ER has been reported in the central nervous system in double ERα/ERβ KO mice, but the nature of this receptor is undefined [14]. Various truncated ERα and ERβ proteins have been identified in various extra-nuclear sites, but their importance to the overall actions of estrogen is not known. Defining the natural expression and functions of these ERs is important.
Trafficking of ER to the Plasma Membrane
Approximately 5–10% of total cellular ER is found at the plasma membrane in many cells. This percentage may include both ERα and ERβ, but there is differential localization depending on the cell type. For instance, both ERα and ERβ are found at the membrane of vascular endothelial cells, existing as both homodimers and heterodimers. The membrane heterodimer in endothelial cells probably mediates the rapid actions of estrogen to induce vasodilation in vivo, resulting from rapid activation of ERK and PI3K, leading to nitric oxide generation that affects smooth muscle tone [15]. It is not clear what structures in the two isoforms dictate hetero-dimerization, and this area is understudied. In other cells, such as breast cancer cells, it is mainly ERα that is found at the plasma membrane with scant ERβ present [5].
What dictates trafficking of ER to the plasma membrane? Serine 522 was first identified to be necessary for ER translocation, as this residue promotes interaction with the caveolin-1 protein [16]. Caveolin-1 is a necessary transporter of ERα to the caveolae rafts in the plasma membrane. In fact, in cells lacking caveolin-1, endogenous ERα is only found in the nucleus (16). Marino and colleagues established that cysteine 447 in the E domain (steroid hormone binding domain) of human ERα (equivalent to 451 in the mouse) is a site for palmitoylation that promotes association of ER with caveolin-1 [17]. It was subsequently determined that the cysteine is part of a larger 9 amino acid palmitoylation motif found in both ER isoforms [18]. As determined by mutational analysis, key residues in this motif are necessary for full palmitoylation of ER. Furthermore, similar palmitoylation motifs were found in both progesterone and androgen receptor E domains.
For all sex steroid receptors, palmitoylation seems necessary for plasma membrane localization and rapid signaling through ERK and PI3 kinases to cell proliferation [18] (Figure 1). Glucocorticoid and Vitamin D receptors are also palmitoylated, suggesting a similar mechanism of trafficking. Cellular proteins that promote receptor palmitoylation are under intensive investigation and could possibly explain why only 5–10% of ERs are found at the plasma membrane despite 100% of ER containing the palmitoylation motif. We propose that the protein abundance of the palmitoylacyltransferase for ER, or other proteins that facilitate palmitoylation, may limit the number of receptors trafficking to the membrane. It will be important to define the proteins that facilitate ER palmitoylation and the subsequent steps of trafficking between cytoplasmic organelles and the plasma membrane.
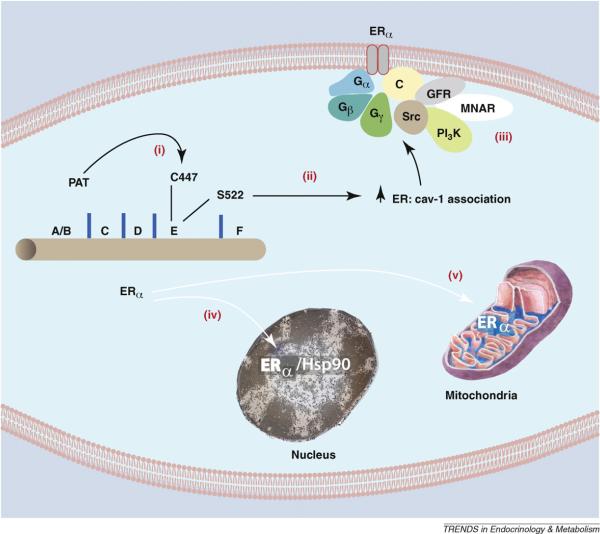
Palmitoylation of Cysteine 447 is required for ERα translocation to the cell membrane. (i) Cysteine 447 in the E (ligand binding) domain of human ERα is the site of palmitoylation by an undetermined palmitoylacyltransferase (PAT). Palmitoylation of this amino acid is necessary for ERα association with (ii) caveolin-1 and subsequent transport to the membrane. Serine 522 also promotes the physical interaction of ER with caveolin-1 by unknown mechanisms. (iii) At the membrane, caveolin-1 serves as a scaffold for other signal molecules that are activated by E2 binding ERα in membrane caveolae rafts. Other ERα proteins are transported to the (iv) nucleus (chaperoned by heat shock protein 90 and dependent on a nuclear localization sequence), or to (v) mitochondria by an undetermined mechanism. GFR, growth factor receptor; MNAR, modulator of non-genomic action of the estrogen receptor; C, caveolin-1.
Posttranslational modification of ERα by methylation at arginine 260 in the DNA binding domain occurs upon ligand binding the receptor. The specific methylation of arginine 260 by the PRMT1 (protein N-arginine methyltransferase) protein promotes cytoplasmic localization of ERα and association with focal adhesion kinase, Src kinase, and the p85 subunit of PI3 [19]. Arginine 260 methylation occurs in the cytoplasm and triggers PI3 kinase activation, contributing to cell cycle progression and cell proliferation. It is not clear why methylation of arginine 260 promotes the cytoplasmic localization of ERα, leading to rapid signaling.
Rapid Signaling by ER at the membrane
It has been clearly shown that membrane-localized ERα and ERβ associate with and activate Gα and Gβγ proteins as perhaps the earliest rapid signals generated [20,21]. This takes place in proximity to the caveolae rafts, leading to calcium and cAMP generation, and the activation of both proximal kinases (Src, PI3K) and distal kinases (ERK, AKT) (Box 1). These and other signals lead to the phosphorylation of many proteins, modulating cell migration, survival, and proliferation. Signaling from the membrane also enhances the transcriptional effects of nuclear ERα by several mechanisms [22].
To better understand the impact of membrane ERα signaling in development, a transgenic mouse was generated that lacks all cellular ERα but carries a knock in of the E domain of this receptor targeted exclusively to the plasma membrane [23]. This membrane–only ERα mouse (MOER) showed impaired female reproductive tract and mammary gland development that was comparable to the complete ERα knock out mouse. Furthermore, the mice were obese and lacked normal regulation of the hypothalamic-pituitary-ovarian sex steroid regulatory axis, again a comparable phenotype to the ERαKO mouse [23]. These results strongly support the importance of nuclear ERα for normal development and function of these organs. Interestingly, signal transduction through ERK and PI3K were comparable in wild type and MOER mouse liver, yet completely absent in the ERαKO liver [23]. This indicates that membrane-localized ERα is necessary and sufficient for rapid signal transduction. The results do not rule out a possible collaboration between the membrane and nuclear ERα pools that facilitates normal development of target organs.. In this sense, loss of either membrane or nuclear ER may compromise the normal development or function of target organs. Also, potential differences between MOER and ERαKO mice in bone biology, cardiovascular responses to stress, and functions of the central nervous system or many other organs have not yet been assessed.
Impact of rapid signaling by ER in the heart and vasculature
Previous studies suggested roles for the membrane ER in rescuing cardiomyocytes from simulated ischemia/reperfusion injury [24]. Recent work implicates membrane ERβ in preventing cardiac hypertrophy, both in-vitro and in-vivo [25,26].E2/ERβ signals through PI3K inducing the transcription of the MCIP (modulatory calcineurin-interacting protein) gene. This gene codes for a protein that binds to protein phosphatase 2B (calcineurin) in the cytoplasm, blocking the increased activity of calcineurin that is stimulated by Angiotensin II (Ang II) and other hypertrophic factor signaling. Blocking calcineurin activity prevents the de-phosphorylation of the NFAT family of transcription factors, a step that is required for nuclear translocation of the transcription factors. Inhibiting NFAT de-phosphorylation sequesters the transcription factors in the cytoplasm, thereby preventing transcription of hypertrophic genes [25]. Also, E2/ERβ activates transcription of the natriuretic peptide genes (ANP, BNP), the protein products inhibiting hypertrophic signaling by AngII through ERK MAP kinase in the cardiomyocyte. In vivo, these effects of E2 were present in wild type and ERαKO mice but not in ERβ KO mice [26]. Interestingly, E2/ERβ prevents the reversal of myosin heavy chain isoform formation and cardiac fibrosis induced by AngII, important steps that prevent the progression of the heart from hypertrophy to dilation and heart failure. It is conceivable that an ERβ specific agonist could prevent cardiac hypertrophy in post-menopausal women who are at risk with poorly controlled hypertension. An ERβ-selective agent would avoid the breast and uterine proliferative effects of E2 that are mediated through ERα.
A newly described endogenous selective estrogen receptor modulator (SERM), 27-hydroxycholesterol (27HC), is produced from cholesterol metabolism and is abundant in the arterial wall of diseased blood vessels [27]. This endogenous SERM competitively inhibits E2 binding to vascular ER and prevents both the rapid and transcriptional actions of E2/ER in blood vessels. Rapid actions modulate NOS activity/NO production, the response to vascular injury, and vasorelaxation. It may be that competition between this atherogenic form of cholesterol and E2 for binding to vascular ER prevents the anti-atherogenic properties of E2 that prevent myocardial infarction [28].
DNA repair signaling in breast cancer
E2 and ERα are known promoters of breast cancer development, perhaps in conjunction with progesterone and its receptor. In response to DNA damage of normal or transformed breast epithelial cells, cell cycle checkpoints are induced, and rapid assembly of DNA repair complexes occurs [29]. Checkpoint induction allows sufficient time for repair of the DNA lesions before either DNA is replicated (S phase) or the cell divides (M phase). Signal transduction is critical for many of these responses to DNA damage and is mediated by the PI3K family members ataxia-telangiectasia mutated (ATM) and ATM and Rad 3-related kinases (ATR). Mutation of ATR, ATM or genes that are downstream in the kinase cascades leads to increased malignancies [30].
It is possible that endogenous inhibitors of these kinases impede cell cycle checkpoint induction and delay/block DNA repair. This could lead to the acquisition of mutations that contribute to the development or progression of cancer.
E2 and membrane ERα were recently shown to block ATR and ATM signaling and the activation of downstream kinases and phosphatases [30] (Figure 2). This occurs when E2 signals through membrane ERα and PI3K/AKT to phosphorylate the TopBP1 protein, a protein necessary for ATR activity. Phosphorylation at the single AKT site of TopBP1 prevents its enhanced interaction with ATR. This enhanced interaction promotes increased ATR activity [31], following radiation or chemotherapy damage of breast cancer or normal mammary epithelial cells [29]. E2/ERα also causes additional chromosomal breaks in the setting of DNA damage induced by gamma radiation, probably resulting from the steroid receptor blocking the ATR signaling cascade prompted by DNA damage, as noted above. Fortunately, ICI182780 (Fulvestrant), an ER antagonist that is used as adjuvant therapy for women with breast cancer, blocks all these actions of E2. This may represent a novel function of this therapeutic agent. These results suggest a new oncogenic mechanism for E2/ER, one that may be relevant to both the development and progressive biology of breast neoplasia.
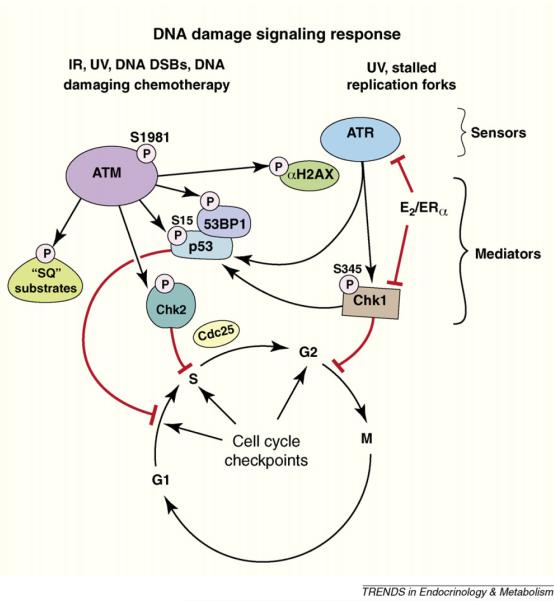
Cartoon of response to DNA damage. Damage-causing double strand DNA breaks or replication fork stalling results in the assembly of unique repair protein complexes at the sites of the DNA lesions. The different complexes trigger activation of ataxia-telangiectasia mutated (ATM) or ATM and Rad 3-related kinases (ATR), respectively, initiating signal transduction cascades. ATM and ATR phosphorylation of serine residues on checkpoint kinases (Chk) 1 and 2 mediates further signaling to enact cell cycle checkpoints. E2 acting through the cell membrane ERα stimulates AKT-induced block of ATR and Chk1 activity, leading to the inhibition of cell cycle checkpoints.
Rapid Signaling in Bone
Recent studies continue to define a role for membrane ER signaling through ERK or other pathways in bone development. In a ligand independent fashion, mechanotransduction stimulates membrane ERα and ERb to activate ERK, leading to osteocyte and osteoblast survival [32]. In addition, membrane ERα activation by an ER-binding compound, estren, stimulates Wnt and bone morphogenic protein 2 (BMP-2) signaling, leading to osteoblast differentiation [33]. Interestingly, E2 suppresses BMP-2 induced osteoblast precursor differentiation when the steroid engages both membrane and nuclear ERα [33]. These findings suggest that ligands interacting selectively with membrane ERα hold promise for stimulating bone formation.
Estrogen modulation of ion channels and G protein Coupled Receptors
Recent studies from the Harvey laboratory show that E2 rapidly stimulates calcium entry into colonic epithelial cells via the TRPV6 channels [34]. E2 also suppresses cAMP-dependent chloride secretion in the distal colonic epithelium of both rats and humans. This occurs by E2 initiating PKCδ and PKA-dependent suppression of the potassium channel, KCNQ1 [35]. Interestingly, this only occurs in female and not male rats, and may contribute to the fluid retention that occurs in female rodents during the estrus cycle (and has a counterpart in humans during the menstrual cycle).
In the central nervous system, membrane ERα and ERβ activate metabotropic glutamate receptors, a family of G protein coupled receptors present in neurons throughout the brain [reviewed in 36]. These interactions may mediate lordosis (sexual) behavior, pain perception, and neuroprogesterone synthesis. E2 has also recently been shown to mediate memory consolidation in mice, through E2-stimulated ERK activation in the hippocampus [37].
Perspective
The effects of steroid hormones reflect their actions at the various intracellular pools in a coordinated but poorly understood fashion, leading to final cell biological outcomes. Delineating the discrete actions of the various receptor pools will help us understand the breadth of steroid hormone action, especially in organs that are not thought of as traditional steroid hormone targets. As an example, ERα KO or Aromatase KO mice exhibit many features of the metabolic syndrome, including insulin resistance and obesity. This phenotype likely derives from direct transcriptional actions, rapid signaling, and mitochondrial effects of ER pools in liver, muscle, fat, and the pancreatic beta cell. To best understand these complicated functions, new in vivo models of selective receptor pool loss must be created, and reagents targeted to specifically activate these pools must be developed. Using such approaches, we may uncover functions of estrogen that are entirely unanticipated, making the designation sex steroid receptor extremely parochial.
References
Full text links
Read article at publisher's site: https://doi.org/10.1016/j.tem.2009.06.009
Read article for free, from open access legal sources, via Unpaywall:
https://europepmc.org/articles/pmc3589572?pdf=render
Citations & impact
Impact metrics
Article citations
Sex Differences in Colon Cancer: Genomic and Nongenomic Signalling of Oestrogen.
Genes (Basel), 14(12):2225, 16 Dec 2023
Cited by: 0 articles | PMID: 38137047 | PMCID: PMC10742859
Review Free full text in Europe PMC
Peering into the Brain's Estrogen Receptors: PET Tracers for Visualization of Nuclear and Extranuclear Estrogen Receptors in Brain Disorders.
Biomolecules, 13(9):1405, 18 Sep 2023
Cited by: 0 articles | PMID: 37759805 | PMCID: PMC10526964
Estrogens, Estrogen Receptors and Tumor Microenvironment in Ovarian Cancer.
Int J Mol Sci, 24(19):14673, 28 Sep 2023
Cited by: 11 articles | PMID: 37834120 | PMCID: PMC10572993
Review Free full text in Europe PMC
Estrogen Receptor Signaling in Breast Cancer.
Cancers (Basel), 15(19):4689, 23 Sep 2023
Cited by: 10 articles | PMID: 37835383 | PMCID: PMC10572081
Review Free full text in Europe PMC
Hormone-mediated neural remodeling orchestrates parenting onset during pregnancy.
Science, 382(6666):76-81, 05 Oct 2023
Cited by: 8 articles | PMID: 37797007 | PMCID: PMC7615220
Go to all (202) article citations
Similar Articles
To arrive at the top five similar articles we use a word-weighted algorithm to compare words from the Title and Abstract of each citation.
Palmitoylation of estrogen receptors is essential for neuronal membrane signaling.
Endocrinology, 154(11):4293-4304, 05 Sep 2013
Cited by: 55 articles | PMID: 24008343 | PMCID: PMC3800757
Nature of functional estrogen receptors at the plasma membrane.
Mol Endocrinol, 20(9):1996-2009, 27 Apr 2006
Cited by: 321 articles | PMID: 16645038
Nervous system physiology regulated by membrane estrogen receptors.
Rev Neurosci, 19(6):413-424, 01 Jan 2008
Cited by: 46 articles | PMID: 19317180 | PMCID: PMC2662393
Review Free full text in Europe PMC
The extra-nuclear interactome of the estrogen receptors: implications for physiological functions.
Mol Cell Endocrinol, 538:111452, 07 Sep 2021
Cited by: 15 articles | PMID: 34500041
Review