Abstract
Free full text

Critical role of STAT3 in leptin’s metabolic actions
Summary
Leptin has pleiotropic effects on glucose homeostasis and feeding behavior. Here, we validate the use of a cell-permeable phosphopeptide that blocks STAT3 activation in vivo. The combination of this biochemical approach with stereotaxic surgical techniques allowed us to pinpoint the contribution of hypothalamic STAT3 to the acute effects of leptin on food intake and glucose homeostasis. Leptin’s ability to acutely reduce food intake critically depends on intact STAT3 signaling. Likewise, hypothalamic signaling of leptin through STAT3 is required for the acute effects of leptin on liver glucose fluxes. Lifelong obliteration of STAT3 signaling via the leptin receptor in mice (s/s mice) results in severe hepatic insulin resistance that is comparable to that observed in db/db mice, devoid of leptin receptor signaling. Our results demonstrate that the activation of the hypothalamic STAT3 pathway is an absolute requirement for the effects of leptin on food intake and hepatic glucose metabolism.
Introduction
The increased incidence of type 2 diabetes mellitus and of other components of the metabolic syndrome closely follows the rapid growth in the worldwide prevalence of obesity (Kopelman, 2000). This epidemiological association fuels the quest for a better understanding of the mechanisms by which defects in energy homeostasis are coupled to alterations in glucose and lipid homeostasis (Flier, 2004). The adipocyte-derived hormone leptin has pleiotropic effects that include major roles in the regulation of energy metabolism (Pelleymounter et al., 1995; Halaas et al., 1995) as well as glucose homeostasis (Liu et al., 1998; Coppari et al., 2005; Schwartz et al., 1996; Chinookoswong et al., 1999; Morton et al., 2005; Barzilai et al., 1997), lipid metabolism (Unger et al., 1999; Frederich et al., 1995; Mantzoros et al., 1998), and reproductive function (Chehab et al., 1996). Mice deficient in either leptin (ob/ob) or the leptin receptor (db/db) display hyperphagia and decreased metabolic rate, marked alterations in insulin action and lipid and carbohydrate metabolism, and infertility with hypothalamic hypogonadism (Ahima and Flier, 2000). Leptin has been shown to acutely restore the luteinizing hormone (LH) surge in estradiol- and progesterone-primed rats that are starved (Watanobe et al., 1999). This is thought to be a mechanism by which the administration of recombinant leptin improves ovulatory function in patients with hypothalamic amenorrhea (Welt et al., 2004). Given that brain-selective knockouts of the long form of the leptin receptor (LRb) faithfully replicate the metabolic as well as reproductive effects of a whole-body deficiency of leptin receptors (Cohen et al., 2001), these pleiotropic actions of leptin appear to be due to its interaction with receptors in the central nervous system (CNS).
LRb belongs to the class I family of cytokine receptors (Tartaglia et al., 1995). Binding of leptin to LRb results in the activation of Janus tyrosine kinase 2 (Jak2) and leads to the phosphorylation of cytoplasmic target proteins, including signal transducer and activator of transcription (STAT) 3 and the ras/mitogen-activated protein kinase (MAPK) (Ghilardi et al., 1996; Vaisse et al., 1996; Baumann et al., 1996).
Genetic models have provided insight into the role of neuronal STAT3 in energy balance, reproduction, and intermediate metabolism. Mice with a neuron-specific disruption of STAT3 throughout the CNS are hyperphagic, obese, diabetic, and infertile (Gao et al., 2004). Furthermore, the deletion of STAT3 via the rat insulin promoter (RIP) leads to a tissue-specific knockout of STAT3 in a subset of hypothalamic cells (in addition to pancreatic β cells), leading to impaired glucose tolerance and obesity (Cui et al., 2004; Gorogawa et al., 2004; Lee and Hennighausen, 2005). Thus, lifelong deletion of brain or hypothalamic STAT3 leads to obesity and impaired glucose tolerance. Similarly, homologous replacement of the leptin receptor in mice by a receptor mutant containing a Y1178S mutation (which abrogates STAT3 signaling) results in animals (s/s) that largely recapitulate the hyperphagia and obesity of db/db mice (Bates et al., 2003). Importantly, s/s mice exhibit improved glycemic control compared to db/db mice and display relatively normal reproductive function (Bates et al., 2005). Because leptin signaling via STAT3 is chronically obliterated, while signaling through STAT3-independent signaling pathways is intact in this model, the lower plasma glucose levels and the preserved, albeit decreased reproductive capacity in these animals presumably reflects the function of STAT3-independent pathways by leptin (Bates and Myers, 2003).
Leptin activation of other signaling molecules downstream of LRb, such as the phosphoinositide-3-kinase (PI3K) (Niswender et al., 2001; Morton et al., 2005) and central melanocortin receptors (Seeley et al., 1997), has been shown to be required for its acute effects on feeding behavior. On the other hand, despite the critical role that STAT3 plays in cytokine signal transduction and in the chronic modulation of energy homeostasis, the lack of specific inhibitors of STAT3 activation has so far hampered the investigation of its role in mediating the acute effects of leptin. The importance of understanding the role of signaling pathways under acute conditions is highlighted not only by the confounding hormonal and metabolic complications that accompany chronic leptin deficiency, but by emerging evidence for the important role of leptin and of other central pathways during pre-and early postnatal development of neural circuits (Bouret et al., 2004). These latter considerations are particularly relevant for STAT3 due to its established role in cell growth and differentiation (Hirabayashi and Gotoh, 2005). Thus, the identification of the role of STAT3 in mediating leptin’s pleiotropic actions requires molecular and biochemical strategies designed to acutely and selectively obliterate leptin signaling in adult animals.
Here, using the stereotaxic delivery of a cell-permeable phosphopeptide inhibitor of STAT3 phosphorylation/dimerization or of an adenovirus expressing a dominant-negative (DN) STAT3 mutant, we assess the contribution of the STAT3 signaling pathway to the acute effects of leptin on food intake, glucose metabolism, and gonadotropin secretion. Additionally, we directly measured the effects of insulin on glucose metabolism in conscious s/s and db/db mice by means of the insulin clamp technique. Our results identify STAT3 as essential for mediating the acute effects of leptin not only on food intake but also on gonadotropin secretion and on hepatic glucose fluxes.
Results
In vivo validation of the cell-permeable STAT3 PI
Upon leptin receptor activation, cytoplasmic STAT3 gets recruited to Tyr1138 of LRb, mediating the Jak2-dependent phosphorylation of STAT3 on Y705. This allows for the formation and nuclear translocation of STAT3 homodimers, which function as transcription factors (Figure 1A). The STAT3 peptide inhibitor (STAT3 PI) is a Src homology 2 (SH2) domain binding phosphopeptide that prevents recruitment of STAT3 to Jak2 and phosphorylation of Y705, thereby impeding the dimerization and the nuclear translocation of STAT3 (Turkson et al., 2001) (Figure 1A). Here, we demonstrate that the central administration of this STAT3 PI selectively negates leptin’s ability to phosphorylate/activate STAT3 in the mediobasal hypothalamus (MBH). A single intracerebroventricular (ICV) bolus injection of 2.5 μg of recombinant leptin in conscious rats induced the activation of STAT3 in arcuate nucleus extracts derived by micro punch biopsies (Figures 1B–1D). Infusion of the STAT3 PI (75 pmol) into the third cerebral ventricle did not alter STAT3 and MAPK under basal conditions but prevented the leptin-induced STAT3 activation at 30 min (Figure 1B) and 180 min (Figure 1C) after leptin ICV injection. The preinfusion followed by coinfusion of a scrambled sequence (control) peptide with leptin failed to alter leptin stimulation of STAT3 phosphorylation (Figure 1B). Thus, the central administration of this STAT3 PI can completely obliterate the effects of ICV leptin on STAT3, and this inhibition is sustained for at least 3 hr (Figure 1C). Importantly, the effect of leptin on the MAPK pathway did not require STAT3 activation, since the phosphorylation of extracellular signal-regulated kinases (ERKs) ERK1 and ERK2 was equally enhanced by ICV leptin in the presence of vehicle, STAT3 PI, and control peptide (Figure 1B). Leptin induces the dimerization and nuclear translocation of STAT3. Nuclear STAT3, in turn, modulates the transcription of various genes (Figure 1A). The rapid induction of the suppressor of cytokine signaling-3 (SOCS3) transcription by leptin (Bjorbaek et al., 1998) is dependent on STAT3 activation and has been used as an index of leptin’s transcriptional activity in neurons (Bjorbaek et al., 1998; Munzberg et al., 2003). In order to probe the effect of the STAT3 PI on leptin-induced transcription, we next assessed the effect of ICV leptin on SOCS3 mRNA in MBH (Figure 1D). Leptin rapidly increased MBH levels of SOCS3 mRNA by more than 3-fold, and this effect was abolished by the central administration of the STAT3 PI (Figure 1D). Collectively, these observations indicate that this experimental approach generates a rapid and selective loss of function in the signaling of leptin through the STAT3 pathway.
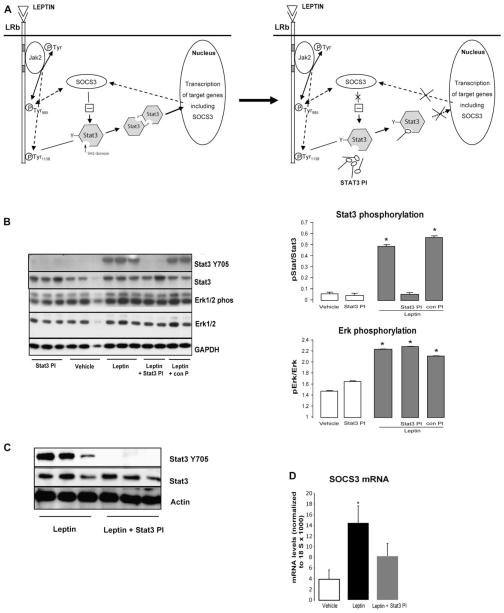
A cell-permeable SH2 domain binding phosphopeptide inhibits leptin-induced STAT3 Y705 phosphorylation in the arcuate nucleus
A) Mechanism by which the STAT3 PI inhibits STAT3 activation: upon phosphorylation of Y705, STAT3 forms homodimers and translocates into the nucleus. The phosphopeptides bind to the SH2 domain of STAT3 and inhibit the recruitment of STAT3 to Jak2 and the phosphorylation of Y705. This in turn leads to inhibition of STAT3 dimerization, nuclear translocation, and transcriptional activity. Nuclear STAT3 rapidly promotes the transcription of SOCS3.
B and C) In vivo validation of the STAT3 PI. Rats received first either vehicle or the Stat3 PI (75 pmol) as indicated. Thirty minutes later, rats received either 2.5 μg of recombinant leptin ± the STAT3 PI or the control peptide (75 pmol). Thirty minutes after leptin administration, rats were sacrificed and the arcuate nucleus of the hypothalamus was isolated by punch biopsy, homogenized, and analyzed by Western blot (B) and quantified by imagequant software. Leptin-induced STAT3 phosphorylation was prevented by coadministration of the STAT3 PI. Notably, the pre- and coadministration of the STAT3 PI does not suppress leptin-induced activation of Erk1/2. Administration of the STAT3 PI was able to inhibit STAT3 activation up to 180 min (C) after leptin administration.
D) mRNA levels of SOCS3 in the MBH 90 min after ICV injection of vehicle, leptin, or leptin plus the STAT3 PI. Leptin induces a >3-fold increase in SOCS3 mRNA levels that is prevented once the STAT3 pathway of LRb signaling is inhibited. p = 0.11 for the vehicle-treated group compared to leptin plus STAT3 PI. *p < 0.05 versus the ICV vehicle-treated group.
Leptin’s anorectic action requires central signaling through STAT3
Leptin potently regulates feeding behavior. Hyperphagia is a prominent feature of mice lacking STAT3 in neurons as well as of mice lacking leptin signaling through STAT3 (Gao et al., 2004; Bates et al., 2003). The anorectic action of leptin can be demonstrated after a single bolus injection of the hormone (Pelleymounter et al., 1995; Halaas et al., 1995). Here, we tested whether the acute effects of leptin on food intake are dependent on the central activation of STAT3. Two hours before the onset of the dark cycle, a single bolus injection of 1.5 μg of recombinant leptin was administered ICV to conscious rats (experimental protocol: Figure 2A). Leptin decreased food consumption over the following 3 hr by 63% compared with ICV injections of either the STAT3 PI alone or vehicle (Figure 2B). Leptin also decreased food consumption over the following 24 hr by 30% compared with ICV injections of either the STAT3 PI alone or vehicle (Figures 2B and 2C). The decrease in food intake was also reflected in parallel leptin-induced changes in body weight (Figure 2D). The coinfusion of the STAT3 PI with leptin negated the effects of leptin on food intake and body weight (Figures 2B–2D). Thus, the acute anorectic action of leptin requires the central activation of the STAT3 pathway.
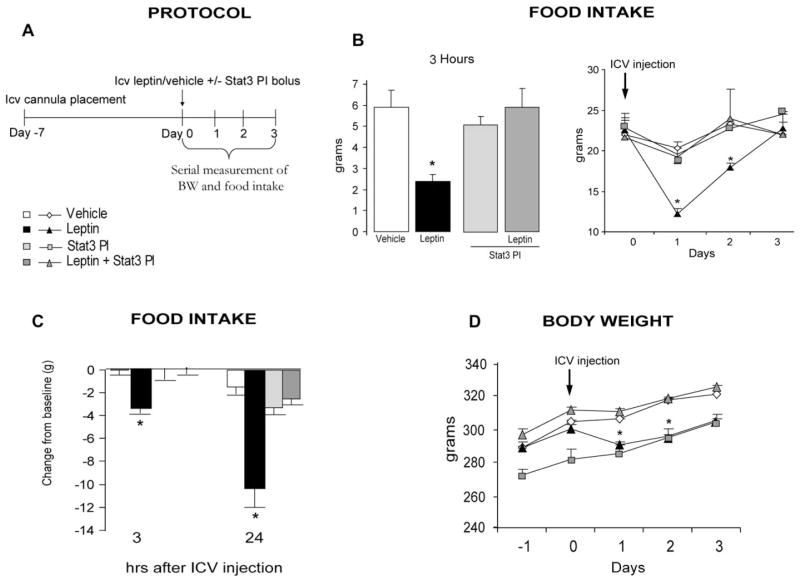
Activation of STAT3 is required for leptin’s anorectic effect
A) Experimental protocol. On day 0, rats received a single ICV injection of vehicle, 1.5 μg of leptin, 75 pmol of the STAT3 PI, or 1.5 μg of leptin plus 75 pmol of the STAT3 PI. Daily food intake and body weight were measured for 3 days.
B) Food intake at 3 hr and 1–3 days after ICV administration of the indicated substances.
C) Changes in food intake from baseline.
D) Daily body weight during the indicated treatments. *p < 0.05 versus the ICV vehicle-treated group.
Leptin’s effect on luteinizing hormone secretion in starved female rats requires central signaling through STAT3
A well-characterized acute effect of leptin on the reproductive axis is the restoration of the LH surge in food-deprived female rats (Watanobe et al., 1999; Kalra et al., 1998; Nagatani et al., 1998; Watanobe and Schioth, 2001). To test whether this acute effect of leptin on the reproductive axis is STAT3 dependent, ovariectomized female rats were food deprived for 72 hr and primed with estradiol and progesterone as described (experimental protocol: Figure 3A) (Watanobe and Schioth, 2001; Watanobe et al., 1999). Baseline LH levels were comparable in the three treatment groups (vehicle, 4.9 ng/ml; leptin, 4.5 ng/ml; leptin plus STAT3 PI, 5.1 ng/ml; p > 0.05). Serial measurements of LH from animals treated with ICV vehicle, leptin (2.5 μg/5 μl), and leptin plus the STAT3 PI (75 pmol) are depicted in Figure 3C. In total, only one out of the seven vehicle-injected rats exhibited a detectable LH surge, whereas six animals had no detectable LH surge. A single bolus of leptin administered ICV at approximately 10:00 a.m. was able to restore the LH surge in seven out of seven rats, consistent with previous reports (Watanobe and Schioth, 2001; Watanobe et al., 1999). This action of leptin was obliterated by the pre- and coadministration of the STAT3 PI in that six out of seven animals that received leptin plus the STAT3 PI had no surge. Peak LH levels were also significantly higher in the leptin-infused animals (28.6 ± 3.4 ng/ml) than in rats treated with vehicle alone (11.2 ± 3.2 ng/ml) or leptin plus STAT3 PI (12.5 ±5.5 ng/ml) (Figure 3B). Thus, the effect of leptin on the secretion of LH is dependent on the central activation of the STAT3 pathway.
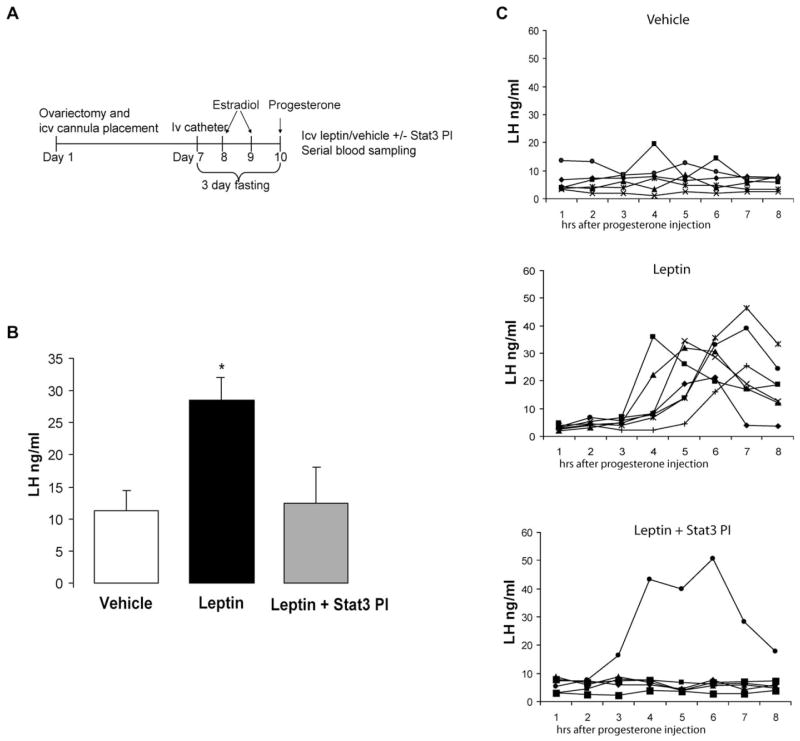
Activation of STAT3 is required for leptin-dependent restoration of LH surges in starved female rats
A) Experimental protocol. Rats underwent ovariectomy and placement of the ICV cannula on day 1. After 7 days of recovery, vascular catheters were implanted and food was removed to begin a 3 day fast. On day 8 and 9 rats were primed with estradiol, and on day 10 they were injected with progesterone to trigger a LH surge.
B) Peak LH value after progesterone injection.
C) Serial measurements of LH levels from individual animals treated with ICV vehicle (upper panel), 2.5 μg of leptin (middle panel), and leptin (2.5 μg) plus the STAT3 PI (75 pmol) (lower panel).
Leptin’s regulation of liver glucose fluxes requires central signaling through STAT3
Leptin has long been recognized to have potent effects on insulin action and on glucose metabolism that are independent of its actions on energy balance (Rossetti et al., 1997; Gutierrez-Juarez et al., 2004). Short-term overfeeding rapidly induces hepatic insulin resistance (Wang et al., 2001), and this defect is completely reversed by the acute administration of leptin into the third cerebral ventricle (Pocai et al., 2005b). Here, we ask whether this effect of central leptin on liver glucose fluxes requires intact STAT3 signaling (Figure 4A). Toward this end we used two complementary approaches designed to negate leptin activation of STAT3 in the hypothalamus: the central administration of the STAT3 PI discussed above and the adenovirus-mediated expression of a dominant-negative mutant of STAT3 (STAT3 DN) in the MBH (Figure 4A). Because leptin can acutely modulate insulin secretion (Cases et al., 2001), we tested the central STAT3-dependent effects of leptin on hepatic glucose fluxes in the presence of fixed and basal plasma insulin concentrations. Accordingly, we combined the ICV infusion of vehicle, leptin (1.5 μg/6 hr), or leptin plus the STAT3 PI (7.5 pmol) with pancreatic basal insulin (~20 μU ml−1) clamps (Figure 4B). The plasma glucose, insulin, and free fatty acid (FFA) concentrations were similar in the three groups prior to and during the clamp procedure (Table S1 in the Supplemental Data available with this article online). In the presence of basal insulin levels, negligible amounts of glucose had to be infused in rats receiving ICV vehicle. However, when leptin was given centrally a glucose infusion of ~6 mg/kg per minute was required to prevent hypoglycemia (Figure 4C). The ICV coadministration of the STAT3 PI with leptin abolished this effect of central leptin on glucose metabolism (Figure 4C). Next, we determined glucose kinetics by tracer dilution methodology in order to examine whether the STAT3-dependent effect of central leptin on glucose requirements was due to the stimulation of glucose utilization or to the inhibition of glucose production. ICV leptin failed to alter glucose utilization (Figure 4E) but markedly suppressed glucose production (by 49% ± 4.3%; Figure 4D). This effect entirely accounted for the effect of leptin on glucose infusion and was negated by the central inhibition of STAT3 activation.
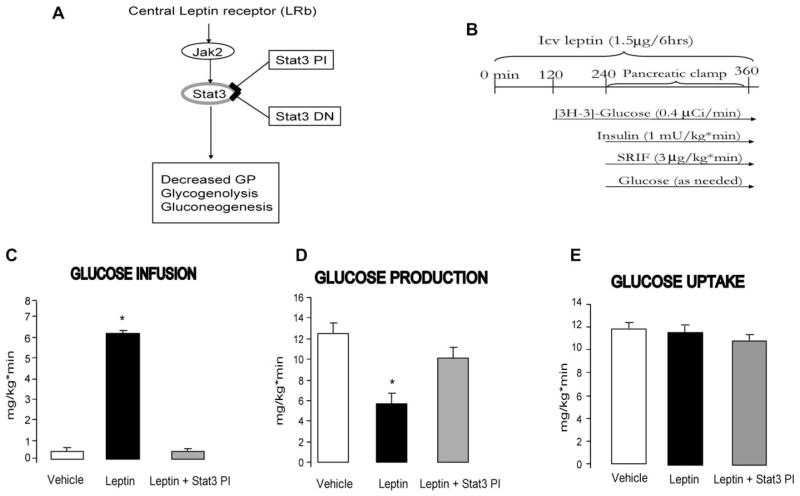
Activation of STAT3 is required for leptin regulation of glucose production
A) To acutely inhibit the STAT3 pathway of LRb signaling, we employed two approaches, the STAT3 PI and the adenovirally mediated expression of a dominant-negative mutant of STAT3 (STAT3DN).
B) Experimental protocol for the pancreatic basal insulin clamp studies in combination with ICV leptin or vehicle infusions. This protocol is common to experiments reported here with the ICV infusion of STAT3 PI and to those in rats with MBH administration of STAT3DN adenovirus (Figure S1).
C) Central administration of leptin markedly increases the rate of glucose infusion that is required to prevent hypoglycemia. This effect is negated by the central coadministration of the STAT3 PI.
D) Rate of glucose production during the clamp studies.
E) Glucose uptake. *p < 0.05 versus the ICV vehicle-treated group.
GP represents the net contribution of glucosyl units derived from gluconeogenesis and glycogenolysis. However, a portion of glucose entering the liver via phosphorylation of glucose is also a substrate for dephosphorylation via glucose-6-phosphatase (G6Pase), creating a futile cycle named glucose cycling. To further define the mechanism by which central activation of STAT3 by leptin rapidly modulates liver glucose homeostasis, we estimated the in vivo flux through G6Pase and the relative contributions of gluconeogenesis and glycogenolysis to glucose production (Table S2). ICV leptin decreased the flux through G6Pase and glucose cycling by ~50% (Figures 5A and 5B), and these effects were negated by the coinfusion of the STAT3 PI. The marked effects of ICV leptin on glucose production and G6Pase flux were accounted for by a 48% decrease in glycogenolysis (Figure 5C; 4.2 ± 0.3 versus 8.1 ± 1.0 mg/kg·min; p < 0.01) and by a 63% decrease in the rate of gluconeogenesis (Figure 5D; 1.5 ± 0.1 versus 4.1 ± 0.6 mg/kg·min; p < 0.01) when compared with the vehicle-infused group. The suppressive effects of ICV leptin on gluconeogenesis and glycogenolysis were prevented by the ICV coinfusion of the STAT3 PI. Thus, central leptin restrains hepatic glucose fluxes through its central activation of the STAT3 pathway. Leptin also markedly inhibited the hepatic expression of G6Pase and phosphoenolpyruvate carboxykinase (PEPCK) (Figures 5E and 5F). These changes in liver mRNA expression were also negated by the central coadministration of the STAT3 PI (Figures 5E and 5F).
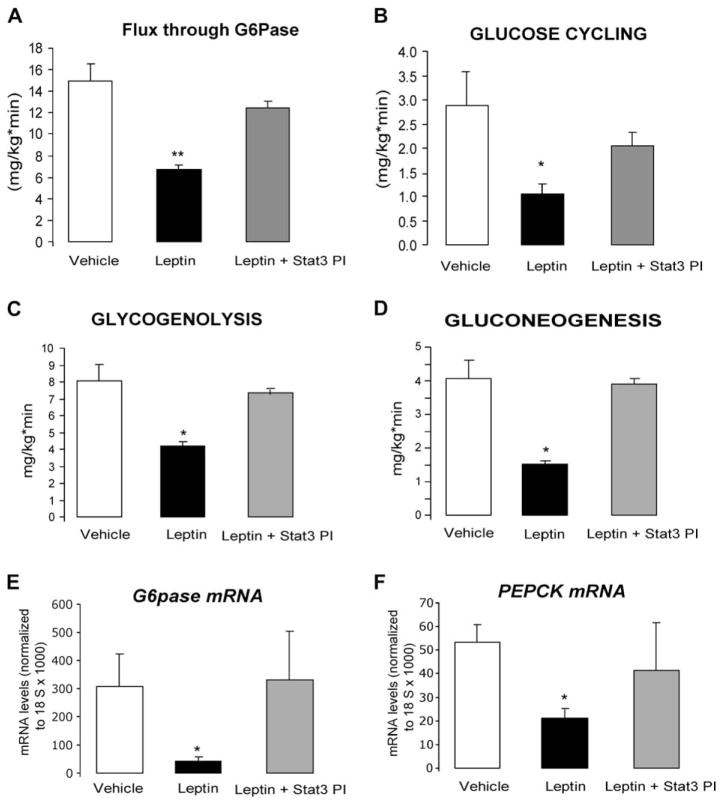
Activation of STAT3 is required for leptin regulation of liver glucose fluxes
A) Flux through G6Pase.
B) Glucose cycling.
C) Rate of glycogenolysis.
D) Rate of gluconeogenesis.
E) G6Pase mRNA.
F) PEPCK mRNA expression in livers at the end of the clamp procedure. *p < 0.05 versus the ICV vehicle-treated group.
An alternative experimental approach to induce a selective inhibition of leptin signaling through STAT3 is to infect the MBH with an adenovirus expressing STAT3 DN (Figure S1A) (Inoue et al., 2004). One week after the MBH infusion of this adenovirus, STAT3 overexpression was demonstrated in MBH extracts (Figure S1B). The expression of the STAT3 DN led to marked inhibition of leptin-induced STAT3 Y705 phosphorylation in the MBH compared to LacZ control-infected rats (Figure S1B), whereas the basal level of Stat3 Y705 phosphorylation in the MBH was not altered by Stat3 DN expression (data not shown). To verify the critical role of central STAT3 in mediating the action of leptin on glucose production, we assessed the acute effects of leptin on glucose metabolism in overfed rats 1 week after the MBH infusion of adenovirus expressing either STAT3 DN or LacZ (experimental protocol: Figure S1A). Toward this end, we combined the MBH infusion of leptin with pancreatic basal insulin (~20 μU ml−1) clamps in rats. The plasma glucose, insulin, and FFA concentrations were similar in the two groups prior to and during the clamp procedure. In the presence of basal insulin levels, central leptin markedly stimulated glucose metabolism so that exogenous glucose was required to prevent hypoglycemia in rats treated with the LacZ adenovirus (Figure S1C). This effect was entirely accounted for by a marked decrease in glucose production (Figure S1D). The expression of the STAT3 DN in the MBH abolished the effect of central leptin on glucose metabolism and on glucose production (Figure S1D). Taken together, the results obtained with STAT3 PI and STAT3 DN adenovirus demonstrate that MBH STAT3 signaling is required for leptin’s ability to suppress hepatic glucose fluxes in rats with diet-induced insulin resistance.
Severe hepatic insulin resistance is a common feature of db/db and s/s mice
Mice carrying a leptin receptor gene mutant for Tyr1138 (s/s mice) fail to mediate the activation of STAT3 by leptin (Bates et al., 2003). These s/s mice lack leptin’s ability to signal through STAT3. s/s mice pair-fed to the level of control mice since weaning do not develop hyperglycemia or glucose intolerance at least up to 8 weeks of age (Bates et al., 2005). However, the same degree of caloric restriction mitigates, but fails to normalize, glucose intolerance in 8-week-old db/db mice. These findings suggest that leptin partly controls glucose tolerance through STAT3-independent pathway(s). Here, we used a similar experimental approach in order to examine whether differences in hepatic insulin action are responsible for the improved glucose tolerance in s/s compared with db/db mice. We studied 11-week-old control, s/s, and db/db mice fed 3.8 g/day since weaning (~4-week-old) (experimental protocol: Figure 6A). Consistent with previous findings in younger mice, the average glycemia of db/db mice was significantly higher than that of s/s mice (Table S3). During the insulin clamp studies, the plasma glucagon levels as well as the increment in circulating insulin levels above basal were similar in all groups. However, despite improved glycemia, insulin clamp studies revealed severe hepatic insulin resistance in s/s mice (Figure 6B). Indeed, the degree of hepatic insulin resistance was similar in s/s and db/db mice, suggesting that the difference in glucose tolerance is due to alternative mechanisms such as subtle differences in endocrine parameters and/or in extrahepatic insulin sensitivity. In this regard, the rates of glucose infusion and glucose uptake tended to be higher in s/s compared with db/db mice (Figures 6C and 6E). Thus physiological increases in plasma insulin levels elicit similar effects in s/s mice (with intact STAT3-independent effects) and in db/db mice (with lack of both STAT3-dependent and -independent effects). This suggests that acute and chronic effects of leptin on hepatic glucose fluxes are mediated via a STAT3-dependent pathway.
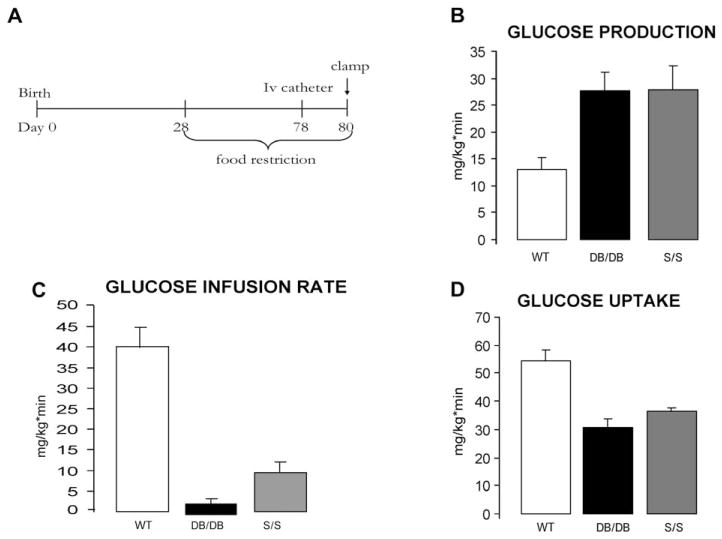
Glucose homeostasis in s/s and db/db mice
A) Experimental design for the clamp studies in wild-type (WT), db/db, and s/s mice. All mice received ~3.8 grams of chow from day 28 until clamp study.
B) Glucose infusion rate during the clamp studies in WT, s/s, and db/db mice.
C) Glucose production.
D) Glucose uptake during the clamp in WT, s/s, and db/db mice.
Discussion
Rapid loss of function in central STAT3 signaling
Leptin exerts pleiotropic actions on energy balance, glucose homeostasis, and reproduction (Ahima and Flier, 2000). Although the hypothalamic STAT3 pathway is regarded as a central mediator of leptin action, its role in the acute effects of leptin has not been addressed. Current information on the role of STAT3 in leptin action is exclusively derived from the phenotype of genetic models designed to harbor a loss of function within this signaling pathway. Because even modest changes in body composition and nutritional status can alter critical endocrine and metabolic parameters, a rapid induction of STAT3 loss of function is required in order to define the role of STAT3 in mediating these effects of leptin (Barzilai et al., 1999). Unfortunately, the lack of a specific inhibitor has so far hampered our ability to map downstream actions of leptin that are dependent on or independent of STAT3.
At the single-cell level, the functional anatomy of signal transduction has greatly benefited by the use of phosphopeptides designed to specifically and selectively inhibit a step within a signaling pathway in order to unveil its physiological role (Dunican and Doherty, 2001). Here, we validate the use of a cell-permeable phosphopeptide to block LRb-induced STAT3 activation in vivo. The combination of this approach with stereotaxic surgical techniques allowed us to pinpoint the role of hypothalamic STAT3 in several biological actions of leptin. Perhaps more importantly, the use of this and other cell-permeable inhibitor peptides should provide a formidable tool for the functional mapping of central signaling and metabolic events, and for the assessment of their impact on key behavioral and metabolic end points.
The focus of the present study is the role of STAT3 in mediating the acute effects of central leptin on feeding behavior, liver glucose metabolism, and gonadotropin secretion. To this end we selectively blocked leptin’s ability to activate STAT3 in conscious rats and then assessed the effects of central leptin on physiological end points.
Leptin and feeding behavior
Genetic evidence firmly implicates the neuronal STAT3 pathway in the regulation of food intake (Gao et al., 2004; Bates et al., 2003). In the present study, we postulated that the central activation of STAT3 by leptin would be required for its rapid action on food intake. A rapid loss of function in central STAT3 activation negated the anorectic action of leptin. Thus, the marked hyperphagia induced by lifelong obliteration of either neuronal or LRb specific STAT3 signaling is largely due to a lack of STAT3 function in the adult brain rather than to STAT3-dependent changes in the development of hypothalamic neuronal circuitries (Bates et al., 2003; Gao et al., 2004; Cui et al., 2004; Gorogawa et al., 2004).
Notably, the central inhibition of PI3K, AMP-activated protein kinase (AMPK), or melanocortin receptors also abolished the acute effects of leptin on feeding behavior. Is STAT3 upstream of these other downstream effector pathways? There is strong evidence in support of STAT3-dependent regulation of proopiomelanocortin (POMC) neurons and therefore of the central melanocortin pathway (Bates et al., 2003). Conversely, the role of STAT3 in mediating the effects of leptin on PI3K and AMPK has not been defined. Our finding suggests either that the leptin-induced activation of STAT3 is required for the activation of PI3K and for the inhibition of AMPK in the hypothalamus or that parallel signaling events play a critical permissive role in mediating the anorectic effects of leptin (Kitamura et al., 2006).
Leptin and reproductive function
Leptin is one critical indicator of nutrient/energy sufficiency that is a prerequisite for successful reproduction. In anorectic females and in some elite female athletes with extreme decreases in body adiposity, the near disappearance of leptin from the circulation is the main cause for the cessation of the menstrual cycle. This is largely due to the inadequate secretion of gonadotropins (LH and follicle stimulating hormone) by the pituitary gland (hypogonadotropic hypogonadism). Importantly, the administration of very low doses of recombinant leptin can restore the menstrual cycle without affecting food intake(Welt etal., 2004).
Neuronal deletion of STAT3 in mice leads to infertility (Gao et al., 2004). On the other hand, s/s mice display relatively normal fertility. One possible interpretation of these findings could be that hypothalamic STAT3 signaling mediated by factors other than leptin is permissive for leptin’s effects on reproductive function in normally fed animals. Our findings, however, strongly suggest that in starved females, STAT3-dependent effects in the hypothalamus are required for leptin to promote spontaneous LH surges. In s/s mice, it is possible that leptin signaling by STAT3-independent mechanisms partially restores reproductive function by permitting pulsatile gonadotropin release, resulting in low levels of estradiol that are not sufficient to support spontaneous preovulatory LH surges. These low levels of estradiol might be sufficient to permit mating to occur on occasion, and the resulting somatosensory stimulation could provoke a normal LH surge (Gibson et al., 1988). This situation might explain why s/s breeding pairs have normal litter sizes when they do reproduce but produce only half as many litters as wild-type mice.
Leptin regulation of liver glucose homeostasis
Rodents with leptin deficiency are severely resistant to the action of insulin on glucose metabolism and often present with frank diabetes. Leptin regulates glucose metabolism and insulin secretion at multiple levels through its central as well as peripheral actions. Some of the effects of leptin on glucose homeostasis are independent of its anorectic action. Among the mechanisms by which leptin improves glucose tolerance are its effects on the accumulation of triglycerides in various tissues, such as pancreas, liver, and muscle, and its regulatory control of hepatic glucose homeostasis. In this regard, leptin modulates hepatic insulin action via central melanocortin-dependent effects, leading to increases in the liver expression of G6Pase and PEPCK, in the rate of gluconeogenesis, and, via melanocortin-independent effects leading to inhibition of the liver expression of these two enzymes and of the rate of glycogenolysis (Gutierrez-Juarez et al., 2004). Importantly, leptin normalizes insulin action in a rat model in which severe hepatic insulin resistance is rapidly induced by voluntary overfeeding (Pocai et al., 2005b).
Here, we used two distinct biochemical and molecular approaches to acutely and selectively blunt the action of leptin on hypothalamic STAT3 signaling in rats with hepatic insulin resistance. These experiments indicate that the ability of central leptin to restrain hepatic glucose fluxes and G6Pase and PEPCK gene expression requires the activation of the STAT3 pathway, within the MBH. These findings are consistent with emerging evidence regarding the critical role of the arcuate nucleus of the hypothalamus in the control of glucose homeostasis by insulin (Obici et al., 2002a; Pocai et al., 2005a), nutrients (Lam et al., 2005; Obici et al., 2002b), and leptin (Morton et al., 2005; Coppari et al., 2005). As previously reported for the regulation of feeding behavior, the effects of insulin and leptin on glucose homeostasis have been shown to be dependent on the activation of the PI3K pathway within the MBH (Niswender et al., 2001). To reconcile the present findings with these previous reports, one has to postulate that either the activation of STAT3 is required for the activation of PI3K and/or that STAT3 exerts a permissive effect that is necessary for leptin modulation of glucose metabolism (Kitamura et al., 2006).
Mice with a selective deficiency in leptin signaling through STAT3 (s/s) display improved glucose tolerance compared with mice lacking LRb (db/db) (Bates et al., 2005). Here, we confirm that early caloric restriction prevents the onset of hyperglycemia in s/s mice up to 11 weeks of age, while the same degree of food restriction only ameliorates but does not normalize glycemia in db/db mice. To directly assess insulin action in these two models, we performed insulin clamp studies in conscious s/s and db/db mice. We show that db/db and s/s mice share a severe defect in insulin action on hepatic glucose fluxes that is not improved by the presence of STAT3-independent signaling in the s/s mice. These findings in mice provide further support for the notion that leptin controls hepatic glucose homeostasis through the central activation of STAT3 signaling.
We demonstrate here that a rapid loss of function in STAT3 signaling can be induced in vivo using a cell-permeable inhibitory peptide. The targeting of this peptide to discrete brain regions should provide a formidable tool for dissecting the contribution of this pathway to signal transduction and to physiological processes. Using this experimental tool, we unveil the critical role of leptin-induced activation of STAT3 in the regulation of food intake, gonadotropin secretion, and liver glucose fluxes. Since leptin deficiency or resistance leads to obesity, diabetes, and reproductive dysfunction, our findings suggest that restoration of STAT3 signaling should be a central objective of the therapy for these conditions.
Experimental procedures
Animals
Rats
Adult male (for feeding, signaling, and clamp studies) and adult female (for the LH surge experiments) Sprague-Dawley rats (Charles River Laboratories, Wilmington, Massachusetts) were studied. Rats were housed in individual cages and subjected to a standard light-dark cycle (lights on at 6 a.m.). Fourteen days before the in vivo study, indwelling catheters were placed either in the third cerebral ventricle or in the MBH by stereotaxic surgery as described (Obici et al., 2002a). The anatomical placement of the bilateral cannulae was validated via infusion of radio-labeled molecules as described (Pocai et al., 2005a), followed by anatomical sampling by micro punches. Radioactivity was recovered only in arcuate, but not in other hypothalamic areas. Following full recovery (~7 days), catheters were placed in the right internal jugular and left carotid artery. As indicated, rats were fed either a standard chow or a highly palatable high-fat diet for 3 days as described (Pocai et al., 2005b). The standard chow diet (catalog no. 5001; Purina Mills) contained 59% calories provided by carbohydrates, 20% provided by protein, and 21% by fat. The high-fat diet (catalog no. 9398; Purina Mills Ltd.) contained 45% of calories provided by carbohydrates, 22% provided by protein, and 33% provided by fat. Starch was the main carbohydrate source in both diets, whereas sucrose accounted for ~3.5%.
Mice
Mice with the Leprs1138 mutation on C57BL/6 background have been described (Bates et al., 2003). C57BL/6 db/db animals were obtained from the Jackson Laboratory (Bar Harbor, Maine). Db/db and s/s mice were pair-fed to the daily food intake of wild-type mice starting at 4 weeks of age (average of 3.8 g/day).
STAT3 PI
The sequence of the STAT3 PI was PYLKTKAAVLLPVLLAAP (where the membrane translocating sequence is underlined) as described (Turkson et al., 2001). The control peptide sequence was PLKTYKAAVLLPVLLAAP and was derived by scrambling the sequence of the STAT3 PI and fusion of this sequence to the intact membrane translocating sequence. Both peptides were synthesized by the protein facility of AECOM and HPLC purified.
Feeding studies
Rats had ICV cannulae implanted, and after complete recovery they were randomized in three groups. Two hours before onset of the dark cycle, they received a single ICV infusion of vehicle (artificial cerebrospinal fluid [aCSF]), 1.5 μg of recombinant mouse leptin, or 1.5 μg of leptin plus 75 pmol of the STAT3 PI in a total volume of 5 μl over 5 min. Food intake and body weight were monitored at the indicated time points (Figure 2A).
LH surge studies
Female Sprague-Dawley rats (220–240 g) were ovariectomized and had their third ventricle chronically cannulated under general anesthesia 7 days before the study. Indwelling jugular vein cannulae were placed 3 days before blood collection. Animals were then fasted for 3 days prior to collection of blood samples to measure LH release (Nagatani et al., 1998; Watanobe and Schioth, 2001). On the second day of fasting, all animals received estradiol benzoate (2 μg) subcutaneously daily for 2 days (Figure 3A). At about 9:00 a.m. on the day of the experiment (2 days after the first estradiol injection), 500 μg of progesterone was injected subcutaneously. Blood samples (200 μl) were collected every 60 min over a total period of 480 min (10:00 a.m.–6:00 p.m.). At 10:00 a.m. the rats received ICV either vehicle (5 μl over 5 min) or STAT3 PI (75 pmol/5 μl) followed 30 min later by an ICV injection of 2.5 μg of leptin or vehicle in a total volume of 5 μl over 5 min (Watanobe et al., 1999; Watanobe and Schioth, 2001). Hourly plasma samples were obtained and stored at −80 until further assay for LH via ELISA (Amersham, NJ).
Euglycemic/hyperinsulinemic clamp studies
Pancreatic insulin clamp studies in mice were performed as previously described to obtain physiological hyperinsulinemia (Buettner et al., 2005). Pancreatic basal insulin clamp studies in rats were performed as described (Pocai et al., 2005a).
Injection of STAT3 DN and control LacZ adenovirus
STAT3 DN adenovirus was a generous gift of Dr. Masato Kasuga and was derived as described (Inoue et al., 2004; Minami et al., 1996). LacZ and STAT3 DN adenovirus were purified by CsCl gradient centrifugation and concentrated to 1.3 × 1010 plaque-forming units per ml, corresponding to 2.5 × 1012 viral particles per ml. Rats were injected stereotaxically via the implanted cannulae into the MBH with either STAT3 DN or LacZ (1 μl = 2.5 × 10× 9 particles). Rats were analyzed by clamp studies 7 days after injection.
Real-time PCR
Real-time PCR was performed as described (Gutierrez-Juarez et al., 2004; Pocai et al., 2005c).
Signaling studies and Western blot analysis
For signaling studies, rats were injected ICV with either 5 μl of vehicle (aCSF), 75 pmol of STAT3 PI, or 75 pmol of control PI (dissolved in aCSF) over 5 min, followed 30 min later by the injection of either 2.5 μg of leptin (5 μl of 0.5 μg/μl over 5 min) or vehicle (aCSF) or 2.5 μg of leptin plus 75 pmol of STAT3 PI or control PI as indicated and sacrificed 30 min later. The arcuate nucleus was dissected by punch biopsy using a needle with 0.5 mm diameter as described (Obici et al., 2003) and homogenized in 20 mM MOPS, 2 mM EGTA, 5 mM EDTA, 30 mM sodium fluoride, 40 mM β-glycerophosphate, 10 mM sodium pyrophosphate, 2 mM orthovanadate, 0.5% NP-40, and complete protease inhibitors cocktail (Roche, Indianapolis, IN). To confirm overexpression of the adenovirally mediated expression of STAT3 DN, 7 days after infection the MBH was isolated as described (Pocai et al., 2005b). Samples were run at least twice on two separate gels for quantification purposes. Primary antibodies against phospho-Akt473, phospho-Akt308, Akt p42/44, phospho-p42/44, and STAT3 Y705 were from Cell Signaling Technology, Inc., Beverly, MA, and primary antibody against Stat 3 and goat anti-rabbit and anti-mouse HRP-conjugated secondary antibodies (Santa Cruz Biotechnology, Inc., Santa Cruz, CA) were used with ECL reagents (Perkin Elmer, Boston, MA). Membranes were stripped with the stripping agent Restore (Pierce, Rockford, IL) according to the protocol of the manufacturer and reprobed with the housekeeping genes actin (Abcam, Cambridge, MA) and GAPDH (RDI, Flanders, NJ) to ensure equal loading. Band intensity on autoradiographic Kodak MS films was quantified by application of ImageQuant software (Amersham, NJ).
Analytical procedures
The levels of glucose and FFAs, the [3H]glucose radioactivity, the rate of glucose uptake, and endogenous glucose production were calculated as previously described (Rossetti et al., 1993).
Measurement of hormones
Plasma levels of insulin, glucagons, and leptin were measured as described (Neal-Perry et al., 2005; Buettner et al., 2005).
Hepatic glucose fluxes
Hepatic glucose fluxes were measured as described (Barzilai et al., 1997).
Statistics
All values are presented as mean ± SEM. Differences were considered statistically significant at p < 0.05. Comparisons among groups were made using ANOVA followed by an unpaired Student’s t test. The study protocol was reviewed and approved by the Institutional Animal Care and Use Committee of the Albert Einstein College of Medicine.
Acknowledgments
We wish to thank Cheryl Shaw, Bing Liu, Clive Baveghems, and Stanislaw Gaweda for expert technical assistance. This work was supported by grants from the National Institutes of Health (R01-DK45024 and R37-DK48321 to L.R., R37MH41414 to A.M.E., and R01-DK57768 to M.G.M.), the American Diabetes Association (L.R. and E.D.M.), the Core laboratories of the Albert Einstein Diabetes Research and Training Center (P60-DK 20541), and the Skirball Foundation.
Footnotes
The Supplemental Data include three supplemental tables and one supplemental figure and can be found with this article online at http://www.cellmetabolism.org/cgi/content/full/4/1/49/DC1/.
References
- Ahima RS, Flier JS. Leptin. Annu Rev Physiol. 2000;62:413–437. [Abstract] [Google Scholar]
- Barzilai N, Wang J, Massilon D, Vuguin P, Hawkins M, Rossetti L. Leptin selectively decreases visceral adiposity and enhances insulin action. J Clin Invest. 1997;100:3105–3110. [Europe PMC free article] [Abstract] [Google Scholar]
- Barzilai N, She L, Liu L, Wang J, Hu M, Vuguin P, Rossetti L. Decreased visceral adiposity accounts for leptin effect on hepatic but not peripheral insulin action. Am J Physiol. 1999;277:E291–E298. [Abstract] [Google Scholar]
- Bates SH, Myers MG., Jr The role of leptin receptor signaling in feeding and neuroendocrine function. Trends Endocrinol Metab. 2003;14:447–452. [Abstract] [Google Scholar]
- Bates SH, Stearns WH, Dundon TA, Schubert M, Tso AW, Wang Y, Banks AS, Lavery HJ, Haq AK, Maratos-Flier E, et al. STAT3 signalling is required for leptin regulation of energy balance but not reproduction. Nature. 2003;421:856–859. [Abstract] [Google Scholar]
- Bates SH, Kulkarni RN, Seifert M, Myers MG., Jr Roles for leptin receptor/STAT3-dependent and -independent signals in the regulation of glucose homeostasis. Cell Metab. 2005;1:169–178. [Abstract] [Google Scholar]
- Baumann H, Morella KK, White DW, Dembski M, Bailon PS, Kim H, Lai CF, Tartaglia LA. The full-length leptin receptor has signaling capabilities of interleukin 6-type cytokine receptors. Proc Natl Acad Sci USA. 1996;93:8374–8378. [Europe PMC free article] [Abstract] [Google Scholar]
- Bjorbaek C, Elmquist JK, Frantz JD, Shoelson SE, Flier JS. Identification of SOCS-3 as a potential mediator of central leptin resistance. Mol Cell. 1998;1:619–625. [Abstract] [Google Scholar]
- Bouret SG, Draper SJ, Simerly RB. Trophic action of leptin on hypothalamic neurons that regulate feeding. Science. 2004;304:108–110. [Abstract] [Google Scholar]
- Buettner C, Patel R, Muse ED, Bhanot S, Monia BP, McKay R, Obici S, Rossetti L. Severe impairment in liver insulin signaling fails to alter hepatic insulin action in conscious mice. J Clin Invest. 2005;115:1306–1313. [Europe PMC free article] [Abstract] [Google Scholar]
- Cases JA, Gabriely I, Ma XH, Yang XM, Michaeli T, Fleischer N, Rossetti L, Barzilai N. Physiological increase in plasma leptin markedly inhibits insulin secretion in vivo. Diabetes. 2001;50:348–352. [Abstract] [Google Scholar]
- Chehab FF, Lim ME, Lu R. Correction of the sterility defect in homozygous obese female mice by treatment with the human recombinant leptin. Nat Genet. 1996;12:318–320. [Abstract] [Google Scholar]
- Chinookoswong N, Wang JL, Shi ZQ. Leptin restores euglycemia and normalizes glucose turnover in insulin-deficient diabetes in the rat. Diabetes. 1999;48:1487–1492. [Abstract] [Google Scholar]
- Cohen P, Zhao C, Cai X, Montez JM, Rohani SC, Feinstein P, Mombaerts P, Friedman JM. Selective deletion of leptin receptor in neurons leads to obesity. J Clin Invest. 2001;108:1113–1121. [Europe PMC free article] [Abstract] [Google Scholar]
- Coppari R, Ichinose M, Lee CE, Pullen AE, Kenny CD, McGovern RA, Tang V, Liu SM, Ludwig T, Chua SC, Jr, et al. The hypothalamic arcuate nucleus: A key site for mediating leptin’s effects on glucose homeostasis and locomotor activity. Cell Metab. 2005;1:63–72. [Abstract] [Google Scholar]
- Cui Y, Huang L, Elefteriou F, Yang G, Shelton JM, Giles JE, Oz OK, Pourbahrami T, Lu CY, Richardson JA, et al. Essential role of STAT3 in body weight and glucose homeostasis. Mol Cell Biol. 2004;24:258–269. [Europe PMC free article] [Abstract] [Google Scholar]
- Dunican DJ, Doherty P. Designing cell-permeant phosphopeptides to modulate intracellular signaling pathways. Biopolymers. 2001;60:45–60. [Abstract] [Google Scholar]
- Flier JS. Obesity wars: Molecular progress confronts an expanding epidemic. Cell. 2004;116:337–350. [Abstract] [Google Scholar]
- Frederich RC, Hamann A, Anderson S, Lollmann B, Lowell BB, Flier JS. Leptin levels reflect body lipid content in mice: evidence for diet-induced resistance to leptin action. Nat Med. 1995;1:1311–1314. [Abstract] [Google Scholar]
- Gao Q, Wolfgang MJ, Neschen S, Morino K, Horvath TL, Shulman GI, Fu XY. Disruption of neural signal transducer and activator of transcription 3 causes obesity, diabetes, infertility, and thermal dysregulation. Proc Natl Acad Sci USA. 2004;101:4661–4666. [Europe PMC free article] [Abstract] [Google Scholar]
- Ghilardi N, Ziegler S, Wiestner A, Stoffel R, Heim MH, Skoda RC. Defective STAT signaling by the leptin receptor in diabetic mice. Proc Natl Acad Sci USA. 1996;93:6231–6235. [Europe PMC free article] [Abstract] [Google Scholar]
- Gibson MJ, Kokoris GJ, Silverman AJ. Positive feedback in hypogonadal female mice with preoptic area brain transplants. Neuroendocrinology. 1988;48:112–119. [Abstract] [Google Scholar]
- Gorogawa S, Fujitani Y, Kaneto H, Hazama Y, Watada H, Miyamoto Y, Takeda K, Akira S, Magnuson MA, Yamasaki Y, et al. Insulin secretory defects and impaired islet architecture in pancreatic beta-cell-specific STAT3 knockout mice. Biochem Biophys Res Commun. 2004;319:1159–1170. [Abstract] [Google Scholar]
- Gutierrez-Juarez R, Obici S, Rossetti L. Melanocortin-independent effects of leptin on hepatic glucose fluxes. J Biol Chem. 2004;279:49704–49715. [Abstract] [Google Scholar]
- Halaas JL, Gajiwala KS, Maffei M, Cohen SL, Chait BT, Rabinowitz D, Lallone RL, Burley SK, Friedman JM. Weight-reducing effects of the plasma protein encoded by the obese gene. Science. 1995;269:543–546. [Abstract] [Google Scholar]
- Hirabayashi Y, Gotoh Y. Stage-dependent fate determination of neural precursor cells in mouse forebrain. Neurosci Res. 2005;51:331–336. [Abstract] [Google Scholar]
- Inoue H, Ogawa W, Ozaki M, Haga S, Matsumoto M, Furukawa K, Hashimoto N, Kido Y, Mori T, Sakaue H, et al. Role of STAT-3 in regulation of hepatic gluconeogenic genes and carbohydrate metabolism in vivo. Nat Med. 2004;10:168–174. [Abstract] [Google Scholar]
- Kalra SP, Xu B, Dube MG, Moldawer LL, Martin D, Kalra PS. Leptin and ciliary neurotropic factor (CNTF) inhibit fasting-induced suppression of luteinizing hormone release in rats: role of neuropeptide Y. Neurosci Lett. 1998;240:45–49. [Abstract] [Google Scholar]
- Kitamura T, Feng Y, Kitamura YI, Chua S, Xu AW, Barsh G, Rossetti L, Accili D. Forkhead protein FoxO1 mediates Agrp-dependent effects of leptin on food intake. Nat Med. 2006;12:534–540. 10.1038/nm1392. Published online April 9, 2006. [Abstract] [CrossRef] [Google Scholar]
- Kopelman PG. Obesity as a medical problem. Nature. 2000;404:635–643. [Abstract] [Google Scholar]
- Lam TK, Pocai A, Gutierrez-Juarez R, Obici S, Bryan J, Aguilar-Bryan L, Schwartz GJ, Rossetti L. Hypothalamic sensing of circulating fatty acids is required for glucose homeostasis. Nat Med. 2005;11:320–327. [Abstract] [Google Scholar]
- Lee JY, Hennighausen L. The transcription factor Stat3 is dispensable for pancreatic beta-cell development and function. Biochem Biophys Res Commun. 2005;334:764–768. [Abstract] [Google Scholar]
- Liu L, Karkanias GB, Morales JC, Hawkins M, Barzilai N, Wang J, Rossetti L. Intracerebroventricular leptin regulates hepatic but not peripheral glucose fluxes. J Biol Chem. 1998;273:31160–31167. [Abstract] [Google Scholar]
- Mantzoros CS, Frederich RC, Qu D, Lowell BB, Maratos-Flier E, Flier JS. Severe leptin resistance in brown fat-deficient uncoupling protein promoter-driven diphtheria toxin A mice despite suppression of hypothalamic neuropeptide Y and circulating corticosterone concentrations. Diabetes. 1998;47:230–238. [Abstract] [Google Scholar]
- Minami M, Inoue M, Wei S, Takeda K, Matsumoto M, Kishimoto T, Akira S. STAT3 activation is a critical step in gp130-mediated terminal differentiation and growth arrest of a myeloid cell line. Proc Natl Acad Sci USA. 1996;93:3963–3966. [Europe PMC free article] [Abstract] [Google Scholar]
- Morton GJ, Gelling RW, Niswender KD, Morrison CD, Rhodes CJ, Schwartz MW. Leptin regulates insulin sensitivity via phosphatidylinositol-3-OH kinase signaling in mediobasal hypothalamic neurons. Cell Metab. 2005;2:411–420. [Abstract] [Google Scholar]
- Munzberg H, Huo L, Nillni EA, Hollenberg AN, Bjorbaek C. Role of signal transducer and activator of transcription 3 in regulation of hypothalamic proopiomelanocortin gene expression by leptin. Endocrinology. 2003;144:2121–2131. [Abstract] [Google Scholar]
- Nagatani S, Guthikonda P, Thompson RC, Tsukamura H, Maeda KI, Foster DL. Evidence for GnRH regulation by leptin: leptin administration prevents reduced pulsatile LH secretion during fasting. Neuroendocrinology. 1998;67:370–376. [Abstract] [Google Scholar]
- Neal-Perry GS, Zeevalk GD, Santoro NF, Etgen AM. Attenuation of preoptic area glutamate release correlates with reduced luteinizing hormone secretion in middle-aged female rats. Endocrinology. 2005;146:4331–4339. [Abstract] [Google Scholar]
- Niswender KD, Morton GJ, Stearns WH, Rhodes CJ, Myers MG, Jr, Schwartz MW. Intracellular signalling. Key enzyme in leptin-induced anorexia. Nature. 2001;413:794–795. [Abstract] [Google Scholar]
- Obici S, Feng Z, Karkanias G, Baskin DG, Rossetti L. Decreasing hypothalamic insulin receptors causes hyperphagia and insulin resistance in rats. Nat Neurosci. 2002a;5:566–572. [Abstract] [Google Scholar]
- Obici S, Feng Z, Morgan K, Stein D, Karkanias G, Rossetti L. Central administration of oleic acid inhibits glucose production and food intake. Diabetes. 2002b;51:271–275. [Abstract] [Google Scholar]
- Obici S, Feng Z, Arduini A, Conti R, Rossetti L. Inhibition of hypothalamic carnitine palmitoyltransferase-1 decreases food intake and glucose production. Nat Med. 2003;9:756–761. [Abstract] [Google Scholar]
- Pelleymounter MA, Cullen MJ, Baker MB, Hecht R, Winters D, Boone T, Collins F. Effects of the obese gene product on body weight regulation in ob/ob mice. Science. 1995;269:540–543. [Abstract] [Google Scholar]
- Pocai A, Lam TK, Gutierrez-Juarez R, Obici S, Schwartz GJ, Bryan J, Aguilar-Bryan L, Rossetti L. Hypothalamic K(ATP) channels control hepatic glucose production. Nature. 2005a;434:1026–1031. [Abstract] [Google Scholar]
- Pocai A, Morgan K, Buettner C, Gutierrez-Juarez R, Obici S, Rossetti L. Central leptin acutely reverses diet-induced hepatic insulin resistance. Diabetes. 2005b;54:3182–3189. [Abstract] [Google Scholar]
- Pocai A, Obici S, Schwartz GJ, Rossetti L. A brain-liver circuit regulates glucose homeostasis. Cell Metab. 2005c;1:53–61. [Abstract] [Google Scholar]
- Rossetti L, Giaccari A, Barzilai N, Howard K, Sebel G, Hu M. Mechanism by which hyperglycemia inhibits hepatic glucose production in conscious rats. Implications for the pathophysiology of fasting hyperglycemia in diabetes. J Clin Invest. 1993;92:1126–1134. [Europe PMC free article] [Abstract] [Google Scholar]
- Rossetti L, Massillon D, Barzilai N, Vuguin P, Chen W, Hawkins M, Wu J, Wang J. Short term effects of leptin on hepatic gluconeogenesis and in vivo insulin action. J Biol Chem. 1997;272:27758–27763. [Abstract] [Google Scholar]
- Schwartz MW, Baskin DG, Bukowski TR, Kuijper JL, Foster D, Lasser G, Prunkard DE, Porte D, Jr, Woods SC, Seeley RJ, Weigle DS. Specificity of leptin action on elevated blood glucose levels and hypothalamic neuropeptide Y gene expression in ob/ob mice. Diabetes. 1996;45:531–535. [Abstract] [Google Scholar]
- Seeley RJ, Yagaloff KA, Fisher SL, Burn P, Thiele TE, van Dijk G, Baskin DG, Schwartz MW. Melanocortin receptors in leptin effects. Nature. 1997;390:349. [Abstract] [Google Scholar]
- Tartaglia LA, Dembski M, Weng X, Deng N, Culpepper J, Devos R, Richards GJ, Campfield LA, Clark FT, Deeds J, et al. Identification and expression cloning of a leptin receptor, OB-R. Cell. 1995;83:1263–1271. [Abstract] [Google Scholar]
- Turkson J, Ryan D, Kim JS, Zhang Y, Chen Z, Haura E, Laudano A, Sebti S, Hamilton AD, Jove R. Phosphotyrosyl peptides block Stat3-mediated DNA binding activity, gene regulation, and cell transformation. J Biol Chem. 2001;276:45443–45455. [Abstract] [Google Scholar]
- Unger RH, Zhou YT, Orci L. Regulation of fatty acid homeostasis in cells: novel role of leptin. Proc Natl Acad Sci USA. 1999;96:2327–2332. [Europe PMC free article] [Abstract] [Google Scholar]
- Vaisse C, Halaas JL, Horvath CM, Darnell JE, Jr, Stoffel M, Friedman JM. Leptin activation of Stat3 in the hypothalamus of wild-type and ob/ob mice but not db/db mice. Nat Genet. 1996;14:95–97. [Abstract] [Google Scholar]
- Wang J, Obici S, Morgan K, Barzilai N, Feng Z, Rossetti L. Overfeeding rapidly induces leptin and insulin resistance. Diabetes. 2001;50:2786–2791. [Abstract] [Google Scholar]
- Watanobe H, Schioth HB. Nitric oxide mediates leptin-induced preovulatory luteinizing hormone and prolactin surges in rats. Brain Res. 2001;923:193–197. [Abstract] [Google Scholar]
- Watanobe H, Suda T, Wikberg JE, Schioth HB. Evidence that physiological levels of circulating leptin exert a stimulatory effect on luteinizing hormone and prolactin surges in rats. Biochem Biophys Res Commun. 1999;263:162–165. [Abstract] [Google Scholar]
- Welt CK, Chan JL, Bullen J, Murphy R, Smith P, DePaoli AM, Karalis A, Mantzoros CS. Recombinant human leptin in women with hypothalamic amenorrhea. N Engl J Med. 2004;351:987–997. [Abstract] [Google Scholar]
Full text links
Read article at publisher's site: https://doi.org/10.1016/j.cmet.2006.04.014
Read article for free, from open access legal sources, via Unpaywall:
http://www.cell.com/article/S1550413106001549/pdf
Citations & impact
Impact metrics
Citations of article over time
Smart citations by scite.ai
Explore citation contexts and check if this article has been
supported or disputed.
https://scite.ai/reports/10.1016/j.cmet.2006.04.014
Article citations
Leptin-activated hypothalamic BNC2 neurons acutely suppress food intake.
Nature, 30 Oct 2024
Cited by: 0 articles | PMID: 39478220
Circulating extracellular vesicle-derived miR-1299 disrupts hepatic glucose homeostasis by targeting the STAT3/FAM3A axis in gestational diabetes mellitus.
J Nanobiotechnology, 22(1):509, 24 Aug 2024
Cited by: 0 articles | PMID: 39182087 | PMCID: PMC11344378
Regulation of Energy and Glucose Homeostasis by the Nucleus of the Solitary Tract and the Area Postrema.
Endocrinol Metab (Seoul), 39(4):559-568, 01 Aug 2024
Cited by: 0 articles | PMID: 39086274 | PMCID: PMC11377841
Review Free full text in Europe PMC
Selective Neuronal Knockout of STAT3 Function Inhibits Epilepsy Progression, Improves Cognition, and Restores Dysregulated Gene Networks in a Temporal Lobe Epilepsy Model.
Ann Neurol, 94(1):106-122, 07 Apr 2023
Cited by: 5 articles | PMID: 36935347 | PMCID: PMC10313781
Astragalus mongholicus powder, a traditional Chinese medicine formula ameliorate type 2 diabetes by regulating adipoinsular axis in diabetic mice.
Front Pharmacol, 13:973927, 15 Aug 2022
Cited by: 2 articles | PMID: 36046814 | PMCID: PMC9420938
Go to all (142) article citations
Data
Data behind the article
This data has been text mined from the article, or deposited into data resources.
BioStudies: supplemental material and supporting data
Similar Articles
To arrive at the top five similar articles we use a word-weighted algorithm to compare words from the Title and Abstract of each citation.
Anorectic estrogen mimics leptin's effect on the rewiring of melanocortin cells and Stat3 signaling in obese animals.
Nat Med, 13(1):89-94, 31 Dec 2006
Cited by: 271 articles | PMID: 17195839
Palmitic acid induces central leptin resistance and impairs hepatic glucose and lipid metabolism in male mice.
J Nutr Biochem, 26(5):541-548, 12 Feb 2015
Cited by: 36 articles | PMID: 25724108
Leptin-dependent STAT3 phosphorylation in postnatal mouse hypothalamus.
Brain Res, 1215:105-115, 10 Apr 2008
Cited by: 37 articles | PMID: 18485333
Leptin Signaling in the Control of Metabolism and Appetite: Lessons from Animal Models.
J Mol Neurosci, 66(3):390-402, 03 Oct 2018
Cited by: 25 articles | PMID: 30284225
Review
Funding
Funders who supported this work.
NIDDK NIH HHS (9)
Grant ID: R01-DK57768
Grant ID: R37-DK48321
Grant ID: R01 DK048321
Grant ID: R01-DK45024
Grant ID: R01 DK057768
Grant ID: R37 DK048321
Grant ID: P60-DK20541
Grant ID: R01 DK045024
Grant ID: P60 DK020541
NIMH NIH HHS (2)
Grant ID: R37 MH041414
Grant ID: R37MH41414