Abstract
Free full text

Aire-expressing thymic medullary epithelial cells originate from β5t-expressing progenitor cells
Associated Data
Abstract
The thymus provides multiple microenvironments that are essential for the development and repertoire selection of T lymphocytes. The thymic cortex induces the generation and positive selection of T lymphocytes, whereas the thymic medulla establishes self-tolerance among the positively selected T lymphocytes. Cortical thymic epithelial cells (cTECs) and medullary TECs (mTECs) constitute the major stromal cells that structurally form and functionally characterize the cortex and the medulla, respectively. cTECs and mTECs are both derived from the endodermal epithelium of the third pharyngeal pouch. However, the molecular and cellular characteristics of the progenitor cells for the distinct TEC lineages are unclear. Here we report the preparation and characterization of mice that express the recombinase Cre instead of β5t, a proteasome subunit that is abundant in cTECs and not detected in other cell types, including mTECs. By crossing β5t-Cre knock-in mice with loxP-dependent GFP reporter mice, we found that β5t-Cre–mediated recombination occurs specifically in TECs but not in any other cell types in the mouse. Surprisingly, in addition to cTECs, β5t-Cre-loxP–mediated GFP expression was detected in almost all mTECs. These results indicate that the majority of mTECs, including autoimmune regulator-expressing mTECs, are derived from β5t-expressing progenitor cells.
Thymic epithelial cells (TECs) are derived from the endodermal epithelium of the third pharyngeal pouch (1–3). Cortical TECs (cTECs) provide a microenvironment that induces the generation of T cells and the positive selection of functionally competent T cells, whereas medullary TECs (mTECs) essentially contribute to the establishment of self-tolerance by the deletion of self-reactive T cells and the generation of regulatory T cells (4, 5). The nuclear protein Autoimmune regulator (Aire) expressed by a subpopulation of mTECs is essential, especially during the perinatal period, for the establishment of self-tolerance in T cells (6, 7). Although the importance of the forkhead transcription factor Foxn1 for the development of both cTECs and mTECs has been established (2, 8), it remains unknown how the endodermal epithelium of the third pharyngeal pouch gives rise to cTECs and mTECs. In particular, the molecular and cellular mechanisms underlying the separate development of the cTEC and mTEC lineages remain unclear.
We previously reported β5t, a proteasome subunit expressed in cTECs (9, 10). β5t is pivotal for the positive selection of immunocompetent CD8+ T cells (11, 12). β5t mRNA and protein are prominently expressed in cTECs and not detected in other cell types, including mTECs (10). To examine whether β5t-expressing cells could contribute to the development of cells other than cTECs, we engineered mice in which the β5t-encoding genomic sequence was replaced with the sequence that encodes the loxP-specific recombinase Cre. Analyzing mice that are crossed to carry the β5t-Cre knock-in allele and the loxP-dependent GFP reporter allele, we demonstrate that β5t-Cre-loxP–mediated GFP expression is detected in practically all cTECs and, surprisingly, in almost all mTECs, including Aire+ mTECs, but not in any other cell types in the body. The results indicate that β5t expression is highly specific for TECs and that in addition to mature cTECs, β5t is expressed in TEC progenitor cells that give rise to mTECs, including Aire+ mTECs.
Results
Generation of β5t-Cre Knock-In Mice.
We produced β5t-Cre knock-in mice by homologous recombination in embryonic stem cells using a gene-targeting vector in that the sole exon sequence encoding the β5t gene was replaced with a sequence encoding the codon-improved Cre recombinase (Fig. S1). Homologous recombination with the targeting vector was confirmed by Southern blot analysis, in which a 5.6-kb BamHI fragment appeared after hybridization with a 3′ probe (Fig. S1 A and B) and by PCR analysis of genomic DNA through amplification of a recombination-specific 765-bp fragment (Fig. S1 A and C). Because the β5t-Cre knock-in allele lacks the sequence encoding β5t protein (Fig. S1A), mice homozygous for the β5t-Cre knock-in alleles were deficient in functional β5t protein and therefore defective in the generation of CD8+ T cells (Fig. 1A). Identical to previously reported β5t-deficient mice (9, 11), homozygous β5t-Cre knock-in mice were defective in the generation of ~80% of CD4−CD8+ T cell antigen receptor (TCR)high mature thymocytes but were comparable to normal mice in the generation of CD4−CD8− and CD4+CD8+ immature thymocytes as well as CD4+CD8− TCRhigh mature thymocytes (Fig. 1A). In contrast, mice heterozygous for the β5t-Cre knock-in allele showed no alterations or defects in thymocyte cellularity (Fig. 1A), in agreement with the normal T-cell development detected in β5t+/− heterozygous mice previously produced (9, 11). These results indicate that homologous recombination of the genome was successfully engineered to knock in the β5t-Cre allele, as designed in Fig. S1A. Because homozygous β5t-Cre knock-in mice lacked β5t protein expression and hence were defective in the generation of CD8+ T cells, the remainder of this article describes the results obtained from mice heterozygous for the β5t-Cre knock-in allele.
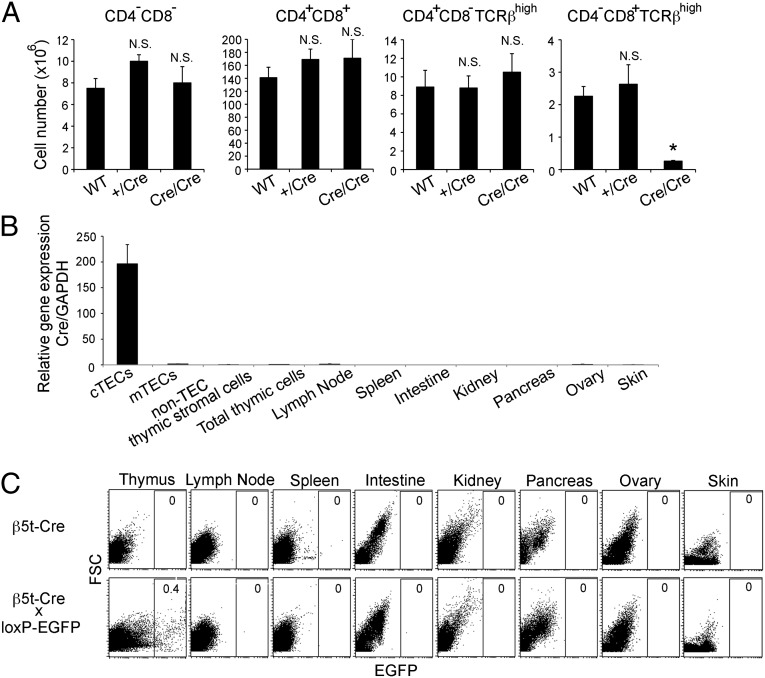
β5t-Cre knock-in mice. (A) Numbers (means and SEs, n = 3) of CD4−CD8−, CD4+CD8+, CD4+CD8− TCRβhigh, and CD4−CD8+ TCRβhigh thymocytes in 4-wk-old WT (+/+) and β5t-Cre knock-in heterozygous (+/Cre) and homozygous (Cre/Cre) mice. *P < 0.005; N.S., not significant. (B) Relative mRNA expression of Cre in indicated cells and organs isolated from 4- to 5-wk-old β5t-Cre knock-in heterozygous mice. The expression levels (means and SEs, n = 3) of Cre measured by quantitative RT-PCR were normalized to that of GAPDH and compared with the level measured in total thymic cells. (C) Representative flow cytometry profiles of forward scatter intensity and EGFP expression in collagenase-digested cells obtained from indicated tissues of 4- to 5-wk-old β5t-Cre knock-in mice and β5t-Cre x loxP-EGFP mice. Numbers indicate frequency of EGFP+ cells in total cells. n = 3.
We first examined the tissue specificity of Cre expression in β5t-Cre knock-in mice. In agreement with the highly specific expression of β5t gene in cTECs (9, 10), Cre mRNA expression in β5t-Cre knock-in mice was specifically detected in cTECs but not in other thymic cell types, including mTECs and a population of non-TEC thymic stromal cells (containing fibroblasts and endothelial cells) (Fig. 1B). All other tissues tested failed to express Cre mRNA (Fig. 1B); thus, the specificity of Cre expression in β5t-Cre knock-in mice faithfully reproduces the expression specificity of β5t.
β5t-Cre-loxP–Mediated GFP Expression Is Specifically Detected in TECs.
To trace the developmental potential of β5t-expressing cells in vivo, β5t-Cre knock-in mice were crossed with CAG-loxP-stop-loxP-EGFP-transgenic (loxP-EGFP) mice, in which EGFP would be ubiquitously expressed under the control of the CAG promoter only when the loxP-flanked stop sequences had been excised by Cre recombinase (13). In β5t-Cre × loxP-EGFP mice, EGFP reporter expression specifically visualizes cells that either express β5t presently or expressed β5t during their development. We initially examined EGFP expression in adult β5t-Cre × loxP-EGFP mice by flow cytometric analysis of collagenase-digested cells isolated from various organs. We found that EGFP+ cells were detected only in the thymus but not in any other organs (Fig. 1C). The thymus-specific EGFP expression in β5t-Cre × loxP-EGFP mice was confirmed by macroscopic fluorescence observation using a transilluminator and by fluorescence microscopy analysis of tissue sections. Within collagenase-digested thymic cells, EGFP+ cells were clearly detected in CD326 [epithelial cell adhesion molecule (EpCAM)]+ TECs but not in CD326− non-TECs, indicating that β5t-Cre-loxP–mediated EGFP is specifically expressed in TECs (Fig. 2A). Immunofluorescence analysis of thymic sections prepared from adult β5t-Venus knock-in mice confirmed that only the cortex but not the medulla expressed the Venus fluorescence (Fig. 2B). In striking contrast, EGFP expression in the adult thymus of β5t-Cre × loxP-EGFP mice was detected throughout the thymus (Fig. 2B). Specifically, the EGFP signals not only identified CD249 (Ly51)+ cTECs localized in the thymic cortex but also keratin 14 (K14)+ mTECs and keratin 5 (K5)+ mTECs localized in the medulla (Fig. 2B). These results indicate that β5t-Cre-loxP–mediated EGFP expression is specifically detected in TECs, and surprisingly, in both cTECs and mTECs.
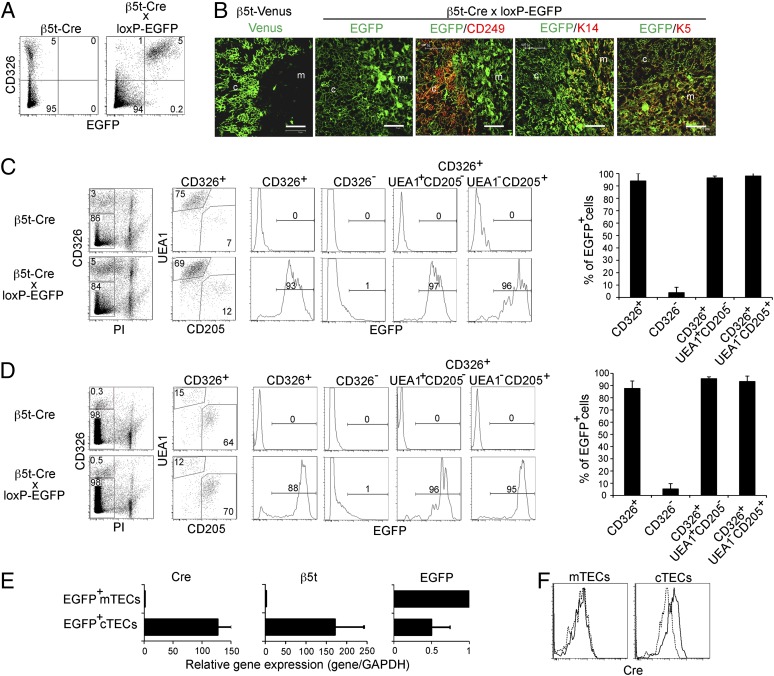
β5t-Cre-loxP–mediated GFP expression is detected in mTECs and cTECs. (A) Representative flow cytometry profiles of CD326 and EGFP expression in collagenase-digested thymic cells from 6- to 7-wk-old mice. Thymocytes were depleted from the thymic cells by using magnetic bead–conjugated anti-CD45 antibody. Numbers indicate frequency of cells within indicated areas. n = 3. (B) Cryosections of the thymus from 6- to 7-wk-old β5t-Venus mice and β5t-Cre × loxP-EGFP mice were analyzed by confocal microscopy. Venus expression and EGFP expression are shown in green. Where indicated, the sections were also stained for CD249 (Ly51), keratin 14 (K14), or keratin 5 (K5) (red). c, cortex; m, medulla. (Scale bar: 75 μm.) Representative results are shown (n = 3). (C and D) Collagenase-digested thymic cells from indicated mice were stained with CD326 antibody and propidium iodide (PI) to identify CD326+PI− viable TECs (Left). EGFP expression on CD326+ TECs, CD326− non-TEC thymic cells, CD326+UEA1+CD205− mTECs, and CD326+UEA1−CD205+ cTECs from 6- to 7-wk-old (C) and 0-d-old (D) mice (Center). Numbers in dot plots and histograms indicate frequency within indicated area. Frequencies (means and SEs, n = 3) of EGFP+ cells within indicated cell populations (Right). (E) The amounts of Cre, β5t, and EGFP mRNAs in EGFP+CD326+UEA1+CD205− mTECs and EGFP+CD326+UEA1−CD205+ cTECs obtained from 2- to 3-wk-old β5t-Cre × loxP-EGFP mice were measured by quantitative RT-PCR analysis and were normalized to the amount of GAPDH mRNAs. Data represent means and SEs of three independent measurements. (F) Flow cytometric analysis of Cre expression in CD45−CD326+CD80+CD249− mTECs and CD45−CD326+CD80−CD249+ cTECs from 1-wk-old β5t-Cre mice (solid line) or WT mice (dashed line). Data are representative of three independent experiments.
β5t-Cre-loxP–Mediated GFP Expression Is Detected in the Majority of mTECs and cTECs.
The histological analysis (Fig. 2B) indicated that large proportions of mTECs and cTECs expressed β5t-Cre-loxP–mediated EGFP. To measure the frequency of β5t-Cre-loxP–mediated EGFP expression in cTECs and mTECs, collagenase-digested thymic cells from β5t-Cre × loxP-EGFP mice were multicolor stained for cell-surface markers of cTECs and mTECs. The cells were also stained with propidium iodide to distinguish between viable and dead cells. Analyzed by flow cytometry, EGFP+ viable cells were detected among TECs (i.e., CD326+ cells) but not CD326− non-TEC thymic cells from both adult (Fig. 2C) and newborn (Fig. 2D) β5t-Cre × loxP-EGFP mice but not β5t-Cre mice, confirming our previous results (Fig. 2A). It was further found that EGFP expression was detected in the majority of both CD326+Ulex europaeus agglutinin 1 (UEA1)+CD205− mTECs and CD326+UEA1−CD205+ cTECs (95 ± 3% in mTECs and 98 ± 2% in cTECs in adult thymus, n = 3; and 96 ± 1% in mTECs and 93 ± 4% in cTECs in newborn thymus, n = 3) (Fig. 2 C and D). These results indicate that the majority of mTECs and cTECs in newborn and adult mice exhibit a developmental signature that indicates their current or previous expression of β5t. Because most cTECs are positive for β5t protein (10), β5t-Cre-loxP–mediated EGFP detected in cTECs likely reflects the present expression of β5t in these cells. On the other hand, because β5t protein expression is not detected in mTECs (10), the detection of EGFP in the majority of mTECs from β5t-Cre × loxP-EGFP mice must reflect a previous expression of the β5t gene during development. In keeping with this interpretation, mRNA specific for EGFP but for neither Cre nor β5t was robustly detected in EGFP+CD326+UEA1+CD205− mTECs isolated from β5t-Cre × loxP-EGFP mice, whereas EGFP+CD326+UEA1−CD205+ cTECs isolated from the same mice concurrently expressed mRNAs specific for EGFP, Cre, and β5t (Fig. 2E). Moreover, Cre protein expression was specifically detected in cTECs but not in mTECs (Fig. 2F). These results indicate that the majority of mTECs in newborn and adult mice must have previously expressed the β5t gene and that most mTECs must therefore originate from cells that express β5t.
To independently confirm our results, we crossed the β5t-Cre-knock-in mice to a separate reporter mouse strain that carried the CAG-loxP-stop-loxP-Zoanthus green (ZsGreen) gene knocked into the Rosa26 locus (14). To ensure that the Cre-mediated recombination in mTECs was not an artifactual consequence of the engineered β5t locus leaving the neo selection cassette in place, the phosphoglycerate kinase (PGK)-neo sequence was removed by crossing β5t-Cre mice to Flippase (Flp)-deleter animals before breeding to loxP-ZsGreen mice (Fig. S1A). On analysis of the resultant β5t-Cre × loxP-ZsGreen mice, we found that ZsGreen was expressed in >99.5% of CD326+UEA1−CD249+ cTECs and in >99.5% of CD326+UEA1+CD249− mTECs of the adult thymus (Fig. 3A). Moreover, β5t-Cre × loxP-ZsGreen mice displayed normal TEC frequencies and a normal distribution of cTECs and mTECs compared with control loxP-ZsGreen littermates (Fig. 3A). Both intrathymic T-cell development and total thymus cellularity were comparable between β5t-Cre × loxP-ZsGreen mice and control loxP-ZsGreen mice (Fig. 3 B and C). Thus, the expression of either Cre or ZsGreen did not influence TEC viability and function. Immunofluorescence analysis of the thymus tissue sections demonstrated ZsGreen expression that identified most β5t protein–expressing cTECs and K14-expressing mTECs to be positive for ZsGreen (Fig. 3D). These results confirm that the β5t-Cre-knock-in locus allows faithful monitoring of present and past β5t expression and that almost all mTECs are derived from cells that previously expressed β5t.
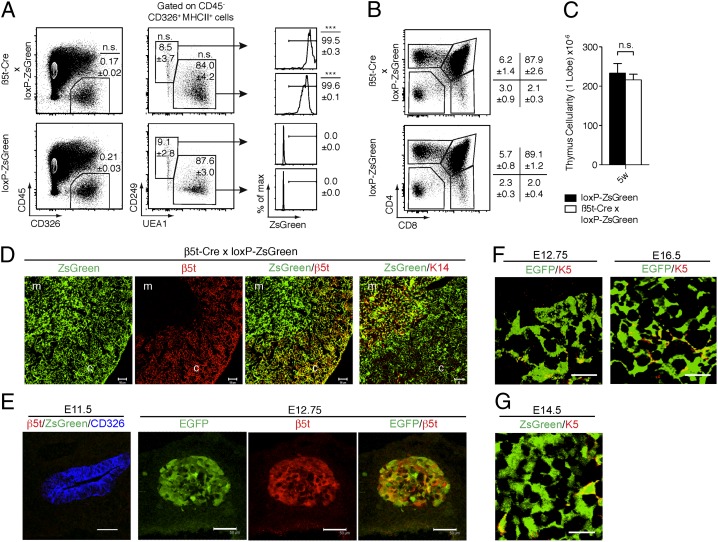
β5t-Cre-knock-in locus allows faithful monitoring of present and past β5t expression. (A) Flow cytometric analysis of CD45− CD326+ MHC class II+ TEC subpopulations from 6-wk-old β5t-Cre × loxP-ZsGreen and loxP-ZsGreen mice. Relative frequencies (means and SEs, n = 3) of all TECs, UEA1−CD249+ cTECs, and UEA1+CD249− mTECs are indicated in dot plots. Histograms display frequencies (means and SEs, n = 3) of ZsGreen-positive cTECs and mTECs. ***P < 0.001; n.s., not significant (comparison between β5t-Cre × loxP-ZsGreen and loxP-ZsGreen cells). (B) Flow cytometric analysis of thymocytes for the cell surface expression of CD4 and CD8. The cells were isolated from 6-wk-old loxP-ZsGreen and β5t-Cre × loxP-ZsGreen mice. Numbers denote frequency of cells within indicated gates (means and SEs, n = 3). (C) Total cellularity (means and SEs, n = 3) of the thymus in 5-wk-old loxP-ZsGreen (black bar) and β5t-Cre x loxP-ZsGreen (white bar) mice. n.s., not significant. (D) Immunofluorescence analysis of thymus tissues from 6-wk-old β5t-Cre x loxP-ZsGreen mice for the expression of ZsGreen (green), β5t (identifying cTEC, red) and K14 (detecting mTECs, red). Data are representative of at least two separate experiments using two mice each. (Scale bar: 50 μm.) c, cortex; m, medulla. (E) E11.5 embryos of β5t-Cre × loxP-ZsGreen mice and E12.75 embryos of β5t-Cre × loxP-EGFP mice were stained with anti-β5t antibody. ZsGreen or EGFP fluorescence (green) and anti-β5t immunofluorescence (red) in the pharyngeal region containing the thymic primordium were measured. Where indicated, the sections were additionally stained with anti-CD326 antibody (blue). Shown are representative results of three independent experiments. (Scale bar: 50 μm.) (F) E12.75 embryos and E16.5 embryonic thymuses of β5t-Cre × loxP-EGFP mice were stained with anti-K5 antibody. Shown are representative results (n = 3) of EGFP fluorescence (green) and anti-K5 immunofluorescence (red) of the thymic primordium (E12.75) and the thymus (E16.5). (Scale bar: 20 μm.) (G) E14.5 embryonic thymuses of β5t-Cre × loxP-ZsGreen mice were stained with anti-K5 antibody. Representative results of ZsGreen fluorescence (green) and anti-K5 immunofluorescence (red) are shown (n = 2). (Scale bar: 20 μm.)
A small subpopulation (~5%) of mTECs did not express β5t-Cre-loxP–mediated EGFP in β5t-Cre × loxP-EGFP mice (Fig. 2 C and D). However, ZsGreen-negative mTECs were barely detectable (<0.5% of total mTECs) in β5t-Cre × loxP-ZsGreen mice, indicating that the majority (>99.5%) of mTECs are derived from β5t-expressing progenitor cells (Fig. 3A). The few EGFP-negative mTECs detected in β5t-Cre × loxP-EGFP mice may reflect a slightly inefficient detection of fluorescence in this particular reporter mouse strain, possibly in part because of the reduced brightness and photo-stability of EGFP compared with ZsGreen (15). Indeed, we detected comparable amount of EGFP mRNA in EGFP− and EGFP+ mTECs isolated from β5t-Cre × loxP-EGFP mice. The expression levels of EGFP and ZsGreen are higher in cTECs than in mTECs in both β5t-Cre × loxP-EGFP mice and β5t-Cre × loxP-ZsGreen mice (Figs. 2 and and3).3). This difference in fluorescence may reflect the larger size of cTECs compared with mTECs (16), which in turn accounts for a more abundant accumulation of EGFP protein in cTECs than mTECs.
β5t-Cre-loxP–Mediated GFP Expression Is Detected in Embryonic mTECs.
EGFP expression in β5t-Cre × loxP-EGFP mice was detectable in TECs as early as embryonic day 12.75 (E12.75) (Fig. 3E), approximately half a day after the first detection of β5t protein between E12 and E12.5 (10). Interestingly, β5t protein expression was undetectable in a minor fraction of EGFP+ cells localized in the central region of the E12.75 thymus (Fig. 3E), indicating that a minor fraction of TECs localized in the central region of embryonic thymus previously transcribed β5t but have terminated β5t expression before E12.75. Indeed, most TECs in β5t-Cre × loxP-EGFP mice at E12.75 and E16.5 (Fig. 3F) and in β5t-Cre × loxP-ZsGreen mice at E14.5 (Fig. 3G) that were localized in the central region of fetal thymus and that highly expressed the mTEC-associated marker K5 (17, 18) also expressed EGFP and ZsGreen, respectively. These results suggest that embryonic mTECs, identified by the abundant expression of K5, are derived from β5t-expressing cells.
β5t-Cre-loxP–Mediated GFP Expression Is Detected in the Majority of Aire+ mTECs.
Finally, we examined whether Aire-expressing mTECs were derived from β5t-expressing progenitor cells. In the adult thymus of β5t-Cre × loxP-EGFP mice, we found that most Aire+ mTECs were EGFP+ (Fig. 4A). Flow cytometric analysis indicated that 97 ± 1% (n = 3) of Aire+UEA1+ mTECs in the adult thymus were EGFP+ (Fig. 4B). During ontogeny, Aire expression in mTECs was detectable as early as E16 (19, 20). We found that most (99 ± 1% and 93 ± 2%, n = 3) Aire+ mTECs at E16.5 and at postnatal day 1 were EGFP+ (Fig. 4 C–E). These results indicate that the majority of Aire-expressing mTECs are derived from β5t-expressing progenitor cells throughout ontogeny, including the perinatal period in which Aire expression is important for the establishment of long-lasting self-tolerance in T cells (7). The expression of EGFP is evident in most mTECs, including the majority of Aire-expressing mTECs, indicating that these cells are derived from progenitor cells that express β5t.
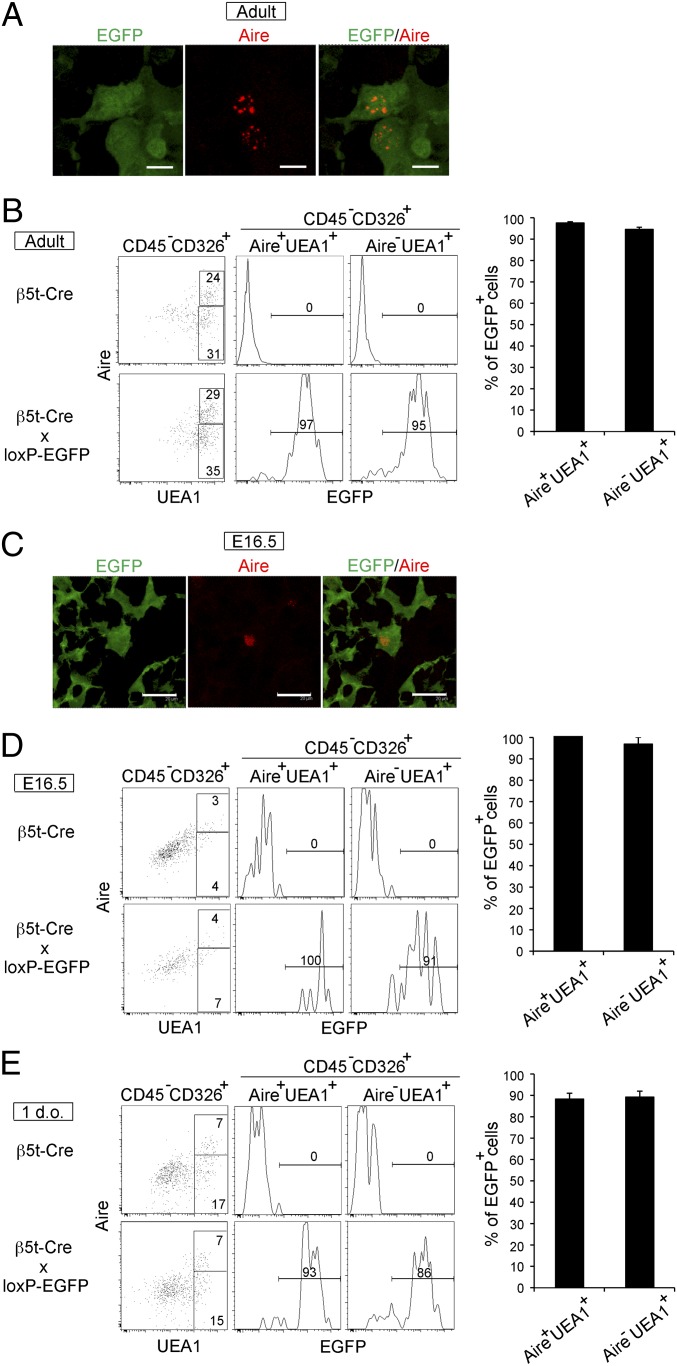
β5t-mediated GFP expression is detected in the majority of Aire+ mTECs. Cryosections of the thymus from 6- to 7-wk-old (A) and E16.5 (C) β5t-Cre × loxP-EGFP mice were analyzed by confocal microscopy. (Scale bars: A, 7 μm; C, 20 μm.) Representative images of EGFP (green) and Aire (red) are shown (n = 3). (B, D, and E) Representative flow cytometry profiles of EGFP expression in CD45−CD326+Aire+UEA1+ and CD45−CD326+Aire−UEA1+ cells from indicated mice at 5–6 wk old (B), E16.5 (D), and 1 d old (E). Numbers in plots indicate frequency within indicated area. Frequencies of EGFP+ cells within CD45−CD326+Aire+UEA1+ and CD45−CD326+Aire−UEA1+ cells are plotted (means and SEs, n = 3).
Discussion
The functional importance of mTECs, particularly Aire-expressing mTECs, in the establishment of self-tolerance in T cells has been well characterized (6, 21). Molecular signals that regulate the development and proliferation of mTECs, such as TNF superfamily receptor signals linked with NF-κB transcription factors, have been described (5, 22). It has further been established that both mTECs and cTECs are derived during embryogenesis from the endodermal epithelium of the third pharyngeal pouch (1). However, developmental pathways from the third pharyngeal pouch epithelial cells to functionally mature TECs remain largely unclear. Progenitor cells capable of giving rise to both cTECs and mTECs are detected in the embryonic thymus (23). These TEC progenitor cells were found to express the transcription factor Foxn1 (8) and the cell-surface molecule placenta expressed transcript 1 (Plet-1) (24). It was also reported that embryonic TECs that highly express Claudins 3 and 4 contained the developmental potential to give rise to mTECs but not cTECs (25). However, TEC progenitor cells that generate the majority of perinatal and postnatal mTECs have not been retrospectively characterized. Our results surprisingly demonstrate that almost all mTECs, including the majority of Aire-expressing mTECs, in embryonic, neonatal, and adult thymus have transiently expressed β5t gene. The expression of β5t-Cre must be transient as Cre and β5t transcripts and proteins cannot be detected in mTECs. Thus, our results reveal that the majority of mTECs originate from progenitor cells that transiently express β5t.
mTECs promiscuously express tissue-restricted self-antigens, which contribute to the establishment of self-tolerance in T cells. Does the β5t-Cre-loxP–mediated EGFP expression in mTECs reflect the promiscuous gene expression in mTECs? Using single-cell analysis of mTECs, promiscuous expression of tissue-restricted self-antigens was measured and found to exhibit expression frequencies between 2% and 15% at mRNAs and between 1% and 3% at proteins (26, 27). On the contrary, our results show that the β5t-Cre-loxP–mediated EGFP expression is detectable in most (>90%) mTECs, including Aire+ mTECs and Aire− mTECs (Fig. 4) as well as embryonic mTECs (Fig. 3 F and G). In addition, neither β5t nor Cre is detected in EGFP+ mTECs (Fig. 2 E and F). Thus, it is unlikely that the β5t-Cre-loxP–mediated EGFP expression in mTECs results from the promiscuous expression of β5t, Cre, and/or EGFP. Rather, the detection of EGFP in mTECs of β5t-Cre × loxP-EGFP mice is likely the consequence of β5t expression in precursor cells leading to the development of mTECs.
β5t-Cre-knock-in mice established in this study constitute a potentially useful tool for the study of TEC biology. Foxn1-Cre–expressing mice have been widely used for Cre-loxP–mediated TEC-targeted gain-of-function and loss-of-function studies (28, 29). However, Foxn1 is additionally expressed in the skin epithelium (30, 31). In contrast, our results show that β5t-Cre is specifically expressed in TECs but not in any other cell types or any other tissues, including the skin. Thus, the β5t-Cre-knock-in mice serve as a unique tool for TEC-specific genomic manipulations.
β5t-Cre-loxP–mediated EGFP expression is already detected during thymus organogenesis in early mTECs identified by the expression of K5 (Fig. 3 F and G). It was reported that K5high mTECs could be detected in the embryonic thymus of Foxn1-deficient mice, suggesting that Foxn1 is not required for the divergence of the mTEC lineage from bipotent cTEC/mTEC progenitors (18). It could therefore be informative to clarify whether progenitor cells that transiently express β5t may also be generated without Foxn1, although the Foxn1 dependence of β5t expression (10) does not readily allow the identification of these cells and their progeny. Nonetheless, because the cellularity and Aire expression of mTECs in β5t-deficient mice are not defective or altered (9, 11), it is conceivable that the transient expression of β5t during early mTEC development is dispensable for the divergence of the mTEC lineage and the consequent development of phenotypically and functionally mature mTECs.
The detection of β5t-Cre-loxP–mediated EGFP expression already in embryonic mTECs points to an early and transient β5t expression during mTEC development. It was previously shown that β5t expression is not detectable in the E11.5 thymus primordium (10), which expresses Foxn1 and presumably contains early TEC progenitor cells. Our results also demonstrate that the β5t-Cre-loxP–mediated reporter expression is not detectable in E11.5 thymus primordia (Fig. 3E). These findings suggest that β5t is not expressed in bipotent TEC progenitor cells at E11.5 and that β5t expression is initiated at the stage of TEC differentiation following the initial emergence of common TEC progenitor cells but before the detection of mTEC-restricted progenitor cells. It is possible that bipotent TEC progenitor cells may begin coexpressing a set of cTEC-associated genes, including β5t, and a set of mTEC-associated genes. Once developmental lineage is determined to be either cTECs or mTECs, the expression of lineage-compatible molecules may be secured and the expression of molecules specific for another lineage may be eventually terminated. Alternatively, it is also possible that bipotent TEC progenitor cells may begin expressing a set of cTEC-associated genes, including β5t, rather than a set of mTEC-associated genes. A developmental program driving the cTEC lineage, or the β5t-expressing lineage, may thus represent a default pathway for TEC development, whereas divergence to the mTEC lineage may require additional signals. It is further possible that β5t may be transiently transcribed after commitment to the mTEC lineage and then terminated during the subsequent differentiation into mature mTECs. Nevertheless, our unexpected observation that β5t is transiently expressed during early mTEC development precludes the possibility that mTECs are generated independent of cTEC-associated genetic programs including the expression of β5t, which is essential for cTEC function.
In conclusion, this study demonstrates that most mTECs are derived from β5t-expressing progenitor cells. mTECs play an essential role in the establishment of self-tolerance in T cells that have been newly generated by cTEC-mediated positive selection in the cortex, so that mTECs are important only when cTECs are present and functional. It can be thus speculated that the development of mTECs may be installed as a secondary consequence to the development of cTECs and that the expression of cTEC-associated β5t during the development of mTECs may reflect the cTEC origin of mTECs. These speculations are supported by the recent observation that Aire-expressing mTECs can be generated upon transplantation of embryonic TECs that express CD205, which is highly expressed by adult cTECs but not mTECs (32). Consequently, it would be interesting to establish whether the mechanisms for the development of mTECs and cTECs are symmetric or asymmetric. Further characterization of TEC progenitors at the clonal level and the molecular mechanisms driving the development of the two distinct TEC lineages will enhance our understanding of how the thymic microenvironment is differentiated into the cortical and medullary compartments and how the separate cortical and medullary regions are maintained.
Materials and Methods
Generation of β5t-Cre knock-in mice is described in SI Materials and Methods. Experiments using mice were carried out under the approval of the Institutional Animal Care and Use Committee of the University of Tokushima and according to Swiss cantonal and federal regulations and permissions. Methods for immunohistology, cell preparation, flow cytometry and quantitative mRNA analysis are also described in SI Materials and Methods.
Acknowledgments
We thank Drs. Kensuke Takada, Michiyuki Kasai, and Hiroshi Nakase for reading the manuscript. This work was supported by Grants-in-Aid for Scientific Research from the Ministry of Education, Culture, Sports, Science, and Technology and the Japan Society for the Promotion of Science (23249025 and 24111004) and by a Strategic Japanese–Swiss Cooperative Program on Molecular Medical Research from Japan Science and Technology Agency and Eidgenössiche Technische Hochschule Zurich.
Footnotes
The authors declare no conflict of interest.
This article is a PNAS Direct Submission. J.Z. is a guest editor invited by the Editorial Board.
This article contains supporting information online at www.pnas.org/lookup/suppl/10.1073/pnas.1301799110/-/DCSupplemental.
References
Articles from Proceedings of the National Academy of Sciences of the United States of America are provided here courtesy of National Academy of Sciences
Full text links
Read article at publisher's site: https://doi.org/10.1073/pnas.1301799110
Read article for free, from open access legal sources, via Unpaywall:
https://www.pnas.org/content/pnas/110/24/9885.full.pdf
Citations & impact
Impact metrics
Citations of article over time
Alternative metrics
Smart citations by scite.ai
Explore citation contexts and check if this article has been
supported or disputed.
https://scite.ai/reports/10.1073/pnas.1301799110
Article citations
Generation and repair of thymic epithelial cells.
J Exp Med, 221(10):e20230894, 09 Jul 2024
Cited by: 0 articles | PMID: 38980292 | PMCID: PMC11232892
Review Free full text in Europe PMC
Vhl safeguards thymic epithelial cell identity and thymopoietic capacity by constraining Hif1a activity during development.
iScience, 27(7):110258, 13 Jun 2024
Cited by: 0 articles | PMID: 39040069 | PMCID: PMC11261450
CellRank 2: unified fate mapping in multiview single-cell data.
Nat Methods, 21(7):1196-1205, 13 Jun 2024
Cited by: 8 articles | PMID: 38871986 | PMCID: PMC11239496
Generating an organ-deficient animal model using a multi-targeted CRISPR-Cas9 system.
Sci Rep, 14(1):10636, 09 May 2024
Cited by: 0 articles | PMID: 38724644 | PMCID: PMC11082136
Functionally diverse thymic medullary epithelial cells interplay to direct central tolerance.
Cell Rep, 43(4):114072, 05 Apr 2024
Cited by: 2 articles | PMID: 38581680
Go to all (91) article citations
Other citations
Data
Data behind the article
This data has been text mined from the article, or deposited into data resources.
BioStudies: supplemental material and supporting data
Similar Articles
To arrive at the top five similar articles we use a word-weighted algorithm to compare words from the Title and Abstract of each citation.
Ectopic Aire Expression in the Thymic Cortex Reveals Inherent Properties of Aire as a Tolerogenic Factor within the Medulla.
J Immunol, 195(10):4641-4649, 09 Oct 2015
Cited by: 10 articles | PMID: 26453754
Ontogeny of thymic cortical epithelial cells expressing the thymoproteasome subunit β5t.
Eur J Immunol, 41(5):1278-1287, 14 Apr 2011
Cited by: 59 articles | PMID: 21469133
Preparation of Single-cell Suspension of Mouse Thymic Epithelial Cells and Staining of Intracellular Molecules for Flow Cytometric Analysis.
J Vis Exp, (209), 26 Jul 2024
Cited by: 0 articles | PMID: 39141542
Development and developmental potential of cortical thymic epithelial cells.
Immunol Rev, 271(1):10-22, 01 May 2016
Cited by: 31 articles | PMID: 27088904
Review