Abstract
Free full text

Aminoglycoside resistance of Pseudomonas aeruginosa biofilms modulated by extracellular polysaccharide
Summary
Pseudomonas aeruginosa is an opportunistic pathogen that produces sessile communities known as biofilms that are highly resistant to antibiotic treatment. Limited information is available on the exact role of various components of the matrix in biofilm-associated antibiotic resistance. Here we show that the presence of extracellular polysaccharide reduced the extent of biofilm-associated antibiotic resistance for one class of antibiotics. Minimal bactericidal concentration (MBC) for planktonic and biofilm cells of P. aeruginosa PA14 was measured using a 96 well microtiter plate assay. The MBC of biofilm-grown ΔpelA mutant, which does not produce the Pel polysaccharide, was 4-fold higher for tobramycin and gentamicin, and unchanged for ΔbifA mutant, which overproduces Pel, when compared to the wild type. Biofilms of pelA mutants in two clinical isolates of P. aeruginosa showed 4- and 8-fold higher MBC for tobramycin as compared to wild type. There was no difference in the biofilm resistance of any of these strains when tested with fluoroquinolones. This work forms a basis for future studies revealing the mechanisms of biofilm-associated antibiotic resistance to aminoglycoside antibiotics by P. aeruginosa.
Introduction
Pseudomonas aeruginosa is a ubiquitous gram-negative bacterium readily isolated from water and soil environments. It is an opportunistic human pathogen frequently causing septicemia in burn patients, chronic infections in individuals with cystic fibrosis, and urinary tract infections in catheterized patients [7,9]. This organism can adopt a planktonic (free swimming) or a biofilm mode of life. In response to environmental cues, planktonic cells of P. aeruginosa adhere to surfaces and adapt a sessile existence. Sessile cells proliferate on surfaces, eventually differentiating to form structured communities called biofilms that are encased in a self-produced matrix comprised of a variety of macromolecules, including exopolysaccharides (EPS) [16].
Two different loci that contribute to the EPS component of the matrix in non-mucoid P. aeruginosa strains have been identified. The pel locus (referring to pellicle, a biofilm formed at the air-medium interface), containing the genes pelA-G, is responsible for synthesis of a glucose-rich component of the matrix, whereas psl (polysaccharide synthesis locus), containing the pslA-O genes, is responsible for a mannose and galactose rich EPS [4–6,10,12]. It has been suggested that exopolysaccharide matrix that surrounds the cells in the biofilm prevents diffusion of antimicrobial agents to the microbes. While this reduced diffusion may be the case for some antimicrobial agents, for others it has been shown that antimicrobial agents can penetrate the matrix but still cannot kill the cells in the biofilm [1,19]. In this report we address the role of the Pel EPS in biofilm-associated antibiotic resistance of P. aeruginosa PA14 grown in a microtiter well system.
Materials and methods
Bacterial strains, plasmids and media
Strains, plasmids and primers used in the study are listed in Table 1. P. aeruginosa PA14 and Escherichia coli were cultured on lysogeny broth (LB) medium [2] or minimal M63 salts supplemented with arginine (0.4%) and MgSO4 (1 mM) [11].
Table 1
Strains, plasmids and primers used in this study
Strain, plasmid, or primer | Relevant genotype or sequence (5′–3′) | Source or reference |
---|---|---|
Pseudomonas aeruginosa strains | ||
PA14 | WT | [17] |
SMC3335 | PA14ΔsadC | [13] |
SMC2893 | PA14ΔpelA | [4,5] |
SMC3351 | PA14ΔbifA | [8] |
SMC3812 | PA14ΔroeA | [14] |
SMC3814 | PA14ΔbifAΔroeA | [14] |
SMC4685 | Clinical isolate #99 from infected wound | This study |
SMC4687 | SMC4685 pelA::pMQ89 | This study |
SMC4686 | Clinical isolate #160 from infected wound | This study |
SMC4688 | SMC4686 pelA::pMQ89 | This study |
Plasmids | ||
pMQ89 | Suicide cloning vector | [18] |
Primers | ||
pelA-F | GCTACGTGCCGTTCGTCAGCA | |
pelA-R | CAGGCCGCCGAGGTAGACGTG | |
pelG-F | TATTGCTGGCGACCCTGTTCGATG | |
pelG-R | ATGAAACGCAGCAGGTAGGCACAG | |
16SrRNA-F | AGAGTTTGATCCTGGCTCAG | |
16SrRNA-R | ACGGGCGGTGTGTRC |
Molecular techniques
PCR amplification for 16SrRNA gene was carried out for the two clinical isolates, isolated from an infected wound from a tertiary care hospital of Pakistan, using primers listed in Table 1. The resulting sequences were analyzed using the BLAST software and percentage similarity to other sequences was determined. Presence of the pel genes was assayed in the clinical isolates by PCR using primers listed in Table 1. The pelA mutation in two clinical isolates was generated by a single-crossover (SCO) insertion using the pMQ89 suicide vector as described by Kuchma et al. [8].
Antibiotic resistance assay
Ninety-six-well microtiter plate-based assay was used to determine biofilm-specific antibiotic resistance as described by Mah et al. [11], with slight modification. The minimal bactericidal concentrations for biofilm grown cells (MBC-B) was determined by exposing 24 h-old biofilms to various concentrations of antibiotic for 24 h, followed by a single wash of biofilms with sterile medium to remove the antibiotics. Bacteria that survived antibiotic treatment were allowed to grow for 24 h in fresh medium lacking antibiotics, and these bacteria subsequently were transferred to LB agar plate using a multipronged device to assess growth. The MBC of planktonic bacteria (MBC-P) was determined by adding antibiotic to bacteria at the time of inoculation, incubating for 24 h followed by plating for viability. Both planktonic and biofilm populations were comprised of ca. 1 × 107 colony forming units (CFU)/well.
Biofilm formation assay and assessment of EPS production
Biofilm formation was measured using a microtiter dish system described previously [3,15]. Congo red assays were performed as reported [3,5,6]. Aliquotes of 2.5 µl of LB grown cultures were spotted onto the plates for 24 h at 37°C followed by 3–4 days of incubation at room temperature.
Results and Discussion
Loss of Pel EPS in lab strains does not lower biofilm antibiotic resistance
For P. aeruginosa PA14 (referred to as PA14), the primary polysaccharide component of the biofilm matrix in vitro is synthesized by the enzymes encoded by the pel locus. The Pel EPS is the cellular component bound by the dye Congo Red (CR) [4,5]. Previous studies have shown that strains of PA14 with mutations in sadCh, pelA and roeA genes, as well as a double bifAroeA mutant (Table 1) show a decrease in Pel-dependent polysaccharide production compared to the wild type [13,14], while the ΔbifA mutant shows increased pel-dependent polysaccharide synthesis [8]. We tested biofilms formed by these mutants and the wild type for their resistance to two different classes of antibiotics using a 96 well dish biofilm assay.
The MBC of biofilm (MBC-B)- and planktonically (MBC-P)-grown wild type and mutants was determined for gentamicin (an aminoglycoside antibiotic) and ciprofloxacin (a fluoroquinolone antibiotic). No difference in the planktonic MBC was observed between wild type and the mutant strains for either of these antibiotics (Tables 2 and and33).
Table 2
Resistance of planktonically and biofilm-grown strains to aminoglycoside antibiotics
Aminoglycosides | ||||||||
---|---|---|---|---|---|---|---|---|
Gentamicin | Tobramycin | |||||||
Strains | MBC-Pa | Fold Change | MBC-Ba | Fold Change | MBC-Pa | Fold Change | MBC-Ba | Fold Change |
PA14 | 39b | _ | 156b | _ | 19.5b | _ | 78b | _ |
ΔpelA | 39b | 1Xc | 625b | 4Xc | 19.5b | 1Xc | 312.5b | 4Xc |
ΔbifA | 39b | 1Xc | 156b | 1Xc | 19.5b | 1Xc | 78b | 1Xc |
ΔbifA ΔroeA | 39b | 1Xc | 1250b | 8Xc | 19.5b | 1Xc | 625b | 8Xc |
ΔsadC | 39b | 1Xc | 312.5b | 2Xc | 19.5b | 1Xc | 625b | 8Xc |
ΔroeA | 39b | 1Xc | 312.5b | 2Xc | 19.5b | 1Xc | 625b | 8Xc |
SMC4685d | 39b | _ | 312.5b | _ | 39b | _ | 156b | _ |
SMC4685 pelA::SCO | NDe | ND | ND | ND | 39b | 1Xc | 1250b | 8Xc |
SMC4686d | 39b | _ | 312.5b | _ | 39b | _ | 312.5b | _ |
SMC4686 pelA::SCO | ND | ND | ND | ND | 39b | 1Xc | 1250b | 4Xc |
Table 3
Resistance of planktonically and biofilm-grown strains to fluoroquinolones antibiotics
Fluoroquinolone | ||||||||
---|---|---|---|---|---|---|---|---|
Ciprofloxacin | Ofloxacin | |||||||
Strains | MBC-Pa | Fold Change | MBC-Ba | Fold Change | MBC-Pa | Fold Change | MBC-Ba | Fold Change |
PA14 | 3.9b | _ | 31.25b | _ | 62.5b | _ | 250b | _ |
ΔpelA | 3.9b | 1Xc | 31.25b | 1Xc | 62.5b | 1Xc | 250b | 1Xc |
ΔbifA | 3.9b | 1Xc | 31.25b | 1Xc | 62.5b | 1Xc | 250b | 1Xc |
ΔbifA ΔroeA | 3.9b | 1Xc | 31.25b | 1Xc | 62.5b | 1Xc | 250b | 1Xc |
ΔsadC | 3.9b | 1Xc | 31.25b | 1Xc | 62.5b | 1Xc | 250b | 1Xc |
ΔroeA | 3.9b | 1Xc | 31.25b | 1Xc | 62.5b | 1Xc | 250b | 1Xc |
SMC4685d | 0.9b | _ | 31.25b | _ | 7.8b | _ | 62b | _ |
SMC4685 pelA::SCO | 0.9b | 1Xc | 31.25b | 1Xc | 7.8b | 1Xc | 62b | 1Xc |
SMC4686d | 1.8b | _ | 31.25b | _ | 7.8b | _ | 250b | _ |
SMC4686 pelA::SCO | 1.8b | 1Xc | 31.25b | 1Xc | 7.8b | 1Xc | 250b | 1Xc |
Wild type biofilms showed a 4-fold higher MBC for gentamicin when compared to planktonic cells. The biofilm formed by the ΔbifA mutant had the same MBC for gentamicin as that of wild type, indicating that increased pel–polysaccharide production in ΔbifA does not result in an increase in resistance (Table 2, left). Although Pel polysaccharide produced by the ΔpelA, ΔsadC, ΔbifAΔroeA and ΔroeA mutants was reduced as compared to wild type, the MBC of these strains increased by 4-fold, 2-fold, 8-fold and 2-fold, respectively, for gentamicin.
For ciprofloxacin, the MBC-B was 8-fold higher for wild type as compared to planktonic cells. In contrast to the studies using gentamicin, all the mutant strains tested for ciprofloxacin showed the same MBC-B as that of wild type (Table 3, left), indicating that the presence or absence of Pel polysaccharide does not affect the resistance to ciprofloxacin.
Loss of Pel EPS results in increased MBC for aminoglycoside antibiotics in biofilms
To test whether loss of Pel EPS has the same role in biofilm antibiotic resistance for other aminoglycoside and fluoroquinolone antibiotics, as described above, we tested tobramycin (an aminoglycoside) and ofloxacin (a fluoroquinolone). There was no difference in MBC-P of wild type and the mutant strains when tested against tobramycin and ofloxacin (Table 2 and and3,3, right).
A 4-fold higher MBC was observed for wild type biofilm as compared to planktonic cells when tested for tobramycin. As observed for the other aminoglycoside (gentamicin), the MBC of biofilm-grown ΔbifA was the same as that of wild type when tested for tobramycin. This confirmed that increased production of Pel polysaccharide does not result in an increase in antibiotic resistance to aminoglycosides. The biofilm formed by the ΔpelA, ΔsadC, ΔbifAΔroeA and ΔroeA mutants, all of which have lower Pel polysaccharide production than the wild type, showed an increase in MBC for tobramycin as compared to wild type (Table 2). Like ciprofloxacin, the MBC-B for ofloxacin was the same for the wild type and all mutants tested (Table 3). These data suggest that at least for aminoglycoside antibiotics, loss of Pel polysaccharide enhanced biofilm resistance of P. aeruginosa.
Clinical isolates defective for Pel polysaccharide production display the same biofilm antibiotic resistance patterns as the PA14 lab strain
In order to assess whether biofilm antibiotic resistance patterns observed in lab strains was also observed in clinical isolates, we repeated the assays described above with two clinical, non-mucoid infected wound isolates of P. aeruginosa, designated SMC4685 and SMC4686. 16SrRNA sequence analysis was used to confirm that these isolates were P. aeruginosa using primers listed in Table 1. C>99% similarity to other well characterized strains of P. aeruginosa in Genbank. The pelA mutation was generated by a single-crossover (SCO) insertion in the two clinical isolates. The SCO insertion was confirmed by PCR, and these mutants showed a decrease in CR binding and biofilm formation (Fig. 1).
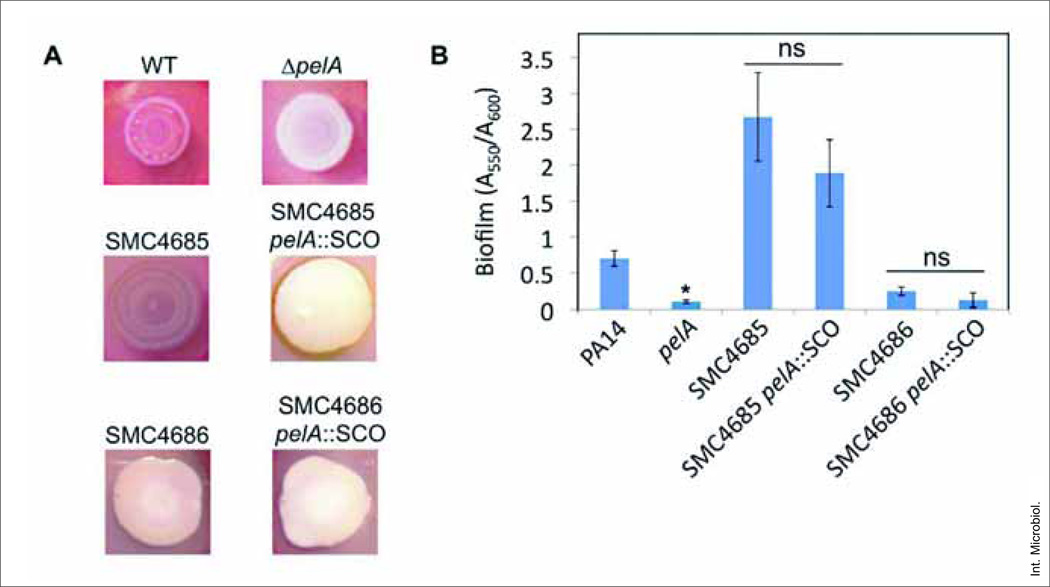
Requirement of pelA for CR binding and biofilm phenotype of wild type. (A) Representative images of CR binding by the wild type, ΔpelA, clinical wound isolates SMC4685 and SMC4686 and their pelA::SCO derivatives. Plates were incubated for 24 h at 37°C, followed by 4 days at room temperature. (B) Quantification of CV-stained biofilms (A550) normalized to their OD (A600). Strains were grown as described in Methods for 24 h prior to CV staining. Asterisks (*): significantly different from the wild type PA14 strain (Student’s t test, P < 0.05); ns, difference not significant from corresponding parental strain (P > 0.05). The average A600 value (±SD) for each of the strains from three independent experiments, which serves as a measure of planktonic growth, is as follows: PA14, 0.223 (±0.024); pelA, 0.281 (±0.045); SMC4685, 0.214 (±0.020); SMC4685 pelA::SCO, 0.208 (±0.044); SMC4686, 0.204 (±0.037); SMC4686 pelA::SCO, 0.198 (±0.077). There is no significant difference in planktonic growth between the wild type and their respective mutants for any of these strains (P > 0.05).
The MBC for planktonic and biofilm cells were measured for the clinical isolates and for their pelA mutants for ciprofloxacin, ofloxacin and tobramycin. Since the single crossover insertion was generated using the suicide vector pMQ89, which carries a gentamicin resistance marker, we could not determine the MBC for gentamicin in the mutants of the clinical isolates. For all the antibiotics tested, there was no difference in the planktonic MBC of the pelA mutant and their respective wild type for any of the antibiotics (Tables 2 and and3).3). Biofilms of the two clinical isolates SMC4685 and SMC4686, respectively, showed a 4-fold and 8-fold higher MBC for tobramycin as compared to planktonically grown cells. The MBC of biofilm grown derivatives of the clinical isolates, SMC4685 pelA::SCO and SMC4686 pelA::SCO, was 8-fold and 4-fold higher for tobramycin when compared to their respective wild type parent.
For these two clinical isolates, loss of Pel polysaccharide production resulted in only a modest decrease in biofilm formation in the microtiter dish assay, which was not statistically significant (Fig. 1B). Thus, these strains showed increased biofilm resistance despite a small, non-significant decrease in total biofilm formation. Like the PA14 lab strain, both the clinical isolates showed no difference in the MBC-B of pelA mutants and their respective wild type when tested for fluoroquinolone resistance.
In conclusion, here we present evidence that presence of Pel polysaccharide is not important for biofilm antibiotic resistance of P. aeruginosa under the conditions tested in this report, that is, in biofilms grown in microtiter dishes. Our data show that biofilm antibiotic resistance increases for aminoglycosides when the Pel polysaccharide is reduced in both lab and clinical isolates of P. aeruginosa. Furthermore, the degree of increased biofilm resistance measured in these Pel-deficient mutants is likely somewhat of an under-estimation given that these mutants typically show some decrease in biofilm formation [13,14]. It is possible that the pelA mutants produce a second EPS molecule, which might help explain our findings. Alternatively, it is possible that decreasing pel-dependent polysaccharide might result in a compensatory up-regulation of other factors that either directly or indirectly cause the observed resistance. A more detailed study of the role of Pel polysaccharide in biofilm antibiotic resistance will be necessary to address questions arising from this work.
Acknowledgements
This work was supported by NIH grant R01AI083256 to G.A.O. and a Canadian Cystic Fibrosis Foundation postdoctoral fellowship to S.P.B. We thank the Higher Education of Pakistan for funding support to W.K.
References
Citations & impact
Impact metrics
Citations of article over time
Article citations
Efficacy of sucrose and povidone-iodine mixtures in peritoneal dialysis catheter exit-site care.
BMC Nephrol, 25(1):151, 02 May 2024
Cited by: 0 articles | PMID: 38698327 | PMCID: PMC11064401
Antibacterial and antibiofilm potentials of Rumex dentatus root extract characterized by HPLC-ESI-Q-TOF-MS.
Saudi J Biol Sci, 31(4):103962, 18 Feb 2024
Cited by: 0 articles | PMID: 38419820 | PMCID: PMC10899039
Binding of GTP to BifA is required for the production of Pel-dependent biofilms in Pseudomonas aeruginosa.
J Bacteriol, 206(2):e0033123, 10 Jan 2024
Cited by: 0 articles | PMID: 38197635 | PMCID: PMC10882990
Microbial Biofilm: A Review on Formation, Infection, Antibiotic Resistance, Control Measures, and Innovative Treatment.
Microorganisms, 11(6):1614, 19 Jun 2023
Cited by: 85 articles | PMID: 37375116 | PMCID: PMC10305407
Review Free full text in Europe PMC
Interplay between biofilm microenvironment and pathogenicity of Pseudomonas aeruginosa in cystic fibrosis lung chronic infection.
Biofilm, 4:100089, 22 Oct 2022
Cited by: 8 articles | PMID: 36324525 | PMCID: PMC9618985
Review Free full text in Europe PMC
Go to all (58) article citations
Similar Articles
To arrive at the top five similar articles we use a word-weighted algorithm to compare words from the Title and Abstract of each citation.
The pel polysaccharide can serve a structural and protective role in the biofilm matrix of Pseudomonas aeruginosa.
PLoS Pathog, 7(1):e1001264, 27 Jan 2011
Cited by: 284 articles | PMID: 21298031 | PMCID: PMC3029257
Loss of the Two-Component System TctD-TctE in Pseudomonas aeruginosa Affects Biofilm Formation and Aminoglycoside Susceptibility in Response to Citric Acid.
mSphere, 4(2):e00102-19, 06 Mar 2019
Cited by: 11 articles | PMID: 30842268 | PMCID: PMC6403454
The effect of alginate lyase on the gentamicin resistance of Pseudomonas aeruginosa in mucoid biofilms.
J Appl Microbiol, 121(1):126-135, 27 May 2016
Cited by: 12 articles | PMID: 27061817
Recent perspectives on the molecular basis of biofilm formation by Pseudomonas aeruginosa and approaches for treatment and biofilm dispersal.
Folia Microbiol (Praha), 63(4):413-432, 19 Jan 2018
Cited by: 44 articles | PMID: 29352409
Review
Funding
Funders who supported this work.
NIAID NIH HHS (2)
Grant ID: R01 AI083256
Grant ID: R01AI083256