Abstract
Free full text

Pyroglutamate-3 Amyloid-β Deposition in the Brains of Humans, Non-Human Primates, Canines, and Alzheimer Disease–Like Transgenic Mouse Models
Associated Data
Abstract
Amyloid-β (Aβ) peptides, starting with pyroglutamate at the third residue (pyroGlu-3 Aβ), are a major species deposited in the brain of Alzheimer disease (AD) patients. Recent studies suggest that this isoform shows higher toxicity and amyloidogenecity when compared to full-length Aβ peptides. Here, we report the first comprehensive and comparative IHC evaluation of pyroGlu-3 Aβ deposition in humans and animal models. PyroGlu-3 Aβ immunoreactivity (IR) is abundant in plaques and cerebral amyloid angiopathy of AD and Down syndrome patients, colocalizing with general Aβ IR. PyroGlu-3 Aβ is further present in two nontransgenic mammalian models of cerebral amyloidosis, Caribbean vervets, and beagle canines. In addition, pyroGlu-3 Aβ deposition was analyzed in 12 different AD-like transgenic mouse models. In contrast to humans, all transgenic models showed general Aβ deposition preceding pyroGlu-3 Aβ deposition. The findings varied greatly among the mouse models concerning age of onset and cortical brain region. In summary, pyroGlu-3 Aβ is a major species of β-amyloid deposited early in diffuse and focal plaques and cerebral amyloid angiopathy in humans and nonhuman primates, whereas it is deposited later in a subset of focal and vascular amyloid in AD-like transgenic mouse models. Given the proposed decisive role of pyroGlu-3 Aβ peptides for the development of human AD pathology, this study provides insights into the usage of animal models in AD studies.
Alzheimer disease (AD) is the most common form of dementia, predicted to affect approximately 42 million people worldwide in the year 2020.1 The two prominent histopathological hallmarks of AD are extracellular neuritic plaques composed of aggregated amyloid-β protein (Aβ) and intracellular neurofibrillary tangles comprising hyperphosphorylated tau.2,3 Aβ is formed via the amyloidogenic pathway in which the amyloid precursor protein (APP) is liberated by two sequential endopeptidase cleavages (β- and γ-secretase).4 Besides a marked C-terminal heterogeneity of Aβ peptides represented by the isoforms Aβ40 and Aβ42, N-terminal variants are also frequently found, eg, pyroGlu-3 Aβ and pyroGlu-11 Aβ.5
N-terminally truncated and modified Aβ species have been shown to be a major component of Aβ deposited in plaques and vessels of AD and Down syndrome (DS) patients.6–9 Current hypotheses suggest that pyroGlu-3 Aβ may play an early and seminal role in the oligomerization and seeding of Aβ in familial AD (FAD) and sporadic AD.10–12 PyroGlu-3 Aβ is formed by cyclization of glutamate residues 3 or 11 by glutaminyl cyclase (QC).13 An N-terminal truncation of Aβ precedes formation of pyroglutamic acid. Such post-translationally modified species have been shown to be highly toxic to neuronal and glial cultures.14 When compared to unmodified Aβ, pyroGlu-3 Aβ has a higher aggregation propensity and stability, and exhibits increased potential to interfere with hippocampal LTP.15–17 Inhibition of QC has been shown to prevent pyroGlu-3 Aβ formation in vitro18 and in vivo.19,20 Accordingly, QC inhibitors are currently in development as a novel pharmacological approach to treat and/or prevent AD.20
With the advent of numerous preclinical models, it is now possible to mimic at least some of the pathological hallmarks of AD for characterization and testing of potential treatments. With regard to the established role of pyroGlu-3 Aβ in AD pathology represented by neuron loss and cognitive decline,12,21,22 a detailed characterization of pyroGlu-3 Aβ distribution of available transgenic (tg) and nontransgenic models and their comparison to human pathology is urgently needed. In this regard, recent research has focused only on select models.23–26
Here, we completed detailed IHC analyses in human AD, nondemented aged controls (AC), and DS brains, as well as in the Caribbean vervet,27 aged beagle canines,28 and 12 AD-like tg mouse strains. Our results support the early deposition of pyroGlu-3 Aβ along with general Aβ in humans, nonhuman primates, and canines, in contrast to later deposition in AD-like tg mouse models, underlining differences in plaque pathology between nontransgenic/nonrodent and transgenic murine model systems.
Materials and Methods
Human Subjects
Brain tissue from 12 AD (average, 84 ± 7 years), 10 AC (average, 71 ± 12 years), and 2 DS (46 and 55 years) subjects were collected postmortem at the time of autopsy, having obtained prior consent from the next of kin, and following protocols approved by the Partners Human Research Committee at Brigham and Women's Hospital (Boston, MA). Brain tissue from 1 DS subject (47 years) was generously provided for a previous study by Dr. Krystyna Wisniewski (deceased 2008; Institute for Basic Research in Developmental Disabilities, Staten Island, NY). Human subject information including age, sex, brain region examined (frontal, occipital, parietal, temporal, temporal/hippocampal), length of tissue fixation, and postmortem interval, as well as semiquantitative staining results for this study can be found summarized in Table 1.
Table 1
Examination Summary for Human Subjects
Identifier | Age (years) | Sex | Fixation![]() | Brain region examined | R1282 IR | PyroGlu-3 IR | PMI (hours) |
---|---|---|---|---|---|---|---|
Control | |||||||
AC-1 | 87 | F | Brief | P | + | + | |
T/HC | − | − | |||||
AC-2 | 72 | F | Brief | T | − | − | |
HC | − | − | |||||
AC-3 | 90 | M | Brief | FR | + | + | 17 |
HC | + | + | |||||
AC-4 | 60 | F | Brief | O | + | + | 11 |
HC | + | + | |||||
AC-5 | 64 | M | Brief | FR | + | + | 9.5 |
HC | + | + | |||||
AC-6 | 76 | F | Brief | HC | − | − | 17 |
AC-7 | 53 | M | Brief | HC | − | − | 9 |
AC-8 | 70 | M | Brief | FR | + | + | 17 |
AC-9 | 74 | M | Brief | FR | + | + | |
AC-10 | 60 | F | Brief | FR | + | + | 35 |
Alzheimer disease | |||||||
AD-1 | 82 | F | Brief | FR | + | + | 15.75 |
HC | + | + | |||||
AD-2 | 79 | F | Brief | FR | + | + | 13 |
HC | + | + | |||||
AD-3 | 91 | F | Brief | FR | + | + | 3.75 |
HC | + | + | |||||
AD-4 | 71 | M | Brief | FR | + | + | 36 |
HC | + | + | |||||
AD-5 | 84 | F | Brief | FR | + | + | |
T | + | + | |||||
AD-6 | 92 | F | Brief | HC | + | + | 7.75 |
AD-7 | 78 | M | Brief | O | + | + | 18 |
HC | + | + | |||||
AD-8 | 96 | F | Brief | O | + | + | 21 |
HC | + | + | |||||
AD-9 | 78 | M | Brief | FR | + | + | |
T/HC | + | + | |||||
AD-10 | 88 | F | Brief | P | + | + | <24 |
HC | + | + | |||||
AD-11 | 84 | F | Brief | FR | + | + | 16.5 |
HC | + | + | |||||
AD-12 | 88 | F | Brief | FR | + | + | |
HC | + | + | |||||
Down syndrome | |||||||
DS-1 | 47 | M | Long | FR | + | + | 34 |
T/HC | + | + | |||||
DS-2 | 55 | M | Brief | FR | + | + | |
T | + | + | |||||
DS-3 | 46 | F | Brief | FR | + | + | 18 |
T/HC | + | + |
Semiquantitative staining results are shown for general Aβ (R1282) and pyroGlu-3 Aβ.
F, female; M, male; FR, frontal; O, occipital; P, parietal; PMI, postmortem interval; T, temporal; T/HC, temporal/hippocampal.

Animal Models
Archived fixed brain tissues or sections were obtained from 22 aged Caribbean vervets (age 13 to 32 years; Behavioral Science Foundation, Basseterre, St. Kitts),27 three aged beagle canines (10 to 14 years; University of California, Irvine),28 and 12 AD-like tg mouse strains including J20 on C57BL/629 and B6D2F130 backgrounds, J20;C3−/−,31 mThy-1-hAPP751,32 Tg2576,33 and Tg2576/CCL2+/+,34 TgSwDI,35 TgCRND8,36 3xTg-AD,37 5xFAD,38 PSAPP,39 and APP/PS1ΔE9.40 Specific mutations used to generate the mouse models are illustrated in Table 2.
Table 2
Semiquantitative (0 to 4+) Scoring of General Aβ (R1282) Immunoreactive Plaques and PyroGlu-3 Aβ–Positive Plaques in 12 AD-Like tg Mouse Models Examined
Mouse model | Reference | Promoter | Age (months) | R1282 IR | PyroGlu-3 IR | 6E10 IR |
---|---|---|---|---|---|---|
J20 C57BL/6 APPK670N/M671L,V717F | 29 | PDGF | 8 (n = 2) | 2+ | 1+ | 2+ |
12 (n = 6) | 2.5+ | 2.67+ | 3+ | |||
17 (n = 4) | 2.75+ | 2.25+ | 2.75+ | |||
30 (n = 2) | 4+ | 3+ | 3+ | |||
J20 B6D2F1 APPK670N/M671L,V717F | 30 | PDGF | 6 (n = 4) | 2.5+ | 1.75+ | 1.75+ |
9 (n = 4) | 2+ | 0.75+ | 1.5+ | |||
16 (n = 5) | 4+ | 3+ | 3+ | |||
24 (n = 2) | 4+ | 3+ | 3+ | |||
J20;C3−/− APPK670N/M671L,V717F Complement factor C3 deficient | 31 | PDGF | 8 (n = 6) | 2.17+ | 1.5+ | 2.17+ |
12 (n = 10) | 2.8+ | 1.9+ | 3+ | |||
17 (n = 5) | 4+ | 3+ | 3+ | |||
hAPP751 APPK670N/M671L,V717I | 32 | mThy-1 | 14 (n = 2) | 4+ | 4+ | 3+ |
Tg2576 Tg2576/CCL2 APPK670N/M671L,V717I | 33, 34 | Hamster PrP (APP) hGFAP (CCL2) | 14 (n = 4) | 2+ | 1.25+ | 2+ |
14 (n = 4) | 2.75+ | 1.75+ | 2.5+ | |||
TgSwDI APPK670N/M671LE693Q,D694N | 35 | mThy-1 | 3 (n = 4) | 1.5+ | 0.75+ | 2.25+ |
6 (n = 2) | 3+ | 2+ | 1.5+ | |||
12 (n = 4) | 3+ | 1.5+ | 3+ | |||
24 (n = 4) | 4+ | 3+ | 3+ | |||
TgCRND8 APPK670N/M671L/V717F | 36 | Hamster PrP | 83 days (n = 3) | 2+ | 0.5+ | 2+ |
<300 days (n = 2) | 4+ | 3+ | 3+ | |||
3xTg-AD APPK670N/M671L,PS1M146V ,TauP301L | 37 | mThy-1 (APP, Tau) PS1 KI | ≥14 (n = 8) | 1+ | 0.5+ | 1+ |
22 (n = 4) | 2+ | 1+ | 2+ | |||
≤25 (n = 3) | 3+ | 1.5+ | 3+ | |||
5xFAD APPK670N/M671L,I716V,V717IPS1M146L,L286V | 38 | mThy-1 | 1.5 (n = 1) | 1+ | 0 | NE |
2 (n = 2) | 2.5+ | 0 | NE | |||
4 (n = 1) | 4+ | 2+ | NE | |||
6 (n = 2) | 4+ | 3+ | NE | |||
9 (n = 1) | 4+ | 3+ | NE | |||
12 (n = 12) | 4+ | 4+ | NE | |||
PSAPP APPK670N/M671Lx PS1M146L | 39 | Hamster PrP (APP) PDGF (PS1) | 4 (n = 6) | 2+ | 0 | NE |
18 (n = 4) | 4+ | 4+ | 3+ | |||
APP/PS1ΔE9 APPK595N/M596LPS1 deletion of exon 9 | 40 | mPrP (APP, PS1) | 6 (n = 2) | 2+ | 1+ | 2+ |
14 (n = 5) | 4+ | 3+ | 3+ | |||
24 (n = 4) | 4+ | 4+ | 3+ |
General Aβ was confirmed with anti-Aβ mAb 6E10. Plaque scoring of each group is given as a mean value calculated from the sum of individual scores divided by the number of animals per group. Scoring key: 1+ = 1 to 5 plaques; 2+ = 6 to 10 plaques, 3+ = 11 to 100 plaques; 4+ = 101 or more plaques.
NE, not examined.
Tissue Preparation
Human cortical and hippocampal brain blocks were fixed in 10% neutral buffered formalin for 2 hours (brief fixation) with the exception of one DS brain that was fixed for multiple years (summarized in Table 1). Archived, formalin-fixed nonhuman primate brains were blocked into nine brain regions (rostrocaudal). Tissue blocks were processed for paraffin embedding and then sectioned at 10 μm for IHC analysis. Canines were euthanized at the University of California, Irvine, via sodium pentobarbital overdose, their brains were removed, and the left hemisphere was fixed in 4% paraformaldehyde for months, blocked, processed for paraffin embedding, and then sectioned at 10 μm. Mice were anesthetized via CO2 inhalation, perfused with 20 mL of ice-cold saline followed by brain removal. Hemibrains were fixed in 10% formalin or 4% paraformaldehyde for 2 to 24 hours before being processed for paraffin embedding and then sectioned at 10 μm. All use of mice at Brigham and Women's Hospital was approved by the Harvard Medical Area Standing Committee on Animals and was in compliance with all state and federal regulations.
PyroGlu-3 Aβ Antibody Characterization
Surface Plasmon Resonance
PyroGlu-3 Aβ–specific clone 24 was characterized by surface plasmon resonance. Briefly, the analyses were performed on a Biacore 3000 (GE Healthcare, Chalfont St Giles, UK). A CM5 chip was covalently conjugated with 100 response units (RU) of test peptides on flow cells (Fc) 2, 3, and 4 (Supplemental Table S1). Fc 1 was prepared for blank subtraction. Clone 24 was diluted in running buffer HEPES-buffered saline, 3 mmol/L EDTA, 0.005% v/v surfactant P20 (HBS-EP) at a concentration of 25 μg/mL. First, a basal signal was determined with HBS-EP, followed by 180 seconds of application of antibody dilution, to determine association of antibody to antigen. Pure HBS-EP was then injected again for another 180 seconds to observe the dissociation of the corresponding antibody. Next, the affinity constants of different Aβ peptides to pyroGlu-3 Aβ–specific antibody clone 24 were determined (Supplemental Table S2). A CM5 chip was covalently conjugated with about 15,000 response units (RU) of an anti-mouse antibody on Fc 4. Fc 3 was prepared for blank subtraction. Clone 24 was diluted in running buffer HBS-EP at a concentration of 25 μg/mL and immobilized by the anti-mouse antibody on Fc 4 with about 1000 to 2000 RU. The test peptides (Supplemental Table S2) were diluted at concentrations ranging from 2 nmol/L to 500 nmol/L. First, a basal signal was determined with HBS-EP, followed by 240 seconds of application of peptide dilution, to determine association of antigen to antibody. Pure HBS-EP was then injected again for another 1200 seconds to observe the dissociation of the corresponding antibody. This cycle was performed for every concentration of the test peptides. Interactions of the test peptides with the anti-mouse capture antibody were not observed (not shown). Data were evaluated by either the 1:1 Langmuir binding fit model or the steady-state model for calculating the dissociation constants.
Dot Blot Assay
To further confirm the specificity of anti-pyroGlu-3 monoclonal antibody (mAb) (clone 24), a dot blot assay was performed. The following Aβ peptides: 1-7, 3-9, pE3-9, 3-13, 1-15, 3-40, pE3-40, and 1-40 were loaded onto a 0.2-μm nitrocellulose membrane (Bio-Rad, Hercules, CA) at 1000, 100, 10, and 1 ng. Bovine serum albumin (Sigma-Aldrich, St. Louis, MO) and PBS were loaded as negative controls. Peptides or PBS were allowed to air dry for 30 minutes at room temperature. Following drying, membranes were blocked for 1 hour at room temperature in 10% Odyssey Blocking Buffer (LI-COR Biosciences, Lincoln, NE) and probed with either clone 24 at 0.54 μg/mL (Supplemental Figure S1) or 6E10 at 0.5 μg/mL (as a positive control; data not shown) overnight at 4°C. The following day, membranes were washed three times in PBS with 0.05% Tween 20 (PBS-T), incubated with the appropriate secondary antibody for 2 hours at room temperature, and visualized using a Li-COR scanner (LI-COR Biosciences).
Antibody Adsorption
In addition, the specificity of clone 24 was confirmed by IHC by preincubation of clone 24 with pE3-40 Aβ synthetic peptide at a concentration of 15 μg of peptide/1 μg of antibody. Clone 24 was diluted to a final concentration of 1 μg/mL, and 15 μg of synthetic pE3-40 Aβ peptide was added per milliliter of diluted antibody solution. The antibody/peptide solution was incubated overnight with shaking at 4°C. The following day, the adsorbed clone 24 antibody was applied to cortical brain sections from a human AD case, aged nonhuman primate, aged beagle canine, and APPswe/PS1ΔE9 brain sections, all with known amyloid pathology. Adjacent serial sections from each case were also immunostained, in parallel, with preadsorbed clone 24 and 82E1 mAb (IBL International, Hamburg, Germany), which is specific for Aβ starting at Asp 1, at 1 μg/mL. Immunoreactivity was visualized using a VectorElite horseradish peroxidase ABC kit (Vector Laboratories, Burlingame, CA) and 3,3′-diaminobenzidine (DAB) as chromogen (Sigma-Aldrich).
Immunohistochemistry
Serial sections (10 μm) were cut for peroxidase IHC and double immunofluorescence labeling. In brief, sections were deparaffinized in two changes of Histo-Clear (National Diagnostics, Atlanta, GA) and rehydrated in graded ethanol solutions. Endogenous peroxidase activity was quenched with 0.3% H2O2 in methanol for 10 minutes. All paraffin sections were pretreated with 88% formic acid for 8 minutes to increase recognition of antigen binding sites. Sections were subsequently washed with water for 10 minutes and incubated with primary antibody overnight at 4°C. After incubation for 30 minutes at room temperature with a biotinylated secondary antibody (Vector Laboratories) IR was visualized with the VectorElite horseradish peroxidase ABC kit (Vector Laboratories) and DAB (Sigma-Aldrich) as chromogen. When using mouse mAbs on mouse brain sections, a Mouse on Mouse (M.O.M.) kit (Vector Laboratories) was used to inhibit nonspecific background staining. General Aβ deposition was analyzed using two antibodies, R1282 and 6E10. R1282 (dilution 1:1000), a general Aβ rabbit polyclonal antibody [a gift from Dennis J. Selkoe (Center for Neurologic Diseases, Boston, MA)], was raised against synthetic Aβ1-40 peptide and recognizes multiple epitopes and conformations. The mouse mAb 6E10 (dilution 1:1000; Covance, Dedham, MA) is reactive to Aβ1-16, specifically recognizing an epitope within residues 3 to 8 of Aβ. An anti–pyroGlu-3 Aβ mAb [clone 24, dilution 1:1000; Probiodrug, Halle (Saale), Germany] was used for comparison with general Aβ. Furthermore, an Aβ42 polyclonal antibody, QCB42 (dilution 1:100; BioSource, Camarillo, CA) and an Asp-1 Aβ–specific mAb, 82E1 (1 μg/mL; IBL International) were used in some tissues. Primary antibody was omitted from sections as a negative control, which consistently yielded negative results (data not shown).
Immunofluorescence
To assess colocalization of general Aβ to pyroGlu-3 Aβ, double immunofluorescence labeling was performed using R1282 and anti–pyroGlu-3 Aβ antibodies in mouse sagittal brain sections, coronal human brain sections, and nonhuman primate brain sections. Paraffin sections were prepared as noted above, including pretreatment with 88% formic acid for 8 minutes with incubation of both primary antibodies at 4°C overnight. Thereafter, sections were incubated for 2 hours at room temperature with goat anti-mouse AlexaFluor 488 (dilution 1:200; Invitrogen, Carlsbad, CA) and goat anti-rabbit Rhodamine Red-X (dilution 1:200; Invitrogen) as secondary antibodies for visualization. Finally, sections were treated with 0.3% Sudan black (Sigma-Aldrich) in 70% ethanol to quench autofluorescence. Controls to exclude nonspecific binding or cross reactivity included omission of both primary antibodies or both fluorescent secondary antibodies. These measures consistently yielded negative results (data not shown). In addition, separate sections were incubated with one primary and a mixture of both secondary antibodies, or both primary antibodies and one secondary antibody. In both cases, IR was detected for only a single primary antibody.
Results
N-Terminally Truncated and PyroGlu-3–Modified Aβ Species in AD, Down Syndrome, and Nondemented Aged Control Brains
Cortical brain sections were stained with polyclonal antibody R1282 (general Aβ) (Figure 1, A–C) and a mAb detecting pyroGlu-3 Aβ (Figure 1, D–F) to assess Aβ deposition in 12 AD cases, 3 DS cases, and 10 AC cases. The pyroGlu-3 Aβ–specific antibody used here is selective for pyroGlu-3 Aβ, showing <1% cross-reactivity to other Aβ peptides or pyroGlu peptides as determined by surface plasmon resonance and dot blot analysis (Supplemental Tables S1 and S2, and Supplemental Figure S1). Furthermore, preadsorption of clone 24 with pyroGlu-3-40 Aβ peptide strongly reduced staining in brain parenchyma in the different species (Supplemental Figure S2). Staining was performed on adjacent serial sections to compare R1282 IR to pyroGlu-3 Aβ IR. A summary of human staining results is provided in Table 1. As an example, general Aβ and pyroGlu-3 Aβ deposition is shown in the frontal cortices of a 78-year-old male AD patient (Figure 1, A and D), a 47-year-old male DS individual (Figure 1, B and E), and a 74-year-old male AC (Figure 1, C and F). IHC analysis revealed pyroGlu-3 Aβ to be present in diffuse and focal Aβ plaques in 12 of 12 AD cases, 3 of 3 DS cases, and the 7 plaque-bearing of 10 AC cases. These results indicated that virtually all plaques that displayed R1282 IR in AD and AC also contained varying levels of pyroGlu-3 Aβ IR. Furthermore, pyroGlu-3 Aβ IR was also noted in parenchymal blood vessels of frontal cortex in AD, DS, and AC cases. In contrast to AD and AC, DS cases showed increased pyroGlu-3 Aβ IR (Figure 1E) compared to R1282 IR (Figure 1B) in diffuse and compacted plaques and cerebral amyloid angiopathy (CAA). However, staining with a C-terminal–specific Aβ42 antibody showed a 1:1 ratio of pyroGlu-3 Aβ to full-length Aβ (data not shown). To further ensure that pyroGlu-3 Aβ colocalized with general Aβ deposition, double immunofluorescence staining was performed. Immunofluorescence using R1282 and pyroGlu-3 Aβ antibodies in a subset of the 12 AD cases confirmed results observed with peroxidase staining, indicating that pyroGlu-3 Aβ deposition was present in all cortical plaques and CAA along with general Aβ deposition (Figure 1, G–I). In addition, pyroGlu-3 Aβ (detected by clone 24) and Asp-1 Aβ (detected by antibody 82E1) showed an almost complete overlap of immunoreactivities in human AD brain (Supplemental Figure S2).
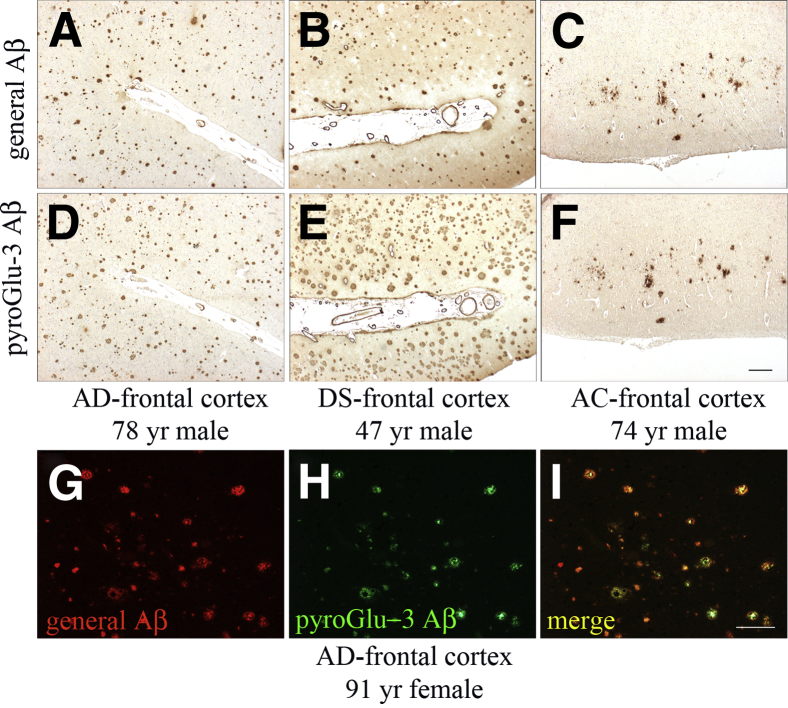
N-terminal–truncated and pyroGlu-3–modified Aβ species were colocalized in AD (A, D, and G–I), DS (B and E), and AC (C and F) frontal cortex. Immunohistochemical staining was performed on adjacent serial paraffin sections with antibodies R1282 (A–C) and anti–pyroGlu-3 Aβ (D–F), respectively. Double immunofluorescence labeling of R1282 (G) and pyroGlu-3 Aβ (H) shows abundant colocalization (I). Scale bars: 100 μm (F and I).
In Caribbean Vervets and Aged Beagle Canines, PyroGlu-3 Aβ Is Initially Deposited in Vascular Amyloid and, Subsequently, in Cortical Plaques
We next examined the presence of pyroGlu-3 Aβ in two species known to sporadically develop cerebral amyloidosis, the Caribbean vervet, a nonhuman primate model that has been previously shown to accumulate cerebral Aβ in plaques and CAA with aging27 and beagle canines.28 Deposition started around 17 years in vervets and increased with age. Of importance was the finding that IHC staining of pyroGlu-3 Aβ was sensitive for fixation time due to a lack of diffuse pyroGlu-3 Aβ deposits in long-term fixed vervet brain. There, pyroGlu-3 Aβ IR (Figure 2F) was only noted in a subset of R1282-positive plaques (Figure 2C). By contrast, pyroGlu-3 Aβ was already present in diffuse deposits (Figure 2E) of briefly fixed (2 hours) vervet brain tissue matching R1282 IR (Figure 2B). Vascular amyloid containing pyroGlu-3 Aβ was shown to precede plaque deposition, starting at the age of 13 years, and was abundant in parenchymal vessels by 17 years (Figure 2D), and colocalized strongly with R1282 IR (Figure 2A). Of the 22 vervets characterized in this study, pyroGlu-3 Aβ was present in frontal cortical plaques of 7 of 22 vervets (age >15 years) and in the blood vessels of 8 of 22 vervets (age >13 years). Double immunofluorescence staining confirmed DAB IHC visualization; however, in contrast to peroxidase single labeling, pyroGlu-3 Aβ deposition (Figure 2H) was at a 1:1 ratio (Figure 2I) with general Aβ (Figure 2G) in plaques and CAA.
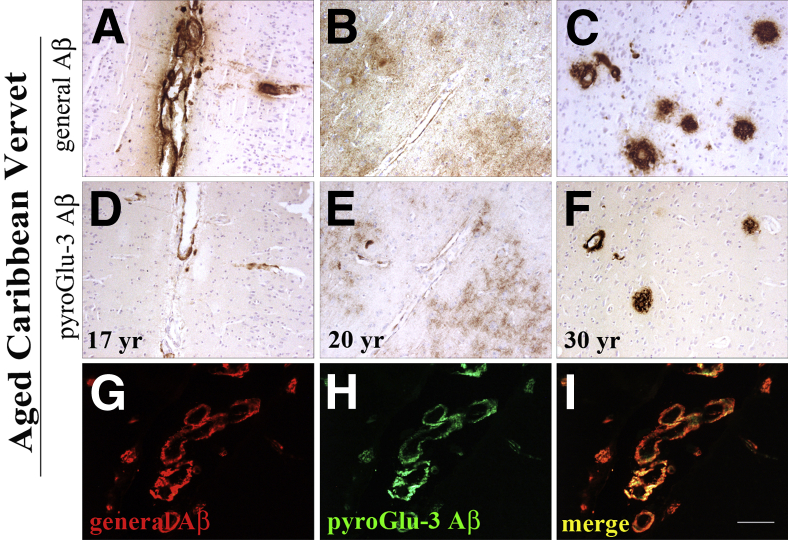
Immunohistochemical analysis of aged Caribbean vervets in formalin-fixed, paraffin-embedded frontal cortex sections comparing R1282 IR (A–C) and pyroGlu-3 Aβ IR (D–F) on adjacent serial sections. Both R1282 (A) and pyroGlu-3 Aβ (D) deposition begins in the parenchymal vasculature, as shown in a 17-year-old monkey. With aging, cerebral pyroGlu-3 Aβ is first found in diffuse deposits of briefly fixed brains (E) and also in plaques and CAA of long-term fixed brains (F); however, there, pyroGlu-3 Aβ is only detected in a subset of R1282-positive plaques (C). G–I: Double immunofluorescence labeling with R1282 (red; G), pyroGlu-3 Aβ (green; H), and merged (I) reveal striking colocalization of N-terminally truncated and pyroGlu-3–modified Aβ species in plaques and CAA in the frontal cortex of a 24-year-old vervet. Scale bar = 100 μm (I).
Moreover, we conducted IHC analyses with long-term fixed prefrontal cortex from three aged beagle canines. The youngest canine (age 10 years) had only diffuse Aβ42-positive plaques in prefrontal cortex (Figure 3A) with no pyroGlu-3 Aβ IR (Figure 3D). By contrast, Aβ42-positive diffuse and compacted plaques, as well as CAA, were detected in the two older canines (12 years) (Figure 3B) and (14 years) (Figure 3C). Aβ42 IR across the entire section was greatest in the oldest canine (14 years), suggesting that plaque deposition in canines increases with age. PyroGlu-3 Aβ deposition was also detected in the two oldest canines (12 and 14 years) (Figure 3, E and F), with higher levels in the older animal. Most of the pyroGlu-3 Aβ IR was seen in blood vessels and colocalized with Aβ42 IR. To a lesser extent, pyroGlu-3 Aβ IR was observed in a few compacted Aβ42-positive plaques at 12 and 14 years of age, respectively (Figure 3F). PyroGlu-3 Aβ IR and Asp-1 Aβ IR strongly overlapped in both vervet and canine brain, as was observed in human brain (Supplemental Figure S2). However, in beagle canine brain, there was somewhat less pyroGlu-3 Aβ IR compared to Asp 1 Aβ IR, likely due to the long fixation time.
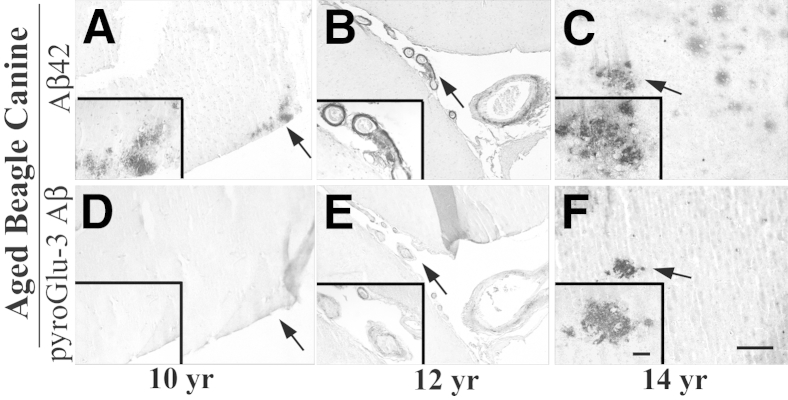
Immunohistochemistry on paraformaldehyde-fixed and paraffin-embedded prefrontal cortex from aged beagle canines with a C-terminal–specific Aβ 42 (A–C) and pyroGlu-3 antibody (D–F) reveals diffuse Aβ42 deposits at 10 years of age (A) that are devoid of pyroGlu-3 Aβ IR (D). Staining in a 12-year-old canine shows Aβ42-positive blood vessels (B) that are concomitantly immunolabeled by pyroGlu-3 Aβ mAb on an adjacent serial section (E). In the oldest canine examined in this study (14 years), focal Aβ42–positive deposits are observed (C); however, only a subset are pyroGlu-3 Aβ positive (F). Insets show the magnified areas indicated by the arrows. Scale bar = 50 μm (F).
PyroGlu-3 Aβ Accumulation Varies in AD-Like tg Mouse Models by Age of Onset, Cortical Region, and General Abundance
Murine models are the primary source for AD research in vivo due to the possibility of genetic manipulation. Consequently, a plethora of AD mouse models reflecting certain aspects of human AD are now available. Therefore, we next investigated the deposition of general and pyroGlu-3 Aβ peptides in 12 different mouse models of AD. The different mouse models were further grouped by their AD-related transgenes. Details on genetic mutations and semiquantitative analysis of general and pyroGlu-3 Aβ IR in each mouse model can be found in Table 2.
PyroGlu-3 Aβ Deposition in tg Mice Based on Expression of Different APP Mutants
Among the mice examined, Tg2576, J20, TgCRND8, and TgSwDI were generated on the basis of the expression of human familial APP mutations.
First, we characterized the widely used AD-like tg mouse model, Tg2576, based on expressing the Swedish mutation. At 14 months of age, R1282 IR was localized in the hippocampus, in particular along the molecular layer of the dentate gyrus (DGm) (not shown), in the neocortex, and in blood vessels along the pial surface (Supplemental Figure S3C). Minute focal deposits of pyroGlu-3 Aβ IR were noted distinctly only in the DGm and subiculum (not shown). When comparing Tg2576 mice with age-matched Tg2576/CCL2+/+ that additionally overexpress human monocyte chemoattractant protein (MCP-1, CCL2),34 we observed an overt increase in general Aβ diffuse and focal deposits (Supplemental Figure S3E) and pyroGlu-3 Aβ focal deposits in the neocortex (Supplemental Figure S3F).
Next, we analyzed J20 and TgCRND8 mice possessing both the Swedish and Indiana mutations. J20 mice show Aβ deposition starting at 6 months in the hippocampus29 that continues to radiate out to the neocortex with aging. We examined a temporal series of J20 mice on a hybrid background (C57BL/6 × DBA/2) at 6, 9, 16, and 24 months, as well as J20 and J20;complement factor 3-deficient (J20;C3−/−) mice at 8, 12, and 17 months on the C57BL/6 background. We further sought to examine immunohistochemically (IHC) whether strain background and/or C3 deficiency influenced pyroGlu-3 Aβ deposition as previously noted for general Aβ deposition.30,31 A summary of the number of mice for each strain analyzed, as well as semiquantitative plaque scoring for R1282 and pyroGlu-3 Aβ IR, can be found in Table 2.
At all ages, and in all three J20 lines, general Aβ was more abundant than pyroGlu-3 Aβ (Supplemental Figure S4, A–F). PyroGlu-3 Aβ IR was noted at modest levels exclusively in the hippocampus in three of four J20 B6D2F1 hybrid mice at 6 months of age. By contrast, J20 and J20;C3−/− mice on a C57BL/6 background displayed less pyroGlu-3 Aβ IR in the hippocampus at 8 months of age compared to hybrid mice at 6 months (Table 2). In all three strains, pyroGlu-3 Aβ deposition started as plaques in hippocampus, increasing with age to cortical plaques, followed by vascular deposits. In the J20 B6D2F1 mice, pyroGlu-3 Aβ IR was additionally present in the DGm at 6 months. By 9 months, J20 B6D2F1 mice displayed increased pyroGlu-3 Aβ IR in the hippocampus and the initial appearance of cortical plaques. At 16 months, J20 hybrid mice had moderate pyroGlu-3 Aβ deposition (plaque score, 3+) (Table 2) in the neocortex, hippocampus, and subiculum. Parenchymal vascular amyloid was first identified at this age. By 24 months, diffuse pyroGlu-3 Aβ IR was noted specifically in the DGm. J20 and J20;C3−/− on the C57BL/6 background showed a similar progression of pyroGlu-3 Aβ deposition at 12 and 17 months relative to the J20 mice on the hybrid background, with an age-dependent increase of pyroGlu-3 Aβ in the hippocampus and pyroGlu-3 Aβ–positive blood vessels appearing in the parenchyma at 16 months. At 17 months, in contrast to J20 on the C57BL/6 and B6D2F1 backgrounds at similar ages, J20;C3−/− mice displayed a marked increase in neocortical diffuse and focal plaques immunolabeled with both R1282 (Supplemental Figure S4C) and pyroGlu-3 Aβ (Supplemental Figure S4F) antibodies, respectively.
Compared to J20 mice, TgCRND8 mice showed much earlier general Aβ deposition in the neocortex and DGm starting at 3 months; however, no pyroGlu-3 Aβ deposition was observed at this age. PyroGlu-3 Aβ deposition in the hippocampus and cortex of TgCRND8 mice occurred later and followed a general Aβ staining pattern, although pyroGlu-3 Aβ IR was not present in all plaques. In the two oldest mice (10 and 11 months, respectively), general Aβ- and pyroGlu-3 Aβ–positive focal deposits were detected in hippocampus (Figure 4, G and H), neocortex, olfactory bulb (Supplemental Figure S3, G and H), and thalamus. General Aβ deposits were found in all four brain regions as well as cerebellum, and were more abundant. Interestingly, pyroGlu-3 Aβ was not noted in any of the vasculature in this model even though general Aβ vascular deposits were observed in the parenchyma and leptomeninges with R1282 (data not shown).
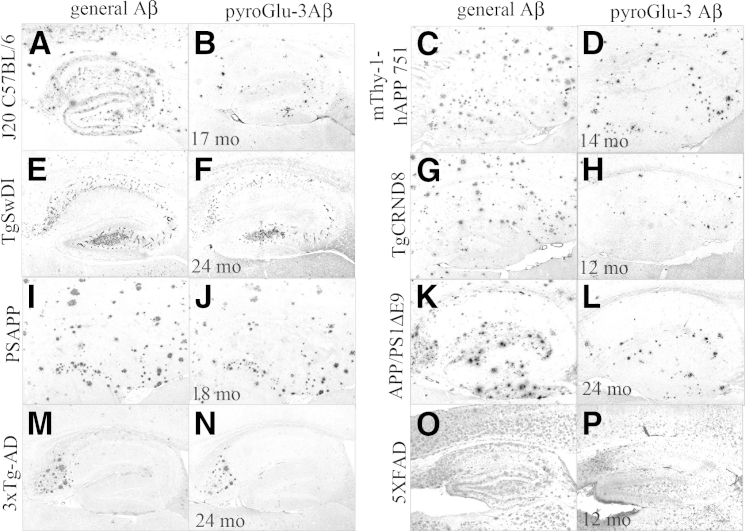
General Aβ (R1282) IR was compared to pyroGlu-3 Aβ IR in the hippocampus of J20 tg mice on the C57Bl/6 background (A and B), mThy-1-hAPP751 (C and D), TgSwDI (E and F), TgCRND8 (G and H), PSAPP (I and J), APP/PS1ΔE9 (K and L), 3xTg-AD (M and N), and 5XFAD (O and P) mice at time points of advanced general Aβ and pyroGlu-3 Aβ deposition. In general, pyroGlu-3 Aβ is less abundant and colocalizes with only a subset of R1282-positive general Aβ deposits, although the amount of pyroGlu-3 Aβ varied by model. Scale bar = 200 μm (P).
Two 14-month-old mThy-1-hAPP751 tg mice bearing the Swedish and London mutations were immunolabeled with R1282 and the pyroGlu-3 Aβ mAb on adjacent serial sections. This APP tg strain begins depositing plaques between 3 to 4 months of age in the neocortex, and by 5 to 7 months, plaques progress to the hippocampus, thalamus, and olfactory bulb.32 Intense pyroGlu-3 Aβ IR (Figure 4D) colocalized with general Aβ deposition (Figure 4C) in diffuse and focal plaques throughout the entire hippocampus at 14 months. In addition, pyroGlu-3 Aβ (Supplemental Figure S3B) followed the general Aβ deposition pattern in the neocortex (Supplemental Figure S3A) and thalamus, as well as in leptomeningeal and parenchymal blood vessels. Interestingly, R1282 IR in diffuse, extracellular deposits was observed in the olfactory bulb and spinal cord; however, no pyroGlu-3 Aβ IR was observed in these two regions (data not shown).
TgSwDI mice harboring three different APP mutations (Swedish/Dutch/Iowa) were examined for general Aβ and pyroGlu-3 Aβ deposition at 3, 6, 9, 12, and 24 months, respectively. Detailed IHC analyses revealed that this AD-like tg mouse had some of the earliest and most abundant cerebral pyroGlu-3 Aβ deposition, seen as vascular deposits that increased with age. Initially, R1282 and pyroGlu-3 Aβ were both observed solely in the subiculum at 3 months (data not shown). At 6 months of age, general Aβ deposition (mostly vascular) had radiated to the thalamus, stratum oriens, CA1 (Figure 5A), DGm, and dentate gyrus polymorphic layers (DGpl), and concomitantly increased in the subiculum. PyroGlu-3 Aβ was observed in a subset of general Aβ plaques in the subiculum, thalamus, and stratum oriens, and CA1 region (Figure 5D). At this age, general Aβ was in its early stages of deposition in the neocortex, but no pyroGlu-3 Aβ was detected. By 12 months, pyroGlu-3 Aβ deposition (Figure 5E) had increased in the aforementioned regions (Figure 5B) and was found in a subset of general Aβ deposits in the hippocampal CA2 region, DGm, and neocortex. By 24 months, pyroGlu-3 Aβ (Figures 4F and and5F)5F) and R1282 (Figures 4E and and5C)5C) were at a near 1:1 ratio in the hippocampus, specifically in the CA1 (Figure 5, C and F), CA2, CA3, DGm, DGpl, stratum oriens, the thalamus, and neocortex (data not shown).
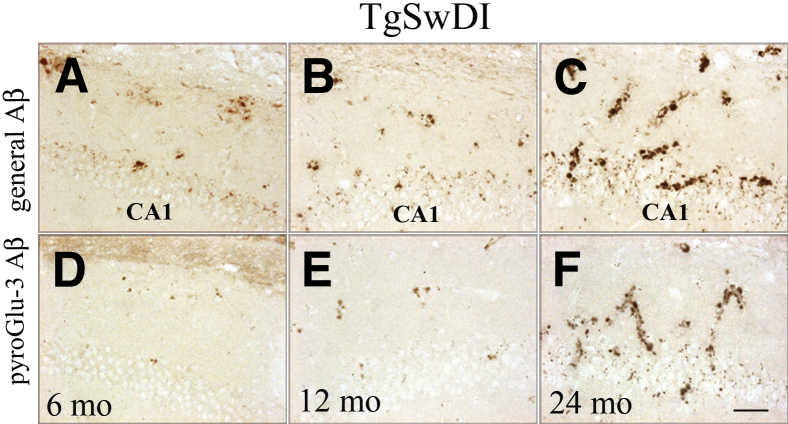
Progression of general Aβ (A–C) corresponds to increases in pyroGlu-3 Aβ (D–F) in the hippocampal CA1 region in TgSwDI. At 6 months, minute amounts of general Aβ (A) and even less pyroGlu-3 Aβ (D) can be detected in the CA1 region. By 12 months, both R1282 (B) and pyroGlu-3 Aβ (E) are increased in the microvasculature between the pyramidal neurons and in the stratum oriens. By 24 months, intense CAA is observed with immunostaining with R1282 (C) that colocalizes almost completely with pyroGlu-3 Aβ (F). Scale bar = 25 μm (F).
In summary, pyroGlu-3 Aβ deposition followed the appearance and was less abundant than plaques positive for general Aβ in APP-overexpressing models. The more aggressive the Aβ pathology, the earlier pyroGlu-3 Aβ was observed. Interestingly, two adjacent mutations within the Aβ sequence (Dutch/Iowa) were associated with very early detection of pyroGlu-3 Aβ vascular deposits in TgSwDI mice.
PyroGlu-3 Aβ in tg Mice Based on Expression of Different APP-Variants with Presenilin Mutations
The next group of models comprised PSAPP, 5XFAD, and APP/PS1ΔE9 mice. These are characterized by the combined expression of mutated human presenilin and human APP mutations.
The first model in this group, PSAPP, are Tg2576 mice possessing the Swedish APP mutation crossed to PS1M146L tg mice. At 4 months of age, all six mice examined were devoid of general and pyroGlu-3 Aβ measured immunohistochemically. When 18-month-old mice (n = 4) were immunolabeled with R1282 and pyroGlu-3 Aβ antibodies on adjacent serial sections, abundant general Aβ (Figure 4I) and pyroGlu-3 Aβ (Figure 4J) deposits were observed throughout the entire brain. General Aβ appeared as both focal and diffuse deposits throughout the cortex (Supplemental Figure S5A), hippocampus, and thalamus. Vascular amyloid was noted in the parenchyma pial surface and only in the leptomeninges of the cerebellum in sections stained with R1282 (Supplemental Figure S5C). As was commonly observed in most of the AD-like tg mouse models, pyroGlu-3 Aβ deposition was observed in focal deposits only, throughout the cortex (Supplemental Figure S5D), hippocampus, thalamus, and olfactory bulb (Supplemental Figure S5E), as well as in R1282-positive blood vessels in the cerebellum (Supplemental Figure S5F), cortical parenchyma, and pial surface in old PSAPP mice (data not shown).
For 5XFAD mice harboring three different APP mutations (Swedish/Florida/London) and two different PS1 mutations (PS1M146L, PS1L286V), cerebral Aβ deposition was detected very early, at 1.5 months of age, with several R1282 IR plaques noted in layer V of the cortex and in subiculum. However, no pyroGlu-3 Aβ IR was detected at this young age. There was a dramatic increase in R1282 IR in the mice examined at 2 months of age in which general Aβ deposition was observed throughout the hippocampus, most intensely in the subiculum, and continued to increase in the cortex from cortical layer V; however, pyroGlu-3 Aβ IR was not observed. At 4 months of age, pyroGlu-3 Aβ was shown to be deposited in focal plaques throughout cortical layer V (Supplemental Figure S3L) and the subiculum, which colocalized with the cores of R1282-positive plaques (Supplemental Figure S3K). By 6 months of age, pyroGlu-3 Aβ continued to increase throughout the entire cortex and subiculum, and was detected in DGpl. By 9 and 12 months of age, respectively, general Aβ (Figure 4O) and pyroGlu-3 Aβ (Figure 4P) were observed as focal deposits covering the entire brain.
Finally, APP/PS1ΔE9 mice, harboring the Swedish APP mutation and a deletion of exon 9 in PS1, displayed general Aβ plaque deposition (R1282 IR) in the hippocampus (Figure 6A) at 6 months, but not in neocortex or cerebellum. Surprisingly, pyroGlu-3 Aβ was observed in a small subset of focal plaques in the hippocampus in two of four mice, but not elsewhere at this young age (Figure 6D). By 14 months, general Aβ-immunopositive diffuse, focal, and vascular deposits had increased dramatically in the neocortex (Figure 6B), hippocampus, and cerebellum. Interestingly, APP/PS1ΔE9 mice at 14 months displayed pyroGlu-3 Aβ IR in diffuse and focal deposits in the neocortex (Figure 6E) as well as in the parenchymal blood vessels and focal deposits in the hippocampus and cerebellum. By 24 months, abundant general Aβ IR was noted in the hippocampus (Figure 4K), neocortex, and cerebellum. Most compacted plaques in the hippocampus (Figure 4L) and a subset of neocortical plaques were pyroGlu-3 Aβ positive. In contrast to PSAPP mice, aged APP/S1ΔE9 mice showed strong IR for general Aβ in the leptomeningeal blood vessels, and plaques in the molecular layer in the cerebellum (Figure 6C) that often colocalized with pyroGlu-3 Aβ IR (Figure 6F).
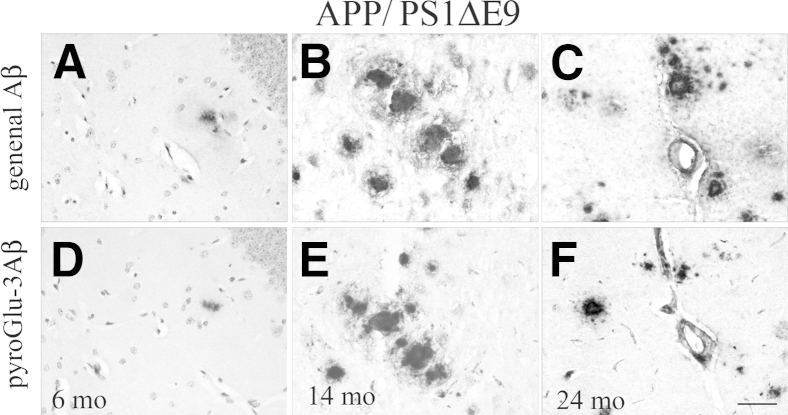
Progression of cerebral general Aβ (A–C) and pyroGlu-3 Aβ (D–F) deposition in APP/PS1ΔE9 mice. Mice were immunolabeled with R1282 and pyroGlu-3 Aβ antibodies at 6, 14, and 24 months, respectively. General Aβ is immunolabeled initially in the neocortex (not shown) and hippocampus (A) at 6 months. One pyroGlu-3 Aβ–positive plaque could be detected at 6 months in the hippocampus (D). By 14 months, pyroGlu-3 Aβ deposition progresses to the neocortex as focal and diffuse deposits (E) that colocalize completely with general Aβ (B). In contrast to PSAPP mice at 18 months, robust general Aβ (C) and pyroGlu-3 Aβ IR is noted in molecular layer and leptomeningeal blood vessels in the cerebellum of 24-month-old mice. Scale bar = 50 μm (F).
In summary, the combination of APP and PS1 mutations resulted in a more aggressive plaque pathology. However, as shown for APP mutants above, general Aβ was deposited first, followed by pyroGlu-3 Aβ deposition in a subset of plaques and blood vessels.
Analysis of 3xTg-AD Mice Bearing Mutations in APP, PS-1, and Tau
In our colony, 3xTg-AD mice possessing the Swedish APP mutation, PS1M145V, and TauP301L deposited Aβ plaques starting at approximately 14 months of age with advanced cerebral amyloidosis at approximately 20 months. At the earliest stages, R1282 IR was observed first in the subiculum and later radiated out to the hippocampal DGm and cortex as diffuse and focal plaques; however, pyroGlu-3 Aβ IR was rare. However, even at 14 months, a small amount of pyroGlu-3 Aβ deposition (Supplemental Figure S3J) was observed in a subset of R1282-positive plaques (Supplemental Figure S3I) in the subiculum. Subsequent examination of cortical brain sections from 20- to 28-month-old 3xTg-AD mice (n = 10) revealed that pyroGlu-3 Aβ IR increased with age but was only observed as intense focal deposits restricted to the subiculum (Figure 4N) and layer 5 of the cortex in the oldest mouse examined (28 months), differing from general Aβ deposition that was abundant in diffuse and focal deposits in the hippocampus (Figure 4M) and cortex.
Discussion
The present study provides a detailed immunohistochemical analysis of general Aβ deposition and N-terminal–truncated and pyroGlu-3–modified Aβ species. The study aimed to characterize the sequence and pattern of appearance of pathology in humans, nonhuman primates, canines, and mice, based on two highly specific antibodies, R1282 and pyroGlu-3 Aβ.
Staining in 12 AD cases revealed that pyroGlu-3 Aβ was present in high abundance in focal and diffuse plaques, as well as in CAA that followed a R1282 staining pattern in all cases. Complementarily, findings were observed in cortical brain tissue from 7 of 10 AC (only those bearing plaques) and 3 of 3 DS patients. Our results in human cases paralleled several previously published reports. Initial studies by Saido et al7 demonstrated both immunohistochemically and biochemically that pyroGlu-3 Aβ is a major component of senile plaques in the brains of AD patients and young DS individuals age <30 years. PyroGlu-3 Aβ was found in comparable or greater levels than Aβ species, starting at aspartate 1 [Aβ1(D)-x]. Therefore, it was concluded that pyroGlu-3 Aβ could play an early and seminal role in AD pathogenesis. Since then, numerous reports have documented pyroGlu-3 Aβ to be present in high abundance in sporadic AD, FAD, and DS brains,41–43 correlating with disease progression and severity.10,44,45 This is mainly based on its pivotal toxicity leading to, eg, the impairment of long-term potentiation in situ12,17 and neurodegeneration in vivo,21 qualifying pyroGlu-3 Aβ as a target for drug development.
Preclinical studies require suitable animal models reflecting major characteristics of the disease. We found pyroGlu-3 Aβ IR to be present in two models of cerebral amyloidosis, the Caribbean vervet and beagle canines. Our laboratory was the first to document cerebral Aβ accumulation in an age-dependent manner in the Caribbean vervet27 in which Aβ deposits first appeared in vascular amyloid as predominantly Aβ40-reactive blood vessels. To our knowledge, we are now the first to characterize N-terminally truncated Aβ peptide variants in this model. With aging, beagles and vervets preferentially begin to deposit pyroGlu-3 Aβ in the vasculature, and later pyroGlu-3 Aβ was found to colocalize in a subset of general Aβ-positive plaques. It became evident that the fixation time has a major impact on pyroGlu-3 Aβ detection. Diffuse deposits comparable to general Aβ IR were only detected in briefly fixed vervet brain tissue, whereas long-term fixed vervet brains show pyroGlu-3 Aβ IR only in a subset of cortical plaques even in the oldest monkey tested. This might lead to the underestimation of pyroGlu-3 IR also in long-term fixed human AD cases and has to be taken into consideration for future immunohistochemical studies on pyroGlu-3 Aβ deposition in human and animal brain tissue.
Despite the desirable usage of animal models showing sporadic development of AD, such studies are hampered by the availability, costs, and time until onset of phenotype. The earliest appearance of pyroGlu-3 Aβ was around 17 years of age in vervets and 12 years of age in beagles. Therefore, mouse models genetically engineered to show AD-like symptoms within months (rather than years) are the standard of current research.
Here, we confirmed the deposition of pyroGlu-3 Aβ in a large variety of AD-like tg mouse models. In contrast to the human phenotype, pyroGlu-3 Aβ is found after initial deposition of general Aβ, albeit to a much lesser extent. Reasons could be a lower activity of murine QC to covert Aβ peptides, as previously suggested by Seifert et al,46 or an expression pattern of the human APP transgene differing from the distinct expression pattern of QC in mouse brain.47 The latter might be overcome by coexpression of QC and APP as, eg, described for human QC–overexpressing mice crossed with 5XFAD mice with both transgenes under the control of the murine Thy-1 promoter. Here, increased levels of pyroGlu-3 Aβ could be detected compared to 5XFAD alone.48 In addition, it has to be noted that the composition of Aβ peptide species has been shown to be fundamentally different in mouse models and human AD.49,50
The age of first pyroGlu-3 Aβ deposition was influenced by the extent of genetic manipulation and by the promoter used in the respective mouse models. PyroGlu-3 Aβ was found at older ages when the Swedish mutation was the only APP mutation applied in a model (Tg2576, 3xTg-AD). Comparison of the pathology of APP(Swedish/Indiana)-expressing mice (J20 and TgCRND8) revealed a much earlier Aβ deposition in TgCRND8 using the Hamster PrP promoter compared to J20 driving gene expression via the PDGF promoter. Furthermore, combined APP and PS1 mutations caused a more aggressive pathology leading also to earlier detection of pyroGlu-3 Aβ. Here, not only the proteolytic function of the γ-secretase complex might be altered, but also the PS1-dependent intracellular transport of APP.51,52 Because FAD PS1 mutations cause a delayed exit of APP from the trans-Golgi network,51 the time QC has access to the Aβ N-terminus in intracellular compartments is increased. Thereby, the mildly acid pH in the Golgi network and in secretory granules would strongly promote glutamate cyclization,13,18 eventually explaining increased pyroGlu-3 Aβ level by PS1 mutations. Similar observations were made in human FAD patients in whom PS1 mutations led to a very aggressive phenotype accompanied by massive pyroGlu-3 Aβ deposition.42,53 However, the sequential appearance of general Aβ followed by pyroGlu-3 Aβ was not changed irrespective of the applied FAD mutation in the mouse models, suggesting that other factors such as genetic manipulation of the β-secretase cleavage by the Swedish mutation might also be involved in the pattern observed. Interestingly, TgSwDI, possessing 2 adjacent FAD mutation within the Aβ sequence, caused a very early deposition of pyroGlu-3 Aβ peptides. Therefore, FAD mutations have a major impact on pyroGlu-3 Aβ deposition in the tested mouse models.
In summary, our results confirm previous studies which indicated that pyroGlu-3 Aβ is a major species found in nearly all diffuse and focal Aβ deposits, as well as vascular amyloid in AD, DS, and AC cases, and affirm that pyroGlu-3 Aβ isoforms are disease-relevant species. Treatments aimed at modulating pyroGlu-3 Aβ generation through QC inhibitors and/or gene expression or through Aβ immunotherapy by selectively targeting pyroGlu-3 Aβ are promising avenues for the prevention and/or treatment of AD. In agreement with humans, previously characterized mammalian models used in the study, Caribbean vervets and beagle canines, contain N-terminally truncated and pyroGlu-3–modified Aβ species. PyroGlu-3 Aβ deposition was further found to vary significantly among different AD-like Tg mouse models examined in this study for age of onset, cortical region, and general abundance; however, the same general paradigm was found among most murine models, that general Aβ precedes pyroGlu-3 Aβ deposition and is usually observed in much lesser quantities, sometimes correlating the age of onset of behavioral deficits. Future directions could be aimed at measuring QC expression in these models to better understand why pyroGlu-3 Aβ varied greatly among each model and whether pyroGlu-3 Aβ deposition correlates with neuron loss and associated gliosis more than full-length, general Aβ.
Acknowledgments
We kindly thank Eva M. Luo, Dr. Jing Sun, Dr. Lori-Ann Christie and Jessica E. Kenison for technical assistance; Dr. Dennis J. Selkoe (Center for Neurological Diseases, Brigham and Women's Hospital, Boston, MA) for providing the R1282 polyclonal antibody; Dr. Karen Duff (Columbia University, New York, NY), Dr. William E. Van Nostrand (Stony Brook University, Stony Brook, NY), and Dr. Eliezer Masliah (University of California San Diego, La Jolla, CA) for generously providing mouse brain sections; and Dr. Lennart Mucke (Gladstone Institute, University of California, San Francisco, San Francisco, CA) and Dr. Frank M. LaFerla (University of California, Irvine, Irvine, CA) for providing J20 and 3xTg-AD breeders, respectively.
Footnotes
Funded in part by the NIH/National Institute on Aging grants R01 AG020159 and AG040092 (C.A.L.) and an anonymous foundation (C.A.L.).
J.L.F. and K.X.L. contributed equally to this work.
Disclosures: H.C., M.K., and S.S. are former or present employees of Probiodrug and own stock options in the company. H.U.D. serves as Chief Scientific Officer of Probiodrug and is a stockholder. T.C.S. and C.A.L. serve on the scientific advisory board of Probiodrug.
Supplemental Data
Several synthetic Aβ peptides, of varying lengths, at concentrations of 1000, 100, 10, and 1 ng were loaded onto a 0.2-μm nitrocellulose membrane. Membranes were either probed with clone 24 or 6E10 (as a positive control; data not shown). Clone 24 was shown to recognize pE3-9 Aβ and pE3-40 Aβ, but not their N-terminally truncated precursors, Aβ3-9 or 3-40, respectively; in addition, clone 24 did not detect Aβ1-7, 3-13, 1-15, or full-length Aβ1-40. Bovine serum albumin and PBS were loaded at negative controls and yielded negative results.
Serial sections of frontal cortex from a 79-year-old AD case (A, E, and I), frontal cortex from a 32-year-old Caribbean vervet (B, F, and J), prefrontal cortex sections from a 14-year-old beagle canine (C, G, and K), and sagittal brain sections from an 18-month-old APPswe/PS1ΔE9 mouse (D, H, and L) were immunolabeled with 82E1, an Asp1-specific anti-Aβ mAb, clone 24, a pyroGlu-3 Aβ mAb, and clone 24 preincubated with synthetic pE3-40-Aβ peptide. Intense immunolabeling of plaques and vascular amyloid in human, nonhuman primate, and canine brain was observed with 82E1 (A–C) and clone 24 (E–G). More Asp1 Aβ than pyroGlu-3 Aβ plaques were detected in APPswe/PS1ΔE9 mice. Importantly, clone 24 IR was abolished after preincubation of clone 24 with synthetic pyroGlu3-40 Aβ peptide in all models examined (I–L). These results further confirm the specificity of clone 24 for pyroGlu-3 Aβ. Plaque labeling was absent when primary antibody was omitted (data not shown). Scale bar = 100 μm (L).
General Aβ (A, C, E, G, I, and K) and pyroGlu-3 Aβ (B, D, F, H, J, and L) staining in mThy-1-APP751 (A and B), Tg2576 (C and D), Tg2576/CCL2+/+ (E and F), TgCRND8 (G and H), 3xTg-AD (I and J), and 5xFAD (K and L). Intense focal deposits were immunolabeled with R1282 (A), which colocalized with pyroGlu-3 Aβ (B) in the neocortex of a 14-month-old hAPP751 mouse. At 14 months, Tg2576 mice displayed some diffuse and focal R1282 IR (C), but no pyroGlu-3 Aβ IR in the neocortex. Tg2576 overexpressing CCL2 displayed both an increase in R1282 IR (E) coinciding with robust pyroGlu-3 Aβ deposition (F) in the neocortex. Interestingly, TgCRND8 at 11 months were one of two murine strains that exhibited R1282 IR (G), as well as pyroGlu-3 Aβ (H) IR in the olfactory bulb. 3xTg-AD displayed robust R1282 IR (I) in the subiculum, which colocalized well with all pyroGlu-3 Aβ–positive plaques on an adjacent, serial section immunolabeled with pyroGlu-3 Aβ (J). Plaques were also observed in 4-month-old 5XFAD mouse in cortical layer V upon staining with R1282 (K) and pyroGlu-3 Aβ (L), respectively. Scale bars = 100 μm (D, F, J, and L); 50 μm (B and H).
Analysis of general Aβ (A–C) and pyroGlu-3 Aβ (D–F) in C3-deficient mice crossed with J20 amyloid precursor protein (APP) tg mice. J20;C3−/− mice show accelerated pyroGlu-3 Aβ deposition in the midfrontal neocortex (F) at 17 months of age when compared to age-matched J20 mice on C57BL/6 (E) and B6D2F1 (D) backgrounds. Increases in R1282 IR in J20;C3−/− mice (C) paralleled increases in cerebral pyroGlu-3 Aβ; however, no changes in R1282 IR were observed between J20 C57BL/6 (B) and J20 B6D2F1 mice (A) at 17 and 16 months, respectively. Scale bar = 100 μm (F).
General Aβ IR (A–C) and pyroGlu-3 Aβ IR (D–F) in neocortex (A and D), olfactory bulb (B and E), and cerebellum (C and F) of PSAPP mice at 18 months of age. Abundant pyroGlu-3 Aβ was deposited in the neocortex and parenchymal blood vessels (asterisk) (D) that colocalized with R1282 IR (A). Both R1282 (B) and pyroGlu-3 Aβ (E) IR were observed in the olfactory bulb of PSAPP mice. Cerebellar Aβ peptides were located exclusively in the leptomeningeal blood vessels for full-length Aβ (C) and pyroGlu-3 Aβ (F). Scale bar = 50 μm (F).
References
Articles from The American Journal of Pathology are provided here courtesy of American Society for Investigative Pathology
Citations & impact
Impact metrics
Citations of article over time
Alternative metrics
Article citations
Metabolic resistance of Aβ3pE-42, a target epitope of the anti-Alzheimer therapeutic antibody, donanemab.
Life Sci Alliance, 7(12):e202402650, 30 Sep 2024
Cited by: 0 articles | PMID: 39348937 | PMCID: PMC11443169
Alzheimer's Disease Neuropathological Change in Aged Non-Primate Mammals.
Int J Mol Sci, 25(15):8118, 25 Jul 2024
Cited by: 0 articles | PMID: 39125687 | PMCID: PMC11311584
Review Free full text in Europe PMC
The Effects of Sevoflurane and Aβ Interaction on CA1 Dendritic Spine Dynamics and MEGF10-Related Astrocytic Synapse Engulfment.
Int J Mol Sci, 25(13):7393, 05 Jul 2024
Cited by: 0 articles | PMID: 39000499
Temporal Characterization of the Amyloidogenic APPswe/PS1dE9;hAPOE4 Mouse Model of Alzheimer's Disease.
Int J Mol Sci, 25(11):5754, 25 May 2024
Cited by: 1 article | PMID: 38891941 | PMCID: PMC11172317
Synthesis and Evaluation of a Novel PET Radioligand for Imaging Glutaminyl Cyclase Activity as a Biomarker for Detecting Alzheimer's Disease.
ACS Sens, 9(5):2605-2613, 08 May 2024
Cited by: 0 articles | PMID: 38718161 | PMCID: PMC11129349
Go to all (69) article citations
Data
Data behind the article
This data has been text mined from the article, or deposited into data resources.
BioStudies: supplemental material and supporting data
Similar Articles
To arrive at the top five similar articles we use a word-weighted algorithm to compare words from the Title and Abstract of each citation.
N-truncated Aβ4-x peptides in sporadic Alzheimer's disease cases and transgenic Alzheimer mouse models.
Alzheimers Res Ther, 9(1):80, 04 Oct 2017
Cited by: 22 articles | PMID: 28978359 | PMCID: PMC5628465
Pyroglutamate Abeta pathology in APP/PS1KI mice, sporadic and familial Alzheimer's disease cases.
J Neural Transm (Vienna), 117(1):85-96, 13 Oct 2009
Cited by: 55 articles | PMID: 19823761 | PMCID: PMC2789212
Phosphorylated Aβ peptides in human Down syndrome brain and different Alzheimer's-like mouse models.
Acta Neuropathol Commun, 8(1):118, 29 Jul 2020
Cited by: 11 articles | PMID: 32727580 | PMCID: PMC7388542
Pyroglutamate-Aβ: role in the natural history of Alzheimer's disease.
Int J Biochem Cell Biol, 42(12):1915-1918, 15 Sep 2010
Cited by: 50 articles | PMID: 20833262
Review
Funding
Funders who supported this work.
NIA NIH HHS (3)
Grant ID: R01 AG040092
Grant ID: AG040092
Grant ID: R01 AG020159
National Institute on Aging (2)
Grant ID: R01 AG020159
Grant ID: AG040092
National Institutes of Health
Wellcome Trust (2)
Mechanisms of neurotoxicity of amyloid aggregates.
Professor Peter St George-Hyslop, University of Cambridge
Grant ID: 089703
Cellular Medicine at the Cambridge Institute for Medical Research.
Prof Gillian Griffiths, University of Cambridge
Grant ID: 100140