Abstract
Purpose
The adoptive transfer of T cells modified to express a chimeric antigen receptor (CAR) comprised of an extracellular single-chain antibody (scFV) fragment specific for a tumor cell surface molecule, and linked to an intracellular signaling module, has activity in advanced malignancies. The receptor tyrosine kinase-like orphan receptor 1 (ROR1) is a tumor-associated molecule expressed in prevalent B-lymphoid and epithelial cancers and is absent on normal mature B cells and vital tissues, making it a candidate for CAR T-cell therapy.Experimental design
We constructed ROR1-CARs from scFVs with different affinities and containing extracellular IgG4-Fc spacer domains of different lengths, and evaluated the ability of T cells expressing each CAR to recognize ROR1(+) hematopoietic and epithelial tumors in vitro, and to eliminate human mantle cell lymphoma (MCL) engrafted into immunodeficient mice.Results
ROR1-CARs containing a short "Hinge-only" extracellular spacer conferred superior lysis of ROR1(+) tumor cells and induction of T-cell effector functions compared with CARs with long "Hinge-CH2-CH3" spacers. CARs derived from a higher affinity scFV conferred maximum T-cell effector function against primary CLL and ROR1(+) epithelial cancer lines in vitro without inducing activation-induced T-cell death. T cells modified with an optimal ROR1-CAR were equivalently effective as CD19-CAR-modified T cells in mediating regression of JeKo-1 MCL in immunodeficient mice.Conclusions
Our results show that customizing spacer design and increasing affinity of ROR1-CARs enhances T-cell effector function and recognition of ROR1(+) tumors. T cells modified with an optimized ROR1-CAR have significant antitumor efficacy in a preclinical model in vivo, suggesting they may be useful to treat ROR1(+) tumors in clinical applications.Free full text

Receptor affinity and extracellular domain modifications affect tumor recognition by ROR1-specific chimeric antigen receptor T-cells
Abstract
Purpose
The adoptive transfer of T-cells modified to express a chimeric antigen receptor (CAR) comprised of an extracellular single chain antibody (scFV) fragment specific for a tumor cell surface molecule, and linked to an intracellular signaling module has activity in advanced malignancies. ROR1 is a tumor-associated molecule expressed on prevalent B-lymphoid and epithelial cancers, and is absent on normal mature B-cells and vital tissues, making it a candidate for CAR T-cell therapy.
Experimental Design
We constructed ROR1-CARs from scFVs with different affinities and containing extracellular IgG4-Fc spacer domains of different lengths, and evaluated the ability of T-cells expressing each CAR to recognize ROR1+ hematopoietic and epithelial tumors in vitro, and to eliminate human mantle cell lymphoma engrafted into immunodeficient mice.
Results
ROR1-CARs containing a short ‘Hinge-only’ extracellular spacer conferred superior lysis of ROR1+ tumor cells and induction of T-cell effector functions compared to CARs with long ‘Hinge-CH2-CH3’ spacers. CARs derived from a higher affinity scFV conferred maximum T-cell effector function against primary CLL and ROR1+ epithelial cancer lines in vitro without inducing activation induced T-cell death. T-cells modified with an optimal ROR1-CAR were equivalently effective as CD19-CAR modified T-cells in mediating regression of JeKo-1 mantle cell lymphoma in immunodeficient mice.
Conclusions
Our results demonstrate that customizing spacer design and increasing affinity of ROR1-CARs enhances T-cell effector function and recognition of ROR1+ tumors. T-cells modified with an optimized ROR1-CAR have significant anti-tumor efficacy in a preclinical model in vivo, suggesting they may be useful to treat ROR1+ tumors in clinical applications.
Introduction
The adoptive transfer of genetically modified T-cells that express a T-cell receptor or a chimeric antigen receptor (CAR) specific for a tumor-associated antigen is emerging as an effective modality for cancer therapy (1-5). CARs are synthetic receptors most often constructed by linking a single chain variable fragment (scFV) of a monoclonal antibody (mAb) specific for a tumor cell surface molecule to a transmembrane domain, one or more intracellular costimulatory signaling modules, and CD3ζ (6-8). CAR-modified T-cells confer non-MHC restricted recognition of tumor cells, and durable responses have been reported in patients with B-cell malignancies after treatment with autologous T-cells modified with CARs specific for the B-cell lineage restricted CD19 molecule. The major toxicities in these patients were related to tumor lysis, cytokine release, and prolonged depletion of normal B-lymphocytes (1-3, 5, 9). A challenge in the field is to identify and validate receptor constructs specific for molecules that are expressed on a greater number of malignancies including common epithelial tumors, and that are restricted in their expression to malignant and not normal cells.
We have been investigating the receptor tyrosine kinase-like orphan receptor 1 (ROR1) as a candidate for immunotherapy with CAR-modified T-cells. ROR1 is a 120-kDa glycoprotein containing extracellular immunoglobulin (Ig)–like, Frizzled, and Kringle domains. ROR1 is expressed during embryogenesis but absent from normal adult tissues, apart from a subset of immature B-cell precursors, and low-level expression on adipocytes (10, 11). ROR1 was first shown to be expressed in B-cell chronic lymphocytic leukemia (B-CLL) by transcriptional profiling (12, 13), and was subsequently identified on the surface of many cancers including mantle cell lymphoma (MCL), acute lymphoblastic leukemia (ALL) with a t(1;19) chromosome translocation, and a subset of lung, breast, colon, pancreas, renal, and ovarian cancers (14-21). In both lung adenocarcinoma and t(1;19) ALL, ROR1 cooperates in oncogenic signaling, and knockdown of ROR1 with siRNA exposed a critical role for this molecule in maintaining tumor cell survival (15, 18, 22, 23). Thus, ROR1 loss may not be readily tolerated by tumors making it an attractive candidate for CAR directed T-cell therapy that could be broadly applied.
We previously described the construction of a ROR1-CAR from the 2A2 mAb that targets a membrane distal epitope in the Ig-like/Frizzled region of ROR1 and demonstrated that T-cells could be re-directed by lentiviral delivery to recognize primary CLL and hematopoietic tumor lines transfected with ROR1 (10). Here, we developed a panel of distinct ROR1-CARs that target the same region of ROR1 but contain modified extracellular spacer domains and differ in scFV affinity. We demonstrate that tailoring the extracellular spacer region and deriving the ROR1-CAR from a scFV with higher affinity improves recognition of hematopoietic tumors in vitro, and that an optimized ROR1-CAR is equivalently effective as a CD19-CAR in an immunodeficient mouse model of human MCL. The higher affinity ROR1-CAR with an optimized spacer domain also confers efficient recognition of epithelial cancer cell lines that express ROR1, suggesting the potential to apply adoptive therapy with ROR1-CAR T-cells for several human malignancies.
Materials and Methods
Human subjects
Peripheral blood mononuclear cells (PBMC) were obtained from healthy donors and patients after written informed consent on research protocols approved by the Institutional Review Board of the Fred Hutchinson Cancer Research Center (FHCRC).
Cell lines
The K562, Raji, JeKo-1, MDA-MB-231, MDA-MB-468, and 293T cell lines were obtained from the American Type Culture Collection. Dr. Edus H. Warren (FHCRC) kindly provided the renal cell cancer lines FARP, TREP and RWL. K562/ROR1 and Raji/ROR1 were generated by lentiviral transduction with the full-length ROR1-gene. JeKo-1/ffluc were derived by lentiviral transduction with the firefly luciferase (ffluc)-gene.
Immunophenotyping
Immunophenotyping was performed with conjugated mAbs: CD3, CD4, CD5, CD8, CD19, CD28, CD45RO, CD62L, CD314 (NKG2D), MICA/B and matched isotype controls (BD Biosciences). Propidium iodide (PI) staining was performed for live/dead cell discrimination. Cell surface expression of ROR1 was analyzed using a polyclonal goat anti-human-ROR1 antibody (R&D Systems) (10). Surface expression of 2A2 ROR1-CARs was analyzed using a polyclonal goat anti-mouse-IgG antibody (Fab-specific) (Jackson ImmunoResearch). Flow analyses were done on a FACSCanto, sort-purifications on a FACSAriaII (Becton Dickinson) and data analyzed using FlowJo software (Treestar).
Vector construction and preparation of CAR-encoding lentivirus
ROR1-specific and CD19-specific CARs were constructed using VL and VH segments of the 2A2 and R12 (ROR1) (24, 25), and FMC63 mAbs (CD19) (26). Each scFV was linked by a (G4S)3 peptide to a spacer domain derived from IgG4-Fc (Uniprot Database: P01861) comprising either ‘Hinge-CH2-CH3’ (229 AA), ‘Hinge-CH3’ (119 AA) or ‘Hinge-only’ (12 AA) sequences. All spacers contained a S→P substitution within the ‘Hinge’ domain located at position 108 of the native IgG4-Fc protein, and were linked to the 27 AA transmembrane domain of human CD28 (Uniprot: P10747) and to a signaling module comprising either (i) the 41 AA cytoplasmic domain of human CD28 with an LL→GG substitution located at positions 186-187 of the native CD28 protein (27) or (ii) the 42 AA cytoplasmic domain of human 4-1BB (Uniprot: Q07011), each of which was linked to the 112 AA cytoplasmic domain of isoform 3 of human CD3ζ (Uniprot: P20963). The construct encoded a T2A ribosomal skip element (28) and a tEGFR sequence downstream of the CAR (29). Codon-optimized nucleotide sequences encoding each transgene were synthesized (Life Technologies) and cloned into the epHIV7 lentiviral vector (29, 30). ROR1-CAR, CD19-CAR or tEGFR-encoding lentiviruses were produced in 293T cells using the packaging vectors pCHGP-2, pCMV-Rev2 and pCMV-G.
Generation of T-cell lines expressing ROR1 and CD19-CARs
CD8+ CD45RO+ CD62L+ central memory T-cells (TCM) or bulk CD4+ T-cells were sorted from PBMC of normal donors, activated with anti-CD3/CD28 beads (Life Technologies), and transduced on day 3 after activation by centrifugation at 800 g for 45 min at 32°C with lentiviral supernatant (MOI = 3) supplemented with 1 μg/mL polybrene (Millipore). T-cells were expanded in RPMI with 10% human serum, 2 mM L-glutamine and 1% penicillin-streptomycin (CTL medium), supplemented with recombinant human IL-2 to a final concentration of 50 U/mL. The tEGFR+ subset of each T-cell line was enriched by immunomagnetic selection with biotin-conjugated anti-EGFR mAb (ImClone Systems) and streptavidin-beads (Miltenyi). ROR1-CAR and tEGFR control T-cells were expanded using a rapid expansion protocol (31), and CD19-CAR T-cells were expanded by stimulation with irradiated (8,000 rad) B-LCL at a T-cell:LCL ratio of 1:7. T-cells were cultured in CTL medium with 50 U/mL IL-2.
Cytotoxicity, cytokine secretion and proliferation assays
Cytotoxicity: Target cells were labeled with 51Cr (PerkinElmer), washed and incubated in triplicate at 1-2×103 cells/well with effector T-cells at various effector to target (E:T) ratios. Supernatants were harvested for γ-counting after a 4-hour incubation and specific lysis calculated using the standard formula. Cytokine secretion: 5×104 T-cells were plated in triplicate with target cells at an E:T ratio of 1:1 (primary CLL), 2:1 (Raji/ROR1; JeKo-1), 4:1 (K562/ROR1, K562/CD19 and K562) or 10:1 (MDA-MB-231), and IFN-γ, TNF-α and IL-2 measured by ELISA or multiplex cytokine immunoassay (Luminex) in supernatant removed after 24-h incubation. In experiments blocking NKG2D signaling, anti-NKG2D (clone 1D11), anti-MICA/B (clone 6D4, all from BD) and anti-ULBP (kindly provided by Dr. Veronika Groh, FHCRC) were used at saturating concentrations. Proliferation: T-cells were labeled with 0.2 μM carboxyfluorescein succinimidyl ester (CFSE, Invitrogen), washed and plated in triplicate with stimulator cells in medium without exogenous cytokines. After 72-h incubation, cells were labeled with anti-CD8 mAb and PI, and analyzed by flow cytometry to assess cell division of live CD8+ T-cells.
Experiments in NOD/SCID/γc-/- (NSG) mice
The Institutional Animal Care and Use Committee approved all mouse experiments. Six-to 8-week old female NOD.Cg-Prkdcscid Il2rgtm1Wjl/SzJ (NSG) mice were obtained from the Jackson Laboratory or bred in-house. Mice were injected with 0.5×106 JeKo-1/ffluc tumor cells via tail vein and received a subsequent tail vein injection of CAR-modified or control T-cells.
For bioluminescence imaging of tumor growth, mice received intraperitoneal injections of luciferin substrate (Caliper Life Sciences) resuspended in PBS (15 μg/g body weight). Mice were anesthetized with isoflurane and imaged using an Xenogen IVIS Imaging System (Caliper) 10, 12 and 14 minutes after luciferin injection in small binning mode at an acquisition time of 1 s to 1 min to obtain unsaturated images. Luciferase activity was analyzed using Living Image Software (Caliper) and the photon flux analyzed within regions of interest that encompassed the entire body or the thorax of each individual mouse.
Statistical analyses
Statistical analyses were performed using Prism Software (GraphPad). Student's t-test was performed as a two-sided paired test with a confidence interval of 95% and results with a p-value of p<0.05 were considered significant. Statistical analysis of survival were done by log-rank testing and results with a p-value of p<0.05 considered significant.
Results
Truncating the spacer domain of the 2A2 ROR1-CAR confers superior recognition of ROR1+ tumors
We previously reported the design of a ROR1-specific CAR using the 2A2 scFV, which binds to an epitope in the NH2-terminal, membrane-distal Ig-like/Frizzled portion of ROR1 (10, 24). The initial 2A2 ROR1-CAR had a long 229 AA spacer that consisted of the ‘Hinge-CH2-CH3’ region of IgG4-Fc, and incorporated CD28 costimulatory and CD3ζ signaling domains. This CAR conferred specific recognition of ROR1+ tumors, but we hypothesized that because of the membrane-distal location of the ROR1 epitope, truncating the spacer domain might enhance tumor recognition and T-cell signaling. Therefore, we constructed 2 additional receptors in which the IgG4-Fc spacer domain was sequentially deleted to derive ‘Hinge-CH3’ (119 AA, intermediate), and ‘Hinge-only’ (12 AA, short) variants. Each of the new receptors contained the identical 2A2 scFV, and CD28 and CD3ζ signaling modules (Suppl. Fig. 1A). The transgene cassette included a truncated EGFR (tEGFR) to serve as a transduction, selection and in vivo tracking marker for CAR-modified T-cells (29).
We transduced purified CD8+ TCM with the 2A2 ROR1-CARs containing full length or truncated IgG4-Fc spacers, and with a tEGFR control vector. The mean transduction efficiency was 15% (range 9-22%), and transgene-positive T-cells were enriched to uniform purity (>90%) on day 10 by selection for tEGFR expression, and expanded (29, 31) (Fig. 1A). Surface expression of each of the CARs was confirmed by staining with F(ab)-specific antibodies (Fig. 1A). Analysis of the in vitro function of CD8+ T-cells modified to express each of the 2A2 ROR1-CARs demonstrated that each CAR conferred specific lysis of JeKo-1 MCL and primary CLL cells that naturally express ROR1, and of K562 cells that had been transduced with ROR1, but did not confer recognition of control ROR1- targets (Fig. 1B). T-cells expressing the short ‘Hinge-only’ 2A2 ROR1-CAR had maximum cytolytic activity, and a hierarchy (short>intermediatelong) of tumor lysis was clearly evident against all ROR1+ tumor targets (Fig. 1B).
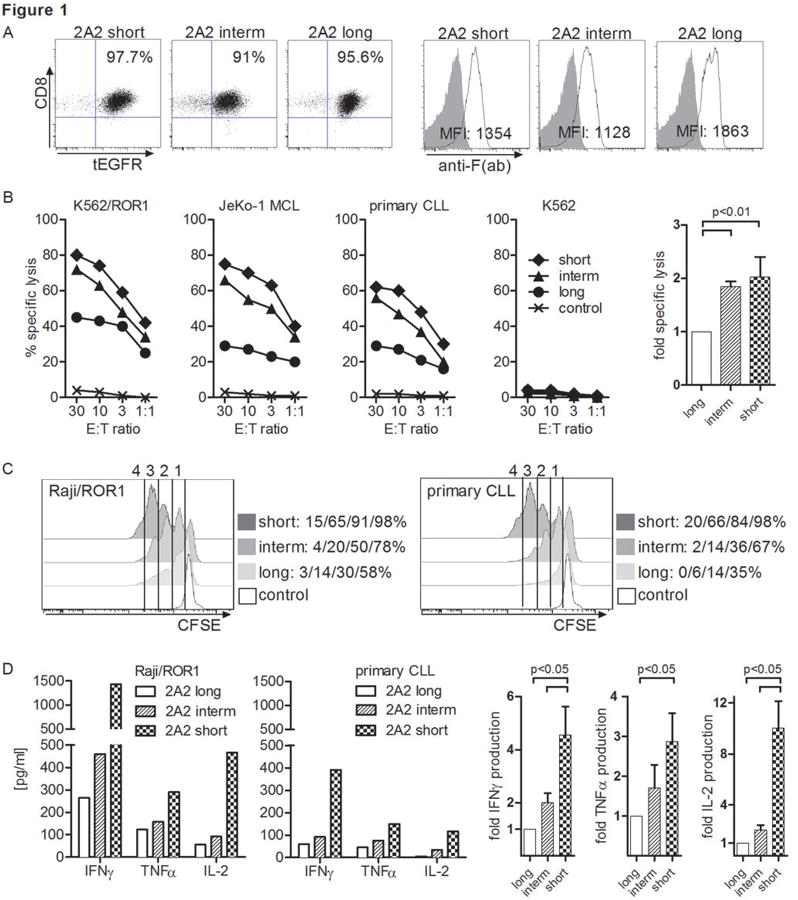
(A) Phenotype of purified CD8+ TCM-derived cell lines modified with each of the 2A2 ROR1-CARs with long, intermediate and short spacer domain. Staining with anti-F(ab) antibody that binds to an epitope in the 2A2 scFV shows surface expression of ROR1-CARs with full length or truncated spacer. (B) Cytolytic activity of T-cells expressing the various 2A2 ROR1-CARs with long, intermediate and short spacer, or a tEGFR control lentiviral vector against ROR1+ and control target cells. The bar diagram summarizes cytotoxicity data from 3 independent experiments (E:T = 30:1) normalized to cytolytic activity by 2A2 ROR1-CAR ‘long’ = 1, and analyzed by Student's t-test. (C) CFSE dye dilution was used to measure proliferation of 2A2 ROR1-CAR and tEGFR control T-cells, 72 hours after stimulation with Raji/ROR1 (left panel) and primary CLL cells (right panel) without addition of exogenous cytokines. For analysis, triplicate wells were pooled and the proliferation of live (PI-), CD8+ T-cells analyzed. Numbers above each histogram indicate the number of cell divisions the proliferating subset underwent, and the fraction of T-cells in each gate that underwent ≥4/3/2/1 cell divisions is provided next to each plot. (D) Multiplex cytokine assay of supernatants obtained after 24 hours from triplicate co-cultures of 5×104 T-cells expressing the various 2A2 ROR1-CARs with Raji/ROR1 and primary CLL cells. Multiplex cytokine data from 3 independent experiments were normalized (cytokine release by 2A2 ROR1-CAR ‘long’ = 1) and analyzed by Student's t-test (right bar diagram).
Anti-tumor efficacy of adoptive T-cell therapy correlates with proliferation and survival of transferred T-cells, which could be altered by signaling through the CAR. We used CFSE dilution assays to analyze proliferation of T-cells modified with each of the 2A2 ROR1-CARs after engagement of Raji/ROR1 or CLL, and found that the short spacer construct promoted the greatest T-cell proliferation following stimulation (Fig. 1C). To ensure that the enhanced proliferation was not associated with greater activation induced cell death (AICD), we also analyzed the proportion of T-cells that stained with propidium iodide (PI) after stimulation with Raji/ROR1 and JeKo-1 tumor cells. We detected a much lower frequency of PI+ CD8+ T-cells in the 2A2 ROR1-CAR T-cell line modified with the short (Raji/ROR1: 17.2%/JeKo-1: 20.2%) compared to the intermediate (41.6%/42.4%) and long (44.5%/48.5%) spacers. Quantitative analysis of cytokine production in response to stimulation with Raji/ROR1 and primary CLL cells showed production of IFN-γ, TNF-α and IL-2 by T-cells expressing each of the 2A2 ROR1-CARs. As observed in cytotoxicity assays, the short spacer construct was superior in mediating cytokine secretion after tumor recognition (Fig. 1D). Thus, this analysis shows that truncating the extracellular IgG4-Fc spacer domain of the 2A2 ROR1-CAR leads to a significant increase in cytotoxicity, proliferation and in vitro effector functions after tumor recognition.
ROR1-CARs derived from a mAb R12 with higher affinity than 2A2 mediate superior anti-tumor reactivity
We next examined whether increasing the affinity of the scFV used to construct the ROR1-CAR might influence tumor recognition and T-cell function. We generated ROR1-specific CARs from the mAb R12 that like 2A2, binds to an epitope in the NH2-terminal Ig-like/Frizzled domain of ROR1 but with >50-fold higher monovalent binding affinity (24, 25). R12 ROR1-CARs were constructed with both long and short IgG4-Fc spacers to determine whether the optimal spacer design for this higher affinity scFV differed from that for a lower affinity scFV. We found that similar to 2A2, the short spacer R12 ROR1-CAR conferred improved cytolytic activity, cytokine secretion and proliferation (data not shown), suggesting that the shorter spacer length provides superior spatial engagement of the T-cell and ROR1+ target cell for T-cell activation.
We then designed R12 and 2A2 ROR1-CARs that contained an optimal (short) extracellular spacer, and either a CD28 or 4-1BB costimulatory domain in tandem with CD3ζ (4 constructs) for comparison (Suppl. Fig. 1B). These ROR1-CAR constructs were expressed in purified CD8+ TCM of healthy donors, and we confirmed equivalent transgene expression by tEGFR staining (Fig. 2A). T-cells modified with each of the 2A2 and R12 ROR1-CARs specifically lysed K562/ROR1 and Raji/ROR1 tumor cells with approximately equivalent efficiency (Fig. 2B). However, analysis of cytokine production showed that the high affinity R12 ROR1-CARs that contained CD28 or 4-1BB conferred significantly higher IFN-γ, TNF-α and IL-2 production compared to the corresponding 2A2 constructs (Fig. 2C). Consistent with previous work, we found that T-cells expressing CARs with a CD28 costimulatory domain produced more IFN-γ, TNF-α and IL-2 compared to those with 4-1BB (32-34). Experiments to analyze the proliferation of ROR1-CAR T-cells showed a higher percentage of proliferating T-cells and a higher number of cell divisions in T-cells expressing the high affinity R12 ROR1-CARs with CD28 and 4-1BB domain compared to T-cells expressing the respective 2A2 counterparts (Fig. 2D). There was more vigorous proliferation in T-cells that expressed CARs with a CD28 domain, consistent with higher IL-2 production induced by these receptors. There was a lower frequency of AICD as measured by PI staining in T-cell lines modified with R12 compared to 2A2 ROR1-CARs after stimulation with Raji/ROR1 and JeKo-1 tumor cells respectively (R12: 5.6%/6.9% vs. 2A2: 10%/9.65%), and T-cell lines that expressed CARs with CD28 domain had lower AICD compared to 4-1BB (R12: 16.4%/18.4% vs. 2A2 38.1%/39.6%).
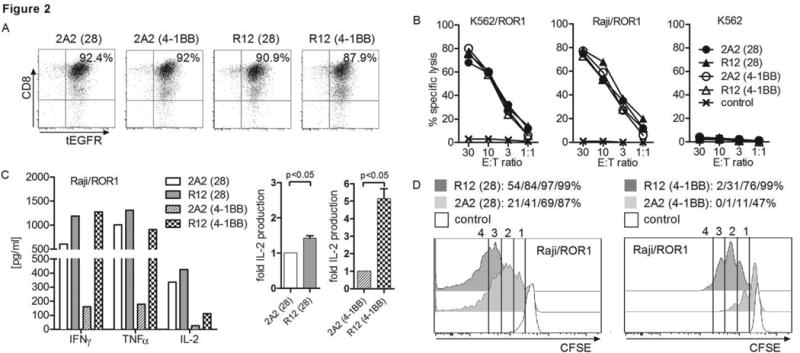
(A) tEGFR expression on purified polyclonal CD8+ TCM-derived T-cell lines modified with each of the R12 and 2A2 ROR1-CARs with short IgG4-Fc ‘Hinge’ spacer, and CD28 or 4-1BB costimulatory domain. (B) Cytotoxicity against ROR1+ and control target cells by T-cells expressing R12 and 2A2 ROR1-CARs or a tEGFR control vector. (C) Multiplex cytokine assay of supernatants obtained after 24 hours from co-cultures of 5×104 T-cells expressing the various ROR1-CARs with Raji/ROR1 tumor cells. The middle/right bar diagrams show normalized multiplex data from 3 independent experiments (cytokine release by ROR1-CAR 2A2 = 1) analyzed by Student's t-test. (D) Proliferation of ROR1-CAR T-cells and tEGFR control T-cells 72 hours after stimulation with Raji/ROR1 cells and without addition of exogenous cytokines was assessed by CFSE dye dilution. Numbers above each histogram indicate the number of cell divisions the proliferating subset underwent, and the fraction of T-cells in each gate that underwent ≥4/3/2/1 cell divisions is provided above each plot.
To determine if the enhanced function observed with R12 ROR1-CARs in CD8+ T-cells extended to CD4+ T-cells, we transduced bulk CD4+ T-cells with the 2A2 and R12 ROR1-CARs containing the short spacer and CD28 costimulatory domain. In response to Raji/ROR1+ tumor cells, CD4+ T-cells that expressed the high affinity R12 scFV produced higher levels of IFN-γ, TNF-α, IL-2, IL-4, and IL-10, and underwent greater proliferation than CD4+ T-cells that expressed 2A2 (Suppl. Fig. 2A, B). Both cytokine production and proliferation was superior in CD4+ compared to CD8+ T-cells modified with the same ROR1-CARs. In summary, our data demonstrate that tailoring both the length of the non-signaling extracellular CAR spacer domain and scFV affinity are independent parameters that affect the function of ROR1-CAR T-cells.
CD8+ T-cells modified with a high affinity ROR1-CAR have comparable activity to a CD19-CAR against primary CLL in vitro
ROR1 and CD19 are both uniformly expressed on all primary CLL (Fig. 3A), however the absolute number of ROR1-molecules per tumor cell is estimated to be 10-fold lower than that of CD19, which has been successfully targeted in clinical trials with CD19-CAR T-cells (1, 5, 10, 14). We compared recognition of primary CLL by CD8+ T-cells expressing the optimized R12 and 2A2 ROR1-CARs, and a CD19-CAR derived from the FMC63 scFV (1-3, 5, 35). We used purified CD8+ TCM for CAR-modification to provide a uniform cell product and each CAR contained a short IgG4-Fc ‘Hinge-only’ spacer and 4-1BB costimulatory domain. We confirmed our CD19-CAR (IgG4 Hinge) was at least equivalently effective in recognizing CD19+ tumors as a CD19-CAR with CD8a Hinge spacer and 4-1BB costimulatory domain that is being used in ongoing clinical trials (Suppl. Fig. 3A-C) (1, 5). The cytolytic activity of R12 ROR1-CAR T-cells against primary tumor cells from multiple CLL patients (n=4) was higher compared to T-cells modified with the lower affinity 2A2 ROR1-CAR, and equivalent to the lysis observed with CD19-CAR T-cells (Fig. 3B). Multiplex cytokine analysis showed nearly equivalent production of IFN-γ and TNF-α, but less IL-2 production by CD8+ T-cells expressing the R12 ROR1 compared with those expressing the CD19-CAR after co-culture with primary CLL (Fig. 3C). 2A2 ROR1-CAR T-cells produced lower amounts of all cytokines than R12 ROR1-CAR T-cells as noted previously. Cytokine production by all of the CAR-transduced T-cells after stimulation with CLL was substantially less than with Raji/ROR1, which unlike CLL expresses both CD80 and CD86 that can engage CD28 expressed on CAR T-cells (Fig. 3A, C).
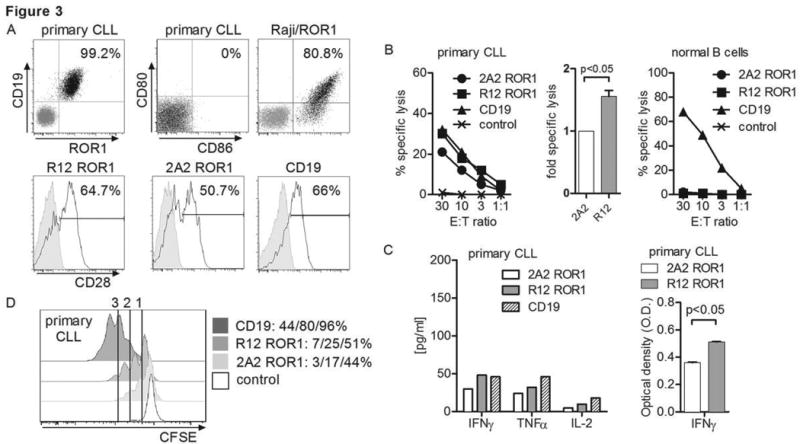
(A) Expression of ROR1/CD19 on primary CLL, and CD80/86 on primary CLL and Raji/ROR1 tumor cells (black dot plots) that can engage CD28 on CAR T-cells (black histograms). Staining with matched isotype control mAbs is shown as grey dot plots/histograms. (B) Cytolytic activity of T-cells expressing the 2A2 and R12 ROR1-CAR, a CD19-specific CAR and T-cells modified with a tEGFR control vector against primary CLL (left diagram) and normal B cells (right diagram) analyzed by chromium release assay. Cytotoxicity data against primary CLL from 4 independent experiments (E:T = 30:1) were normalized (cytolytic activity by ROR1-CAR 2A2 = 1) and analyzed by Student's t-test (bar diagram). (C) Multiplex cytokine analysis after a 24 hour stimulation of 5×104 CAR T-cells with primary CLL cells. Cytokine release of unstimulated CAR T-cells was below 3.6 pg/mL (detection limit) (left bar diagram). ELISA for IFN-γ production by 5×104 2A2 and R12 ROR1-CAR T-cells after a 24-hour co-culture with primary CLL. O.D. of 1 corresponds to approximately 250 pg/mL (right bar diagram). (D) Proliferation of CD8+ T-cells modified with the 2A2 ROR1, R12 ROR1 and a CD19-CAR, 72 hours after stimulation with primary CLL cells. Numbers above each histogram indicate the number of cell divisions, and the fraction of T-cells in each gate that underwent ≥3/2/1 cell divisions is provided next to each plot.
We observed less proliferation of T-cells expressing the R12 and 2A2 ROR1-CAR compared to the CD19-CAR after stimulation with CLL (CD19>R12>2A2) (Fig. 3D). We hypothesized that proliferation of CD8+ ROR1-CAR T-cells in response to CLL may be augmented in the presence of CAR-modified CD4+ T-cells because of their higher secretion of IL-2 compared to CD8+ TCM (Suppl. Fig. 2A; Suppl. Fig. 4A). To test this possibility, we performed in vitro co-culture experiments where CD4+ and CD8 TCM were separately modified with the R12 ROR1, 2A2 ROR1 and CD19-CARs respectively, enriched for CAR expression, and combined at a 1:1 ratio to ensure equivalent proportions of CD8+ and CD4+ T-cells modified with each of the vectors. These cells were CFSE-labeled and stimulated with primary CLL. We observed a dramatic increase in proliferation of CD8+ R12 ROR1-CAR T-cells after addition of CAR-transduced, but not untransduced CD4+ T-cells (Suppl. Fig. 4B). Notably, when provided with CD4-help, we observed equivalent proliferation of R12 ROR1 and CD19-CAR CD8+ T-cells in response to CLL, whereas proliferation of CD8+ T-cells expressing the lower affinity 2A2 ROR1-CAR remained less. Collectively, our data show that the high affinity R12 ROR1-CAR confers superior reactivity compared to 2A2 against primary CLL cells in vitro.
ROR1-CAR T-cells mediate in vivo anti-tumor activity in a mouse model of systemic mantle cell lymphoma
It remained uncertain whether the superior in vitro activity of T-cells modified with the higher affinity R12 CAR would translate into improved anti-tumor activity in vivo, and how targeting ROR1 would compare to CD19. To address these questions, we inoculated cohorts of immunodeficient NSG mice with the human MCL line JeKo-1/ffluc by tail vein injection, and seven days later when tumor was disseminated, treated the mice with a single intravenous dose of R12 ROR1, 2A2 ROR1 or CD19-CAR CD8+ T-cells. Control mice were treated with tEGFR T-cells or untreated. All CARs had the optimal short spacer and the 4-1BB costimulatory domain. Untreated NSG/JeKo-1 mice developed a rapidly progressive systemic lymphoma necessitating euthanasia approximately 4 weeks after tumor inoculation (Fig. 4A-C). We observed tumor regression and improved survival in all mice treated with R12 ROR1, 2A2 ROR1 and CD19-CAR T-cells. Mice treated with R12 ROR1-CAR T-cells had a superior anti-tumor response and survival compared to mice treated with 2A2 ROR1-CAR T-cells (p<0.01), and comparable anti-tumor activity to mice treated with CD19-CAR T-cells (Fig. 4A-C).
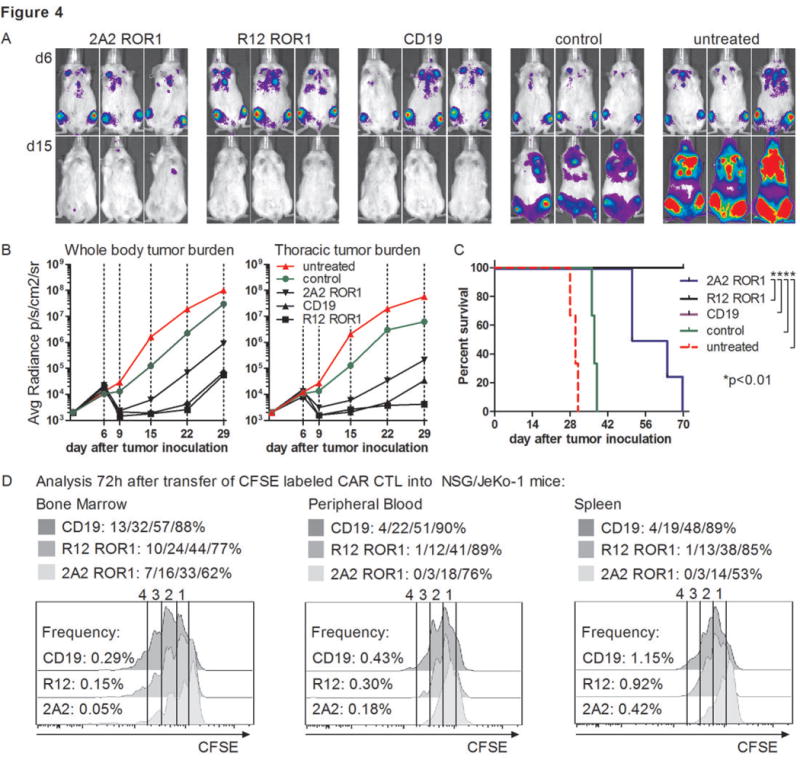
Cohorts of mice were inoculated with 0.5×106 JeKo-1/ffluc MCL via tail vein injection, and 5×106 2A2 ROR1, R12 ROR1 or CD19-CAR T-cells, or T-cells expressing a tEGFR control vector were administered 7 days after tumor inoculation. All CAR constructs had the short IgG4 ‘Hinge-only’ spacer and a 4-1BB costimulatory domain. (A, B) Serial bioluminescence imaging of tumor in cohorts of mice treated with T-cells expressing the 2A2 ROR1-CAR, the high affinity R12 ROR1-CAR, a CD19-specific CAR, with T-cells transduced with tEGFR alone, and untreated mice. Bioluminescence imaging showed tumor manifestations in the bone marrow and thorax and thus, signal intensity was measured in regions of interest that encompassed the entire body and thorax of each individual mouse. (C) Kaplan-Meier analysis of survival in individual treatment and control groups. Statistical analyses were performed using the log-rank test. The data shown in A-C are representative of results obtained in 2 independent experiments. (D) Proliferation of 2A2 ROR1, R12 ROR1 and CD19-CAR T-cells in vivo. Tumor bearing NSG/JeKo-1 mice received a single dose of 5×106 CFSE-labeled 2A2 ROR1, R12 ROR1 or CD19-CAR T-cells on day 7 after tumor inoculation, and 72 h later peripheral blood, bone marrow and spleen were collected from each individual mouse. The frequency and proliferation of live (PI-), CD45+ CD8+ tEGFR+ T-cells was analyzed. The frequency of 2A2 ROR1, R12 ROR1 and CD19-CAR T-cells respectively is provided on the left of each histogram as percentage of live cells, and the fraction of T-cells that underwent ≥4/3/2/1 cell divisions is provided above each plot.
We analyzed the frequency of CAR T-cells in the peripheral blood following adoptive transfer and detected higher numbers of tEGFR+ T-cells in mice treated with the R12 CAR compared to the 2A2 ROR1-CAR, suggesting more vigorous proliferation in vivo improved tumor control. To confirm this, we administered CFSE-labeled CD19-CAR, R12 and 2A2 ROR1-CAR T-cells to cohorts of NSG mice bearing JeKo-1/ffluc, and analyzed T-cell proliferation in the peripheral blood, bone marrow and spleen 72 hours after transfer. A higher percentage of the R12 and CD19-CAR T-cells proliferated and underwent a greater number of cell divisions compared to 2A2 ROR1-CAR T-cells (Fig. 4D). The JeKo-1 tumor eventually recurred in all mice treated with ROR1 or CD19-CAR T-cells (Fig. 4A-C). Tumor recurrence was not a result of the selection of ROR1 or CD19 loss variants, as recurrent tumors were positive for both molecules. For comparison, we analyzed anti-tumor efficacy of CD19-CAR T-cells in NSG mice engrafted with ROR1- Raji tumors and observed complete tumor eradication, indicating the recurrence of JeKo-1 reflects difficulty eradicating this tumor (data not shown). In summary, this data is the first to show that ROR1-CAR T-cells have anti-tumor efficacy in vivo, and suggest that for B-cell malignancies, an optimized ROR1-CAR such as R12 may be effective and spare normal CD19+ B-cells that lack ROR1 expression (10, 14).
T-cells expressing the R12 ROR1-CAR have superior reactivity compared to 2A2 against ROR1+ epithelial tumor cells
ROR1 has been detected on many epithelial tumors, although it is unknown whether ROR1 expression is sufficient for recognition by ROR1-CAR T-cells (18-20). Using flow cytometry, we confirmed ROR1 expression on breast cancer lines MDA-MB-231 and 468, and on the renal cell carcinoma lines FARP, TREP, and RWL (Suppl. Fig. 5A). We then analyzed tumor recognition by CD8+ T-cells transduced with the R12 ROR1-CARs with the optimal short spacer and 4-1BB domain, and observed efficient recognition of MDA-MB-231, MDA-MB-468, FARP, TREP and RWL (Fig. 5A). We analyzed cytokine secretion and proliferation of T-cells modified with the R12 and 2A2 ROR1-CARs after co-culture with MDA-MB-231, and observed greater cytokine production and proliferation with the R12 ROR1-CAR (Fig. 5B, C). Similar to what we observed with ROR1+ B cell malignancies, the superior activation of R12 ROR1-CAR T-cells after stimulation with MDA-MB-231 was not associated with increased AICD (R12: 9.8% vs. 2A2: 10.9%).
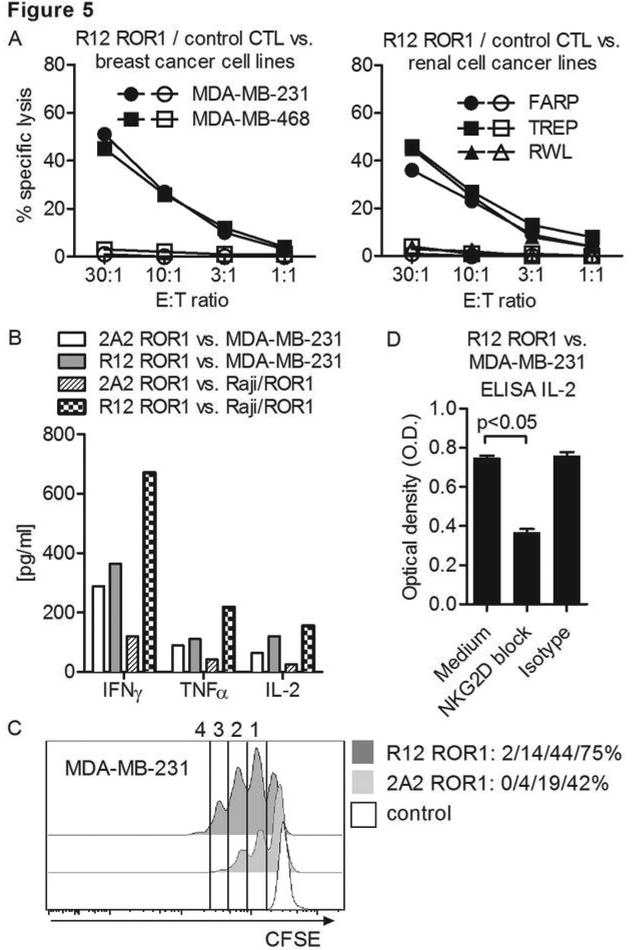
(A) Chromium release assay to evaluate the cytolytic activity of R12 ROR1-CAR modified T-cells (short spacer/4-1BB domain, closed symbols) and tEGFR control T-cells (open symbols) against ROR1+ breast cancer and renal cell cancer lines. (A-D) The 2A2 and R12 ROR1-CARs had the optimal short spacer and a 4-1BB costimulatory domain. (B) Multiplex cytokine analysis after stimulation of T-cells expressing the 2A2 and R12 ROR1-CAR with MDA-MB-231 and Raji/ROR1 tumor cells. (C) Proliferation of CD8+ T-cells modified with the 2A2 and R12 ROR1-CAR 72 hours after stimulation with MDA-MB-231 tumor cells. For analysis, triplicate wells were pooled and the proliferation of live (PI-), CD8+ T-cells analyzed. Numbers above each histogram indicate the number of cell divisions the proliferating subset underwent, and the fraction of T-cells in each gate that underwent ≥4/3/2/1 cell divisions is provided next to each histogram. (D) ELISA for IL-2 production by R12 ROR1-CAR T-cells after a 24-hour co-culture with MDA-MB-231 in plain medium, and after addition of an antibody cocktail blocking of the NKG2D pathway [anti-NKG2D (clone 1D11), anti-MICA/B (clone 6D4) and anti-ULBP] or matched isotype control mAbs. O.D. of 0.6 corresponds to approximately 1900 pg/mL.
Surprisingly, the difference in cytokine secretion between 2A2 and R12 CAR T-cells in response to ROR1+ epithelial tumor cells was less than that observed with ROR1+ lymphoma. Since both targets lack CD80 and CD86, this suggested MDA-MB-231 might express alternative costimulatory ligands that augment the function of CAR T-cells. Many epithelial tumors including MDA-MB-231 express multiple NKG2D ligands (36), and we confirmed a high level of expression of MICA/B on MDA-MB-231 but not on Raji/ROR1 (Suppl. Fig. 5B). The addition of a cocktail of mAbs specific for NKG2D ligands but not matched isotype control mAbs to co-cultures of MDA-MB-231 and ROR1-CAR T-cells resulted in a marked reduction of IL-2 secretion consistent with costimulation being provided through the NKG2D pathway (Fig. 5D). Taken together, our data show for the first time that ROR1+ epithelial tumors can be targeted with ROR1-CAR T-cells and suggest that expression of NGK2D ligands on epithelial cancer cells may have a favorable effect on CAR T-cell functions.
Discussion
ROR1 has attracted interest as a potential target for cancer immunotherapy due to its expression on the surface of many B-lymphoid and epithelial cancers, including subsets of lung, colorectal and renal cell cancer (10, 14, 15, 18-21). We previously showed that CLL and MCL were specifically recognized by T-cells modified to express a ROR1-specific CAR (10). Here we extend this work by improving the design and function of ROR1-CARs through modification of the extracellular spacer domain and deriving the CAR from an scFV of higher affinity, and demonstrate that T-cells modified with optimally designed ROR1-CARs have in vivo activity against ROR1+ B-cell lymphoma and in vitro activity against a wide range of epithelial tumors.
Efforts to improve the functions of CARs have typically focused on the intracellular signaling domain. A consensus has emerged, based primarily on in vitro data, that second generation CARs that provide costimulation through CD28 or 4-1BB are superior to CARs that contain only CD3ζ as a signaling moiety, and that including both CD28 and 4-1BB in tandem may provide an additional benefit for some CARs (32-35, 37, 38). A previous study also showed that the distance of the target epitope from the cell membrane is an important parameter for CD22-specific CARs, and a long spacer improved tumor recognition by 5T4- and NCAM-specific CARs that recognize membrane proximal epitopes, putatively because the spacer provided flexibility required for efficient target binding (39, 40). These studies suggested the need to tailor the extracellular spacer domain for different target molecules. We compared the function of T-cells modified with ROR1-CARs derived from the 2A2 mAb that contained either the original long IgG4-Fc ‘Hinge-CH2-CH3’ spacer that we have shown enables high level cell surface expression (10, 41), or truncated intermediate ‘Hinge-CH3’ and short ‘Hinge-only’ spacer variants. We preserved the 12 AA Hinge domain in our short spacer construct based on prior data that a flexible spacer was required for separating the scFV from the T-cell membrane and allowing antigen recognition on tumor cells (42). Our studies with the 2A2 ROR1-CAR show that T-cell cytokine secretion and proliferation after tumor cell recognition are superior with the intermediate and short spacer constructs compared to the long spacer construct. Staining with anti-F(ab) Abs showed equivalent CAR expression of all three receptors, demonstrating the improved T-cell function with the short spacer CAR was not due to differences in CAR density. This data is consistent with prior studies that spatial requirements are important for the interaction between CAR T-cells and tumor cells, and reaffirms the principle that the design of extracellular spacers should be tailored for each target molecule and epitope.
The affinity of the scFV selected for designing a CAR is an additional parameter that could affect T-cell recognition. We previously generated and characterized a panel of ROR1-specific mAbs of different affinities and selected the R12 mAb, which recognizes an epitope in the Ig-like/Frizzled region as 2A2. R12 has a higher affinity for ROR1-protein due to a much slower dissociation (24, 25). The R12 CAR, like the 2A2 CAR conferred optimal T-cell recognition and function when designed with a short extracellular spacer. A direct comparison of proliferation and cytokine production after tumor engagement by T-cells modified with the 2A2 and R12 CARs demonstrated that the R12 CAR derived from the higher affinity mAb was superior. We were concerned that the slower dissociation of R12 from ROR1 could prolong T-cell activation and confer an increased susceptibility to AICD. However, we detected a lower rate of AICD in T-cells modified with the R12 ROR1-CAR compared to 2A2, demonstrating that the increased affinity of R12 had no detrimental effect on T-cell survival in our preclinical models. It is conceivable that when T-cells are administered to patients, factors such as tumor burden and infusion into a lymphodepleted environment may influence the susceptibility of CAR T-cells to AICD, reinforcing the need to monitor the fate of CAR T-cells in clinical trials. It is uncertain if CARs with higher affinity than R12 would be superior as it is presumed there will be a threshold for CAR affinity beyond which T-cells may undergo AICD. We are attempting to derive higher and lower affinity variants of R12 by mutating the CDR3 region to address this.
ROR1 has a potential advantage over CD19 as a target for CLL and MCL since it is not expressed on normal mature naïve and memory B-cells. However, there is a lower number of ROR1 molecules on B-cell tumors compared with CD19 and it is uncertain if an optimized ROR1-CAR would be as effective as a CD19-CAR similar in design to those being used in the clinic (1-3, 5, 14). Unfortunately, B-cell tumor xenograft models used previously in NSG mice to evaluate the function of CD19-CAR T-cells including Raji, Daudi and Nalm-6, are not derived from CLL or MCL and do not constitutively express ROR1. Thus, to compare targeting CD19 and ROR1 in vivo, we used the JeKo-1 MCL cell line, which naturally expresses both CD19 and ROR1 and engrafts in NSG mice. To make our model clinically relevant, we inoculated JeKo-1 lymphoma cells intravenously to generate systemic tumors, and treated mice with T-cell products of uniform consistency once tumors were established. We found that T-cells expressing the high affinity R12 CAR conferred equivalent anti-tumor activity in vivo as CD19-CAR T-cells. Consistent with our in vitro analysis, the R12 ROR1-CAR also mediated superior activity in vivo compared to the optimal 2A2 ROR1-CAR. These results should be interpreted cautiously since murine tumor models may not predict the efficacy of adoptive therapy in clinical settings. However, the results suggest that ROR1 warrants consideration as an alternative to CD19, or to provide an additional target to minimize the potential for CD19 loss variants to emerge.
ROR1 appears to play a decisive role in survival of some epithelial tumors (18, 22). Thus, an advantage of targeting ROR1 is that a single CAR may be useful to treat patients with a large number of hematopoietic and non-hematopoietic tumors. Our data shows for the first time that T-cells that express an optimally designed ROR1-CAR efficiently recognize epithelial cancers in vitro. Cytokine secretion and T-cell proliferation induced by ROR1+ breast cancer cells were higher than that induced by leukemia cells, despite the absence of the CD80/86 costimulatory ligand. This at least in part reflects the expression of NKG2D ligands on epithelial tumor cell lines that can engage NKG2D and costimulate CAR T-cells (36). Whether these ligands will serve to costimulate CAR T-cells in vivo or inhibit recognition as reported for tumor infiltrating lymphocytes will require careful analysis in clinical trials (43).
The studies reported here demonstrate that the design of the extracellular spacer domain and CAR affinity are parameters that can be modulated to enhance the recognition of ROR1+ hematologic and epithelial tumors in vitro and in vivo by ROR1-CAR modified T-cells. The development of ROR1-CARs with enhanced tumor reactivity provides the opportunity for clinical applications in a variety of human cancers. On-target toxicities have been observed in clinical trials with CAR T-cells specific for antigens that are shared on some normal cells (9, 44), and a critical issue to be addressed is whether targeting ROR1 will be safe. We have shown previously that ROR1 is absent in all vital organs, but detected a low level of expression in adipose tissues, pancreas, and a subset of immature B-cells, illustrating the potential for toxicity to normal cells (10). The development of higher affinity ROR1-CARs with greater anti-tumor activity could theoretically increase the risk of on-target toxicity and mandates careful safety studies in a relevant model. We have designed ROR1-CARs that bind to the murine and non-human primate isoforms of ROR1, and toxicity studies are ongoing in our laboratory to inform the potential to translate ROR1-specific T-cell therapy to cancer patients.
Acknowledgments
The authors thank Cynthia Nourigat, Melissa Comstock and LaKeisha Perkins (Shared Resources, FHCRC) for expertise in performing the mouse experiments. We thank Edus H. Warren and Veronika Groh for cells lines and reagents.
Grant support: This work was supported by the Lembersky family and by grants from the National Institutes of Health CA136551 (S.R.R., M.C.J.), CA114536 and P50CA138293-01 (S.R.R.) and the University of Würzburg (Interdisziplinäres Zentrum für Klinische Forschung, IZKF, Z-4/109 and D-244). M.H. was supported by the Leukemia and Lymphoma Society (LLS). M.H. and D.S. were supported by the German Research Foundation (DFG, Deutsche Forschungsgemeinschaft).
Author contributions: M.H. designed and performed research, analyzed data, and wrote the manuscript. M.T.L.S. and D.S. designed and performed research and analyzed data. P.L.K. performed research and analyzed data. M.C.J. and C.R. provided key reagents and expert advice, and designed experiments. S.R.R. designed and analyzed experiments and wrote the manuscript.
Footnotes
Conflict of interest disclosure: The authors declare no relevant conflicts of interest.
References
Full text links
Read article at publisher's site: https://doi.org/10.1158/1078-0432.ccr-13-0330
Read article for free, from open access legal sources, via Unpaywall:
https://clincancerres.aacrjournals.org/content/clincanres/19/12/3153.full.pdf
Citations & impact
Impact metrics
Article citations
Split-design approach enhances the therapeutic efficacy of ligand-based CAR-T cells against multiple B-cell malignancies.
Nat Commun, 15(1):9751, 11 Nov 2024
Cited by: 0 articles | PMID: 39528513 | PMCID: PMC11555413
Strategies for Improving CAR T Cell Persistence in Solid Tumors.
Cancers (Basel), 16(16):2858, 16 Aug 2024
Cited by: 1 article | PMID: 39199630 | PMCID: PMC11352972
Review Free full text in Europe PMC
Novel OX40 and 4-1BB derived spacers enhance CD30 CAR activity and safety in CD30 positive lymphoma models.
Mol Ther, 32(10):3504-3521, 29 Jun 2024
Cited by: 1 article | PMID: 38946142
Artificial Intelligence-Powered Molecular Docking and Steered Molecular Dynamics for Accurate scFv Selection of Anti-CD30 Chimeric Antigen Receptors.
Int J Mol Sci, 25(13):7231, 30 Jun 2024
Cited by: 1 article | PMID: 39000338
Chimeric antigen receptor T cell therapy for autoimmune disease.
Nat Rev Immunol, 24(11):830-845, 03 Jun 2024
Cited by: 1 article | PMID: 38831163
Review
Go to all (332) article citations
Data
Data behind the article
This data has been text mined from the article, or deposited into data resources.
BioStudies: supplemental material and supporting data
Genes & Proteins (4)
- (1 citation) UniProt - P20963
- (1 citation) UniProt - P10747
- (1 citation) UniProt - P01861
- (1 citation) UniProt - Q07011
Similar Articles
To arrive at the top five similar articles we use a word-weighted algorithm to compare words from the Title and Abstract of each citation.
The B-cell tumor-associated antigen ROR1 can be targeted with T cells modified to express a ROR1-specific chimeric antigen receptor.
Blood, 116(22):4532-4541, 11 Aug 2010
Cited by: 173 articles | PMID: 20702778 | PMCID: PMC2996114
Sleeping Beauty Transposition of Chimeric Antigen Receptors Targeting Receptor Tyrosine Kinase-Like Orphan Receptor-1 (ROR1) into Diverse Memory T-Cell Populations.
PLoS One, 10(6):e0128151, 01 Jun 2015
Cited by: 32 articles | PMID: 26030772 | PMCID: PMC4451012
The nonsignaling extracellular spacer domain of chimeric antigen receptors is decisive for in vivo antitumor activity.
Cancer Immunol Res, 3(2):125-135, 11 Sep 2014
Cited by: 313 articles | PMID: 25212991 | PMCID: PMC4692801
Design and implementation of adoptive therapy with chimeric antigen receptor-modified T cells.
Immunol Rev, 257(1):127-144, 01 Jan 2014
Cited by: 102 articles | PMID: 24329794 | PMCID: PMC3991306
Review Free full text in Europe PMC
Funding
Funders who supported this work.
NCI NIH HHS (7)
Grant ID: P01 CA018029
Grant ID: P50 CA138293
Grant ID: R01 CA136551
Grant ID: CA114536
Grant ID: P50CA138293-01
Grant ID: R01 CA114536
Grant ID: CA136551
NIDDK NIH HHS (1)
Grant ID: P30 DK056465