Abstract
Free full text

Use of ex vivo and in vitro cultures of the human respiratory tract to study the tropism and host responses of highly pathogenic avian influenza A (H5N1) and other influenza viruses
The tropism of influenza viruses for the human respiratory tract is a key determinant of host-range, and consequently, of pathogenesis and transmission. Much of the available data on the tropism of influenza viruses in the human respiratory tract has been derived from autopsy studies of fatal influenza. While this provides important insights into the cells and tissues infected by different respiratory viruses, these data are of necessity restricted to late-stages of severe influenza disease, often following mechanical ventilation. Of the available animals models, only ferrets (and possibly guinea pigs in relation to transmission) are regarded as relevant surrogates for human influenza infection. Nevertheless, ferrets are not humans, and studies of the human respiratory tract remains of paramount importance.
The human respiratory tract is sub-divided into the upper respiratory tract including the nasopharynx and larynx, the conducting airways (trachea and bronchi) and the lower respiratory tract which comprises of the lung parenchyma. Each of these anatomical regions has different types of epithelial cells and influenza viruses differ in their tropism for different cell types at these sites.
The tropism of an influenza virus for the upper, conducting and lower airways has consequences for transmission and pathogenesis. Viruses with tropism for the alveolar epithelium may well cause severe viral pneumonia but may be less transmissible from person-to-person, while those that infect the upper airways are likely to be more transmissible but may cause less severe disease. Thus, experimental models that reflect these anatomical and physiological differences are relevant.
Available experimental models used for assessment of virus tropism and replication competence in humans include a) ex vivo cultures of human respiratory tissues removed at biopsy or surgical excision which are excess to diagnostic requirements, b) primary cells from the human respiratory tract, used with or without differentiation in air-liquid interface cultures, or c) continuous cell lines derived from the human respiratory tract (e.g. A549) or cell lines derived from other animal tissues (e.g. Mardin-Darby Canine Kidney (MDCK)).
Experimental use of ex vivo cultures of human respiratory tissues removed at biopsy or surgical excision was first adopted in 1960’s (Higgins et al., 1969; Roome et al., 1971; Tyrrell and Blamire, 1967; Tyrrell and Hoorn, 1965) as attempts to provide substrates for the isolation and growth of respiratory viruses. Subsequently, for convenience and reproducibility, semi-continuous and transformed continuous cells from various species have been used for the purposes of diagnostic virology. Primary and semi-continuous cell cultures were derived from aborted human embryos (e.g. human embryo lung fibroblast cultures) and from non-human primates (e.g. primary and secondary Rhesus monkey kidney epithelial cell cultures). Continuous transformed cell cultures used for influenza virus studies include MDCK cells and A549 cells derived from a type II pneumocyte lung carcinoma (Giard et al., 1973). These continuous cell lines require the addition of exogenous trypsin for cleavage of the precursor hemagglutinin molecule (HA0) to the HA1 and HA2 components of the mature virion.
While these different cell substrates may be useful for virus isolation, diagnostics and vaccine production, they are not equally useful for providing insights on the tropism of influenza viruses in the human respiratory tract. In this review, we summarize available data on influenza virus receptor distribution, the profiles of virus binding and on virus infection and replication in relevant experimental models and compare these with what is known for the human respiratory tract, to identify physiologically relevant experimental systems for investigating the tropism of influenza viruses for the human respiratory tract.
Distribution of influenza virus receptors
Sialic acids linked to proteins or lipids on the cell surface are binding receptors for influenza viruses. Studies in the 1980s using desialylated and resialylated red blood cells demonstrated that human influenza viruses preferentially bind α2-6 Gal-linked sialic acids while avian influenza viruses preferentially attach to sialic acids with an α2-3 linkage (Rogers and Paulson, 1983). We used the lectin Sambuscus nigra agglutinin (SNA) which binds α2-6 Gal, Maackia amurensis agglutinin (MAA) I which has a preference for α2-3Galβ1-4GlcNAc and MAAII which has a preference to bind α2-3Galβ1-4Gl-3GalNAc to compare the glycan expression in ex vivo cultures of the human nasopharynx, bronchus and lung alveolar tissues with corresponding tissues from patients at autopsy who died of non-respiratory causes (Table 1). These findings are also compared with the glycan expression profiles in undifferentiated and differentiated primary human cell cultures (Chan et al., 2010b; Yu et al., 2011).
Table 1
Summary table comparing the expression of sialic acid receptor of ex vivo organ culture models, in vitro primary epithelial cell cultures and continuous cell lines of human respiratory tract to the nasopharynx, bronchus and lung of humans, using lectin binding method
Lectin | MAAI | MAAII | SNA | ||
---|---|---|---|---|---|
Anatomical site | Culture Model | Differentiation Stage | α2-3 N- linked | α2-3 O- linked | α2-6 |
Nasopharynx | In vivo | ++ | − | ++ | |
Ex vivo | ++ | − | ++ | ||
In vitro | Undifferentiated | ++ | + | − | |
Bronchus | In vivo | ++ | − | ++ | |
Ex vivo | ++ | − | ++ | ||
In vitro | Undifferentiated | ++ | ++ | + | |
Well- differentiated | ++ | − | ++ | ||
Cell line | Calu-3 polarized | O-linked α2-3 ≥ α2-6 (Zeng et al., 2007) | |||
Lung | In vivo | + | ++ | + | |
Ex vivo | + | ++ | + | ||
In vitro | Type-I like | ++ | ++ | + | |
Type-II like | ++ | + | + | ||
Cell line | A549 (Type II-like), NCI-H661 cells | α2-3 << α2-6 (Guo et al., 2009; Sieben et al., 2012) |
Overall, there is good concordance between the expression of the sialic acid receptors in the in vivo tissues and ex vivo organ cultures as well as the well-differentiated primary cell culture models but the receptor distribution in some undifferentiated primary human cell cultures and continuous cell lines do not correspond well with what is found in corresponding human tissues.
In humans, an almost complete differentiation of nasopharyngeal epithelium is found in a 12-13 week fetus in utero with two distinct cellular types, ciliated and microvillus-provided cells (Gulisano et al., 1992). The nasopharyngeal epithelium has five characteristic cellular types found on the surface, including the tall cylindrical ciliated cells; cylindrical cells covered only with microvilli, secretory cells, flat epithelial cells, cubodial cells with microvilli on the surface and many vesicles in the cytoplasm (Karchev and Kabakchiev, 1984). The cell surface of the nasopharyngeal epithelial cells is decorated with glycans with different terminal residues including sialic acids (Chew et al., 1991). Lectin binding studies of ex vivo nasopharyngeal, traceho-bronchial and lung organ cultures accurately reflect the receptor profile observed in normal human tissues (Nicholls et al., 2007a; Nicholls et al., 2007c).
In endemic areas, exposure of humans to H5N1 infected poultry is very common but H5N1 disease remains exceedingly rare. It has been hypothesized those who get H5N1 disease may have differences in the distribution of avian influenza binding Sia α2-3 receptors in the respiratory tract. In a clinical study, the differences of sialic acid receptors distributed in different human tissues, including extrapulmonary organs, in H5N1-infected and non-infected patients was compared using lectin histochemistry (Yao et al., 2008). This study used biotinylated MAAII and SNA lectins to identify the Siaα2-3 and Siaα2-6 receptors present. They found no significant differences in Siaα2-3 and Siaα2-6 receptor distribution between the H5N1 infected and patients dying of non-pulmonary diseases in the trachea, brain, liver, kidney, heart and intestine. Unfortunately, severe distortion of the architecture in the nasal mucosa, pharynx, bronchi, bronchioles and lung parenchyma of H5N1-infected patients precluded comparison of the receptor distribution in these tissues from the control samples. The data from the non-infected human tissues indicated that Siaα2-3 glycans were scarce in the epithelial cells of the upper respiratory tract and in the bronchus. However, it should be noted that only MAAII, which detects the O-linked Sia α2-3 receptor was used. MAAI detects the N-linked Sia α2-3 receptor and would have provided additional relevant information on the distribution of N-linked Sia α2-3 receptors, but was not used in this study.
A number of physiological and pathological factors can affect the expression of sialic acids on the human respiratory tract, e.g. age, inflammation, etc. The upper and lower respiratory tissues of children have a higher abundance of N-linked Siaα2-3 receptors than adults (Nicholls et al., 2007a). Furthermore, inflammatory conditions can affect the expression of sialic acid receptors and therefore the epithelial susceptibility to influenza infection. A study using normal nasal mucosa and nasal polyp tissues explant cultures indicated that nasal polyps expressed higher levels of Siaα2-3 and Siaα2-6, as well as a more efficient influenza virus replication kinetics for both seasonal and H5N1 avian viruses (Suptawiwat et al., 2010). The upregulation of sialytransferease (the enzymes responsible for adding sialic acid to galactose) expression detected in the nasal polyp could be a result of the inflammatory condition leading to the increased abundance of the expression of the corresponding sialic acid receptor. The increased availability of the receptor, together with the change in the inflammatory condition, including the up-regulation of various cytokines and transcriptional factors, e.g. NF-κB, could be one reason for the NF-κB dependence of influenza virus replication (Nimmerjahn et al., 2004).
The extracellular mucus secreted by respiratory epithelial cells (goblet cells and submucosal glands) can also profoundly affect the susceptibility of the respiratory epithelium to influenza viruses. Tests on the effect of mucin on influenza virus infection in hamsters was carried out by Wheeler and Nungester in 1942 (Wheeler and Nungester, 1942). As mucin in the respiratory tract contains an abundance of Sia α2-3Gal (Baum and Paulson, 1990), it may preferentially entrap avian influenza viruses whose HA expresses an affinity for these Sia α2-3 receptors (Couceiro et al., 1993). The activity of the virus neuraminidase in cleaving such linkages is likely to be important in allowing the virus access to the respiratory epithelium. Thorough washing of the epithelial surface to remove such mucus in differentiated respiratory epithelial cultures in vitro does alter its susceptibility to specific viruses (Wong et al., 2012). It is important to note that such removal of mucus does not take place under physiological conditions and thus the presence of mucus in studies of virus tropism may need be taken into account. The pH of the human respiratory epithelium is also likely to affect the permissiveness of the cells to influenza viruses. Neuraminidase activity is enhanced at lower pH. Influenza viruses from different species differ in the optimal pH that triggers HA activation for fusion; human seasonal influenza requiring a lower pH than avian highly pathogenic H5N1. This in turn affects pH stability of different viruses (Krenn et al., 2011).
Binding of labeled influenza viruses to respiratory epithelium
The binding of inactivated labeled influenza viruses to respiratory epithelial tissues provides an alternative approach to investigate virus tropism (Imai et al., 2012; van Riel et al., 2006; van Riel et al., 2007). Formalin fixed paraffin embedded tissues of the human respiratory tract were used in these studies. It is known that sialic acids are not affected by the fixation process, thus the results appear physiologically relevant. H5N1 virus binds to type II cells within the lung while seasonal influenza virus predominantly binds type I cells (van Riel et al., 2007). Supporting this finding, it was recently reported that H5N1 virus infection and budding of new virus particles in ex vivo human lung cultures occurs solely within type II, rather than type I cells (Weinheimer et al., 2012). Studies of the human nasal septum, conchae, nasopharynx, paranasal sinuses and larynx revealed that the highly pathogenic H5N1 virus rarely attaches to the ciliated and goblets cells at these anatomical sites (van Riel et al., 2010).
Binding of whole influenza virus or influenza virus HA on glycan-arrays for determining virus binding preference for sialic acids
Glycan arrays were developed in 2004 (Blixt et al., 2004) and are a powerful tool which allows a more comprehensive analysis of virus binding preference to various glycans. Typically, virus or virus HA is added onto a glass slide coated with hundreds of glycans with different structures, degrees of sialyation and topologies. This allows an assessment of the influenza virus or virus HA binding affinity to a spectrum of glycans to be done in one experiment. Currently, four published glycan arrays are available and used in influenza virus-receptor binding studies with each of them containing a different collections of glycans (Childs et al., 2009; Liao et al., 2010; Stevens et al., 2006a), ranging from few dozens up to more than six hundred found in the array that is developed and updated by the Consortium for Functional Glycomics (http://www.functionalglycomics.org/). Using such technology, it has been possible to define the effect of a few amino acid changes in the HA which change the binding affinity of a H5 virus from a Sia α2-3 to a dual Sia α2-3 and Sia α2-6 binding profile HA (Stevens et al., 2008; Stevens et al., 2006b). However, until recently, the glycans that are present in the human respiratory tract were not well defined, and thus it was not clear which of the glycans found in these arrays were physiologically relevant for humans and also whether all the glycans found on the respiratory epithelium were represented in the available arrays. Recent studies have used mass-spectroscopy to comprehensively characterize the glycans present in the human respiratory tract. These studies reveal that of approximately 50 glycans present in the human respiratory tract, around one third are not represented in any of the glycan arrays currently available (Trevenan et al., 2013). Therefore, the conclusions based on glycan array studies on virus receptor-binding profiles so far should be treated with some cautions and the arrays need to be updated to include those glycans physiologically relevant to the human respiratory tract.
Infection and replication of influenza
Human ex vivo cultures
As expected from studies of influenza virus receptor distribution in human respiratory tract tissues, seasonal human influenza viruses as well as the pandemic H1N1 (2009) viruses efficiently infect and replicate in ex vivo cultures of the nasopharynx and upper airways. Influenza A H5N1 viruses can also infect and replicate in ex vivo cultures of the nasopharynx (Nicholls et al., 2007c) including the tonsils and adenoid although they apparently lack Sia α2-3 receptors, and also in the alveolar epithelia (Figure 2B) and alveolar macrophages in lung (Nicholls et al., 2007c; Shinya et al., 2006; van Riel et al., 2006). As expected, there is little H5N1 infection of the conducting airways, including the epithelia of bronchi (Figure 2C) and bronchioles. It is also noted that H5N1 viruses appear to replicate to high titer in the upper respiratory tract of ferrets but without transmission (Imai et al., 2012). The virus has also been reported to infect the ferret brain via spread along the olfactory nerves supplying the cribriform plate which implies competence of this virus to replicate in the ferret nasopahrynx, which also apparently lacks the avian Sia α2-3 receptors as detected by lectin binding. The ex vivo cultures thus highlight some discordance with accepted dogma which is an area that deserves further research.
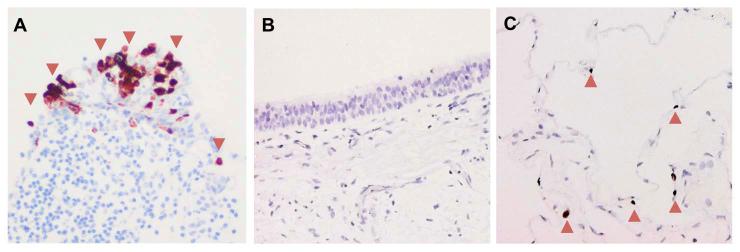
H5N1 virus infected ex vivo cultures of human A) nasopharyngeal, B) bronchial and C) alveolar epithelium with red arrows indicating the infected cells stained positive with influenza viral antigen in reddish brown.
Although swine influenza viruses have a predilection for human-like Sia α2-6 receptors, interestingly, not all swine influenza viruses replicate in human ex vivo cultures of nasopharynx and bronchi (Chan et al., 2011b). Replication in human ex vivo cultures of nasopharynx and bronchi appears to identify swine influenza viruses that have the capacity to transmit by airborne droplets in the ferret model, and therefore those that pose a greater threat to transmit in humans (Yen et al., 2011). This suggests that replication of influenza viruses in human ex vivo cultures of the upper airways can be one factor used in risk-assessing animal viruses for pandemic threat.
Primary human respiratory epithelial cell cultures in vitro
While ex vivo cultures are superior in mimicking the physiological microenvironment of the respiratory tract, the complexity of the organ culture system makes it difficult to evaluate the role of individual cell types and these cultures can be maintained with good viability for a limited time (ranging from 4 to 10 days) (Booth et al., 2004; McBride et al., 2000). More importantly, the availability of human ex vivo organ cultures are limited and donor-to-donor variation cannot be well controlled. Therefore, in vitro cultures based on primary cells isolated from its original anatomical site, with or without differentiation in air-liquid interfaces in vitro, provide an important alternative and that can provide physiological relevance (as compared with immortalized transformed cell lines) and greater availability and reproducibility for more detailed experimentation. Available data on influenza virus tropism and infection in primary human respiratory epithelial cultures is summarized below.
Primary human nasal and nasopharyngeal epithelial cultures in vitro
Because of its anatomical location in the upper airways, nasal and nasopharyngeal epithelial cells are usually those first exposed to a respiratory pathogen such as influenza virus. Therefore, data generated from these cells are important in understanding the tropism and pathogenesis of influenza viruses in humans. Matrosovich and colleagues have developed well-differentiated nasal epithelial cell cultures in vitro, but have not systematically documented influenza virus tropism in these cells (Matrosovich et al., 2004). Highly pathogenic influenza H5N1 viruses and seasonal H1N1 virus replicated efficiently in undifferentiated nasopharyngeal epithelial cell in vitro cultures at both 33°C and 37°C (Nicholls et al., 2007b), correlating with observations in ex vivo cultures of the human nasopharynx. However, the use of undifferentiated nasopharyngeal cells needs to be treated with caution as the Sia receptor distribution of undifferentiated nasopharyngeal epithelial cells do not closely mimic that seen in the human nasopharynx or in ex vivo nasopharyngeal cultures. The undifferentiated nasopharyngeal cells express both O-linked and N-linked α2-3 and Sia α2-6 receptors whereas human nasopharyngeal epithelium and ex vivo nasopharyngeal culture only express N-linked Sia α2-3 and Sia α2-6 receptors as determined by lectin binding studies.
Primary human differentiated and undifferentiated tracheo-bronchial epithelial cultures in vitro
Tracheobronchial epithelial (TBE) cells or the normal bronchial epithelial (NHBE) cells are cells derived from the conducting airways. These primary cells have been used extensively to study respiratory disease, including influenza virus infections, because in vitro cultures can be differentiated in air-liquid interface cultures to generate epithelia that contain ciliated epithelial cells and mucus secreting goblet cells together with the secretion of human airway trypsin like proteases that mimic the conditions found in the human conducting airways, For example, as within human airways, influenza viruses replicate in these cultures without the need for adding exogenous trypsin to these cultures for the cleavage of the HA0 to HA1 and HA2. A comparison of the virus infection and replication kinetics as well as innate immune responses of human seasonal influenza H1N1 and avian H5N1 viruses in undifferentiated and well differentiated NHBE cells has been reported (Chan et al., 2010b). It was found that both differentiated and undifferentiated NHBE cells were permissive to H5N1 virus infection but productive H5N1 replication was observed only in non-differentiated NHBE. While H1N1 can replicate efficiently both differentiated and undifferentiated in vitro cultures, the well-differentiated NHBE cells correlate better with the observations from ex vivo bronchial organ cultures. These findings also correspond to the observation that while O-linked Sia α2-3 receptors may not be as prominent as N-linked Sia α2-3 receptors in human bronchial tissues, ex vivo cultures and well differentiated NHBE cells, they are well expressed in the undifferentiated NHBE cells, explaining the (non-physiological) permissiveness of these cells to the avian H5N1 virus which may dependent on binding to Sia α2-3 receptors (Chan et al., 2011c; Nicholls et al., 2007a)
In studies with well-differentiated tracheobronchial epithelial cell cultures reported by Matrosovich’s group, H5N1 virus preferentially infects ciliated respiratory epithelial cells rather than non-ciliated cells and this was thought to be related to the binding preference of the virus HA protein to the 2-3-linked sialic acids, which was mainly expressed on ciliated cells (Matrosovich et al., 2004). In the studies reported by others (Chan et al., 2009; Ibricevic et al., 2006), differentiated epithelial cells express functional Sia α2-6 in both ciliated and goblet cells. In a more recent study using a low pathogenic H5N1 which primarily bound sia α2-3 moieties, removal of Sia α2-3 moieties in fully differentiated NHBE cells by specific sialidase failed to abrogate infection (Oshansky et al., 2011). These authors concluded that, at least with the low pathogenic H5N1 viruses, infection is not constrained by the need for sialic acid α2-3 residues. These findings highlight the need a better evaluation of the binding profiles of these viruses as well as a better understanding of the virus receptors on respiratory cells that are needed for virus replication. It is also important to note that virus attachment, though necessary, is not always predictive for efficient infection of the cells (Rimmelzwaan et al., 2007).
Ilyushina et al in 2008 attempted to evaluate the viral load at one-day post infection of 31 recombinant viruses with the A/Vietnam/1203/04 (H5N1) backbone. These viruses possessed mutations of the HA which altered binding affinity or specificity for Sia receptors. Comparison of the efficiency of infection and virus replication kinetics of these viruses would have potentially provided useful information on the contribution of these mutations on human bronchial epithelial cell tropism. However, as the purpose of their study was to evaluate the effect of changes in HA that affect neuraminidase-inhibitor susceptibility of H5N1 viruses, only the fold-change of viral load with or without the neuraminidase-inhibitor treatments was shown. Thus we do not have the information needed to assess the effect of these H5-HA protein mutations on respiratory epithelial tropism, except for the conclusion that “human-like” HA specificity does not affect the neuraminidase-inhibitor susceptibility of the virus (Ilyushina et al., 2008). In a follow up study, they showed that mutant H5N1 viruses with decreased NA activity are better adaptated to human airway epithelium (Ilyushina et al., 2012). This study also highlighted the possibility of using serial passage of virus in differentiated NHBE cells as a model to study the virus mutations relevant to the process of human adaptation. Likewise, influenza virus tropism in NHBE is being used as a model to assess whether a virus has potential to cause human infection (Suksatu et al., 2009). In this study, the two of the three H5N1 viruses isolated from cloacal swabs of open-bill storks replicated and caused cytopathic effect in NHBE cells. It has thus been suggested that open-bill stork-derived H5N1 viruses may not require additional mutations to cross the species barrier to infect humans.
Primary human alveolar epithelial cell cultures in vitro
The tropism of viruses for type I and type II alveolar epithelial cells is important for understanding virus pathogenesis in the lung. Although type I pneumocytes are numerically less than 50% of the total number of type II pneumocytes in the lung, they contribute to 90% of the alveolar surface area because of their flattened morphology. The type I pneumocyte is thin and flattened to allow gaseous exchange to take place and does not undergo further cell division. Type II pneumocytes are more cuboidal and account for less than 10 % of the alveolar surface area (Crapo et al., 1982). They act as the defenders and regenerators of the alveoli rather than contributing to gaseous exchange. They are responsible for the production of surfactant proteins (Crouch et al., 2000) which functions to lower the surface tension between air and alveolar fluid and thus to prevent alveolar collapse at end of the expiration. In addition, surfactant proteins contribute to antiviral defense (Hillaire et al., 2012). Pulmonary surfactant consists of lipids (90–95%) and four surfactant proteins (SP-A, SP-B, SP-C, and SP-D, 5–10%). Type II alveolar pneumocytes are progenitor cells which can proliferate and differentiate into type I pneumocytes following lung injury (Adamson and Bowden, 1974). They also play an important role in controlling the lung fluid transport between the apical and basolateral surface by means of the sodium channel to maintain the normal function of the lung (Mason, 2006).
The alveolar epithelium is the main site for the replication of highly pathogenic avian H5N1 viruses as shown by the demonstration of viral antigens in alveolar epithelial cells as well as in alveolar macrophages in autopsy lungs (Liem et al., 2008). Although viral antigen could be found in other extrapulmonary organs, major pathological changes, high viral load and frequent virus isolation are most reproducibly seen in the respiratory tract and these patients die primarily of their lung pathology and acute respiratory distress syndrome (Abdel-Ghafar et al., 2008; Suptawiwat et al., 2010; Uiprasertkul et al., 2005; Zhang et al., 2009).
There are only a few studies on influenza virus tropism in type I and II pneumocytes because the maintenance of type II cells on plastic surfaces in vitro is very difficult as they differentiate rapidly into a type I-like phenotype (Daum et al., 2012; Fang et al., 2006; Wang et al., 2007; Yu et al., 2011). The replication kinetics of human seasonal H1N1 and avian highly pathogenic H5N1 viruses were compared in primary human alveolar epithelial cells (Chan et al., 2005), demonstrating that both viruses infected these cells with similar efficiency (93-100% following infections with multiplicity of infection = 2) as evaluated by nucleoprotein staining and produced similar viral yield at 48hpi. However, given the rapid differentiation of type II pneumocytes to type I cells in vitro, the exact phenotype (type I vs type II) of these alveolar epithelial cells could not be defined with certainty. Later studies investigated virus infection and productive replication of influenza viruses in polarized type I-like epithelial cells in vitro. These studies showed that both seasonal H1N1 and highly pathogenic H5N1 virus can efficiently infect type I cells from their apical and basolateral surfaces, but release of the virus is restricted to the apical side of the cells (Chan et al., 2009). In a comparison of H5N1 virus infection and one-step growth kinetics in cultures of differentiated primary human alveolar type I-like cells and alveolar type II cells grown on matrix gel, it was observed that influenza viruses have a higher efficiency of infection in type-I like cells (> 95%) than in type-II cells (26% ± 4%). The replication kinetics of H5N1 virus peaked earlier at 48 hpi with a 2.5 log increase in viral titer in type I pneumocytes compared to type II-like cells where replication peaks at 72 hpi but with a higher (4.5 log) increase in viral titres (Yu et al., 2011). This highlights the biological differences of type I-like cells and type II cells for both H5N1 and seasonal H1N1 influenza virus replication.
Continuous transformed cell lines
Continuous cell lines derived from the human respiratory tract have been used in some studies of influenza virus tropism and pathogenesis. However, continuous cell lines are often not representative of respiratory cells found in vivo. A549 cells were derived from a type II pneumocyte lung tumor (Giard et al., 1973) and are commonly used to represent normal human lung epithelium in studies of virus tropism and host responses. Although A549 cells express some characteristics of type II pneumocyte, including the synthesis of phospholipids, cytoplasmic lamellar bodies and apical microvilli, they do not mimic the true phenotype of its primary counterpart. For example they lack the expression of surfactant proteins A, B, Pro-surfactant C and furthermore, the produced surfactant C is expressed as a mutated form. More importantly, while low pathogenic influenza viruses can replicate in primary human pneumocytes without the addition of exogenous trypsin because these cells produce endogenous human airway proteases, exogenous trypsin must be added to support multiple rounds of virus replication in A549 cells (Das and Boggaram, 2007; Swain et al., 2008). When the sialic acid distribution in A549 cells is compared with those in primary type II-like pneumocyte (Table 1), A549 cells have far more Sia α2-6 than α2-3, which is the reverse of what is observed in type II pneumocytes in vivo and in vitro. Thus, A549 cells are not ideal surrogate for the study of influenza viral cell tropism.
Calu-3 or BEAS-2B cells are alternative continuous cell lines that have been used for studies of influenza viruses and compared with primary human bronchial epithelial cells (Stewart et al., 2012). Calu-3 cells are derived from a human lung adenocarcinoma and give reproducible transepithelial electrical resistance on polarization. However, ciliated cells are comparatively less abundant (or absent) when compared to normal bronchial epithelium. Furthermore, the high abundance of O-linked Sia α2-3 (Zeng et al., 2007) which is detected by MAAII binding, differs from the human bronchus where MAAII binding is absent. BEAS-2B cells are another continuous human bronchial epithelial cell line obtained from non-cancerous individuals that was transformed using adenovirus. However, these cells cannot differentiate into ciliated or goblet cells and cannot develop to form tight junctions. Moreover, there is so far no report on the sialic acid distribution in this cell line. It is less commonly used than Calu-3 for studies on influenza.
H5N1 tropism in non-epithelial cell types found in the respiratory tract
In addition to alveolar epithelial cells, the lung microenvironment also contains resident immune cells, including alveolar macrophages, dendritic cells, T cells, NK cells and lung stem cells. Some of these cell types have been found to stain positive for influenza virus antigen by immune-histochemistry in fatal cases of human H5N1 disease (Uiprasertkul et al., 2005). It is therefore relevant to investigate these cells with regard to their susceptibility and response towards H5N1 infection. Data from in vitro cultures of primary human cells are summarized below.
Alveolar Macrophage and peripheral blood monocyte derived macrophage
Extensive infiltration of macrophages into the alveolar space is reported in H5N1 disease (Peiris et al., 2004). Alveolar macrophages are consistently found to be positive for virus antigen in autopsy tissues of H5N1 infected patients (Gu et al., 2007). In ex vivo lung cultures, alveolar macrophages are positively stained with viral antigen after H5N1 virus infection ex vivo (Nicholls et al., 2007c; Weinheimer et al., 2012). Viral antigen within the macrophages in the lung could reflect productive or abortive infection, or may represent phagocytosis of other virus-infected cells by macrophages. Therefore it is important to examine the H5N1 virus tropism in this cell type.
In a comparison of the susceptibility of human alveolar macrophages and peripheral blood monocyte derived macrophage to H5N1 virus infection (at a multiplicity of infection of 2), over 95% of cells were infected in both cell types, as demonstrated by expression of viral protein at 16 hours post infection. Productive virus replication was seen in alveolar macrophages (2 log increase in viral yield) and in peripheral blood derived macrophages (1 log increase in viral yield). Seasonal H1N1 influenza virus led to a similar efficiency of infection (as demonstrated by virus antigen expression) in both cell types and the replication kinetics was comparable to that of H5N1 virus. However, H1N1 virus failed to replicate productively in alveolar macrophages (Yu et al., 2011). Others also found differences between alveolar and peripheral blood derived macrophages and reported that H5N1 virus replicates less efficiently in alveolar, as compared with peripheral blood monocyte derived macrophages (van Riel et al., 2011).
Endothelial cell tropism, host responses and the importance of endothelial integrity
The loss of endothelial barrier function and the development of microvascular leakage has been found to be a key determinant in the pathogenesis of bacterial sepsis. A recent study demonstrated that the endothelial barrier breakdown may occur in other viral infections such as H5N1 avian influenza virus (Steinberg et al., 2012). In the human lung microenvironment, the microvascular endothelial cells are essentially an immense capillary plexus. The capillary endothelium is composed of the flattened cytoplasmic extension of the endothelial cells, which by their contiguous arrangement forms a thin vascular tube. The cytoplasm of endothelial cells, like the squamous epithelial cells lining alveoli, rarely contain organelles. The microvascular capillaries run through the lung and are overlain by the thin layer of alveolar type I epithelial cells. In addition to its important but passive role in gas and liquid exchange, the capillary endothelium is also a major site of liquid and solute exchange in the lungs which is essential for the vital function of the gas exchanges.
Infectious H5N1 virus can be found in the blood and cerebrospinal fluid in birds (Muramoto et al., 2006) and several mammalian species and there is evidence of disseminated infection with a fatal outcome in some humans (Buchy et al., 2007a; de Jong et al., 2005; de Jong et al., 2006; Gu et al., 2007; Uiprasertkul et al., 2007). These observations suggest that the spectrum of H5N1 influenza A virus is wider than previously thought (de Jong et al., 2005; de Jong et al., 2006; Gu et al., 2007). However, the mechanism of dissemination of the H5N1 influenza virus is still unclear. Although autopsy data in influenza H5N1 virus infected patients is limited, diffuse intravascular coagulation (Claas et al., 1998; Shu et al., 2006; Wiwanitkit, 2008), diffuse alveolar damage with hyaline membrane formation, patchy interstitial lymphoplasmacytic infiltrates, bronchiolitis with squamous metaplasia and pulmonary congestion with varying degrees of hemorrhage have been identified (Buchy et al., 2007a; Gu et al., 2007; Uiprasertkul et al., 2007; Zhou et al., 2006a). The microvascular endothelium has a critical role in determining the permeability of the alveolar capillary barrier and hence controlling the influx of protein-rich oedema fluid into the airspaces which occurs in acute lung injury. Inflammatory mediators are thought to play a critical role in the pathogenesis of this disorder (Minamino and Komuro, 2006).
Influenza virus antigen has been detected in lung endothelial cells of patients with pandemic H1N1 2009 virus infection (Shieh et al., 2010). Although viral antigen has not so far been detected in endothelial cells in patients with fatal H5N1 disease, given evidence of extra-pulmonary dissemination, it is likely that virus does infect lung microvascular endothelium.
The distribution of sialic acid receptors on endothelial cell of human tissues has been studied using staining with lectins, MAA II and SNA. Endothelial cells of all human organs express both avian influenza (Sia α2-3) and human influenza (Sia α2-6) receptors (Yao et al., 2008). It is therefore plausible that H5N1 virus may infect endothelial cells and that this may facilitate the dissemination of highly pathogenic avian H5N1 viruses to multiple organs (Gu et al., 2007). Although receptors for human adapted seasonal influenza viruses are also ubiquitous in the endothelial cells, the lack of a multi-basic cleavage site of the hemagglutinin in these viruses (in contrast to highly pathogenic avian viruses) restricts their capacity for replication in sites beyond the respiratory and gastrointestinal tracts because these other organs lack the human-airway-like proteases needed for the cleavage and activation of these hemagglutinin molecules. The presence of avian influenza receptor is thus not the only factor determining the capability of avian influenza A virus to infect cells.
In a study of polarized microvascular endothelial cells infected in vitro, H5N1 but not seasonal H1N1 virus efficiently infected these cells (Chan et al., 2009; Ocana-Macchi et al., 2009). H5N1 virus infected these cells from both an apical and basolateral side of the cell and new progeny virus was also released from both the apical and basolateral aspect of the cells. This provides a mechanistic explanation for how H5N1 may disseminate beyond the respiratory tract and also how virus may invade the lung from the systemic circulation, for example after infection via the gastro-intestinal route. There is anecdotal evidence of humans being infected with H5N1 virus though consumption of infected uncooked food. There is also evidence that inoculation of ferrets with H5N1 virus via the intragastric route can lead to virus infection that disseminates to the lung and other organs inoculation of ferrets (Shinya et al., 2011). Others have suggested that the higher expression of the Sia α2-3-linked receptors on pulmonary microvascular endothelial cells could explain the higher permissiveness of these cells to H5N1 infection and they found that the deletion of the H5 hemagglutinin multibasic cleavage site does not affect virus infectivity but results in decreased virus replication in endothelial cells (Zeng et al., 2012).
Neutrophils
During acute influenza virus infection, the infected alveolar epithelium secretes inflammatory mediators that leads to the recruitment of inflammatory cells including neutrophils and macrophages to the lung (Wareing et al., 2007). To date, there is little data on the significance of neutrophils in human influenza because neutrophil infiltration occurs during the early phase of inflammation (Tate et al., 2009; White et al., 2007) at a time when clinical biopsy or autopsy material is not readily available. One autopsy study of H5N1 disease in a pregnant woman found evidence of viral antigen in neutrophils in the placental blood and hypothesized that neutrophils may be a vehicle for virus dissemination (Zhao et al., 2008). Bring short-lived cells, there have not been studies so far to systematically investigate whether neutrophils can get infected by H5N1 virus in vitro.
We infected human neutrophils with seasonal H1N1 or highly pathogenic H5N1 virus in vitro. In six independent experiments using neutrophils from five different donors, we found a significant increase in influenza A matrix gene viral load in H5N1 infected cells whereas that for seasonal H1N1 virus was marginal (Figure 1) (Chan et al – unpublished data). These findings suggest that H5N1 virus infects and replicates in human neutrophils. The findings also indicate a difference between H5N1 and seasonal H1N1 virus in this regard.
Dendritic cell and lymphocytes
Dendritic cells are professional antigen presenting cells which acquire virus antigen at the site of infection. This is followed by maturation of these cells with the expression of co-receptors and their migration to the draining lymph nodes. This may potentially also provide a route for dissemination of H5N1 infection. H5N1 virus can infect and replicate in monocyte-derived and blood myeloid dendritic cells whereas blood plasmacytoid dendritic cells are resistant to infection (Thitithanyanont et al., 2007). DC-SIGN on the surface of dendritic cells was responsible for the capture and transfer of H5N1 pseudotyped and recombinant particles to target cells (Thitithanyanont et al., 2007).
There has so far not been systematic study reporting on experimental infection of lymphocytes with H5N1 virus in vitro although some have reported H5N1 virus antigen in autopsy tissues (Zhang et al., 2009).
H5N1 infection of extrapulmonary cells
Astrocytes
Neuronal cells and glial cells of the brain have been found to express H5N1 virus antigen in autopsy tissues of H5N1 cases (Gu et al., 2007) (Zhang et al 2009). Differentiated human astrocytes and neuronal cell can be infected with both seasonal H1N1 and H5N1 virus in vitro but only astrocytes supported productive replication (Ng et al., 2010).
Placenta and fetus
H5N1 viral antigen was detected in the Hofbauer cells and cytotrophoblasts of the placenta from a pregnant woman who died from H5N1 infection. In the fetus, viral RNA and antigen was found in the lungs, circulating mononuclear cells, and macrophages of the liver. This appears to be the first evidence of transplacental transmission of H5N1 from mother to fetus (Gu et al., 2007).
Digestive tract
The digestive tract has been suspected to be a potential site for replication of H5N1 virus, given the high H5N1 viral load in rectal swabs (Buchy et al., 2007b) and the isolation of virus from faeces in a patient with H5N1 encephalitis (de Jong et al., 2005). However, detection of virus in the gastrointestinal tract may reflect swallowed respiratory secretions containing virus and is not conclusive. H5N1 virus antigen has been detected in the small and large intestinal epithelial cells at autopsy (Zhang et al., 2009). Siaα2-6-Gal was absent from epithelial surface of small and large intestines and was found only in lamina propria cells. In contrast, Siaα2,3-Gal was found on large intestinal epithelial cells and in lamina propria cells. Virus-binding studies showed that neither seasonal influenza A (H1N1) nor A (H3N2) virus bound to small and large intestinal epithelial surface, but they did bind to a subset of CD45+ leukocytes (Chan et al., 2011a)
Conjunctival epithelium
The conjunctival sac is connected to the nasopharynx via the lacrimal duct. The human conjunctival epithelium contains Sia α2-3 sialic acid receptors but not Sia α2-6 receptors. Ex vivo cultures of conjunctival epithelium culture also possess similar sialic acid expression profiles (Chan et al., 2010a; Olofsson et al., 2005; Terraciano et al., 1999). Therefore, it is considered as an alternative entry route for avian influenza virus infection (Olofsson et al., 2005). Human seasonal H1N1 (pre-2009) and H3N2 influenza viruses fail to infect or replicate in ex vivo cultures of the conjunctival epithelium while avian H5N1 and H7N7 viruses do. Conjunctivitis has been repeatedly associated with human H7N7 infections in the Netherlands and elsewhere (Fouchier et al., 2004). H5N1 and H7N7 viruses can infect and replicate well in ex vivo conjunctival cultures but seasonal H1N1 and H3N2 viruses do not efficiently infect the conjunctiva. Surprisingly, pandemic H1N1 virus can efficiently infect and replicate in the conjunctival ex vivo cultures (Chan et al., 2010a), given its receptor binding profile being similar to that of seasonal influenza viruses (Maines et al., 2009) although one study using glycan array has reported dual binding of the pandemic H1N1 virus to both Sia α2-3 and Sia α2-6 receptors (Childs et al., 2009). The conjunctival tropism of pandemic H1N1 virus was further supported by a recent clinical report of bilateral acute conjunctivitis in patients with pandemic H1N1 infection (Mansour et al., 2012).
As with conjunctival epithelium, H5N1 but not seasonal influenza strains replicated to high titers in human retinal pigment epithelial cells (Michaelis et al., 2009). Ferret infection studies have shown that ocular inoculation of H5N1 virus can lead to lethal infection with systemic spread without involvement of the lower respiratory tract (Belser et al., 2012).
Investigation of Host Responses using primary human cells
Primary human cells and ex vivo cultures also provide insights into host responses to virus infection. The mechanisms underlying the severity of human disease, primarily as a result of a fulminant primary viral pneumonia caused by H5N1 virus remain unclear. Although early use of antivirals have a significant impact on reducing mortality associated with human disease, even oseltamivir treatment within 2 days of symptom onset is still associated with a case fatality ratio of >15% (Chan et al., 2012), a worrying scenario if H5N1 viruses with current virulence characteristics were ever to become pandemic. While the replication competence of the virus, lack of protective host immune responses and the tropism for the alveolar epithelium contribute to this unusually severe lung pathology, there is accumulating evidence that dysregulated host responses also contribute to the pathogenesis of this disease (Peiris et al., 2010). Data from studies of patients with H5N1 disease and experimental animal models are compatible with this hypothesis (Baskin et al., 2009; de Jong et al., 2006; Perrone et al., 2008). However, studies of H5N1 or seasonal influenza infected humans or animals in vivo does not allow differentiation of the contribution of increased viral replication efficiency from intrinsic differences between these viruses in eliciting aberrant host responses. By comparing host responses elicited by H5N1 and other seasonal or pandemic viruses in experiments using primary human cells in vitro, where the dose of infecting virus can be controlled, the intrinsic differences between these viruses can be investigated.
Apoptosis and cell death
Avian influenza H5N1 virus was shown to induce apoptosis in many cell types including macrophages and dendritic cells. In human monocyte-derived macrophages, TNF-related apoptosis-inducing ligand (TRAIL) was shown to be involved in the apoptosis-associated mechanisms in H5N1 virus infection through the activation of caspase-10. H5N1 virus triggered apoptosis, including the activation of caspases and PARP, instead of necrosis (Ekchariyawat et al., 2012; Zhou et al., 2006b). Viruses differed in the speed with which they led to apoptosis (Geiler et al., 2011; Mok et al., 2007). It is notable that apoptosis, by reducing the opportunity for productive virus replication and release of inflammatory mediators from the infected cells, can be a host defense mechanism in response to virus infection. iNOS has been found to be involved in the induction of target cell apoptosis. Kruppel-like factor 6 (KLF6) is a critical transcription factor essential for iNOS gene expression following influenza virus infection. KLF6 gene silencing in human lung epithelial cells resulted in the loss of NO production, iNOS promoter-specific luciferase activity and the expression of iNOS. A significant reduction in influenza virus-mediated apoptosis was observed in KLF6-silenced cells, cells treated with iNOS inhibitor, and primary murine macrophages derived from iNOS knockout mice (Mgbemena et al., 2012).
Influenza H5N1 virus, but not seasonal H1N1 virus, has been shown to be involved in autophagic cell death, an alternative programmed cell death pathway in alveolar epithelial cells upon infection by the H5N1 virus (Sun et al., 2012). In addition, autophagy has been demonstrated to play a role in influenza induced cytokine induction. H9N2 influenza A viruses of the G1-lineage (which possess 6 internal gene segments in common with the H5N1 viruses that caused the outbreak in Hong Kong in 1997) was a more potent inducer of autophagy, in association with cytokine (CXCL10 and interferon-alpha) hyper-induction when compared with seasonal H1N1 and pandemic influenza H1N1 viruses in human blood macrophages (Law et al., 2010).
Innate immune responses
A major difference between the low pathogenic seasonal influenza and lethal influenza viruses such as H5N1 or the 1918 H1N1 virus is an increased release of inflammatory cytokines and chemokines that occurs in H5N1 or 1918 H1N1 virus infected macrophages or epithelial cells (Chan et al., 2005; Cheung et al., 2002; Kobasa et al., 2007; Perrone et al., 2008; Tumpey et al., 2000; Wong and Yuen, 2006; Yu et al., 2011). Comparative transcriptomic analysis of H5N1 and seasonal H1N1 infected human macrophages showed that both viruses elicit similar host response pathways but that H5N1 virus was a more potent inducer of these pathways including those of interferon induction and signaling, of tumor-necrosis factor alpha induction and signaling, and differentially activated apoptosis pathways (Lee et al., 2009). H5N1 virus strains may differ in their potency in activating innate immune responses (Sakabe et al., 2011). The host innate immune responses may also be affected by the differentiation status of the respiratory epithelial (NHBE) cells, in part because of the reduced permissiveness of these cells to avian H5N1 virus infection (Chan et al., 2009). Similarly, alveolar macrophages express much less pro-inflammatory cytokines in response to H5N1 virus infection compared to peripheral blood monocyte derived macrophages (van Riel et al., 2011; Yu et al., 2011). Since there is a massive increase of macrophages in the lung of H5N1 virus infected patients, it is important to understand whether such infiltrating macrophages have a cytokine phenotype more akin to alveolar macrophages or to peripheral blood monocyte derived macrophages. These provide good illustrations of the need to choose physiologically relevant cell subsets for such investigations.
Primary human peripheral blood monocyte derived macrophages have been used to study the signaling mechanisms associated with H5N1-mediated cytokine hyper-induction including the role of RIG-I, IFN regulatory factor 3 (IRF3), NF-κB nuclear translocation and p38 kinase activation in the induction of IFN-beta, IFN-lambda1 and MCP-1, and their partial contribution to the induction of TNF-alpha (Hui et al., 2011; Hui et al., 2009). Human peripheral blood monocyte derived macrophage infected with influenza H3N2 or H5N1, but not with H1N1 viruses was associated with pronounced pro-inflammatory cytokine production and activation of relevant mitogen-activated protein kinase pathways as indicated by phosphorylation of p38, JNK and ERK 1/2 (Geiler et al., 2011). Soluble mediators secreted from influenza H5N1-infected human monocyte derived macrophages up-regulated RIG-I, MDA5, and TLR3 in adjacent un-infected cells thereby potentially sensitizing these cells by paracrine effects acting via IFNAR1/JAK but not IFN-λ receptor signaling (Hui et al., 2011). When compared with virus free supernatants of H1N1 virus infected cells, H5N1 virus infected cell supernatants were more potent at inducing these effects in adjacent cells and in enhancing the cytokine responses to PolyIC and to both the seasonal H1N1 and H5N1 virus infection in a RIG-I-dependent manner. Thus, sensitizing neighboring cells by up-regulation of RIG-I contributed to the amplified cytokine cascades during H5N1 infection (Hui et al., 2011; Lee et al., 2008). Some of these paracrine mediator cascades were dependent on COX-2 activation (Lee et al., 2008). In dendritic cells and macrophages, influenza virus RNA interacting with TLR7 provides the first signal and the virus M2 ion channel protein provides the second signal for inflammasome activation by influenza viruses (Ichinohe et al., 2010).
The proteomic responses in the cytoplasm of H5N1 or H1N1 virus infected macrophages was evaluated using mass spectrometry-based quantitative proteomic profiling and showed significant perturbation of the host proteome upon viral infection as early as one hour after infection. This early host response distinguished H5N1 infection from H1N1 infection, the latter inducing less of a response. The most pronounced effect was observed on the translational machinery, suggesting that H5N1 might gain advantage in replication by using the cell protein synthesis machinery early in the infection (Cheung et al., 2012).
Interferon (IFN) is the first line of defense against influenza virus infections. Influenza viruses have developed various mechanisms to counteract this response, in order to gain a replication advantage in the host cells. The non-structural protein 1 (NS1) protein of influenza contributes to evading the host interferon responses (Geiss et al., 2002). H5N1 virus was showed to block the IFN signaling by reducing the IFN-inducible tyrosine phosphorylation of STAT1, STAT2 and STAT3 and inhibit the nuclear translocation of phospho-STAT2 and the formation of IFN-inducible STAT1:1-, STAT1:3- and STAT3:3- DNA complexes. Besides, NS1 induced the up-regulation of the JAK/STAT inhibitors, SOCS1 and SOCS3, suggested that NS1 can directly interfere with IFN signaling to enhance viral replication (Jia et al., 2010). Influenza A viruses not only suppress IFN-beta gene induction but also inhibit type I IFN signaling through a mechanism involving induction of the suppressor of cytokine signaling-3 (SOCS-3) protein (Pauli et al., 2008).
Recombinant viruses generated by reverse genetic methods that contain different combinations of genes from high-cytokine (A/Vietnam/1203/04; H5N1) and low-cytokine (A/WSN/33; H1N1) phenotype viruses suggested that the H5N1 polymerase gene segments, and to a lesser extent the NS gene segment, contribute to cytokine hyper-induction phenotype in primary human macrophages. Thus, a putative H5 pandemic virus that may arise through genetic reassortment between H5N1 and one of the current seasonal influenza viruses may have a markedly altered cytokine phenotype (Mok et al., 2009).
Many studies have been carried out to determine the functional contribution of inflammatory mediators released from the respiratory epithelium and macrophages to inflammation (summarized above). Consequences of the infection of endothelial cells have been less intensively studied. The most frequently used endothelial cell model in influenza A virus research is the umbilical vein endothelial cell (Ishiguro et al., 2004; Sumikoshi et al., 2008; Taub et al., 1993; Visseren et al., 1999). It is known to produce IL-6 (Ishiguro et al., 2004; Visseren et al., 1999), IP-10 and MIG (Ishiguro et al., 2004) in response to influenza A virus infection. There have been varying reports on the permissiveness of endothelial cells to influenza viruses (Colden-Stanfield et al., 1993; Friedman et al., 1981; Ishiguro et al., 2004; Sumikoshi et al., 2008; Visseren et al., 2000). The response of endothelial cell, in particular, the lung microvascular endothelial cells, to the highly pathogenic H5N1 influenza A virus subtypes remains unknown.
There is increasing evidence of heterogeneity (Craig et al., 1998) among endothelial cells from different tissues from in vivo (Swerlick et al., 1992) and in vitro (Haraldsen et al., 1996; Nilsen et al., 1998). Endothelial cells from different sites may respond differently to inflammatory stimuli and have variable susceptibility to injury (Murphy et al., 1998). Data extrapolated from non-pulmonary or macro-vascular endothelium (Beekhuizen and van Furth, 1994), e.g. human umbilical vein endothelial cells (HUVEC), might not adequately represent cells from the lung. It is therefore important to use appropriate endothelial cell models that exhibits characteristics with direct relevance to respiratory diseases processes.
Conclusion
Primary human cells, differentiated cultures of respiratory epithelium and ex vivo cultures contribute to understanding influenza virus tropism for the human respiratory tract and the host responses to influenza virus infection. Taken together with data from clinical studies on infected humans and relevant animal models, these data can provide insights into virus transmission and pathogenesis and may provide understanding that leads to novel therapeutic interventions.
Table 2
Attachment of labeled influenza virions to human respiratory epithelia for comparison with distribution of influenza receptors by lectin binding (see table 1)
Virus Attachment | |||||
---|---|---|---|---|---|
HPAV H5N1 | Seasonal H1N1 | Seasonal H3N2 | H7N7 (2003) | H1N1pdm (2009) | |
Conjunctiva | No Data | No Data | No Data | No Data | No Data |
Nasal/
Nasopharynx | No (scant binding to nasopharynx and paranasal sinuses, ciliated cells) | Yes (Ciliated > goblet cells) | Yes (Ciliated > goblet cells) | No Data | Yes (Ciliated > goblet cells) |
Trachea | No | Yes | Yes | No Data | Yes |
Bronchus | Minimal | Yes | Yes | No Data | No Data |
Bronchiole | Yes | No | Minimal | Yes | No Data |
Lung | Yes (Type II) | Yes | Yes | Yes | No Data |
References | (Chutinimitkul et al., 2010; van Riel et al., 2006; van Riel et al., 2007) | (van Riel et al., 2010; van Riel et al., 2007) | (Chutinimitk ul et al., 2010; van Riel et al., 2007) | (de Wit et al., 2010) | (van Riel et al., 2010) |
Table 3
Infection and replication of influenza viruses of different subtypes in human respiratory and conjunctival epithelia
Virus Infection and Replication | |||||
---|---|---|---|---|---|
HPAV H5N1 | Seasonal H1N1 | Seasonal H3N2 | 2003 H7N7 | 2009 H1N1pdm | |
Conjunctiva | Yes | No | No | Yes | Yes |
Nasal/ Nasopharynx | Yes | Yes | Yes | No Data | Yes |
Bronchus | Limited infection without productive replication | Yes | Yes | No Data | Yes |
Lung | Yes (type II pneumocytes) | Yes | Yes/ No (strain dependent) | Yes | Yes |
Reference | (Nicholls et al., 2007c; Weinheimer et al., 2012) | (Chan et al., 2010a; Nicholls et al., 2007c) | (Chan et al., 2010a; Chan et al., 2011c; Nicholls et al., 2007c) | Chan et al., unpublish ed data. | (Chan et al., 2010a; Chan et al., 2011b) |
Acknowledgements
The authors’ research is supported by funding from the Area of Excellence Scheme of the University Grants Committee (AoE/M-12/06), Hong Kong SAR and Contract HHSN266200700005C from the National Institute of Allergy and Infectious Disease, National Institutes of Health, USA.
Footnotes
Publisher's Disclaimer: This is a PDF file of an unedited manuscript that has been accepted for publication. As a service to our customers we are providing this early version of the manuscript. The manuscript will undergo copyediting, typesetting, and review of the resulting proof before it is published in its final citable form. Please note that during the production process errors may be discovered which could affect the content, and all legal disclaimers that apply to the journal pertain.
Reference
- Abdel-Ghafar AN, Chotpitayasunondh T, Gao Z, Hayden FG, Nguyen DH, de Jong MD, Naghdaliyev A, Peiris JS, Shindo N, Soeroso S, Uyeki TM. Update on avian influenza A (H5N1) virus infection in humans. N Engl J Med. 2008;358(3):261–273. [Abstract] [Google Scholar]
- Adamson IY, Bowden DH. The type 2 cell as progenitor of alveolar epithelial regeneration. A cytodynamic study in mice after exposure to oxygen. Lab Invest. 1974;30(1):35–42. [Abstract] [Google Scholar]
- Baskin CR, Bielefeldt-Ohmann H, Tumpey TM, Sabourin PJ, Long JP, Garcia-Sastre A, Tolnay AE, Albrecht R, Pyles JA, Olson PH, Aicher LD, Rosenzweig ER, Murali-Krishna K, Clark EA, Kotur MS, Fornek JL, Proll S, Palermo RE, Sabourin CL, Katze MG. Early and sustained innate immune response defines pathology and death in nonhuman primates infected by highly pathogenic influenza virus. Proc Natl Acad Sci U S A. 2009;106(9):3455–3460. [Europe PMC free article] [Abstract] [Google Scholar]
- Baum LG, Paulson JC. Sialyloligosaccharides of the respiratory epithelium in the selection of human influenza virus receptor specificity. Acta Histochem Suppl. 1990;40:35–38. [Abstract] [Google Scholar]
- Beekhuizen H, van Furth R. Growth characteristics of cultured human macrovascular venous and arterial and microvascular endothelial cells. J Vasc Res. 1994;31(4):230–239. [Abstract] [Google Scholar]
- Belser JA, Gustin KM, Maines TR, Pantin-Jackwood MJ, Katz JM, Tumpey TM. Influenza virus respiratory infection and transmission following ocular inoculation in ferrets. PLoS Pathog. 2012;8(3):e1002569. [Europe PMC free article] [Abstract] [Google Scholar]
- Blixt O, Head S, Mondala T, Scanlan C, Huflejt ME, Alvarez R, Bryan MC, Fazio F, Calarese D, Stevens J, Razi N, Stevens DJ, Skehel JJ, van Die I, Burton DR, Wilson IA, Cummings R, Bovin N, Wong CH, Paulson JC. Printed covalent glycan array for ligand profiling of diverse glycan binding proteins. Proc Natl Acad Sci U S A. 2004;101(49):17033–17038. [Europe PMC free article] [Abstract] [Google Scholar]
- Booth JL, Coggeshall KM, Gordon BE, Metcalf JP. Adenovirus type 7 induces interleukin-8 in a lung slice model and requires activation of Erk. J Virol. 2004;78(8):4156–4164. [Europe PMC free article] [Abstract] [Google Scholar]
- Buchy P, Mardy S, Vong S, Toyoda T, Aubin JT, Miller M, Touch S, Sovann L, Dufourcq JB, Richner B, Tu PV, Tien NT, Lim W, Peiris JS, Van der Werf S. Influenza A/H5N1 virus infection in humans in Cambodia. J Clin Virol. 2007a;39(3):164–168. [Abstract] [Google Scholar]
- Buchy P, Mardy S, Vong S, Toyoda T, Aubin JT, Miller M, Touch S, Sovann L, Dufourcq JB, Richner B, Tu PV, Tien NT, Lim W, Peiris JS, Van der Werf S. Influenza A/H5N1 virus infection in humans in Cambodia. J Clin Virol. 2007b;39(3):164–168. [Abstract] [Google Scholar]
- Chan MC, Chan RW, Yu WC, Ho CC, Chui WH, Lo CK, Yuen KM, Guan YI, Nicholls JM, Peiris JS. Influenza H5N1 virus infection of polarized human alveolar epithelial cells and lung microvascular endothelial cells. Respiratory research. 2009;10:102. [Europe PMC free article] [Abstract] [Google Scholar]
- Chan MC, Chan RW, Yu WC, Ho CC, Yuen KM, Fong JH, Tang LL, Lai WW, Lo AC, Chui WH, Sihoe AD, Kwong DL, Wong DS, Tsao GS, Poon LL, Guan Y, Nicholls JM, Peiris JS. Tropism and innate host responses of the 2009 pandemic H1N1 influenza virus in ex vivo and in vitro cultures of human conjunctiva and respiratory tract. Am J Pathol. 2010a;176(4):1828–1840. [Europe PMC free article] [Abstract] [Google Scholar]
- Chan MC, Cheung CY, Chui WH, Tsao SW, Nicholls JM, Chan YO, Chan RW, Long HT, Poon LL, Guan Y, Peiris JS. Proinflammatory cytokine responses induced by influenza A (H5N1) viruses in primary human alveolar and bronchial epithelial cells. Respir Res. 2005;6:135. [Europe PMC free article] [Abstract] [Google Scholar]
- Chan MC, Lee N, Chan PK, To KF, Wong RY, Ho WS, Ngai KL, Sung JJ. Seasonal influenza A virus in feces of hospitalized adults. Emerging infectious diseases. 2011a;17(11):2038–2042. [Europe PMC free article] [Abstract] [Google Scholar]
- Chan PK, Lee N, Zaman M, Adisasmito W, Coker R, Hanshaoworakul W, Gasimov V, Oner AF, Dogan N, Tsang O, Phommasack B, Touch S, Bamgboye E, Swenson A, Toovey S, Dreyer NA. Determinants of Antiviral Effectiveness in Influenza Virus A Subtype H5N1. J Infect Dis. 2012 [Abstract] [Google Scholar]
- Chan RW, Kang SS, Yen HL, Li AC, Tang LL, Yu WC, Yuen KM, Chan IW, Wong DD, Lai WW, Kwong DL, Sihoe AD, Poon LL, Guan Y, Nicholls JM, Peiris JS, Chan MC. Tissue tropism of swine influenza viruses and reassortants in ex vivo cultures of the human respiratory tract and conjunctiva. J Virol. 2011b;85(22):11581–11587. [Europe PMC free article] [Abstract] [Google Scholar]
- Chan RW, Yuen KM, Yu WC, Ho CC, Nicholls JM, Peiris JS, Chan MC. Influenza H5N1 and H1N1 virus replication and innate immune responses in bronchial epithelial cells are influenced by the state of differentiation. PLoS One. 2010b;5(1):e8713. [Europe PMC free article] [Abstract] [Google Scholar]
- Chan RWY, Yuen KM, Yu WCL, Tang LLS, Li AC, Nicholls JM, Chan MCW. Replication of avian and seasonal influenza viruses in human bronchus and lung. Influenza and other respiratory viruses. 2011c;5:425–426. [Europe PMC free article] [Abstract] [Google Scholar]
- Cheung CY, Chan EY, Krasnoselsky A, Purdy D, Navare AT, Bryan JT, Leung CK, Hui KP, Peiris JS, Katze MG. H5N1 Virus Causes Significant Perturbations in Host Proteome Very Early in Influenza Virus-Infected Primary Human Monocyte-Derived Macrophages. J Infect Dis. 2012 [Europe PMC free article] [Abstract] [Google Scholar]
- Cheung CY, Poon LL, Lau AS, Luk W, Lau YL, Shortridge KF, Gordon S, Guan Y, Peiris JS. Induction of proinflammatory cytokines in human macrophages by influenza A (H5N1) viruses: a mechanism for the unusual severity of human disease? Lancet. 2002;360(9348):1831–1837. [Abstract] [Google Scholar]
- Chew EC, Yuen KW, Lee JC. Lectin histochemistry of normal and neoplastic nasopharyngeal epithelium. Anticancer Res. 1991;11(2):697–704. [Abstract] [Google Scholar]
- Childs RA, Palma AS, Wharton S, Matrosovich T, Liu Y, Chai W, Campanero-Rhodes MA, Zhang Y, Eickmann M, Kiso M, Hay A, Matrosovich M, Feizi T. Receptor-binding specificity of pandemic influenza A (H1N1) 2009 virus determined by carbohydrate microarray. Nat Biotechnol. 2009;27(9):797–799. [Europe PMC free article] [Abstract] [Google Scholar]
- Chutinimitkul S, van Riel D, Munster VJ, van den Brand JM, Rimmelzwaan GF, Kuiken T, Osterhaus AD, Fouchier RA, de Wit E. In vitro assessment of attachment pattern and replication efficiency of H5N1 influenza A viruses with altered receptor specificity. J Virol. 2010;84(13):6825–6833. [Europe PMC free article] [Abstract] [Google Scholar]
- Claas EC, Osterhaus AD, van Beek R, De Jong JC, Rimmelzwaan GF, Senne DA, Krauss S, Shortridge KF, Webster RG. Human influenza A H5N1 virus related to a highly pathogenic avian influenza virus. Lancet. 1998;351(9101):472–477. [Abstract] [Google Scholar]
- Colden-Stanfield M, Ratcliffe D, Cramer EB, Gallin EK. Characterization of influenza virus-induced leukocyte adherence to human umbilical vein endothelial cell monolayers. Journal of immunology. 1993;151(1):310–321. [Abstract] [Google Scholar]
- Couceiro JN, Paulson JC, Baum LG. Influenza virus strains selectively recognize sialyloligosaccharides on human respiratory epithelium; the role of the host cell in selection of hemagglutinin receptor specificity. Virus Res. 1993;29(2):155–165. [Abstract] [Google Scholar]
- Craig LE, Spelman JP, Strandberg JD, Zink MC. Endothelial cells from diverse tissues exhibit differences in growth and morphology. Microvasc Res. 1998;55(1):65–76. [Abstract] [Google Scholar]
- Crapo JD, Barry BE, Gehr P, Bachofen M, Weibel ER. Cell number and cell characteristics of the normal human lung. Am Rev Respir Dis. 1982;125(6):740–745. [Abstract] [Google Scholar]
- Crouch E, Hartshorn K, Ofek I. Collectins and pulmonary innate immunity. Immunol Rev. 2000;173:52–65. [Abstract] [Google Scholar]
- Das A, Boggaram V. Proteasome dysfunction inhibits surfactant protein gene expression in lung epithelial cells: mechanism of inhibition of SP-B gene expression. Am J Physiol Lung Cell Mol Physiol. 2007;292(1):L74–84. [Abstract] [Google Scholar]
- Daum N, Kuehn A, Hein S, Schaefer UF, Huwer H, Lehr CM. Isolation, cultivation, and application of human alveolar epithelial cells. Methods Mol Biol. 2012;806:31–42. [Abstract] [Google Scholar]
- de Jong MD, Bach VC, Phan TQ, Vo MH, Tran TT, Nguyen BH, Beld M, Le TP, Truong HK, Nguyen VV, Tran TH, Do QH, Farrar J. Fatal avian influenza A (H5N1) in a child presenting with diarrhea followed by coma. N Engl J Med. 2005;352(7):686–691. [Abstract] [Google Scholar]
- de Jong MD, Simmons CP, Thanh TT, Hien VM, Smith GJ, Chau TN, Hoang DM, Chau NV, Khanh TH, Dong VC, Qui PT, Cam BV, Ha do Q, Guan Y, Peiris JS, Chinh NT, Hien TT, Farrar J. Fatal outcome of human influenza A (H5N1) is associated with high viral load and hypercytokinemia. Nat Med. 2006;12(10):1203–1207. [Europe PMC free article] [Abstract] [Google Scholar]
- de Wit E, Munster VJ, van Riel D, Beyer WE, Rimmelzwaan GF, Kuiken T, Osterhaus AD, Fouchier RA. Molecular determinants of adaptation of highly pathogenic avian influenza H7N7 viruses to efficient replication in the human host. J Virol. 2010;84(3):1597–1606. [Europe PMC free article] [Abstract] [Google Scholar]
- Ekchariyawat P, Thitithanyanont A, Sirisinha S, Utaisincharoen P. Apoptosis induced by avian H5N1 virus in human monocyte-derived macrophages involves TRAIL-inducing caspase-10 activation. Innate Immun. 2012;18(3):390–397. [Abstract] [Google Scholar]
- Fang X, Song Y, Hirsch J, Galietta LJ, Pedemonte N, Zemans RL, Dolganov G, Verkman AS, Matthay MA. Contribution of CFTR to apical-basolateral fluid transport in cultured human alveolar epithelial type II cells. Am J Physiol Lung Cell Mol Physiol. 2006;290(2):L242–249. [Abstract] [Google Scholar]
- Fouchier RA, Schneeberger PM, Rozendaal FW, Broekman JM, Kemink SA, Munster V, Kuiken T, Rimmelzwaan GF, Schutten M, Van Doornum GJ, Koch G, Bosman A, Koopmans M, Osterhaus AD. Avian influenza A virus (H7N7) associated with human conjunctivitis and a fatal case of acute respiratory distress syndrome. Proc Natl Acad Sci U S A. 2004;101(5):1356–1361. [Europe PMC free article] [Abstract] [Google Scholar]
- Friedman HM, Macarak EJ, MacGregor RR, Wolfe J, Kefalides NA. Virus infection of endothelial cells. J Infect Dis. 1981;143(2):266–273. [Abstract] [Google Scholar]
- Geiler J, Michaelis M, Sithisarn P, Cinatl J., Jr. Comparison of pro-inflammatory cytokine expression and cellular signal transduction in human macrophages infected with different influenza A viruses. Med Microbiol Immunol. 2011;200(1):53–60. [Abstract] [Google Scholar]
- Geiss GK, Salvatore M, Tumpey TM, Carter VS, Wang X, Basler CF, Taubenberger JK, Bumgarner RE, Palese P, Katze MG, Garcia-Sastre A. Cellular transcriptional profiling in influenza A virus-infected lung epithelial cells: the role of the nonstructural NS1 protein in the evasion of the host innate defense and its potential contribution to pandemic influenza. Proc Natl Acad Sci U S A. 2002;99(16):10736–10741. [Europe PMC free article] [Abstract] [Google Scholar]
- Giard DJ, Aaronson SA, Todaro GJ, Arnstein P, Kersey JH, Dosik H, Parks WP. In vitro cultivation of human tumors: establishment of cell lines derived from a series of solid tumors. J Natl Cancer Inst. 1973;51(5):1417–1423. [Abstract] [Google Scholar]
- Gu J, Xie Z, Gao Z, Liu J, Korteweg C, Ye J, Lau LT, Lu J, Zhang B, McNutt MA, Lu M, Anderson VM, Gong E, Yu AC, Lipkin WI. H5N1 infection of the respiratory tract and beyond: a molecular pathology study. Lancet. 2007;370(9593):1137–1145. [Europe PMC free article] [Abstract] [Google Scholar]
- Gulisano M, Montella A, Orlandini SZ, Pacini P. Morphological study of fetal nasopharyngeal epithelium in man. Acta Anat (Basel) 1992;144(2):152–159. [Abstract] [Google Scholar]
- Guo Y, Rumschlag-Booms E, Wang J, Xiao H, Yu J, Guo L, Gao GF, Cao Y, Caffrey M, Rong L. Analysis of hemagglutinin-mediated entry tropism of H5N1 avian influenza. Virol J. 2009;6:39. [Europe PMC free article] [Abstract] [Google Scholar]
- Haraldsen G, Kvale D, Lien B, Farstad IN, Brandtzaeg P. Cytokine-regulated expression of E-selectin, intercellular adhesion molecule-1 (ICAM-1), and vascular cell adhesion molecule-1 (VCAM-1) in human microvascular endothelial cells. Journal of immunology. 1996;156(7):2558–2565. [Abstract] [Google Scholar]
- Higgins PG, Ellis EM, Woolley DA. A comparative study of standard methods and organ culture for the isolation of respiratory viruses. J Med Microbiol. 1969;2(2):109–115. [Abstract] [Google Scholar]
- Hillaire ML, van Eijk M, Nieuwkoop NJ, Vogelzang-van Trierum SE, Fouchier RA, Osterhaus AD, Haagsman HP, Rimmelzwaan GF. The number and position of N-linked glycosylation sites in the hemagglutinin determine differential recognition of seasonal and 2009 pandemic H1N1 influenza virus by porcine surfactant protein D. Virus Res. 2012 [Abstract] [Google Scholar]
- Hui KP, Lee SM, Cheung CY, Mao H, Lai AK, Chan RW, Chan MC, Tu W, Guan Y, Lau YL, Peiris JS. H5N1 influenza virus-induced mediators upregulate RIG-I in uninfected cells by paracrine effects contributing to amplified cytokine cascades. J Infect Dis. 2011;204(12):1866–1878. [Abstract] [Google Scholar]
- Hui KP, Lee SM, Cheung CY, Ng IH, Poon LL, Guan Y, Ip NY, Lau AS, Peiris JS. Induction of proinflammatory cytokines in primary human macrophages by influenza A virus (H5N1) is selectively regulated by IFN regulatory factor 3 and p38 MAPK. Journal of immunology. 2009;182(2):1088–1098. [Abstract] [Google Scholar]
- Ibricevic A, Pekosz A, Walter MJ, Newby C, Battaile JT, Brown EG, Holtzman MJ, Brody SL. Influenza virus receptor specificity and cell tropism in mouse and human airway epithelial cells. J Virol. 2006;80(15):7469–7480. [Europe PMC free article] [Abstract] [Google Scholar]
- Ichinohe T, Pang IK, Iwasaki A. Influenza virus activates inflammasomes via its intracellular M2 ion channel. Nat Immunol. 2010;11(5):404–410. [Europe PMC free article] [Abstract] [Google Scholar]
- Ilyushina NA, Bovin NV, Webster RG. Decreased neuraminidase activity is important for the adaptation of H5N1 influenza virus to human airway epithelium. J Virol. 2012;86(9):4724–4733. [Europe PMC free article] [Abstract] [Google Scholar]
- Ilyushina NA, Govorkova EA, Gray TE, Bovin NV, Webster RG. Human-like receptor specificity does not affect the neuraminidase-inhibitor susceptibility of H5N1 influenza viruses. PLoS Pathog. 2008;4(4):e1000043. [Europe PMC free article] [Abstract] [Google Scholar]
- Imai M, Watanabe T, Hatta M, Das SC, Ozawa M, Shinya K, Zhong G, Hanson A, Katsura H, Watanabe S, Li C, Kawakami E, Yamada S, Kiso M, Suzuki Y, Maher EA, Neumann G, Kawaoka Y. Experimental adaptation of an influenza H5 HA confers respiratory droplet transmission to a reassortant H5 HA/H1N1 virus in ferrets. Nature. 2012;486(7403):420–428. [Europe PMC free article] [Abstract] [Google Scholar]
- Ishiguro N, Takada A, Yoshioka M, Ma X, Kikuta H, Kida H, Kobayashi K. Induction of interferon-inducible protein-10 and monokine induced by interferon-gamma from human endothelial cells infected with Influenza A virus. Arch Virol. 2004;149(1):17–34. [Abstract] [Google Scholar]
- Jia D, Rahbar R, Chan RW, Lee SM, Chan MC, Wang BX, Baker DP, Sun B, Peiris JS, Nicholls JM, Fish EN. Influenza virus non-structural protein 1 (NS1) disrupts interferon signaling. PLoS One. 2010;5(11):e13927. [Europe PMC free article] [Abstract] [Google Scholar]
- Karchev T, Kabakchiev P. M-cells in the epithelium of the nasopharyngeal tonsil. Rhinology. 1984;22(3):201–210. [Abstract] [Google Scholar]
- Kobasa D, Jones SM, Shinya K, Kash JC, Copps J, Ebihara H, Hatta Y, Kim JH, Halfmann P, Hatta M, Feldmann F, Alimonti JB, Fernando L, Li Y, Katze MG, Feldmann H, Kawaoka Y. Aberrant innate immune response in lethal infection of macaques with the 1918 influenza virus. Nature. 2007;445(7125):319–323. [Abstract] [Google Scholar]
- Krenn BM, Egorov A, Romanovskaya-Romanko E, Wolschek M, Nakowitsch S, Ruthsatz T, Kiefmann B, Morokutti A, Humer J, Geiler J, Cinatl J, Michaelis M, Wressnigg N, Sturlan S, Ferko B, Batishchev OV, Indenbom AV, Zhu R, Kastner M, Hinterdorfer P, Kiselev O, Muster T, Romanova J. Single HA2 mutation increases the infectivity and immunogenicity of a live attenuated H5N1 intranasal influenza vaccine candidate lacking NS1. PLoS One. 2011;6(4):e18577. [Europe PMC free article] [Abstract] [Google Scholar]
- Law AH, Lee DC, Yuen KY, Peiris M, Lau AS. Cellular response to influenza virus infection: a potential role for autophagy in CXCL10 and interferon-alpha induction. Cell Mol Immunol. 2010;7(4):263–270. [Europe PMC free article] [Abstract] [Google Scholar]
- Lee SM, Cheung CY, Nicholls JM, Hui KP, Leung CY, Uiprasertkul M, Tipoe GL, Lau YL, Poon LL, Ip NY, Guan Y, Peiris JS. Hyperinduction of cyclooxygenase-2-mediated proinflammatory cascade: a mechanism for the pathogenesis of avian influenza H5N1 infection. J Infect Dis. 2008;198(4):525–535. [Abstract] [Google Scholar]
- Lee SM, Gardy JL, Cheung CY, Cheung TK, Hui KP, Ip NY, Guan Y, Hancock RE, Peiris JS. Systems-level comparison of host-responses elicited by avian H5N1 and seasonal H1N1 influenza viruses in primary human macrophages. PLoS One. 2009;4(12):e8072. [Europe PMC free article] [Abstract] [Google Scholar]
- Liao HY, Hsu CH, Wang SC, Liang CH, Yen HY, Su CY, Chen CH, Jan JT, Ren CT, Cheng TJ, Wu CY, Wong CH. Differential receptor binding affinities of influenza hemagglutinins on glycan arrays. J Am Chem Soc. 2010;132(42):14849–14856. [Abstract] [Google Scholar]
- Liem NT, Nakajima N, Phat le P, Sato Y, Thach HN, Hung PV, San LT, Katano H, Kumasaka T, Oka T, Kawachi S, Matsushita T, Sata T, Kudo K, Suzuki K. H5N1-infected cells in lung with diffuse alveolar damage in exudative phase from a fatal case in Vietnam. Jpn J Infect Dis. 2008;61(2):157–160. [Abstract] [Google Scholar]
- Maines TR, Jayaraman A, Belser JA, Wadford DA, Pappas C, Zeng H, Gustin KM, Pearce MB, Viswanathan K, Shriver ZH, Raman R, Cox NJ, Sasisekharan R, Katz JM, Tumpey TM. Transmission and pathogenesis of swine-origin 2009 A(H1N1) influenza viruses in ferrets and mice. Science. 2009;325(5939):484–487. [Europe PMC free article] [Abstract] [Google Scholar]
- Mansour DE, El-Shazly AA, Elawamry AI, Ismail AT. Comparison of Ocular Findings in Patients with H1N1 Influenza Infection versus Patients Receiving Influenza Vaccine during a Pandemic. Ophthalmic Res. 2012;48(3):134–138. [Abstract] [Google Scholar]
- Mason RJ. Biology of alveolar type II cells. Respirology. 2006;11(Suppl):S12–15. [Abstract] [Google Scholar]
- Matrosovich MN, Matrosovich TY, Gray T, Roberts NA, Klenk HD. Human and avian influenza viruses target different cell types in cultures of human airway epithelium. Proc Natl Acad Sci U S A. 2004;101(13):4620–4624. [Europe PMC free article] [Abstract] [Google Scholar]
- McBride S, Rannie D, Harrison DJ. Gene transfer to adult human lung tissue ex vivo. Gene Ther. 2000;7(8):675–678. [Abstract] [Google Scholar]
- Mgbemena V, Segovia JA, Chang TH, Tsai SY, Cole GT, Hung CY, Bose S. Transactivation of Inducible Nitric Oxide Synthase Gene by Kruppel-like Factor 6 Regulates Apoptosis during Influenza A Virus Infection. Journal of immunology. 2012;189(2):606–615. [Europe PMC free article] [Abstract] [Google Scholar]
- Michaelis M, Geiler J, Klassert D, Doerr HW, Cinatl J., Jr. Infection of human retinal pigment epithelial cells with influenza A viruses. Invest Ophthalmol Vis Sci. 2009;50(11):5419–5425. [Abstract] [Google Scholar]
- Minamino T, Komuro I. Regeneration of the endothelium as a novel therapeutic strategy for acute lung injury. J Clin Invest. 2006;116(9):2316–2319. [Europe PMC free article] [Abstract] [Google Scholar]
- Mok CK, Lee DC, Cheung CY, Peiris M, Lau AS. Differential onset of apoptosis in influenza A virus H5N1- and H1N1-infected human blood macrophages. J Gen Virol. 2007;88(Pt 4):1275–1280. [Abstract] [Google Scholar]
- Mok KP, Wong CH, Cheung CY, Chan MC, Lee SM, Nicholls JM, Guan Y, Peiris JS. Viral genetic determinants of H5N1 influenza viruses that contribute to cytokine dysregulation. J Infect Dis. 2009;200(7):1104–1112. [Europe PMC free article] [Abstract] [Google Scholar]
- Muramoto Y, Ozaki H, Takada A, Park CH, Sunden Y, Umemura T, Kawaoka Y, Matsuda H, Kida H. Highly pathogenic H5N1 influenza virus causes coagulopathy in chickens. Microbiol Immunol. 2006;50(1):73–81. [Abstract] [Google Scholar]
- Murphy HS, Bakopoulos N, Dame MK, Varani J, Ward PA. Heterogeneity of vascular endothelial cells: differences in susceptibility to neutrophil-mediated injury. Microvasc Res. 1998;56(3):203–211. [Abstract] [Google Scholar]
- Ng YP, Lee SM, Cheung TK, Nicholls JM, Peiris JS, Ip NY. Avian influenza H5N1 virus induces cytopathy and proinflammatory cytokine responses in human astrocytic and neuronal cell lines. Neuroscience. 2010;168(3):613–623. [Abstract] [Google Scholar]
- Nicholls JM, Bourne AJ, Chen H, Guan Y, Peiris JS. Sialic acid receptor detection in the human respiratory tract: evidence for widespread distribution of potential binding sites for human and avian influenza viruses. Respir Res. 2007a;8:73. [Europe PMC free article] [Abstract] [Google Scholar]
- Nicholls JM, Chan MC, Chan WY, Wong HK, Cheung CY, Kwong DL, Wong MP, Chui WH, Poon LL, Tsao SW, Guan Y, Peiris JS. Tropism of avian influenza A (H5N1) in the upper and lower respiratory tract. Nat Med. 2007b;13(2):147–149. [Abstract] [Google Scholar]
- Nicholls JM, Chan MC, Chan WY, Wong HK, Cheung CY, Kwong DL, Wong MP, Chui WH, Poon LL, Tsao SW, Guan Y, Peiris JS. Tropism of avian influenza A (H5N1) in the upper and lower respiratory tract. Nat Med. 2007c;13(2):147–149. [Abstract] [Google Scholar]
- Nilsen EM, Johansen FE, Jahnsen FL, Lundin KE, Scholz T, Brandtzaeg P, Haraldsen G. Cytokine profiles of cultured microvascular endothelial cells from the human intestine. Gut. 1998;42(5):635–642. [Europe PMC free article] [Abstract] [Google Scholar]
- Nimmerjahn F, Dudziak D, Dirmeier U, Hobom G, Riedel A, Schlee M, Staudt LM, Rosenwald A, Behrends U, Bornkamm GW, Mautner J. Active NF-kappaB signalling is a prerequisite for influenza virus infection. J Gen Virol. 2004;85(Pt 8):2347–2356. [Abstract] [Google Scholar]
- Ocana-Macchi M, Bel M, Guzylack-Piriou L, Ruggli N, Liniger M, McCullough KC, Sakoda Y, Isoda N, Matrosovich M, Summerfield A. Hemagglutinin-dependent tropism of H5N1 avian influenza virus for human endothelial cells. J Virol. 2009;83(24):12947–12955. [Europe PMC free article] [Abstract] [Google Scholar]
- Olofsson S, Kumlin U, Dimock K, Arnberg N. Avian influenza and sialic acid receptors: more than meets the eye? Lancet Infect Dis. 2005;5(3):184–188. [Abstract] [Google Scholar]
- Oshansky CM, Pickens JA, Bradley KC, Jones LP, Saavedra-Ebner GM, Barber JP, Crabtree JM, Steinhauer DA, Tompkins SM, Tripp RA. Avian influenza viruses infect primary human bronchial epithelial cells unconstrained by sialic acid alpha2,3 residues. PLoS One. 2011;6(6):e21183. [Europe PMC free article] [Abstract] [Google Scholar]
- Pauli EK, Schmolke M, Wolff T, Viemann D, Roth J, Bode JG, Ludwig S. Influenza A virus inhibits type I IFN signaling via NF-kappaB-dependent induction of SOCS-3 expression. PLoS Pathog. 2008;4(11):e1000196. [Europe PMC free article] [Abstract] [Google Scholar]
- Peiris JS, Hui KP, Yen HL. Host response to influenza virus: protection versus immunopathology. Curr Opin Immunol. 2010;22(4):475–481. [Europe PMC free article] [Abstract] [Google Scholar]
- Peiris JS, Yu WC, Leung CW, Cheung CY, Ng WF, Nicholls JM, Ng TK, Chan KH, Lai ST, Lim WL, Yuen KY, Guan Y. Re-emergence of fatal human influenza A subtype H5N1 disease. Lancet. 2004;363(9409):617–619. [Europe PMC free article] [Abstract] [Google Scholar]
- Perrone LA, Plowden JK, Garcia-Sastre A, Katz JM, Tumpey TM. H5N1 and 1918 pandemic influenza virus infection results in early and excessive infiltration of macrophages and neutrophils in the lungs of mice. PLoS Pathog. 2008;4(8):e1000115. [Europe PMC free article] [Abstract] [Google Scholar]
- Rimmelzwaan GF, Nieuwkoop NJ, de Mutsert G, Boon AC, Kuiken T, Fouchier RA, Osterhaus AD. Attachment of infectious influenza A viruses of various subtypes to live mammalian and avian cells as measured by flow cytometry. Virus Res. 2007;129(2):175–181. [Abstract] [Google Scholar]
- Rogers GN, Paulson JC. Receptor determinants of human and animal influenza virus isolates: differences in receptor specificity of the H3 hemagglutinin based on species of origin. Virology. 1983;127(2):361–373. [Abstract] [Google Scholar]
- Roome AP, Dickinson V, Caul EO. Simplified organ cultures of human embryo trachea in the diagnosis of viral respiratory disease of children. J Clin Pathol. 1971;24(6):487–490. [Europe PMC free article] [Abstract] [Google Scholar]
- Sakabe S, Iwatsuki-Horimoto K, Takano R, Nidom CA, Le M, Nagamura-Inoue T, Horimoto T, Yamashita N, Kawaoka Y. Cytokine production by primary human macrophages infected with highly pathogenic H5N1 or pandemic H1N1 2009 influenza viruses. J Gen Virol. 2011;92(Pt 6):1428–1434. [Europe PMC free article] [Abstract] [Google Scholar]
- Shieh WJ, Blau DM, Denison AM, Deleon-Carnes M, Adem P, Bhatnagar J, Sumner J, Liu L, Patel M, Batten B, Greer P, Jones T, Smith C, Bartlett J, Montague J, White E, Rollin D, Gao R, Seales C, Jost H, Metcalfe M, Goldsmith CS, Humphrey C, Schmitz A, Drew C, Paddock C, Uyeki TM, Zaki SR. 2009 pandemic influenza A (H1N1): pathology and pathogenesis of 100 fatal cases in the United States. Am J Pathol. 2010;177(1):166–175. [Europe PMC free article] [Abstract] [Google Scholar]
- Shinya K, Ebina M, Yamada S, Ono M, Kasai N, Kawaoka Y. Avian flu: influenza virus receptors in the human airway. Nature. 2006;440(7083):435–436. [Abstract] [Google Scholar]
- Shinya K, Makino A, Tanaka H, Hatta M, Watanabe T, Le MQ, Imai H, Kawaoka Y. Systemic dissemination of H5N1 influenza A viruses in ferrets and hamsters after direct intragastric inoculation. J Virol. 2011;85(10):4673–4678. [Europe PMC free article] [Abstract] [Google Scholar]
- Shu Y, Yu H, Li D. Lethal avian influenza A (H5N1) infection in a pregnant woman in Anhui Province, China. N Engl J Med. 2006;354(13):1421–1422. [Abstract] [Google Scholar]
- Sieben C, Kappel C, Zhu R, Wozniak A, Rankl C, Hinterdorfer P, Grubmuller H, Herrmann A. Influenza virus binds its host cell using multiple dynamic interactions. Proc Natl Acad Sci U S A. 2012;109(34):13626–13631. [Europe PMC free article] [Abstract] [Google Scholar]
- Steinberg BE, Goldenberg NM, Lee WL. Do viral infections mimic bacterial sepsis? The role of microvascular permeability: A review of mechanisms and methods. Antiviral Res. 2012;93(1):2–15. [Abstract] [Google Scholar]
- Stevens J, Blixt O, Chen LM, Donis RO, Paulson JC, Wilson IA. Recent avian H5N1 viruses exhibit increased propensity for acquiring human receptor specificity. J Mol Biol. 2008;381(5):1382–1394. [Europe PMC free article] [Abstract] [Google Scholar]
- Stevens J, Blixt O, Paulson JC, Wilson IA. Glycan microarray technologies: tools to survey host specificity of influenza viruses. Nat Rev Microbiol. 2006a;4(11):857–864. [Europe PMC free article] [Abstract] [Google Scholar]
- Stevens J, Blixt O, Tumpey TM, Taubenberger JK, Paulson JC, Wilson IA. Structure and receptor specificity of the hemagglutinin from an H5N1 influenza virus. Science. 2006b;312(5772):404–410. [Abstract] [Google Scholar]
- Stewart CE, Torr EE, Mohd Jamili NH, Bosquillon C, Sayers I. Evaluation of differentiated human bronchial epithelial cell culture systems for asthma research. J Allergy (Cairo) 2012;2012:943982. [Europe PMC free article] [Abstract] [Google Scholar]
- Suksatu A, Sangsawad W, Thitithanyanont A, Smittipat N, Fukuda MM, Ubol S. Characteristics of stork feces-derived H5N1 viruses that are preferentially transmitted to primary human airway epithelial cells. Microbiol Immunol. 2009;53(12):675–684. [Abstract] [Google Scholar]
- Sumikoshi M, Hashimoto K, Kawasaki Y, Sakuma H, Suzutani T, Suzuki H, Hosoya M. Human influenza virus infection and apoptosis induction in human vascular endothelial cells. J Med Virol. 2008;80(6):1072–1078. [Abstract] [Google Scholar]
- Sun Y, Li C, Shu Y, Ju X, Zou Z, Wang H, Rao S, Guo F, Liu H, Nan W, Zhao Y, Yan Y, Tang J, Zhao C, Yang P, Liu K, Wang S, Lu H, Li X, Tan L, Gao R, Song J, Gao X, Tian X, Qin Y, Xu KF, Li D, Jin N, Jiang C. Inhibition of autophagy ameliorates acute lung injury caused by avian influenza A H5N1 infection. Sci Signal. 2012;5(212):ra16. [Abstract] [Google Scholar]
- Suptawiwat O, Tantilipikorn P, Boonarkart C, Lumyongsatien J, Uiprasertkul M, Puthavathana P, Auewarakul P. Enhanced susceptibility of nasal polyp tissues to avian and human influenza viruses. PLoS One. 2010;5(9):e12973. [Europe PMC free article] [Abstract] [Google Scholar]
- Swain RJ, Kemp SJ, Goldstraw P, Tetley TD, Stevens MM. Spectral monitoring of surfactant clearance during alveolar epithelial type II cell differentiation. Biophys J. 2008;95(12):5978–5987. [Europe PMC free article] [Abstract] [Google Scholar]
- Swerlick RA, Lee KH, Li LJ, Sepp NT, Caughman SW, Lawley TJ. Regulation of vascular cell adhesion molecule 1 on human dermal microvascular endothelial cells. Journal of immunology. 1992;149(2):698–705. [Abstract] [Google Scholar]
- Tate MD, Deng YM, Jones JE, Anderson GP, Brooks AG, Reading PC. Neutrophils ameliorate lung injury and the development of severe disease during influenza infection. Journal of immunology. 2009;183(11):7441–7450. [Abstract] [Google Scholar]
- Taub DD, Lloyd AR, Conlon K, Wang JM, Ortaldo JR, Harada A, Matsushima K, Kelvin DJ, Oppenheim JJ. Recombinant human interferon-inducible protein 10 is a chemoattractant for human monocytes and T lymphocytes and promotes T cell adhesion to endothelial cells. J Exp Med. 1993;177(6):1809–1814. [Europe PMC free article] [Abstract] [Google Scholar]
- Terraciano AJ, Wang N, Schuman JS, Haffner G, Panjwani N, Zhao Z, Yang Z. Sialyl Lewis X, Lewis X, and N-acetyllactosamine expression on normal and glaucomatous eyes. Curr Eye Res. 1999;18(2):73–78. [Abstract] [Google Scholar]
- Thitithanyanont A, Engering A, Ekchariyawat P, Wiboon-ut S, Limsalakpetch A, Yongvanitchit K, Kum-Arb U, Kanchongkittiphon W, Utaisincharoen P, Sirisinha S, Puthavathana P, Fukuda MM, Pichyangkul S. High susceptibility of human dendritic cells to avian influenza H5N1 virus infection and protection by IFN-alpha and TLR ligands. Journal of immunology. 2007;179(8):5220–5227. [Abstract] [Google Scholar]
- Trevenan W, Karamansja R, Chan R, Chan M, Air G, Hopton C, Dell A, Peiris J, Haslam S, Wong M, Nicholls J. Glycomic Analysis of Human Respiratory Tract Tissues and Correlation with Influenza Virus Infection. PLoS Pathog. 2013 in press. [Europe PMC free article] [Abstract] [Google Scholar]
- Tumpey TM, Lu X, Morken T, Zaki SR, Katz JM. Depletion of lymphocytes and diminished cytokine production in mice infected with a highly virulent influenza A (H5N1) virus isolated from humans. J Virol. 2000;74(13):6105–6116. [Europe PMC free article] [Abstract] [Google Scholar]
- Tyrrell DA, Blamire CJ. Improvements in a method of growing respiratory viruses in organ cultures. Br J Exp Pathol. 1967;48(2):217–227. [Europe PMC free article] [Abstract] [Google Scholar]
- Tyrrell DA, Hoorn B. The growth of some myxoviruses in organ cultures. Br J Exp Pathol. 1965;46(5):514–518. [Europe PMC free article] [Abstract] [Google Scholar]
- Uiprasertkul M, Kitphati R, Puthavathana P, Kriwong R, Kongchanagul A, Ungchusak K, Angkasekwinai S, Chokephaibulkit K, Srisook K, Vanprapar N, Auewarakul P. Apoptosis and pathogenesis of avian influenza A (H5N1) virus in humans. Emerg Infect Dis. 2007;13(5):708–712. [Europe PMC free article] [Abstract] [Google Scholar]
- Uiprasertkul M, Puthavathana P, Sangsiriwut K, Pooruk P, Srisook K, Peiris M, Nicholls JM, Chokephaibulkit K, Vanprapar N, Auewarakul P. Influenza A H5N1 replication sites in humans. Emerg Infect Dis. 2005;11(7):1036–1041. [Europe PMC free article] [Abstract] [Google Scholar]
- van Riel D, den Bakker MA, Leijten LM, Chutinimitkul S, Munster VJ, de Wit E, Rimmelzwaan GF, Fouchier RA, Osterhaus AD, Kuiken T. Seasonal and pandemic human influenza viruses attach better to human upper respiratory tract epithelium than avian influenza viruses. Am J Pathol. 2010;176(4):1614–1618. [Europe PMC free article] [Abstract] [Google Scholar]
- van Riel D, Leijten LM, van der Eerden M, Hoogsteden HC, Boven LA, Lambrecht BN, Osterhaus AD, Kuiken T. Highly pathogenic avian influenza virus H5N1 infects alveolar macrophages without virus production or excessive TNF-alpha induction. PLoS Pathog. 2011;7(6):e1002099. [Europe PMC free article] [Abstract] [Google Scholar]
- van Riel D, Munster VJ, de Wit E, Rimmelzwaan GF, Fouchier RA, Osterhaus AD, Kuiken T. H5N1 Virus Attachment to Lower Respiratory Tract. Science. 2006;312(5772):399. [Abstract] [Google Scholar]
- van Riel D, Munster VJ, de Wit E, Rimmelzwaan GF, Fouchier RA, Osterhaus AD, Kuiken T. Human and avian influenza viruses target different cells in the lower respiratory tract of humans and other mammals. Am J Pathol. 2007;171(4):1215–1223. [Europe PMC free article] [Abstract] [Google Scholar]
- Visseren FL, Bouwman JJ, Bouter KP, Diepersloot RJ, de Groot PH, Erkelens DW. Procoagulant activity of endothelial cells after infection with respiratory viruses. Thromb Haemost. 2000;84(2):319–324. [Abstract] [Google Scholar]
- Visseren FL, Verkerk MS, Bouter KP, Diepersloot RJ, Erkelens DW. Interleukin-6 production by endothelial cells after infection with influenza virus and cytomegalovirus. J Lab Clin Med. 1999;134(6):623–630. [Abstract] [Google Scholar]
- Wang J, Edeen K, Manzer R, Chang Y, Wang S, Chen X, Funk CJ, Cosgrove GP, Fang X, Mason RJ. Differentiated human alveolar epithelial cells and reversibility of their phenotype in vitro. Am J Respir Cell Mol Biol. 2007;36(6):661–668. [Europe PMC free article] [Abstract] [Google Scholar]
- Wareing MD, Shea AL, Inglis CA, Dias PB, Sarawar SR. CXCR2 is required for neutrophil recruitment to the lung during influenza virus infection, but is not essential for viral clearance. Viral Immunol. 2007;20(3):369–378. [Abstract] [Google Scholar]
- Weinheimer VK, Becher A, Tonnies M, Holland G, Knepper J, Bauer TT, Schneider P, Neudecker J, Ruckert JC, Szymanski K, Temmesfeld-Wollbrueck B, Gruber AD, Bannert N, Suttorp N, Hippenstiel S, Wolff T, Hocke AC. Influenza A viruses target type II pneumocytes in the human lung. J Infect Dis. 2012 [Europe PMC free article] [Abstract] [Google Scholar]
- Wheeler AH, Nungester WJ. Effect of Mucin on Influenza Virus Infection in Hamsters. Science. 1942;96(2482):92–93. [Abstract] [Google Scholar]
- White MR, Tecle T, Crouch EC, Hartshorn KL. Impact of neutrophils on antiviral activity of human bronchoalveolar lavage fluid. Am J Physiol Lung Cell Mol Physiol. 2007;293(5):L1293–1299. [Abstract] [Google Scholar]
- Wiwanitkit V. Hemostatic disorders in bird flu infection. Blood Coagul Fibrinolysis. 2008;19(1):5–6. [Abstract] [Google Scholar]
- Wong DD, Choy KT, Chan RW, Sia SF, Chiu HP, Cheung PP, Chan MC, Peiris JS, Yen HL. Comparable Fitness and Transmissibility between Oseltamivir-Resistant Pandemic 2009 and Seasonal H1N1 Influenza Viruses with the H275Y Neuraminidase Mutation. J Virol. 2012;86(19):10558–10570. [Europe PMC free article] [Abstract] [Google Scholar]
- Wong SS, Yuen KY. Avian influenza virus infections in humans. Chest. 2006;129(1):156–168. [Europe PMC free article] [Abstract] [Google Scholar]
- Yao L, Korteweg C, Hsueh W, Gu J. Avian influenza receptor expression in H5N1-infected and noninfected human tissues. FASEB J. 2008;22(3):733–740. [Abstract] [Google Scholar]
- Yen HL, Liang CH, Wu CY, Forrest HL, Ferguson A, Choy KT, Jones J, Wong DD, Cheung PP, Hsu CH, Li OT, Yuen KM, Chan RW, Poon LL, Chan MC, Nicholls JM, Krauss S, Wong CH, Guan Y, Webster RG, Webby RJ, Peiris M. Hemagglutinin-neuraminidase balance confers respiratory-droplet transmissibility of the pandemic H1N1 influenza virus in ferrets. Proc Natl Acad Sci U S A. 2011;108(34):14264–14269. [Europe PMC free article] [Abstract] [Google Scholar]
- Yu WC, Chan RW, Wang J, Travanty EA, Nicholls JM, Peiris JS, Mason RJ, Chan MC. Viral replication and innate host responses in primary human alveolar epithelial cells and alveolar macrophages infected with influenza H5N1 and H1N1 viruses. J Virol. 2011;85(14):6844–6855. [Europe PMC free article] [Abstract] [Google Scholar]
- Zeng H, Goldsmith C, Thawatsupha P, Chittaganpitch M, Waicharoen S, Zaki S, Tumpey TM, Katz JM. Highly pathogenic avian influenza H5N1 viruses elicit an attenuated type i interferon response in polarized human bronchial epithelial cells. J Virol. 2007;81(22):12439–12449. [Europe PMC free article] [Abstract] [Google Scholar]
- Zeng H, Pappas C, Belser JA, Houser KV, Zhong W, Wadford DA, Stevens T, Balczon R, Katz JM, Tumpey TM. Human pulmonary microvascular endothelial cells support productive replication of highly pathogenic avian influenza viruses: possible involvement in the pathogenesis of human H5N1 virus infection. J Virol. 2012;86(2):667–678. [Europe PMC free article] [Abstract] [Google Scholar]
- Zhang Z, Zhang J, Huang K, Li KS, Yuen KY, Guan Y, Chen H, Ng WF. Systemic infection of avian influenza A virus H5N1 subtype in humans. Hum Pathol. 2009;40(5):735–739. [Europe PMC free article] [Abstract] [Google Scholar]
- Zhao Y, Lu M, Lau LT, Lu J, Gao Z, Liu J, Yu AC, Cao Q, Ye J, McNutt MA, Gu J. Neutrophils may be a vehicle for viral replication and dissemination in human H5N1 avian influenza. Clin Infect Dis. 2008;47(12):1575–1578. [Europe PMC free article] [Abstract] [Google Scholar]
- Zhou J, Law HK, Cheung CY, Ng IH, Peiris JS, Lau YL. Differential expression of chemokines and their receptors in adult and neonatal macrophages infected with human or avian influenza viruses. J Infect Dis. 2006a;194(1):61–70. [Europe PMC free article] [Abstract] [Google Scholar]
- Zhou J, Law HK, Cheung CY, Ng IH, Peiris JS, Lau YL. Functional tumor necrosis factor-related apoptosis-inducing ligand production by avian influenza virus-infected macrophages. J Infect Dis. 2006b;193(7):945–953. [Europe PMC free article] [Abstract] [Google Scholar]
Full text links
Read article at publisher's site: https://doi.org/10.1016/j.virusres.2013.03.003
Read article for free, from open access legal sources, via Unpaywall:
https://europepmc.org/articles/pmc3805758?pdf=render
Citations & impact
Impact metrics
Citations of article over time
Alternative metrics

Discover the attention surrounding your research
https://www.altmetric.com/details/119587754
Smart citations by scite.ai
Explore citation contexts and check if this article has been
supported or disputed.
https://scite.ai/reports/10.1016/j.virusres.2013.03.003
Article citations
Infection of the Ex Vivo Tonsil Model by HTLV-1 Envelope-Pseudotyped Viruses.
Pathogens, 12(2):182, 24 Jan 2023
Cited by: 3 articles | PMID: 36839454 | PMCID: PMC9958901
Recent advances in airborne pathogen detection using optical and electrochemical biosensors.
Anal Chim Acta, 1234:340297, 23 Aug 2022
Cited by: 9 articles | PMID: 36328717 | PMCID: PMC9395976
Review Free full text in Europe PMC
Human-type sialic acid receptors contribute to avian influenza A virus binding and entry by hetero-multivalent interactions.
Nat Commun, 13(1):4054, 13 Jul 2022
Cited by: 25 articles | PMID: 35831293 | PMCID: PMC9279479
Could Interleukin-33 (IL-33) Govern the Outcome of an Equine Influenza Virus Infection? Learning from Other Species.
Viruses, 13(12):2519, 15 Dec 2021
Cited by: 1 article | PMID: 34960788 | PMCID: PMC8704309
Review Free full text in Europe PMC
Risk Assessment for Highly Pathogenic Avian Influenza A(H5N6/H5N8) Clade 2.3.4.4 Viruses.
Emerg Infect Dis, 27(10):2619-2627, 01 Oct 2021
Cited by: 10 articles | PMID: 34545790 | PMCID: PMC8462306
Go to all (28) article citations
Similar Articles
To arrive at the top five similar articles we use a word-weighted algorithm to compare words from the Title and Abstract of each citation.
Tropism, replication competence, and innate immune responses of influenza virus: an analysis of human airway organoids and ex-vivo bronchus cultures.
Lancet Respir Med, 6(11):846-854, 11 Jul 2018
Cited by: 72 articles | PMID: 30001996
Tropism and Infectivity of a Seasonal A(H1N1) and a Highly Pathogenic Avian A(H5N1) Influenza Virus in Primary Differentiated Ferret Nasal Epithelial Cell Cultures.
J Virol, 93(10):e00080-19, 01 May 2019
Cited by: 18 articles | PMID: 30814288 | PMCID: PMC6498040
Tropism and innate host responses of a novel avian influenza A H7N9 virus: an analysis of ex-vivo and in-vitro cultures of the human respiratory tract.
Lancet Respir Med, 1(7):534-542, 25 Jul 2013
Cited by: 63 articles | PMID: 24461614 | PMCID: PMC7164816
Avian influenza viruses in mammals.
Rev Sci Tech, 28(1):137-159, 01 Apr 2009
Cited by: 66 articles | PMID: 19618623
Review
Funding
Funders who supported this work.
NIAID NIH HHS (1)
Grant ID: HHSN266200700005C
PHS HHS (1)
Grant ID: 266200700005C