Abstract
Background
Acute exposure to air pollution has been linked to myocardial infarction, but its effect on heart failure is uncertain. We did a systematic review and meta-analysis to assess the association between air pollution and acute decompensated heart failure including hospitalisation and heart failure mortality.Methods
Five databases were searched for studies investigating the association between daily increases in gaseous (carbon monoxide, sulphur dioxide, nitrogen dioxide, ozone) and particulate (diameter <2·5 μm [PM2·5] or <10 μm [PM10]) air pollutants, and heart failure hospitalisations or heart failure mortality. We used a random-effects model to derive overall risk estimates per pollutant.Findings
Of 1146 identified articles, 195 were reviewed in-depth with 35 satisfying inclusion criteria. Heart failure hospitalisation or death was associated with increases in carbon monoxide (3·52% per 1 part per million; 95% CI 2·52-4·54), sulphur dioxide (2·36% per 10 parts per billion; 1·35-3·38), and nitrogen dioxide (1·70% per 10 parts per billion; 1·25-2·16), but not ozone (0·46% per 10 parts per billion; -0·10 to 1·02) concentrations. Increases in particulate matter concentration were associated with heart failure hospitalisation or death (PM2·5 2·12% per 10 μg/m(3), 95% CI 1·42-2·82; PM10 1·63% per 10 μg/m(3), 95% CI 1·20-2·07). Strongest associations were seen on the day of exposure, with more persistent effects for PM2·5. In the USA, we estimate that a mean reduction in PM2·5 of 3·9 μg/m(3) would prevent 7978 heart failure hospitalisations and save a third of a billion US dollars a year.Interpretation
Air pollution has a close temporal association with heart failure hospitalisation and heart failure mortality. Although more studies from developing nations are required, air pollution is a pervasive public health issue with major cardiovascular and health economic consequences, and it should remain a key target for global health policy.Funding
British Heart Foundation.Free full text
Global association of air pollution and heart failure: a systematic review and meta-analysis
Summary
Background
Acute exposure to air pollution has been linked to myocardial infarction, but its effect on heart failure is uncertain. We did a systematic review and meta-analysis to assess the association between air pollution and acute decompensated heart failure including hospitalisation and heart failure mortality.
Methods
Five databases were searched for studies investigating the association between daily increases in gaseous (carbon monoxide, sulphur dioxide, nitrogen dioxide, ozone) and particulate (diameter <2·5 μm [PM2·5] or <10 μm [PM10]) air pollutants, and heart failure hospitalisations or heart failure mortality. We used a random-effects model to derive overall risk estimates per pollutant.
Findings
Of 1146 identified articles, 195 were reviewed in-depth with 35 satisfying inclusion criteria. Heart failure hospitalisation or death was associated with increases in carbon monoxide (3·52% per 1 part per million; 95% CI 2·52–4·54), sulphur dioxide (2·36% per 10 parts per billion; 1·35–3·38), and nitrogen dioxide (1·70% per 10 parts per billion; 1·25–2·16), but not ozone (0·46% per 10 parts per billion; −0·10 to 1·02) concentrations. Increases in particulate matter concentration were associated with heart failure hospitalisation or death (PM2·5 2·12% per 10 μg/m3, 95% CI 1·42–2·82; PM10 1·63% per 10 μg/m3, 95% CI 1·20–2·07). Strongest associations were seen on the day of exposure, with more persistent effects for PM2·5. In the USA, we estimate that a mean reduction in PM2·5 of 3·9 μg/m3 would prevent 7978 heart failure hospitalisations and save a third of a billion US dollars a year.
Interpretation
Air pollution has a close temporal association with heart failure hospitalisation and heart failure mortality. Although more studies from developing nations are required, air pollution is a pervasive public health issue with major cardiovascular and health economic consequences, and it should remain a key target for global health policy.
Funding
British Heart Foundation.
Introduction
The adverse effects of air pollution on cardiovascular health have been established in a series of major epidemiological and observational studies.1–4 WHO estimates that air pollution is responsible for over a million premature deaths worldwide every year.5 Even brief exposures to air pollution have been associated with increases in cardiovascular mortality,6,7 particularly in susceptible populations.
Heart failure is an escalating public health issue that affects more than 23 million people worldwide,8 with an increasing prevalence in elderly people.9,10 It has an annual hospitalisation rate of 2% with subsequent 1-year mortality of 30%.11 Heart failure ranks as the most frequent reason for hospitalisation and rehospitalisation in older people,12,13 accounting for 5% of all hospital discharge diagnoses. The triggers of acute cardiac decompensation especially in susceptible individuals are therefore a major public health concern.
Population and individual level exposures to air pollution are associated with acute cardiovascular events such as myocardial infarction.14,15 However, the effect of air pollution on other cardiovascular conditions, such as acute decompensated heart failure, has been less well described.16 This issue is important because there are major differences in the mechanisms that trigger myocardial infarction compared with acute decompensated heart failure.17–19
Several studies of short-term exposure to air pollution have included heart failure hospitalisation and mortality, although these endpoints have not been the primary focus in most analyses. We therefore systematically reviewed the evidence examining the association between air pollution and acute decompensated heart failure, including hospitalisation and heart failure mortality.
Methods
Databases
We searched Ovid Medline, Embase, Global Health, Cumulative Index to Nursing and Allied Health Literature (CINAHL), and Web of Science using the following keywords: “heart failure”, “congestive cardiac failure”, “air pollution”, “particulate matter”, “ozone”, “carbon monoxide”, “sulphur dioxide”, and “nitrogen dioxide”. The full search criteria are available in the appendix. Bibliographic reference lists of studies selected for inclusion in our meta-analysis and relevant review articles were manually searched (appendix). We limited our search to studies published between 1948 and July 15, 2012.
Selection of articles and extraction of data
Studies were included if they presented original data for gaseous (carbon monoxide, sulphur dioxide, nitrogen dioxide, ozone) or particulate (PM2·5 or PM10) air pollutants and reported heart failure hospitalisation or heart failure mortality. We included all studies that reported associations between exposure and outcome up to and including lag (day) 7. There were no language restrictions and we included only peer-reviewed original articles.
Data were extracted independently by two investigators (ASVS and JPL) and conflicts were adjudicated by a third investigator (ALH). We contacted authors for additional data or clarification where needed.
Both case-crossover and time-series studies were included. The case-crossover design compares exposure in a case period when the event occurred with exposure in specified control periods.20 This design can control for individual characteristics such as age, sex, and comorbidity, as well as secular trends and seasonal patterns using a time-stratified approach, but assumes time-varying risk factors are constant within reference periods.21 Time-series studies were used to assess the relation between exposure and outcome using regression analysis accounting for confounding factors, such as meteorological parameters, but are less effective at controlling for secular trends such as seasonality.22 The study design, study population, and adjustment undertaken for potential confounders have been summarised for each study in the appendix.
Data synthesis
Relative risks (RR) were pooled for a standardised increment in pollutant concentration as follows: 10 μg/m3 for PM2·5 and PM10, 10 parts per billion for nitrogen dioxide (NO2), sulphur dioxide (SO2), and ozone (O3), and 1 part per million for carbon monoxide (CO). Many studies used generalised linear models and therefore we assumed a linear relation between exposure and outcome. Standardised risk estimates were calculated for each study using the following formula:
Four studies reported stratified risk estimates by age,23 location,24 and temperature25,26 rather than overall risk estimates, and the stratified estimates were included in our meta-analysis. Two studies reported results from the same population using both case-crossover and time-series analysis27,28 and estimates from the time-series analyses were included. Three studies29–31 subsequently revised their time series analyses and the revised estimates were included.32,33 Time-series analyses were mainly based on routine administrative datasets and did not adjust for individual characteristics such as age, sex, or socioeconomic status. For all studies, we pooled adjusted risk estimates controlling for meteorological, temporal, and seasonal parameters (appendix).
Many studies provided multiple estimates for single lags (for example lag 0 or lag 1) and were pooled separately. We only pooled estimates for single lags where more than three estimates were available. The shortest lag was used to assess overall risk estimates. A few studies only provided cumulative lags (for example lag 0–1 or 0–2), and were not suitable for pooling in the single lag analysis, but were used to determine overall risk estimates.
Additional analyses
We did additional analyses stratifying studies by study design (time-series vs case-crossover), geographical location (USA vs non-USA), age (all ages vs ≥65 years of age), and outcome (heart failure hospitalisation vs heart failure mortality). We assumed that the prevalence of air pollution exposure was 100% and therefore calculated population-attributable risks per pollutant using our overall risk estimates and the formula:
Funnel plots were constructed for assessment of publication bias (data not shown) and assessed for asymmetry using Egger's regression test.34 Asymmetry was then corrected using the trim and fill method, with adjusted relative risks and number of studies adjusted presented per pollutant.35
We used PM2·5 to illustrate the potential effect of reducing air pollution concentration on heart failure hospitalisations in the USA. For each state we obtained the number of heart failure hospitalisations and average cost per hospitalisation (amount charged for the hospital stay excluding professional fees) from the US Healthcare Cost and Utilization Project State Inpatient Database13 and the Chronic Condition and Data Warehouse (appendix). The median daily PM2·5 concentration was calculated for each state from the Centers for Disease Control and Prevention's Wide-ranging Online Data for Epidemiologic Research (WONDER) database. In each state, we estimated the population-attributable risks and annual reduction in heart failure hospitalisations per 100 000 people for a reduction in PM2·5 concentration to 5·8 μg/m3. This concentration represents a target threshold below which the adverse health effects of PM2·5 are uncertain.36,37
Statistical analysis
We anticipated heterogeneity between studies due to different study designs, methods of analysis, different lag exposures, and geographical and population differences. We used a random-effects model to account for both within and between study heterogeneity. Heterogeneity was examined using the standard I2 test. As this test has limited power when applied to a small number of studies, we considered the presence of heterogeneity at 10% level of significance and I2 exceeding 30%. The analysis was done using Comprehensive Meta-Analysis (version 2.0, 2005, Biostat Inc, NJ, USA) and Stata Software (Version 11·2 2011, StataCORP, TX, USA). Statistical significance was taken as two-sided p<0·05.
Role of the funding source
The sponsor of the study had no role in study design, data collection, data analysis, data interpretation, or writing of the report. The corresponding author had full access to all the data in the study and had final responsibility for the decision to submit for publication.
Results
The abstracts of 1146 articles were assessed and 195 studies underwent in-depth review, with 35 studies fulfilling the inclusion criteria. Ten studies used a case-crossover design,26,28,38–46 24 used a times-series design,24,27,29,30,47–66 and one used both study designs67 incorporating four million events across the world (table 1).
Table 1
Contextual details of studies included in the meta-analysis by publication year
Location | Published | Period | Study design | Data source | Population | Number of events* | Outcome | |
---|---|---|---|---|---|---|---|---|
Belleudi et al38 | Italy | 2010 | 2001–05 | Case-crossover | Hospital discharge registry | ≥65 years | 17 561 | HA |
Bell et al47 | USA | 2009 | 1999–2005 | Time-series | Medicare data | All | 1 142 928 | HA |
Haley et al39 | USA | 2009 | 2001–05 | Case-crossover | NYSDOH registry | All | 170 502 | HA |
Stieb et al49 | Canada | 2009 | 1999–2000 | Time-series | Emergency department registry | All | 32 313 | HA |
Ueda et al50 | Japan | 2009 | 2002–04 | Time-series | Ministry of Health | ≥65 years | 17 548 | Mortality |
Zanobetti et al51 | USA | 2009 | 2000–03 | Time-series | Medicare data | All | 238 587 | HA |
Colais et al40,46† | Italy | 2009 | 2001–05 | Case-crossover | Hospital discharge registry | ≥65 years | 55 339 | HA |
Forastiere et al41 | Italy | 2008 | 1997–2004 | Case-crossover | Regional registries of cause of death | All | 9569 | Mortality |
Yang et al26 | Taiwan | 2008 | 1996–2004 | Case-crossover | National Health Institute registry | All | 24 240 | HA |
Lee et al25 | Taiwan | 2007 | 1996–2004 | Time-series | National Health Institute registry | All | 13 475 | HA |
Peel et al28‡ | USA | 2007 | 1993–2000 | Case-crossover | Billing records | >64 years | 20 073 | HA |
Martins et al53 | Brazil | 2006 | 1996–2001 | Time-series | Department of Data Analysis of the Unified Health System | ≥65 years | 24 476 | HA |
Dominici et al54 | USA | 2006 | 1999–2002 | Time-series | Medicare data | ≥65 years | 986 392 | HA |
Wellenius et al43 | USA | 2006 | 1986–99 | Case-crossover | Medicare and Medicaid data | All | 292 918 | HA |
Barnett et al23 | Australia and New Zealand | 2006 | 1998–2001 | Case-crossover | Government health departments (Australia) and Ministry of Health (NZ) | ≥65 years | NR | HA |
Wellenius et al42 | USA | 2005 | 1987–99 | Case-crossover | Medicare and Medicaid data | ≥65 years | 55 019 | HA |
Bateson et al45 | USA | 2004 | 1988–91 | Case-crossover | Medicare and Medicaid data | All | 26 923 | Mortality |
Metzger et al27‡ | USA | 2004 | 1993–2000 | Time-series | Billing data | All | 20 073 | HA |
Goldberg et al29,32§ | Canada | 2003 | 1984–93 | Time-series | Billing and prescription data | ≥65 years | 16 794 | Mortality |
Koken et al55 | USA | 2003 | 1993–97 | Time-series | Agency for Healthcare Research and Quality | All | 1860 | HA |
McGowan et al56 | New Zealand | 2002 | 1988–98 | Time-series | Hospital data admission registry | All | 5146 | HA |
Hoek et al30,32§ | Netherlands | 2001 | 1986–94 | Time-series | Death certificates | All | 45 333 | Mortality |
Kwon et al67 | South Korea | 2001 | 1994–98 | Case-crossover and time-series | Mortality records | ≥65 years | 1807 | Mortality |
Ye et al57 | Japan | 2001 | 1980–95 | Time-series | Ministry of Health | ≥65 years | 4469 | HA |
Lippmann et al32,58§ | USA | 2000 | 1992–94 | Time-series | Medicare data | All | 18 615 | HA |
Stieb et al48¶ | Canada | 2000 | 1992–94 | Time-series | Emergency department registry | >30 years | 1312 | HA |
Linn et al59 | USA | 2000 | 1992–95 | Time-series | CA OSHPD | All | 71 540 | HA |
Wong TW et al60 | Hong Kong | 1999 | 1994–95 | Time-series | Hospital data admission registry | All | NR | HA |
Burnett et al63 | Canada | 1999 | 1980–94 | Time-series | Ontario Ministry of Health | All | 49 311 | HA |
Wong CM et al61 | Hong Kong | 1999 | 1995–97 | Time-series | Hospital authority data | ≥65 years | NR | HA |
Morris et al64 | USA | 1998 | 1986–89 | Time-series | Medicare data | ≥65 years | 49 640 | HA |
Burnett et al62 | Canada | 1997 | 1981–91 | Time-series | Hospital discharge records | ≥65 years | 157 865 | HA |
Poloniecki et al66 | UK | 1997 | 1987–94 | Time-series | Hospital episode records | ≥65 years | 62 853 | HA |
Morris et al24‖ | USA | 1995 | 1986–89 | Time-series | Medicare data | ≥65 years | 227 985 | HA |
Schwartz et al65‖ | USA | 1995 | 1986–89 | Time-series | Medicare data | ≥65 years | 38 862 | HA |
HA=Hospital admissions. NYSDOH=New York State Department of Health. NR=not reported. CA OSHPD=California Office of Statewide Health Planning and Development.
There was a positive association between heart failure hospitalisation or heart failure mortality, and all gaseous and particulate air pollutants except ozone (figure 1). The strongest associations were seen at lag 0, with this effect diminishing at longer lag times. Carbon monoxide was the most frequently studied gaseous pollutant, and showed a 3·52% (95% CI 2·52–4·54%) increase in heart failure hospitalisations or mortality per 1 part per million increment across nearly two million events. Both PM2·5 (2·12%, 95% CI 1·42–2·82) and PM10 (1·63%, 1·20–2·07) were positively associated with heart failure hospitalisation or mortality with a marked temporal relation and the strongest associations present at lag 0.

Association between (A) gaseous and (B) particulate air pollutants and heart failure hospitalisation or heart failure mortality
ppm=parts per million. ppb=parts per billion.
We did additional analyses by outcome, study design, age, and geographical location (figure 2). There was no change in effect direction across all pollutants in these analyses. Publication bias (Egger's test for asymmetry, p<0·05) was noted for all pollutants except ozone (table 2). Adjusting for asymmetry using the trim and fill method did not alter the effect direction but, as expected, did attenuate the effect size. We observed heterogeneity across all pollutants, which was most evident for nitrogen dioxide and carbon monoxide (I2 of 91%) and least evident for PM2·5 (I2 of 53%).
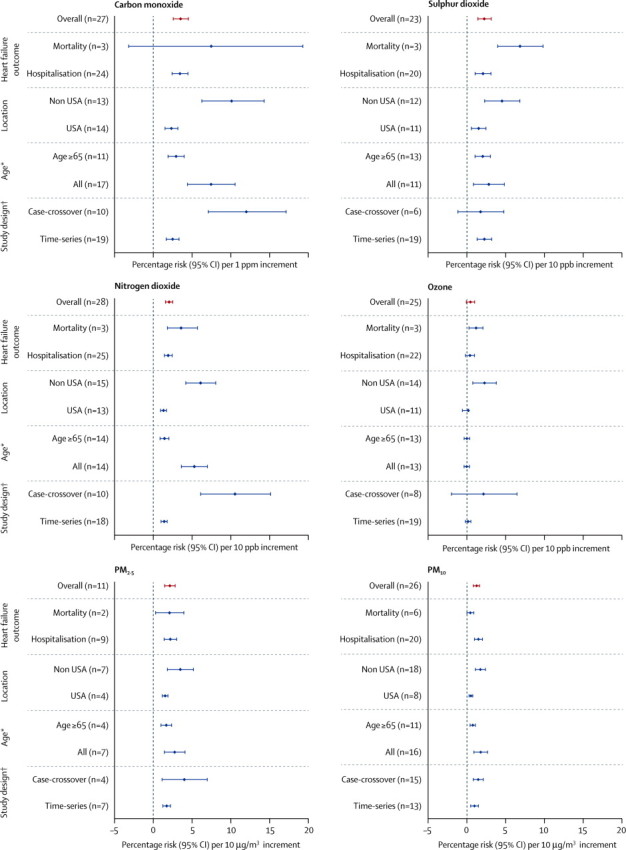
Additional analysis across all gaseous and particulate air pollutants
*Kwon et al67 provided separate estimates for all age groups and for people older than 75 years. This study therefore appears twice in the additional analysis when stratified by age. For the overall analysis, we have used the estimates provided for all age groups. †Kwon and Peel et al27,28,67 provided separate estimates stratified by study design and therefore appear twice in the additional analysis. For the overall analysis, we have used the estimates provided for the time-series study design. ppm=parts per million. ppb=parts per billion.
Table 2
Heterogeneity, population-attributable risk, and assessment for publication bias stratified by gaseous and particulate air pollutants
Gaseous pollutants | Particulate matter | ||||||
---|---|---|---|---|---|---|---|
Carbon monoxide (ppm) | Nitrogen dioxide (ppb) | Sulphur dioxide (ppb) | Ozone(ppb) | PM2.5 (μg/m3) | PM10(μg/m3) | ||
Increment | 1 ppm | 10 ppb | 10 ppb | 10 ppb | 10 μg/m3 | 10 μg/m3 | |
Median pollutant concentration (IQR)* | 1·1 (0·9–1·6) | 26·4 (22·5–30·1) | 6·3 (4·7–11·9) | 23·5 (17·6–32·0) | 15·0 (10·8–17·6) | 38·0 (27·0–45·5) | |
Range (min–max)† | 0·6–5·6 | 16·0–77·0 | 3·0–32·0 | 12·3–75·0 | 4·5–20·5 | 19·0–75·3 | |
Number of studies | 18 | 18 | 14 | 18 | 10 | 22 | |
Number of estimates | 27 | 28 | 23 | 25 | 11 | 26 | |
Heterogeneity, I2 | 91% | 91% | 78% | 87% | 53% | 75% | |
Population-attributable risk, % (95% CI)‡ | 3·41 (2·46–4·34) | 1·67 (1·23–2·11) | 2·31 (1·33–3·27) | N/A | 2·06 (1·38–2·72) | 1·60 (1·18–2·03) | |
Publication bias | |||||||
Egger regression test, p value | <0·001 | 0·028 | 0·009 | 0·304 | 0·003 | 0·007 | |
Non-adjusted RR (95% CI)§ | 1·035 (1·025–1·045) | 1·017 (1·012–1·022) | 1·024 (1·014–1·034) | 1·005 (0·999–1·011) | 1·021 (1·014–1·028) | 1·016 (1·012–1·021) | |
Adjusted RR (95% CI) ¶ | 1·018 (1·007–1·029) | 1·009 (1·004–1·014) | 1·014 (1·003–1·026) | 1·001 (0·995–1·007) | 1·016 (1·008–1·023) | 1·010 (1·005–1·016) | |
Number of studies adjusted | 12 | 10 | 6 | 2 | 6 | 6 |
ppm=parts per million. ppb=parts per billion. PM=particulate matter. PAR=population-attributable risk. IQR=interquartile range.
Median daily PM2·5 concentrations varied across states, with the highest population-attributable risks seen in Mississippi, Kentucky, and Tennessee and the lowest in Utah, Wyoming, and North Dakota (appendix). Reducing PM2·5 concentrations to 5·8 μg/m3 in each state would require a mean reduction in PM2·5 of 3·9 μg/m3 across the USA. The greatest effect on heart failure hospitalisations would be in those states with the highest median daily PM2·5 concentrations (figure 3). We estimate that this reduction would prevent 7978 heart failure hospitalisations and would be associated with savings of around US$307 million per year (appendix).
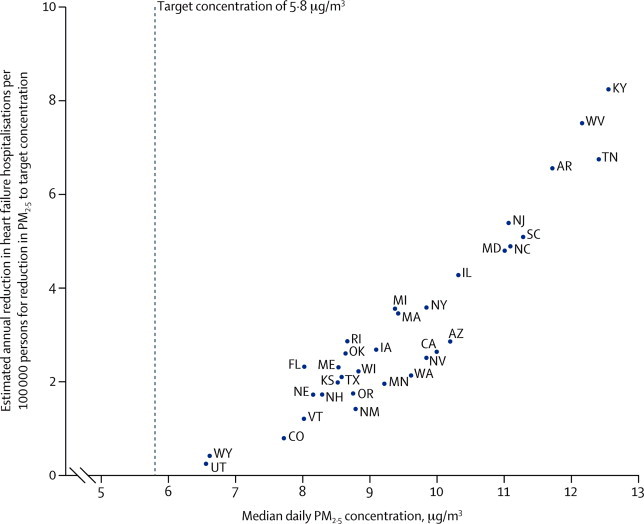
Median daily PM2·5 concentrations and estimated impact of a reduction in PM2·5 to a target concentration on heart failure hospitalisation per US state
Heart failure hospitalisation rates were not available for 15 states (appendix); data not shown for Mississippi (median daily PM2·5 13·4 μg/m3; annual reduction in heart failure hospitalisations 15 per 100 000). US state abbreviations are defined in the appendix.
Discussion
There were robust and clear temporal associations between exposure to air pollutants and heart failure hospitalisations and mortality. The magnitude and direction of our overall estimates persisted despite conservative modelling. All studies except one were done in developed countries where even modest improvements in air quality standards are projected to have major population health benefits and substantial health-care cost savings.
The effect of air pollution on heart failure hospitalisation and mortality might be underestimated. First, our estimates are based on acute events associated with short-term exposures and do not take into account the adverse effects of chronic exposure to air pollution.3 We have not considered long-term studies of air pollution in the current meta-analysis and therefore are unable to quantify any additive temporal effects of air pollution. Second, although our meta-analysis estimates the effect of short-term increases in air pollution on the population, this effect is likely to be greater in patients with pre-existing heart failure. Unfortunately, we were unable to stratify our analysis on the presence, severity, or phenotype of pre-existing heart failure, since these data were not available. Third, regional monitoring sites are likely to underestimate personal exposure in individuals living near major roadways. This may be an important consideration in estimating individual risk given that traffic-related air pollutants are thought to be the primary mediators of the cardiovascular effects of air pollution.19
A recent assessment of the global burden of disease ranked PM2·5 air pollution as one of the leading causes of death and disability worldwide.37 The American Thoracic Society recently advocated stricter standards for PM2·5 recommending a 10 μg/m3 reduction in daily maximum concentrations to 25 μg/m3.68 Recent studies indicate the persistence of adverse health effects at concentrations below those recommended by WHO.69 In our impact analysis, we estimate that reducing median daily PM2·5 concentrations by a mean of 3·9 μg/m3 would prevent roughly 8000 heart failure hospitalisations in the USA, with an associated saving of nearly a third of a billion dollars per annum. Smaller reductions in PM2·5 would prevent fewer hospitalisations, but could still confer significant public health benefits.
Urban cities in developing countries are likely to have PM2·5 concentrations up to 10-fold higher than the US National Ambient Air Quality Standards.70,71 So-called megacities, with populations well above 10 million people such as New Delhi in India and Beijing in China, have daily PM2·5 concentrations of 100–300 μg/m3 compared with a median PM2·5 concentration of 15 μg/m3 in the cities included in our meta-analysis.70,72 However, assessment of the effect of air pollution in developing countries is difficult because of a lack of cohesive air quality policies in combination with poor environmental monitoring and a paucity of disease surveillance data.73
The lack of data from developing countries is concerning because these regions are likely to be affected most and have the greatest potential to improve health. The problem is highlighted in our meta-analysis where only one of the 35 studies was done in a developing country.53 In areas with high levels of air pollution there are likely to be more frequent and more marked changes in air pollution exposure on a daily basis.72 Whether actual or relative increases in exposure would determine outcomes in these regions is uncertain.
In our additional analysis stratifying studies by location, we found risk estimates were almost twice as high in countries outside the USA where ambient concentrations are generally higher. As such, caution is necessary when extrapolating overall risk estimates from our meta-analysis to regions with higher air pollution concentrations.
Most hospitalisations in patients with heart failure are due to acute decompensated heart failure and dysrhythmias,74 with fewer patients hospitalised because of coexisting coronary heart disease and pulmonary disease.16 The biological mechanisms precipitating acute decompensation in patients with heart failure are likely to differ substantially from those involved in triggering acute myocardial infarction.19 Acute decompensated heart failure can be caused by increasing demand on the heart, such as increased heart rate, blood pressure, and filling pressures, or further impairment of cardiac performance, such as reduced contractility and increased myocardial injury. Exposure to particulate matter air pollution has been associated with increased systemic blood pressure and vasoconstriction.75–77 Both pulmonary and right ventricular diastolic filling pressures are increased by exposure to ambient particulate matter, suggesting a pulmonary vasoconstrictor effect of air pollution.78 Together with arrhythmias,79 these effects of air pollution will markedly increase the demands on the failing heart and thereby potentially precipitate acute decompensation. In addition to loss of contractile capacity through myocardial infarction,80 inhalation of particulate matter is associated with adverse ventricular remodelling and a worsening of myocardial fibrosis.81 These factors could have synergistic detrimental effects on cardiac function.
Although particulate matter is considered to be responsible for most adverse cardiovascular outcomes,82 we cannot exclude an effect of non-particulate air pollutants either in isolation or combination. We noted an adverse relation between exposure to all gaseous pollutants except for ozone and heart failure outcomes. The acute effects of carbon monoxide exposure on cardiac function are well known,83 but most of these studies have assessed the effects of exposure to more than 1000 parts per million of carbon monoxide as a model of cigarette smoking.84,85 Ambient carbon monoxide or nitrogen dioxide concentrations might simply reflect exposure to road traffic or combustion derived particles. Chamber studies also show that exposure to gaseous pollutants alone at high ambient concentrations does not cause acute cardiovascular dysfunction.86,87
Several limitations of our study should be considered. First, we found significant heterogeneity across all pollutants, which could indicate differences in population demographics, sample size, patient characteristics, and exposure misclassification due to variation in the accuracy of regional air pollution monitoring. However, pooled risk estimates showed consistency across all pollutants and the effect direction was not changed in our additional analyses. Second, we report estimates for single pollutants, which do not take into consideration potential additive effects of multiple pollutants or adjustments for collinearity.88 Third, meta-analysis of observational studies has limitations with inherent biases. We noticed significant publication bias across all pollutants, except ozone. However, after adjustment for asymmetry, the overall effect direction remained unchanged. Fourth, most studies pooled in our meta-analysis used data from routine administrative sources. There was limited validation of outcomes, with coding error and misclassification potentially giving rise to non-differential bias. However, nine of the 35 studies in our meta-analysis, encompassing almost 2 million events, used Medicare's hospital claims database. Coding for heart failure has been validated by case note review and found to have 84% agreement with the principal diagnosis.89 Finally, we did not have access to primary data and were unable to establish whether multiple hospitalisations might have occurred in the same patient. This point is important, since patients with recurrent hospitalisations could be more susceptible to the effects of air pollution.
Acute decompensated heart failure is a common, costly, and often fatal condition. Change in gaseous and particulate air pollutant concentrations have a marked and close temporal association with adverse outcomes in heart failure. More high-quality studies are urgently needed to establish the effect of air pollution on heart failure outcomes in middle-income and low-income countries. Although the causality and biological mechanisms need further exploration, air pollution is a pervasive public health issue with major cardiovascular and health-care economic consequences presenting a key target for national and international intervention.
Acknowledgments
ASVS, DEN, and NLM are supported by a Clinical Research Fellowship (SS/CH/09/002), Chair (CH/09/002), and Intermediate Clinical Research Fellowship (FS/10/024/28266), respectively, from the British Heart Foundation. This research is supported by a British Heart Foundation Programme Grant (RG/10/9/28286). We thank Michelle Bell (Yale University), Mark Goldberg (McGill University), and William Linn (University of Southern California) for providing additional data for this meta-analysis.
Contributors
ASVS conceived and designed the study. ASVS, JPL, and ALH acquired the data. ASVS, HN, DEN, and NLM analysed and interpreted the data. ASVS, DEN, and NLM drafted the initial manuscript. ASVS, JPL, HN, DAM, ALH, KD, DEN, and NLM made critical revisions of the manuscript for important intellectual content. All authors approved the final version of the report.
References
Full text links
Read article at publisher's site: https://doi.org/10.1016/s0140-6736(13)60898-3
Read article for free, from open access legal sources, via Unpaywall:
http://www.thelancet.com/article/S0140673613608983/pdf
Citations & impact
Impact metrics
Article citations
Inhaling Poor Health: The Impact of Air Pollution on Cardiovascular Kidney Metabolic Syndrome.
Methodist Debakey Cardiovasc J, 20(5):47-58, 05 Nov 2024
Cited by: 0 articles | PMID: 39525378
Review
Impact of Indoor Air Pollutants on the Cardiovascular Health Outcomes of Older Adults: Systematic Review.
Clin Interv Aging, 19:1629-1639, 01 Oct 2024
Cited by: 0 articles | PMID: 39372166 | PMCID: PMC11453128
Review Free full text in Europe PMC
Lung megakaryocytes engulf inhaled airborne particles to promote intrapulmonary inflammation and extrapulmonary distribution.
Nat Commun, 15(1):7396, 27 Aug 2024
Cited by: 0 articles | PMID: 39191805 | PMCID: PMC11349891
Estimating the short-term effect of PM2.5 on the mortality of cardiovascular diseases based on instrumental variables.
BMC Public Health, 24(1):2085, 01 Aug 2024
Cited by: 0 articles | PMID: 39090601 | PMCID: PMC11295497
Air pollution and kidney cancer risk: a systematic review and meta-analysis.
J Nephrol, 37(7):1779-1790, 24 Jun 2024
Cited by: 0 articles | PMID: 38913266 | PMCID: PMC11519201
Review Free full text in Europe PMC
Go to all (409) article citations
Data
Data behind the article
This data has been text mined from the article, or deposited into data resources.
BioStudies: supplemental material and supporting data
Similar Articles
To arrive at the top five similar articles we use a word-weighted algorithm to compare words from the Title and Abstract of each citation.
Association between short-term exposure to ambient air pollution and heart failure: An updated systematic review and meta-analysis of more than 7 million participants.
Front Public Health, 10:948765, 23 Jan 2023
Cited by: 4 articles | PMID: 36755739 | PMCID: PMC9900180
Review Free full text in Europe PMC
Part 2. Association of daily mortality with ambient air pollution, and effect modification by extremely high temperature in Wuhan, China.
Res Rep Health Eff Inst, (154):91-217, 01 Nov 2010
Cited by: 20 articles | PMID: 21446212
Part 5. Public health and air pollution in Asia (PAPA): a combined analysis of four studies of air pollution and mortality.
Res Rep Health Eff Inst, (154):377-418, 01 Nov 2010
Cited by: 12 articles | PMID: 21446215
Air pollution exposure and heart failure: A systematic review and meta-analysis.
Sci Total Environ, 872:162191, 11 Feb 2023
Cited by: 5 articles | PMID: 36781139
Review
Funding
Funders who supported this work.
British Heart Foundation (5)
Derivation and characterisation of endothelial cells from induced pluripotent stem cells in patients with atherosclerosis
Professor Nicholas Mills, University of Edinburgh
Grant ID: FS/10/024/28266
Atherothrombotic effects of air pollution (renewal)
David Newby, University of Edinburgh
Grant ID: RG/10/9/28286
Grant ID: SS/CH/09/002
Fire-fighters and acute myocardial infarction: understanding the mechanisms and reducing cardiovascular risk
Professor Nicholas Mills, University of Edinburgh
Grant ID: PG/11/27/28842
Grant ID: CH/09/002
British Heart Foundation Programme (1)
Grant ID: RG/10/9/28286
Chair (1)
Grant ID: CH/09/002
Clinical Research Fellowship (1)
Grant ID: SS/CH/09/002
Intermediate Clinical Research Fellowship (1)
Grant ID: FS/10/024/28266
Medical Research Council (1)
University of Edinburgh/MRC Centre for Inflammation Research
Professor John Iredale, University of Edinburgh
Grant ID: G0901697