Abstract
Free full text

Mammary gland tumor formation in transgenic mice overexpressing stromelysin-1
Abstract
An intact basement membrane (BM) is essential for the proper function, differentiation and morphology of many epithelial cells. The disruption or loss of this BM occurs during normal development as well as in the disease state. To examine the importance of BM during mammary gland development in vivo, we generated transgenic mice that inappropriately express autoactivating isoforms of the matrix metalloproteinase stromelysin-1. The mammary glands from these mice are both functionally and morphologically altered throughout development. We have now documented a dramatic incidence of breast tumors in several independent lines of these mice. These data suggest that overexpression of stromelysin-1 and disruption of the BM may be a key step in the multi-step process of breast cancer.
Proteinases have been shown to play a role in tissue remodeling during the normal processes of embryo implantation, wound healing, ovulation, and involution of the uterus, prostate and mammary gland (for review see ref 1). Extracellular matrix (ECM)-degrading proteinases have also been implicated in pathological processes such as rheumatoid arthritis and in all stages of tumor progression, including growth, angiogenesis, invasion and metastasis (for review see ref 2). In order for tumor cells to spread to other parts of the body, they must gain entry to the stroma, where they penetrate the lymph nodes or blood vessels (intravasation) and thereby the systemic circulation. Once carried to a distant site from the primary tumor, surviving cells may exit the circulation (extravasation) into the target organ and form a secondary tumor colony (metastases). ECM-degrading proteinases have been implicated in the intra- and extravasation of tumor cells across basement membranes (BM) (for review see refs 3,4). A variety of ECM-degrading enzymes, including matrix metalloproteinases and serine proteinases, have been shown to be induced or activated in tumor cells.4–6
We have recently described the production and characterization of transgenic mice that overexpress the matrix metalloproteinase stromelysin-1 (SL-1) in the mammary gland.7 The mammary gland of prepubertal female mouse is essentially quiescent. However, with the onset of ovarian activity (between 20–25 days), primary ducts, once confined to the immediate area of the teat, form large, club-shaped terminal end buds and infiltrate the fat pad. These ducts usually arrive at the end of the fat pad at about day 70 (mature virgin), and the gland remains dormant until pregnancy. Examination of glands taken from normal 70-day virgin females revealed the typical branching pattern (Figure 1A). However, the mammary glands from SL-1 transgenic mice, which express very low levels of the transgene, showed precocious alveolar development and maturation, with primary and secondary ducts that filled the fat pad (Figure 1C). This phenotype is both morphologically and functionally (as measured by their ability to express (β-casein) very similar to a normal 9–12-day pregnant gland. An intermediate phenotype was captured at day 50 of virgin transgenic development (Figure 1B). The right side of the panel represents the most mature part of the gland, near the teat structure, which displays alveolar development similar to the transgenic gland in panel C. However, the most immature part of the gland, on the left side of the panel, resembles the normal virgin gland that is still branching as evidenced by the large end buds.
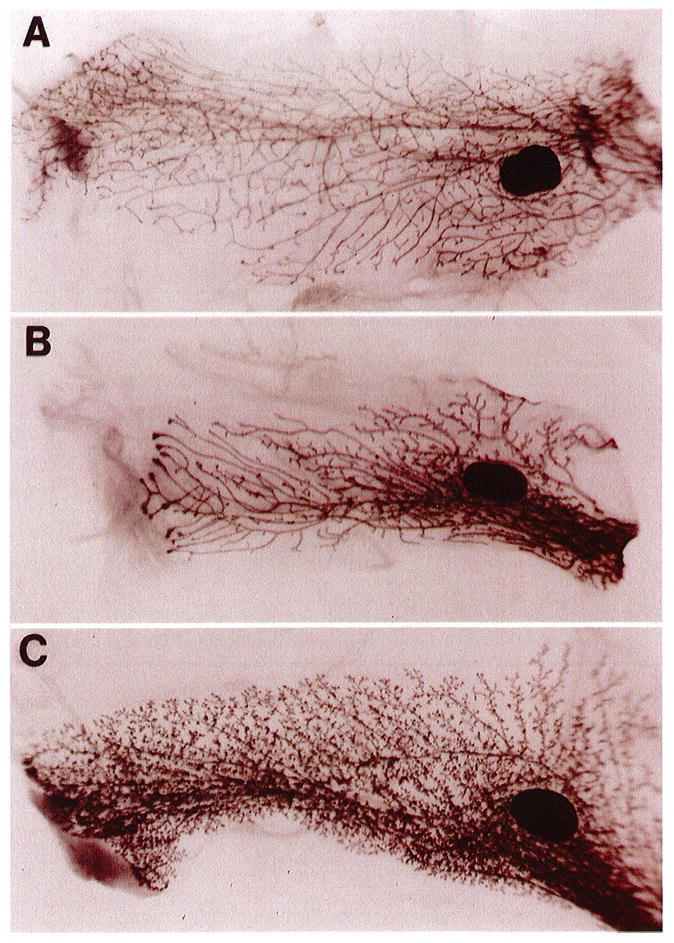
Phenotype of normal and transgenic mammary glands in virgin mice. Whole-mount carmine-stained glands from normal CD-1 mice: 70-day virgin (A), 50-day virgin transgenic (B), and 70-day virgin transgenic (C). The 70-day transgenic gland (C) is highly branched with prominent alveolar structures and closely resembles a normal 9–12-day pregnant gland. An intermediate phenotype can be captured at day 50 of virgin transgenic development (B). The dark-staining oval structures are the lymph nodes. Photographs are 6 × magnification. See Sympson et al, 1994, for details.
The mechanism by which SL-1 acts as a morphogen, leading to hyperbranching, proliferation and differentiation, has not been elucidated but is currently under investigation. We have hypothesized three possibilities for how the proteolytic action of SL-1 could directly or indirectly result in a proliferative response: (a) generation or activation of a growth stimulator; (b) destruction of a growth inhibitor; or (c) release of growth factors sequestered by the BM. It is known from our own studies that an intact BM is inhibitory for growth of both murine and human mammary epithelial cells. In rodent epithelial cells, it was shown that BM strongly down-regulates the expression of transforming growth factor (TGF)-(β and TGF-α.8,9 For normal human cells, which grow at the same rates as malignant cells on tissue culture plastic, embedding in BM will lead to a complete arrest of growth.10,11 Alternatively, the cleavage of BM molecules by ECM-degrading proteinases could unmask ECM molecules or generate fragments of ECM molecules that may in turn act as growth stimulators (for review see ref 12).
Proteinases appear to play a role in the branching morphogenesis of the salivary gland and kidney, as well as in capillary sprouting. Fukuda et al13 have shown that branching of the salivary gland organoids can be severely affected by the addition of exogenous collagenase, suggesting a role for matrix metal-loproteinases in this process. Madin-Darby canine kidney (MDCK) epithelial cells can undergo branching morphogenesis in three dimensional collagen or fibrin gels.14 This morphogenic effect can be inhibited by the addition of exogenous serine proteinase inhibitors and stimulated by hepatocyte growth factor (HGF), also known as scatter factor. It has been shown that recombinant human HGF causes a dramatic increase in both urokinase-type plasminogen activator (uPA) and its receptor.15
The formation of capillaries requires focal dissolution of the BM, followed by the migration of endothelial cells, which then form blood vessels upon canalization. Pepper et al16 found that capillary formation is dependent upon the balance between uPA and its inhibitor, PAI-1. Angiogenic factors, basic fibroblast growth factor (bFGF) and TGF-β1, stimulate this response by causing an increase in uPA expression. This proteinase activity may be focal, because endothelial cells have functional uPA receptors, and the number of these receptors also increases upon stimulation with bFGF.17,18 Using amnionic invasion assays, others have found that invasion of bFGF stimulated bovine endothelial cell invasion requires both plasmin and collagenase activity.19 To add to this ever-growing cascade of events, others have shown that TGF-β1-stimulated macrophages secrete increased levels of uPA, which in turn releases sequestered bFGF from the BM.20 In addition, BM-tethered TGF-β1 has been shown to be released by the action of proteinases.21 It can be deduced from these experiments, using a variety of model systems, that extracellular proteolysis is most probably required for branching morphogenesis.
As the SL-1 transgenic mice progress through the pregnancy stage of mammary gland development, many of the alveoli appear collapsed and surrounded by a thin layer of BM.7,22 This morphological phenotype is accompanied by a decrease in the expression of milk-specific genes, β-casein and whey acidic protein. Further characterization of the mid-pregnant gland has revealed that the mammary epithelial cells are undergoing programmed cell death, as measured by in-situ labelling of fragmented DNA.23 In-vitro evidence also suggests a role for an intact BM for cell survival and maintenance of tissue-specific morphology and function. These mice are capable of lactating even though the BM is severely disrupted and the alveoli are collapsed. Despite gross abnormalities during the growth and differentiation phases of the transgenic glands, the involution process appears to be normal.
We have now observed a dramatic incidence of tumors in four independent lines of these mice. All transgenic mice, thus far, display reactive stromas and phenotypic abnormalities ranging from severe hyperplasia, resembling a lactational phenotype, to adenocarcinomas (Figure 2). Hormonal stimulation does not appear to be absolutely necessary for tumor formation because tumors have developed in glands of virgin mice as young as four months old.
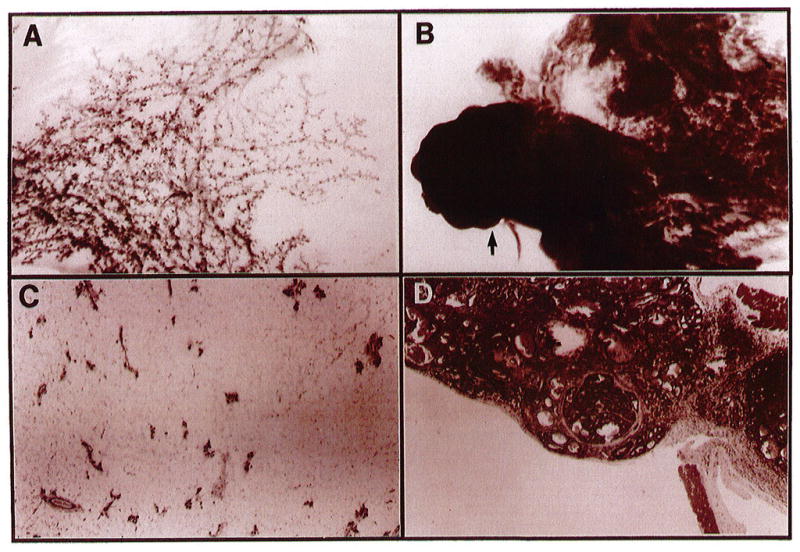
Morphology of mammary glands from normal and SL-1-overexpressing transgenic mice. Whole-mount carmine-stained glands from normal (A) and transgenic tumor-bearing (B) mice and the corresponding hematoxylin-eosin-stained cross sections, C and D, respectively. The arrow in panel B corresponds to the enlarged cross-section in panel D, which demonstrates an encapsulated adenocarcinoma. Both glands are the thoracic (nos 2/3) taken 4 months after the first lactation. Photographs in A and B are 6 × magnification and C and D are 40 × magnification.
The mechanism by which disruption of the BM leads to tumor formation is not yet known. It is clear from our culture studies that the ECM is a potent inhibitor of growth and an important regulator of cell survival and tissue-specific differentiation of mouse mammary epithelial cells. The signal transduction pathway by ECM is only now being unravelled.24 We have hypothesized that the massive disruption of the BM could lead to induction of growth factors, leading to rapid proliferation of the epithelial cells and thus to increased genetic errors. This process in turn could give rise to a select population of cells that have a growth advantage. The availability of this breast tumor model should lead to an elucidation of the early steps in the malignant process and to an understanding of how an abnormal microenvironment in epithelial tissues could lead to cancer induction and progression.
Acknowledgments
This work was supported by the U.S. Department of Energy, Office of Health and Environmental Research (contracts DE-AC03-76-SF01012 and DE-AC03-76-SF00098), and by a grant from the National Institutes of Health (NCI CA 57621).
References
Full text links
Read article at publisher's site: https://doi.org/10.1006/scbi.1995.0022
Read article for free, from open access legal sources, via Unpaywall:
https://europepmc.org/articles/pmc3815639?pdf=render
Citations & impact
Impact metrics
Citations of article over time
Smart citations by scite.ai
Explore citation contexts and check if this article has been
supported or disputed.
https://scite.ai/reports/10.1006/scbi.1995.0022
Article citations
Stromal-Modulated Epithelial-to-Mesenchymal Transition in Cancer Cells.
Biomolecules, 13(11):1604, 01 Nov 2023
Cited by: 3 articles | PMID: 38002286 | PMCID: PMC10669774
Review Free full text in Europe PMC
Matrix metalloproteinases as therapeutic targets in breast cancer.
Front Oncol, 12:1108695, 19 Jan 2023
Cited by: 14 articles | PMID: 36741729 | PMCID: PMC9897057
Review Free full text in Europe PMC
The not-so-sweet side of sugar: Influence of the microenvironment on the processes that unleash cancer.
Biochim Biophys Acta Mol Basis Dis, 1866(12):165960, 09 Sep 2020
Cited by: 2 articles | PMID: 32919034 | PMCID: PMC9489132
Review Free full text in Europe PMC
Inflammatory peroxidases promote breast cancer progression in mice via regulation of the tumour microenvironment.
Int J Oncol, 50(4):1191-1200, 20 Feb 2017
Cited by: 31 articles | PMID: 28260049
Therapeutic Potential of Matrix Metalloproteinase Inhibition in Breast Cancer.
J Cell Biochem, 118(11):3531-3548, 17 Jul 2017
Cited by: 68 articles | PMID: 28585723 | PMCID: PMC5621753
Review Free full text in Europe PMC
Go to all (65) article citations
Similar Articles
To arrive at the top five similar articles we use a word-weighted algorithm to compare words from the Title and Abstract of each citation.
Extracellular matrix remodeling as a regulator of stromal-epithelial interactions during mammary gland development, involution and carcinogenesis.
Braz J Med Biol Res, 29(9):1087-1097, 01 Sep 1996
Cited by: 31 articles | PMID: 9181050
Expression of stromelysin-1 and TIMP-1 in the involuting mammary gland and in early invasive tumors of the mouse.
Int J Cancer, 59(4):560-568, 01 Nov 1994
Cited by: 43 articles | PMID: 7960227
Matrix metalloproteinases are expressed during ductal and alveolar mammary morphogenesis, and misregulation of stromelysin-1 in transgenic mice induces unscheduled alveolar development.
Mol Biol Cell, 6(10):1287-1303, 01 Oct 1995
Cited by: 131 articles | PMID: 8573787 | PMCID: PMC301288
Extracellular matrix remodeling and the regulation of epithelial-stromal interactions during differentiation and involution.
Kidney Int Suppl, 54:S68-74, 01 May 1996
Cited by: 47 articles | PMID: 8731199 | PMCID: PMC2937007
Review Free full text in Europe PMC
Funding
Funders who supported this work.
NCI NIH HHS (3)
Grant ID: R01 CA057621
Grant ID: CA 57621
Grant ID: R01 CA057621-07