Abstract
Free full text

Fear of the unexpected: Hippocampus mediates novelty-induced return of extinguished fear in rats
Abstract
Several lines of evidence indicate an important role for the hippocampus in the recovery of fear memory after extinction. For example, hippocampal inactivation prevents the renewal of fear to an extinguished conditioned stimulus (CS) when it is presented outside the extinction context. Renewal of extinguished responding is accompanied by associative novelty (an unexpected occurrence of a familiar CS in a familiar place), the detection of which may require the hippocampus. We therefore examined whether the hippocampus also mediates the recovery of extinguished fear caused by other unexpected events, including presenting a familiar CS in a novel context or presenting a novel cue with the CS in a familiar context (e.g., external disinhibition). Rats underwent Pavlovian fear conditioning and extinction using an auditory CS and freezing behavior served as the index of conditioned fear. In Experiment 1, conditioned freezing to the extinguished CS was renewed in a novel context and this was eliminated by intra-hippocampal infusions of the GABAA agonist, muscimol, prior to the test. In Experiment 2, muscimol inactivation of the hippocampus reduced the external disinhibition of conditioned freezing that occurred when a novel white noise accompanied the extinguished tone CS. Collectively, these results suggest that the hippocampus mediates the return of fear when extinguished CSs are unexpected, or when unexpected stimuli accompany CS presentation. Ultimately, a violation of expectations about when, where, and with what other stimuli an extinguished CS will occur may form the basis of spontaneous recovery, renewal, and external disinhibition.
1. Introduction
A major challenge to behavioral therapies for anxiety disorders, including post-traumatic stress disorder, is the relapse of fear that occurs outside of the clinical context. One account of relapse builds on the observation that the extinction of Pavlovian conditioned responses (CRs), including fear responses, is context-specific (Bouton & Bolles, 1979; Bouton, Mineka, & Barlow, 2001; Craske et al., 2008). For example, if a conditional stimulus (CS) that once predicted an aversive footshock unconditional stimulus (US) is presented alone several times in a distinct context, fear to the CS will be lost—but only in the extinction context (Bouton & Bolles, 1979). That is, the fear CR will return or renew’ if the CS is encountered outside of the extinction context, including the conditioning context (‘ABA’ renewal, where the letters denote the contexts used for conditioning, extinction, and testing) or an equally familiar context in which the CS has never been experienced (‘AAB’ or ABC renewal’, which is often found to be weaker than ABA renewal). This reveals that extinction does not erase the CS-US association but encourages the formation of a new inhibitory CS- no US’ memory (Konorski, 1967; Pavlov, 1927). It has been posited that these inhibitory memories are particularly context-bound, and that animals use contexts as retrieval cues to determine how to respond to a CS (Bouton, 1993; Bouton, Westbrook, Corcoran, & Maren, 2006). By this view, inhibition acquired during extinction (e.g., the formation of a CS- no US’ association) is linked to the extinction context, and is not retrieved outside of that context. In other contexts, fear is supported by the context-independent expression of the CS-US association—as a result, conditioned fear is broadly generalized, whereas extinction is not.
Another possibility is that the inhibition acquired during extinction fails to generalize to other contexts because the circumstances of the test situation produce “inhibition of an inhibition” (Pavlov, 1927). Pavlov observed that novel or unexpected events (including his own presence during a colleague’s experiment) caused a spontaneous return of an extinguished salivary response, a phenomenon he termed external disinhibition’(Pavlov, 1927). The loss of inhibition that occurs when novel stimuli accompany an extinguished CS might account for other forms of CR recovery that are accompanied by unexpected events, including renewal. In this case, extinguished CSs are unexpectedly encountered in contexts in which they had never been presented (AAB, ABC), or had not been encountered recently (ABA). Hence, renewal may be a form of disinhibition produced by associative novelty when testing occurs in a familiar context. This account has found recent support in a computational model of extinction that includes attentional mechanisms and novelty in determining conditioned responding (Larrauri & Schmajuk, 2008).
Interestingly, there is considerable evidence in both rats and humans that the hippocampus plays an important role in novelty detection, particularly in the detection of associative novelty (Honey & Good, 2000; Knight, 1996; Kumaran & Maguire, 2006; Vinogradova, 2001). For example, hippocampal lesions in rats reduce the orienting response to the novel pairing of habituated stimuli, a phenomenon that requires the detection of an associative mismatch among familiar cues (Honey, Watt, & Good, 1998). In addition, human neuroimaging experiments have revealed that hippocampal activity is elevated under conditions in which unexpected sequences of familiar stimuli are encountered (Kumaran & Maguire, 2006; Wessel, Danielmeier, Morton, & Ullsperger, 2012). Moreover, it is now apparent that the hippocampus and its afferents are critical for the renewal of extinguished fear that occurs when an extinguished is unexpectedly encountered outside the extinction context (Corcoran, 2004; Corcoran & Maren, 2001; Corcoran, Desmond, Frey, & Maren, 2005; Hobin, Ji, & Maren, 2006; Ji & Maren, 2005; 2008a; 2008b; Maren & Hobin, 2007; Zelikowsky, Pham, & Fanselow, 2011). While most authors have interpreted these hippocampal lesion effects in terms of contextual retrieval processes and occasion setting (Maren, 2005; Maren & Holt, 2000), a more parsimonious account appeals to the role of the hippocampus in novelty detection. That is, the hippocampus may mediate the renewal effect, because it is detects associative novelty during the retrieval test that leads to the disinhibition of extinguished fear. If true, hippocampal inactivation should limit the return of extinguished fear that accompanies exposure to a novel stimulus (external disinhibition) or presentation of the extinguished CS in a novel context (renewal). To examine this question, we examined the effect of hippocampal inactivation with the GABAA agonist muscimol on the expression of fear to an extinguished CS presented in either a novel context (Experiment 1) or after delivery of a novel stimulus (white noise) in the extinction context (Experiment 2). Insofar as the hippocampus is required for detecting novel or unexpected events, we hypothesized that hippocampal inactivation would impair the return of fear driven by the presence of novel contexts or cues.
2. Materials and methods
2.1 Subjects
The subjects were 64 (Experiment 1) and 32 (Experiment 2) adult male Long-Evans rats (200 – 224 g) obtained from a commercial supplier (Harlan Sprague Dawley, Indianapolis, IN). After arrival, the rats were housed individually in Plexiglas hanging cages on a 14:10 hr light/dark cycle (lights on at 7:00 a.m.) and were allowed unlimited access to food and water. After being housed, the rats were handled (10 – 20 sec per rat per day) for 5 days to habituate them to the experimenter. All experiments were UCUCA-approved and conducted at the University of Michigan.
2.2 Surgical procedures
Rats underwent surgical procedures before any behavioral testing. Rats were treated with atropine methyl nitrate (0.3 mg/kg, i.p.), anesthetized with sodium pentobarbital (Nembutal; 65 mg/kg, i.p.), and mounted in a stereotaxic apparatus (Kopf Instruments, Tujunga, CA). The scalp was incised and retracted, and the head was positioned to place bregma and lambda in the same horizontal plane. Small holes were drilled through the skull for bilateral placement of stainless steel guide cannulas (23 gauge; 10 mm in length; Small Parts, Inc., Miami Lakes, FL) into the DH (3.8 mm posterior, 2.5 mm lateral, 2.5 mm ventral to bregma) and three jeweler’s screws. Cannulas were affixed to the skull and the scalp incision was closed with dental acrylic. After surgery, stainless steel obturators (30 gauge; 10 mm in length; Small Parts) were placed in the guide cannulas. Obturators were replaced every other day throughout the remainder of the experiment.
2.3 Behavioral apparatus
Eight identical observation chambers (30 × 24 × 21cm; MED-Associates, St. Albans, VT) were used in all of the experiments. The chambers were constructed from aluminum (side walls) and Plexiglas (rear wall, ceiling, and hinged front door) and were situated in sound-attenuating cabinets located in a brightly lit and isolated room. The floor of each chamber consisted of 19 stainless steel rods (4 mm in diameter) spaced 1.5 cm apart (center-to-center). Rods were wired to a shock source and solid-state grid scrambler (MED-Associates) for the delivery of footshock USs. A speaker mounted outside a grating in one wall of the chamber was used for the delivery of acoustic CSs. Video cameras mounted above each chamber were used to visualize the behavior of each rat, and each chamber rested on a load-cell platform that was used to detect locomotor activity and quantify freezing behavior.
Two contexts were used in these experiments. For one context, the overhead lights in the room and the houselights (15W) in each observation chamber were illuminated. The chambers were cleaned with a 2% acetic acid solution, and stainless steel pans containing a thin film of the same solution were placed underneath the grid floors to provide a distinct odor before the rats were placed inside. Ventilation fans in each cabinet supplied background noise (65 dB). Rats were transported to this context in white plastic boxes. For the second context, all room and chamber houselights were turned off; a pair of red lights (40W) provided illumination. Additionally, the doors on the sound-attenuating cabinets were closed, the ventilation fans were turned off, and the chambers were cleaned with a 1% ammonium hydroxide solution. To provide a distinct odor, stainless steel pans containing a thin film of this solution were placed underneath the grid floors before the rats were placed inside. Rats were transported to this context in black plastic boxes.
2.4 Behavioural procedure for Experiment 1
Rats were given one-week recovery after surgery, then submitted to three phases of training: fear conditioning, extinction, and retrieval testing. We used a two-context design (AAB or ABB; each letter refers to the context for the three phases of training); all contexts were counterbalanced. Unlike our previous designs of this nature (Corcoran, 2004), we did not familiarize the animals in the AAB condition with the B context prior to testing. In other words, we arranged the test context to be novel for the AAB animals, whereas it was familiar for the ABB animals insofar as it had hosted extinction for them.
For fear conditioning, rats were transported in squads of eight and placed in the conditioning chambers; chamber position was counterbalanced for each squad. The rats received five tone (10 s; 80 dB; 2 kHz)-footshock (1 s; 1 mA) trials (70 s intertrial interval, ITI) beginning 3 min after being placed in the chambers. Sixty seconds after the final shock, the rats were returned to their home cages.
The extinction phase began 24 hr after the conditioning session; it was conducted in a different context from conditioning. During each extinction session, rats were presented with 30 tone presentations (10 s; 80 dB; 2 kHz; 70 s ITI) without the footshock US, beginning 3 min after placement in the context. The extinction sessions were 38 min in duration, and a total 90 extinction trials were administered (30 trials/day for three consecutive days).
Twenty-four hours after the last extinction session, all rats received a retrieval test shortly after intracranial infusions of muscimol or saline. Twenty to twenty-five minutes after the infusions, the rats were returned back to the observation chambers and presented with 10 tones (10 s; 80 dB; 2 kHz; 70 s ITI), beginning 3 min after placing in the testing context. Testing occurred either in the extinction context (ABB; SAME’ condition) or in a novel context (AAB; NOVEL’ condition). The squads were counterbalanced for both infusions and test context. This yields a total of 4 groups in a 2 × 2 design (infusion X test context): SAL-SAME (n = 16), SAL-NOVEL (n = 16), MUS-SAME (n = 16), and MUS-NOVEL (n = 16).
2.5 Behavioral procedure for Experiment 2
The behavioral procedures for experiment two were identical to Experiment 1 with two exceptions: 1) all rats were conditioned, extinguished and tested with an ABB design and 2) half of the animals received a retrieval test in which each CS presentation was preceded by a novel white noise (10 s; 80 dB; 2 kHz; 80 s ITI). As before, the rats received either muscimol or saline infusions shortly before the test. This yielded a total of 4 groups in a 2 × 2 design (infusion X test condition): SAL-CS (n = 8), SAL-N+CS (n = 7), MUS-CS (n = 8), and MUS-N+CS (n = 7).
2.6 Microinfusions
Twenty to twenty-five min prior to this session, the rats (squads of four) were transported to an infusion room in opaque white plastic buckets with pine shavings covering their floors. Upon arrival in the infusion room, the obturators were removed from the rats’ guide cannulas. Stainless steel injection cannulas (30 gauge; 11 mm in length; Small Parts, Inc.), which were connected by polyethylene tubing (PE-20; Small Parts, Inc.) to 10 μL Hamilton syringes mounted in an infusion pump (Harvard Apparatus; South Natick, MA), were placed in the guide cannulas. Rats received an infusion of either sterile physiological saline (0.9%; SAL group) or muscimol (1 μg/μL dissolved in 0.9% sterile saline; Sigma, St. Louis, MO; MUS group) at a rate of 0.2 μL/min for 2.5 min. This resulted in a 0.5 μL infusion (i.e., 0.5 μg of muscimol per hemisphere). After the infusion, rats remained in the buckets with the injection cannulas in place for 1 min to allow for diffusion of the drug. The injection cannulas were removed, obturators were placed in the guide cannulas, and the rats were returned to their home cages until testing began. The infusion squads were counterbalanced for both testing context and infusion.
2.7 Histology
Histological verification of the lesions was performed after behavioral testing. Rats were perfused across the heart with physiological saline followed by a 10% formalin solution. After extraction from the skull, brains were post-fixed in 10% formalin solution for 2 days, at which time the solution was replaced with a 10% formalin/30% sucrose solution until sectioning. Sections (50 μm thick) were cut on a cryostat (−19 °C), wet-mounted on microscope slides, and stained with 0.25% thionin for visualization of the lesions.
2.8 Data analysis
We assessed fear by measuring freezing behavior as described previously (Maren, 1998). Freezing was quantified by computing the number of observations for each rat that had a value less than the freezing threshold (a load cell value below which animals are observed to be freezing) for each 1-minute block of the conditioning, extinction, and test sessions. Freezing for each CS trial was computed from the 1-min interstimulus interval after each CS. To avoid counting momentary inactivity as freezing, we only scored an observation as freezing if it fell within a continuous group of at least five observations that were all less than the freezing threshold. Thus, freezing was only scored if the rat was immobile for at least one second. For each session, the freezing data were transformed to a percentage of the total observations, a probability estimate that is amenable to analysis with parametric statistics. These probability estimates of freezing were analyzed using ANOVA. Post hoc comparisons in the form of Fisher’s PLSD tests were performed after a significant omnibus F-ratio. All data are represented as means ± SEMs.
3. Results
3.1 Histology
A representative cannula placement in the dorsal hippocampus is illustrated in Figure 1. Placements in both Experiments 1 and 2 were similar to those reported in our previously published work using this methodology. None of the rats in Experiment 1were excluded from analysis based on their cannula placements, and two rats were excluded in Experiment 2 because their cannula were placed ventral to the target.
3.2 Experiment 1
Conditioned freezing during the conditioning, extinction, and test sessions is shown in Figure 2. On the conditioning day, freezing behavior in the 1-min block after each trial increased significantly across the five conditioning trials (F (7,420) = 312.4, p < 0.0001); there were no differences between the groups. For the extinction sessions, freezing was averaged across all 30 post-CS blocks (1-min blocks) and plotted for each of the three extinction sessions, which were conducted over three days. Conditioned freezing declined significantly across the extinction sessions (F (2,120) = 133.2, p < 0.0001). Although the terminal level of fear suppression was similar in all groups, the context in which rats were extinguished produced significantly different levels of fear (main effect of context, F (1,60) = 23.5, p < 0.0001) and influenced extinction rate (context x day interaction, F (2,120) = 6.2, p < 0.005). Animals that were extinguished in the conditioning context (here designated as Novel’ groups, because of their ultimate test context in theAAB procedure) exhibited higher levels of freezing than those extinguished in a novel context (here designated as Same’ groups’, because of their ultimate test context in the ABB procedure). This difference in the contextual specificity of conditioning expressed during the first extinction session was unexpected, as we have previously observed similar levels of conditioned freezing in the two contexts (Corcoran, 2004; Corcoran et al., 2005; Corcoran & Maren, 2001). Nonetheless, all animals reached a similar level of freezing at the end of the extinction phase.
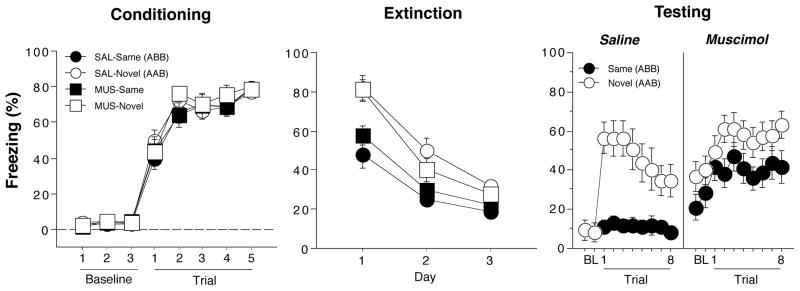
Hippocampal inactivation disrupts renewal of fear in a novel context (Experiment 1). (Left panel) Mean (±SEM) percentage of freezing on the conditioning day. Data are 1-min averages for a 3-min baseline period (BL) and after each of the five tone-shock conditioning trials. (Middle panel) Freezing behavior during the extinction sessions. Freezing was averaged across the 30-trials extinction session. Extinction was conducted in the conditioning context for the ‘Novel’ groups (AAB design) and a novel context for the ‘Same’ groups (ABB design). Hence, the group labels refer to the treatment conditions that would later be imposed during retrieval testing (not during conditioning or extinction). (Right panel) Freezing behavior during the retrieval test. Data are 1-min averages for a 2-min baseline period (BL) and after each of the eight CS-alone trials. Rats were tested in either the extinction context (SAME) or in a novel context (NOVEL).
After extinction, rats were infused with muscimol or saline and tested either in the extinction context (‘Same’ groups) or in a novel context (‘Novel’ groups). As shown in the last panel of Figure 2, saline-treated rats exhibited a robust renewal of fear to the extinguished CS when tested in the novel context. Interestingly, this effect was eliminated in rats receiving muscimol infusions into the DH. A comparison of CS-evoked freezing (an average of freezing during the 8 post-CS intervals minus the pre-CS baseline) supported this conclusion (Figure 3). An ANOVA with variables of context and drug revealed significant main effects of context (F (1,60) = 12.4, p < 0.005), drug (F(1,60) = 4.3, p < 0.05), and a significant interaction of the two variables (F (1,60) = 10.7, p < 0.002). The significant interaction reveals that saline-treated rats exhibited renewal of fear in the novel context, whereas muscimol-treated rats did not. It should be noted that in this experiment, muscimol elevated baseline (pre-CS) freezing. We have not observed this in other published work (Corcoran, 2004; Corcoran et al., 2005; Corcoran & Maren, 2001), including Experiment 2. We do not believe, however, that the shifted baseline mitigated the renewal effect insofar as there was ample room for muscimol-treated rats to increase freezing behavior to the CS. It should also be noted that this design confounds context familiarity with context change; that is, animals in the NOVEL condition experience both a novel context and a change in context in between the extinction and retention testing. However, we have previously observed that context change alone is not sufficient for fear renewal in within-subjects designs in which animals maintain extinguished responding despite context shifts (Hobin, Goosens, & Maren, 2003).
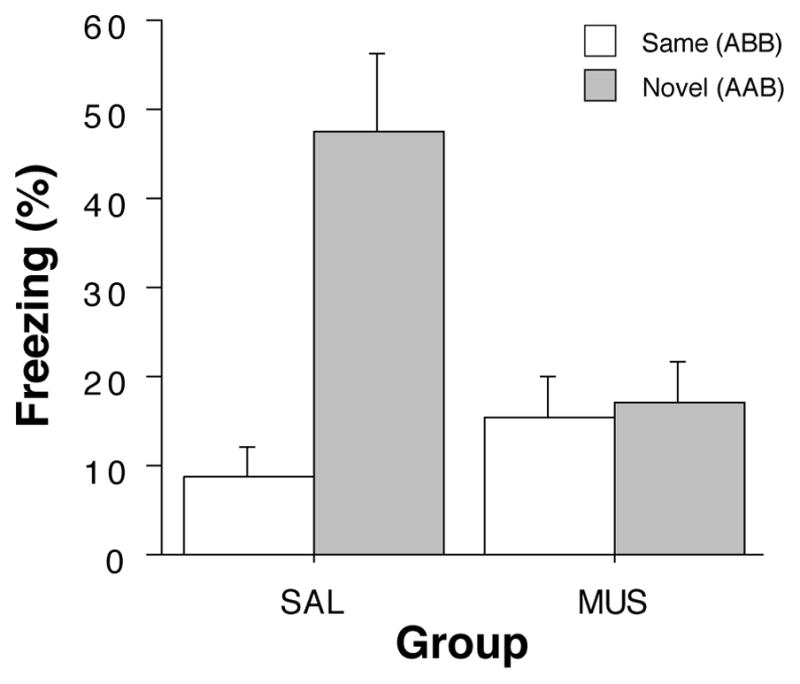
Hippocampal inactivation disrupts renewal of fear in a novel context (Experiment 1). Mean (±SEM) percentage of freezing during the retrieval test. Data are averages of eight post-CS blocks minus the average of the pre-CS baseline (as shown in the last panel of Figure 2). Rats were tested in either the extinction context (SAME; open bars) or in a novel context (NOVEL; gray bars). Dorsal hippocampal inactivation with muscimol (MUS) impaired the renewal of conditioned freezing to the CS in a novel context compared to saline-treated (SAL) rats.
3.3 Experiment 2
The procedures for Experiment 2 were similar to those of Experiment 1, except that all animals were conditioned, extinguished and, tested using an ABB procedure. We used this procedure reduce the contribution of contextual fear to our assessment of cue-evoked fear during the test. Conditioned freezing during the conditioning, extinction, and test sessions is shown in Figure 4. On the conditioning day, freezing behavior in the 1-min block after each trial increased significantly across the five conditioning trials (F(7,175) = 153.8, p < 0.0001); there were no differences between the groups. For the extinction sessions, freezing was averaged across all 30 post-CS blocks (1-min interstimulus intervals after each CS) and plotted for each of the three extinction sessions, which were conducted over three days. Conditioned freezing declined significantly across the extinction sessions (F (2,50) = 72.5, p < 0.0001). All animals reached a similar level of freezing at the end of the extinction phase.
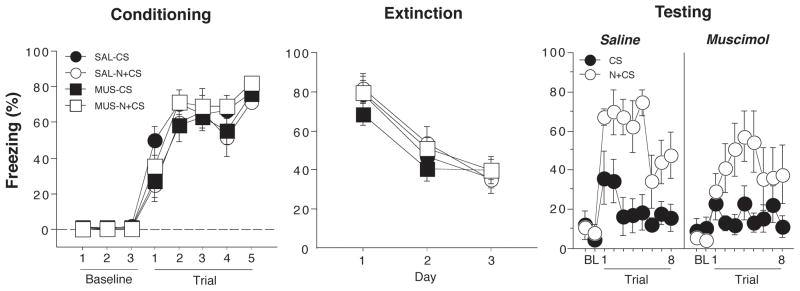
Hippocampal inactivation disrupts renewal of fear by a novel white noise (Experiment 2). (Left panel) Mean (±SEM) percentage of freezing on the conditioning day. Data are 1-min averages for a 3-min baseline period (BL) and after each of the five tone-shock conditioning trials. (Middle panel) Freezing behavior during the extinction sessions. Freezing was averaged across all 30 trials of each extinction session. Extinction was conducted in a novel context for the all groups (ABB design). The group labels refer to the treatment conditions that would later be imposed during retrieval testing (not during conditioning or extinction). (Right panel) Freezing behavior during the retrieval test. Data are 1-min averages for the 2-min baseline period (BL) and after each of the eight CS-alone trials. Rats were tested in the extinction context and the CS was either preceded on each trial by a novel white noise (N+CS) or was presented alone (CS).
After extinction, rats were infused with muscimol or saline and tested in the extinction context. In half of the animals, each CS test trial was immediately preceded by a novel white noise (N+CS), whereas the other half of the animals received the CS alone (CS). As shown in the last panel of Figure 4, presentation of the novel white noise greatly increased fear to the CS in saline-treated rats as indicated by increased freezing in the post-CS blocks (1-min blocks) in CS+N relative to CS groups. Interestingly, this effect was also apparent in rats receiving muscimol infusions into the DH. A comparison of CS-evoked freezing (an average of freezing during the 8 post-CS intervals minus the pre-CS baseline) supported this conclusion (Figure 5A). An ANOVA with variables of cue and drug revealed a significant main effect of cue (F (1,25) = 27.3, p < 0.0001, a non-significant main effect of drug and a non-significant drug x cue interaction (Fs < 1.6). This indicates that over the duration of the test, the novel white noise augmented conditioned freezing to the CS relative to the CS alone.
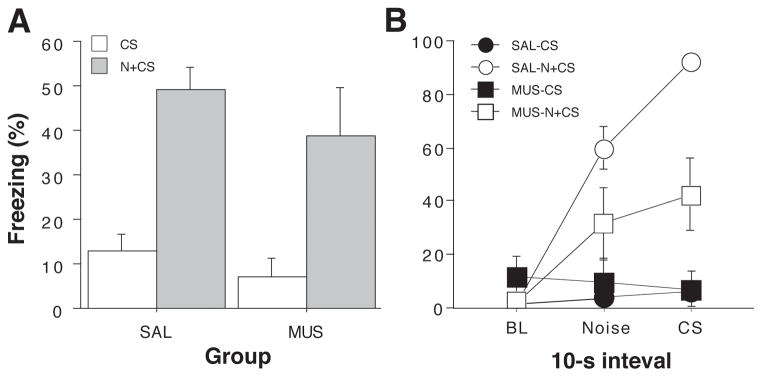
Hippocampal inactivation disrupts renewal of fear in a novel context (Experiment 1). (A) Mean (±SEM) percentage of freezing during the retrieval test. Data are averages of eight post-CS blocks minus the average of the pre-CS baseline (as shown in the right panel of Figure 4). Rats were tested in the extinction context and the CS was either preceded on each trial by a novel white noise (N+CS) or was presented alone (CS). Dorsal hippocampal inactivation with muscimol (MUS) did not impair the renewal of conditioned freezing by a novel stimulus. (B) Mean (±SEM) percentage of freezing during first test trial. Freezing data are 10-sec averages for the pre-trial period, the white noise stimulus, and the tone CS (an equivalent stimulus-free period is shown for CS rats that did not receive white noise presentations). Hippocampal muscimol infusions attenuated the renewal of fear elicited by the novel white noise on the first test trial.
However, close inspection of the test data in Figure 4 reveals that muscimol-treated rats exhibited a transient impairment in the noise-induced augmentation of freezing behavior during the first post-CS period. To understand this effect more completely, we performed an analysis of freezing behavior during 10-sec intervals immediately before the trial (baseline), during the white noise stimulus in the subsequent 10-sec interval, and during the first CS. For animals in the CS groups that did not receive white noise, we used the 10-sec stimulus free periods that corresponded to the interval sampled during the noise in the N+CS groups. These data are shown in Figure 5B. Saline-treated rats exhibited low levels of freezing before the first trial, and responded to the novel noise and consequent CS with robust freezing behavior. Freezing to the CS was substantially greater than that to the noise, although whether this freezing reflects fear to the CS, the preceding noise, or both cannot be determined with this design. Moreover, it is possible that generalized fear to the noise augments freezing to the CS, an effect that might occur along with disinhibition. Notably, muscimol-treated rats exhibited a much smaller increase in freezing after presentation of the novel white noise, and fear did not increment when the subsequent CS was delivered. Animals that did not receive white noise stimuli exhibited low levels of freezing during each of these intervals. These observations were confirmed in repeated measures ANOVA with between-subjects variables of drug and cue, and a within-subjects variable of interval. This analysis revealed a main effect of cue (F (1,25)= 28.5, p < 0.0001), a significant drug x cue interaction (F (1,25) = 7.0, p < 0.02), and a significant three-way interaction (F (2, 50) = 4.1, p < 0.05). Hence, muscimol inactivation of the dorsal hippocampus impaired the disnhibition of freezing to an extinguished CS by a novel white noise, although this effect was transient, lasting only a single trial. This pattern of deficit is not entirely unexpected, particularly because renewal phenomena can be short-lived and therefore may be most sensitive to disruption early in retrieval testing (but see (Zelikowsky et al., 2011). It is also conceivable that muscimol inactivation of the hippocampus disrupted generalized fear to the noise that may have promoted consequent CS freezing.
4. Discussion
The present results reveal that inactivation of the dorsal hippocampus attenuates the return of fear to an extinguished CS in a novel context (Experiment 1) and when the CS is accompanied by a novel stimulus in the extinction context (Experiment 2). Interestingly, the hippocampal impairment differed in severity in the two experiments, and was relatively transient when a novel stimulus renewed fear. Together, these results add to the growing list of studies pointing to an important role of the hippocampus in regulating the expression of fear extinction. Moreover, the present results suggest that the role of the hippocampus in regulating fear after extinction might be understood in terms of mnemonic functions that are used in predicting future events based upon past experience (Buckner, 2010; Cohen & Eichenbaum, 1995; Honey & Good, 2000). Here we propose that the hippocampus is essential for detecting unexpected events, and unexpected events, at least in the present case, restore conditioned fear to an extinguished CS.
The first idea in this proposition—that the hippocampus is essential for detecting unexpected events—was first proposed by Vinogradova, who pioneered studies of hippocampal single-unit responses during orienting responses to novel stimuli (Vinogradova, 2001). Orienting responses are driven by the absence of information about a stimulus in memory (Sokolov, 1963), and Vinogradova argued that detecting novel stimuli rests on recognition memory processes mediated by the hippocampus. In addition to detecting stimulus novelty, the hippocampus is also widely believed to detect novel arrangements of familiar stimuli, so-called “associative novelty” as well as familiar stimuli in novel places (“spatial novelty”) (Gabriel, Sparenborg, & Stolar, 1987; Honey et al., 1998; Jeewajee, Lever, Burton, O’Keefe, & Burgess, 2008; Kaye & Pearce, 1984; Kumaran & Maguire, 2006). For example, Honey and colleagues have shown that hippocampal lesions in rats prevented orienting responses to familiar and physically identical visual cues whose relationship to a familiar auditory signal had changed (Honey et al., 1998). Interestingly, hippocampal damage did not affect orienting responses to stimulus novelty per se, or mismatches in which the spatial and temporal properties of the stimulus changed; hippocampal lesions appeared to have a selective role in associative novelty.
In the behavioral experiments reported here, a familiar, extinguished CS is presented in a novel place (Experiment 1) or after a novel cue in a familiar place (Experiment 2). We found that control rats exhibited a return of conditioned responding to the CS in both cases. In the first case, the return of fear might be understood as an example of “renewal”, in which fear returns outside of the extinction context. Current theories of renewal (Bouton, 1993; Pearce & Bouton, 2001) posit that the extinction context possesses occasion setting properties that modulate the response to the extinguished CS presumably by activating an inhibitory CS- no US’ association learned anew in that context (Konorski, 1967; Pavlov, 1927). Outside of that context, conditioned responding to the CS returns because the CS-US memory is not context bound, although the conditioning memory may become more context-bound after extinction (Harris, Jones, Bailey, & Westbrook, 2000). In essence, this view assumes that the renewal of fear results from the failure of the current context (whether novel or familiar) to retrieve the inhibitory extinction memory. Lesions or inactivation of the hippocampus or its afferents prevent renewal of conditioned fear (Corcoran, 2004; Corcoran et al., 2005; Corcoran & Maren, 2001; Hobin et al., 2006; Ji & Maren, 2005; 2008a; Orsini, Kim, Knapska, & Maren, 2011), an effect we have attributed to a loss of contextual control of the inhibitory extinction memory (Maren, 2005; 2011; Maren & Quirk, 2004; Orsini & Maren, 2012). In other words, performance in animals with hippocampal damage appears to reflect the sum of the associative strengths of the excitatory CS-US and inhibitory CS- no US’ memories, which might be anticipated by theories positing an important role for the hippocampus in indexing associations to the contexts in which they occur (Hirsh, 1974; Maren & Holt, 2000). Consistent with this perspective, there is evidence that negative occasion setting requires the hippocampus (Holland, Lamoureux, Han, & Gallagher, 1999), although not all forms of occasion setting are hippocampal-dependent (Holland & Bouton, 1999; Yoon, Graham, & Kim, 2011).
Despite the substantial evidence for the involvement of occasion setting in extinction, a more parsimonious account for renewal effects and their hippocampal dependence may be found in Pavlov’s original account of extinction as a loss of CS processing that can be recovered by novel events (Pavlov, 1927; Robbins, 1990). As Pavlov first described, novel stimuli result in an external disinhibition of extinguished responding, which he attributed to an “inhibition of an inhibition” (Pavlov, 1927). By this view, extinction memories are context-specific not because the extinction context has a special role in retrieving the inhibitory CS- no US’ association, but because those associations are easily disrupted by unexpected events including unexpected events in the extinction context).
The possibility that extinction involves attentional decrements to the CS that could limit conditioned responding has received considerable support in the literature (Delamater, 2004; Kaye & Pearce, 1984; 1987; Kehoe & White, 2002; Pavlov, 1927; Robbins, 1990). For example, Robbins (1990) found pronounced declines in attention to extinguished stimuli that could be dissociated from their occasion setting properties, and dissipation of the attentional effects over time accounted for spontaneous recovery (Robbins, 1990). It is conceivable then that other factors that restore attention to an extinguished CS, including stimulus or associative novelty, might promote conditioned responding (Larrauri & Schmajuk, 2008). Insofar as loss of attention to an extinguished CS is driven by an habituation process (Chang & Maren, 2009; Kamprath & Wotjak, 2004; McSweeney & Swindell, 2002; Plendl & Wotjak, 2010)(e.g., habituation of the orienting response), novel stimuli or contexts may lead to dishabituation and restore conditioned responding. Bouton and Ricker (1994) examined this issue and considered whether dishabituation might account for renewal in a conditioned suppression procedure in which orienting responses to the CS have the opportunity to compete with lever pressing and masquerade as renewed conditioned suppression (Bouton & Ricker, 1994). They reasoned that if dishabituation of the orienting response accounted for lever press suppression, that it should have the opposite effect (impairing renewal) in an appetitive procedure in which an orienting response would prevent (not mimic) the CR. However, they found that a context-shift that would be expected to dishabituate orienting responses to the CS still produced robust renewal in an appetitive procedure. That said, the response systems mediating conditioned suppression generate behavior that far outlasts the orienting response. A contextual change or novel stimulus that increments attention to the CS may therefore increase conditioned responding to that stimulus by increasing attention to the CS independent of response competition or compatibility (Larrauri & Schmajuk, 2008; Schmajuk, Gray, & Lam, 1996).
The neural mechanism by which novelty might inhibit the expression or retrieval of extinction memories is not understood. However, if the hippocampus is essential for detecting unexpected events, then hippocampal inactivation would be expected to eliminate the return of fear experienced by presenting an extinguished CS in a novel context (Experiment 1), presenting a novel stimulus along with an extinguished CS in the extinction context (Experiment 2), presenting an extinguished CS in a familiar context in which it has never been encountered (AAB or ABC renewal) (Corcoran, 2004; Corcoran & Maren, 2001), or even presenting the extinguished CS in the original conditioning context (Ji & Maren, 2005). All of these outcomes have been confirmed. Interestingly, hippocampal deficits in fear renewal are most pronounced when an extinguished CS is presented in a novel context or in another context in which it has not been presented, both of which engender associative novelty. Consistent with earlier work, this implies that the hippocampus has a weaker role in detecting (or acting upon) stimulus novelty per se (Honey et al., 1998). One might question whether experiencing an extinguished CS in the conditioning context (as in ABA renewal) should be regarded as an unexpected event’ given that the animal has experienced conditioning in that context. However, proper ABA renewal designs extinguish fear of the conditioning context prior to testing to allow for an unambiguous interpretation of CS renewal in that context. Hence, the reoccurrence of the CS in the conditioning context after extensive exposure to that context in the absence of the CS would surely be unexpected. One can extend the same logic to experiencing the extinguished CS in the conditioning context after a long delay, which, by this view, is an unexpected event that yields spontaneous recovery.
Associative learning models (Rescorla & Wagner, 1972) (Mackintosh, 1975; Pearce & Hall, 1980) have considerable explanatory power in accounting for a variety of associative learning phenomena, yet, in their original form, they fall short in fully accounting for the context-dependence of extinction. Elaborations of these models that introduce context-CS associations or configural representations of elemental and configural stimuli begin to capture some elements of the context-dependence of extinction memory (Pearce, 1994; Wagner, Spear, & Miller, 1981), but ultimately resort to those configurations guiding performance through direct associations with the US. In contrast, it has been argued that direct context-US associations or configural (context-CS)-US associations are not essential for the contextual control of extinguished CSs (Bouton, 2004). Recently, there has been interest in understanding conditioning processes from a non-associative perspective that considers how animals make inferences about the likelihood of events (or the likelihood of an outcome given a particular response) given their past experience. This approach has roots in Krechevsky’s work on hypothesis testing’ (Krechevsky, 1932), and has been formalized in temporal accounts of conditioning (Gallistel & Gibbon, 2000) and so-called latent cause theory (Courville, Daw, & Touretzky, 2006; Gershman & Niv, 2012). In the latter case, computational models have been developed that attempt to capture how animals act upon latent causes’ that may be hidden from observation (Gershman, Blei, & Niv, 2010). From this perspective, animals gather statistical information about the probability of events in their world, and then use this information to make inferences in novel situations (e.g., when a CS occurs in a context in which it has never been presented, such as ABC renewal). This approach has had some success in explaining the context-dependence of extinction (Gershman et al., 2010).
From a functional behavioristic perspective, natural selection may have favored psychological and cognitive systems in which aversive experiences establish latent causes for an event (e.g., the environmental context, the cue, the experimenter, transport cues, the time of day), and this myriad of causes promotes fear that broadly generalizes to other cues and contexts. Extinction may represent a narrowing of these latent causes, establishing a unique observation in which a CS is known to be safe in a particular context. Unexpected events, such as the presence of a novel stimulus or the occurrence of an extinguished CS in an unfamiliar context or indeed the unexpected occurrence of an extinguished CS in a familiar context, challenge these narrowed causes. The immediate response in the face of this expectancy violation may be to engage defensive behaviors; at least until additional extinction trials occur—indeed, once bitten, twice shy.
The present data suggest that the hippocampus is important for novelty-induced renewal of fear, which is consistent with its long-standing role in memory processes (Cohen & Eichenbaum, 1995; Fanselow, 2010) and novelty detection (Honey & Good, 2000; Vinogradova, 2001). There is emerging evidence that the hippocampus mediates renewal effects via connections with the amygdala and prefrontal cortex (Herry et al., 2008; Knapska & Maren, 2009; Knapska et al., 2012; Maren, 2011; Orsini & Maren, 2012). To restore conditioned fear after extinction, the hippocampus might either inhibit the expression of the extinction memory (“inhibition of an inhibition”) or promote attention to the CS or expression of the fear memory, allowing the return of fear. Increases in Fos expression in brain areas associated with fear expression, including the prelimbic cortex and lateral and central amygdaloid nuclei, are consistent with the latter possibility (Knapska et al., 2012; Knapska & Maren, 2009). Yet it remains possible that hippocampal inhibition of infralimbic cortical circuits involved in the expression of extinction are also involved in renewal, and further work is required to determine how prefrontal cortical circuits are regulated by associative novelty.
In sum, novel events challenge animals’ expectations about what is intended to happen in a given situation, and when expectations are violated behavior changes to anticipate alternative outcomes—freezing behavior in the present case. Violations in expectancy produced by novel stimuli, associative novelty, or prediction errors (e.g., unexpected reinforcers) may not only support new learning (Huh et al., 2009; Kamin, 1967; Mcnally, Johansen, & Blair, 2011), but also influence the expression of what has already been learned. In the present work, we now show that the hippocampus is critical for either detecting or acting upon novel information that restores extinguished conditioned responding. This has important implications for how fear recovery phenomena and their neural substrates are understood.
Acknowledgments
This research was supported by a grant from the NIMH (R01065961) to SM. The authors would like to thank Jinzhao Ji and April Qian for assisting with data collection.
Footnotes
Publisher's Disclaimer: This is a PDF file of an unedited manuscript that has been accepted for publication. As a service to our customers we are providing this early version of the manuscript. The manuscript will undergo copyediting, typesetting, and review of the resulting proof before it is published in its final citable form. Please note that during the production process errors may be discovered which could affect the content, and all legal disclaimers that apply to the journal pertain.
References
- Bouton M. Context, time, and memory retrieval in the interference paradigms of Pavlovian learning. Psychological Bulletin. 1993;114(1):80–99. [Abstract] [Google Scholar]
- Bouton ME. Context and Behavioral Processes in Extinction. Learning & memory (Cold Spring Harbor, NY) 2004;11(5):485–494. 10.1101/lm.78804. [Abstract] [CrossRef] [Google Scholar]
- Bouton ME, Westbrook RF, Corcoran KA, Maren S. Contextual and temporal modulation of extinction: behavioral and biological mechanisms. Biological psychiatry. 2006;60(4):352–360. 10.1016/j.biopsych.2005.12.015. [Abstract] [CrossRef] [Google Scholar]
- Bouton M, Bolles R. Contextual control of the extinction of conditioned fear. Learning and Motivation. 1979;10(4):445–466. [Google Scholar]
- Bouton M, Ricker S. Renewal of extinguished responding in a second context. Animal Learning and Behavior. 1994;22(3):317–324. [Google Scholar]
- Bouton M, Mineka S, Barlow D. A modern learning theory perspective on the etiology of panic disorder. Psychological Review. 2001;108(1):4–32. [Abstract] [Google Scholar]
- Buckner RL. The role of the hippocampus in prediction and imagination. Annual Review of Psychology. 2010;61(1):27–48. 10.1146/annurev.psych.60.110707.163508. [Abstract] [CrossRef] [Google Scholar]
- Chang CH, Maren S. Early extinction after fear conditioning yields a context-independent and short-term suppression of conditional freezing in rats. Learning & Memory. 2009;16(1):62–68. 10.1101/lm.1085009. [Europe PMC free article] [Abstract] [CrossRef] [Google Scholar]
- Cohen NJ, Eichenbaum H. Memory, Amnesia, and the Hippocampal System. The MIT Press; 1995. [Google Scholar]
- Corcoran KA. Factors regulating the effects of hippocampal inactivation on renewal of conditional fear after extinction. Learning & Memory. 2004;11(5):598–603. 10.1101/lm.78704. [Europe PMC free article] [Abstract] [CrossRef] [Google Scholar]
- Corcoran KA, Maren S. Hippocampal inactivation disrupts contextual retrieval of fear memory after extinction. The Journal of neuroscience : the official journal of the Society for Neuroscience. 2001;21(5):1720–1726. [Europe PMC free article] [Abstract] [Google Scholar]
- Corcoran KA, Desmond TJ, Frey KA, Maren S. Hippocampal inactivation disrupts the acquisition and contextual encoding of fear extinction. The Journal of neuroscience : the official journal of the Society for Neuroscience. 2005;25(39):8978–8987. 10.1523/JNEUROSCI.2246-05.2005. [Europe PMC free article] [Abstract] [CrossRef] [Google Scholar]
- Courville AC, Daw ND, Touretzky DS. Bayesian theories of conditioning in a changing world. Trends In Cognitive Sciences. 2006;10(7):294–300. [Abstract] [Google Scholar]
- Craske MG, Kircanski K, Zelikowsky M, Mystkowski J, Chowdhury N, Baker A. Optimizing inhibitory learning during exposure therapy. Behaviour research and therapy. 2008;46(1):5–27. 10.1016/j.brat.2007.10.003. [Abstract] [CrossRef] [Google Scholar]
- Delamater AR. Experimental extinction in Pavlovian conditioning: Behavioural and neuroscience perspectives. Quarterly Journal of Experimental Psychology Section B. 2004;57(2):97–132. [Abstract] [Google Scholar]
- Fanselow MS. From contextual fear to a dynamic view of memory systems. Trends In Cognitive Sciences. 2010;14(1):7–15. 10.1016/j.tics.2009.10.008. [Europe PMC free article] [Abstract] [CrossRef] [Google Scholar]
- Gabriel M, Sparenborg S, Stolar N. Hippocampal control of cingulate cortical and anterior thalamic information processing during learning in rabbits. Experimental Brain Research. 1987;67(1):131–152. [Abstract] [Google Scholar]
- Gallistel CR, Gibbon J. Time, rate, and conditioning. Psychological Review. 2000;107(2):289–344. [Abstract] [Google Scholar]
- Gershman SJ, Niv Y. Exploring a latent cause theory of classical conditioning - Springer. Learning & behavior 2012 [Abstract] [Google Scholar]
- Gershman SJ, Blei DM, Niv Y. Context, learning, and extinction. Psychological Review. 2010;117(1):197–209. 10.1037/a0017808. [Abstract] [CrossRef] [Google Scholar]
- Harris J, Jones M, Bailey G, Westbrook R. Contextual control over conditioned responding in an extinction paradigm. Journal of Experimental Psychology: Animal Behavior Processes. 2000;26(2):174–185. 10.1037//0097-7403.26.2.174. [Abstract] [CrossRef] [Google Scholar]
- Herry C, Ciocchi S, Senn V, Demmou L, Müller C, Lüthi A. Switching on and off fear by distinct neuronal circuits. Nature. 2008;454(7204):600–606. 10.1038/nature07166. [Abstract] [CrossRef] [Google Scholar]
- Hirsh R. The hippocampus and contextual retrieval of information from memory: a theory. Behavioral Biology. 1974;12(4):421–444. [Abstract] [Google Scholar]
- Hobin JA, Goosens KA, Maren S. Context-dependent neuronal activity in the lateral amygdala represents fear memories after extinction. Journal of Neuroscience. 2003;23(23):8410–8416. [Europe PMC free article] [Abstract] [Google Scholar]
- Hobin JA, Ji J, Maren S. Ventral hippocampal muscimol disrupts context-specific fear memory retrieval after extinction in rats. Hippocampus. 2006;16(2):174–182. 10.1002/hipo.20144. [Abstract] [CrossRef] [Google Scholar]
- Holland P, Bouton M. Hippocampus and context in classical conditioning. Current opinion in neurobiology. 1999;9(2):195–202. [Abstract] [Google Scholar]
- Holland P, Lamoureux J, Han J, Gallagher M. Hippocampal lesions interfere with Pavlovian negative occasion setting. Hippocampus. 1999;9(2):143–157. [Abstract] [Google Scholar]
- Honey RC, Good M. Associative components of recognition memory. Current opinion in neurobiology. 2000;10(2):200–204. [Abstract] [Google Scholar]
- Honey RC, Watt A, Good M. Hippocampal lesions disrupt an associative mismatch process. Journal of Neuroscience. 1998;18(6):2226–2230. [Europe PMC free article] [Abstract] [Google Scholar]
- Huh K, Guzman Y, Tronson N, Guedea A, Gao C, Radulovic J. Hippocampal Erk mechanisms linking prediction error to fear extinction: Roles of shock expectancy and contextual aversive valence. Learning & Memory. 2009;16(4):273–278. 10.1101/Lm.1240109. [Europe PMC free article] [Abstract] [CrossRef] [Google Scholar]
- Jeewajee A, Lever C, Burton S, O’Keefe J, Burgess N. Environmental novelty is signaled by reduction of the hippocampal theta frequency. Hippocampus. 2008;18 (4):340–348. 10.1002/hipo.20394. [Europe PMC free article] [Abstract] [CrossRef] [Google Scholar]
- Ji J, Maren S. Electrolytic lesions of the dorsal hippocampus disrupt renewal of conditional fear after extinction. Learning & memory (Cold Spring Harbor, NY) 2005;12 (3):270–276. 10.1101/lm.91705. [Europe PMC free article] [Abstract] [CrossRef] [Google Scholar]
- Ji J, Maren S. Differential roles for hippocampal areas CA1 and CA3 in the contextual encoding and retrieval of extinguished fear. Learning & memory (Cold Spring Harbor, NY) 2008a;15(4):244–251. 10.1101/lm.794808. [Europe PMC free article] [Abstract] [CrossRef] [Google Scholar]
- Ji J, Maren S. Lesions of the entorhinal cortex or fornix disrupt the context-dependence of fear extinction in rats. Behavioural brain research. 2008b;194(2):201–206. 10.1016/j.bbr.2008.07.011. [Europe PMC free article] [Abstract] [CrossRef] [Google Scholar]
- Kamin LJ. Predictability, surprise, attention, and conditioning. Presented at the Symposium on Punishment; Princeton, NJ. 1967. [Google Scholar]
- Kamprath K, Wotjak C. Nonassociative learning processes determine expression and extinction of conditioned fear in mice. Learning & memory (Cold Spring Harbor, NY) 2004;11(6):770–786. [Europe PMC free article] [Abstract] [Google Scholar]
- Kaye H, Pearce J. The strength of the orienting response during Pavlovian conditioning. Journal of Experimental Psychology: Animal Behavior Processes. 1984;10(1):90–109. [Abstract] [Google Scholar]
- Kaye H, Pearce JM. Hippocampal lesions attenuate latent inhibition and the decline of the orienting response in rats. The Quarterly Journal of Experimental … 1987;39(2-B):107–125. [Abstract] [Google Scholar]
- Kehoe EJ, White NE. Extinction revisited: Similarities between extinction and reductions in US intensity in classical conditioning of the rabbit’s nictitating membrane response. Learning & behavior. 2002;30(2):96–111. [Abstract] [Google Scholar]
- Knapska E, Maren S. Reciprocal patterns of c-Fos expression in the medial prefrontal cortex and amygdala after extinction and renewal of conditioned fear. Learning & Memory. 2009;16(8):486–493. 10.1101/lm.1463909. [Europe PMC free article] [Abstract] [CrossRef] [Google Scholar]
- Knapska E, Macias M, Mikosz M, Nowak A, Owczarek D, Wawrzyniak M, et al. Functional anatomy of neural circuits regulating fear and extinction. Proceedings of the National Academy of Sciences (USA) 2012;109(42):17093–17098. 10.1073/pnas.1202087109. [Europe PMC free article] [Abstract] [CrossRef] [Google Scholar]
- Knight RT. Contribution of human hippocampal region to novelty detection. Nature. 1996;383(6597):256–259. [Abstract] [Google Scholar]
- Konorski J. Integrative activity of the brain: An interdisciplinary approach. Chicago: University of Chicago Press; 1967. [Google Scholar]
- Krechevsky I. “Hypotheses” in rats. Psychological Review. 1932;39:516–532. [Google Scholar]
- Kumaran D, Maguire EA. An unexpected sequence of events: mismatch detection in the human hippocampus. PLoS biology. 2006;4(12):e424. 10.1371/journal. [Europe PMC free article] [Abstract] [CrossRef] [Google Scholar]
- Larrauri JA, Schmajuk NA. Attentional, associative, and configural mechanisms in extinction. Psychological Review. 2008;115(3):640–676. 10.1037/0033-295X.115.3.640. [Abstract] [CrossRef] [Google Scholar]
- Mackintosh N. A theory of attention: Variations in the associability of stimuli with reinforcement. Psychological Review. 1975;82(4):276–298. [Google Scholar]
- Maren S. Overtraining does not mitigate contextual fear conditioning deficits produced by neurotoxic lesions of the basolateral amygdala. The Journal of Neuroscience. 1998;18(8):3088–3097. [Europe PMC free article] [Abstract] [Google Scholar]
- Maren S. Building and burying fear memories in the brain. The Neuroscientist : a review journal bringing neurobiology, neurology and psychiatry. 2005;11(1):89–99. 10.1177/1073858404269232. [Abstract] [CrossRef] [Google Scholar]
- Maren S. Seeking a spotless mind: extinction, deconsolidation, and erasure of fear memory. Neuron. 2011;70(5):830–845. 10.1016/j.neuron.2011.04.023. [Europe PMC free article] [Abstract] [CrossRef] [Google Scholar]
- Maren S, Hobin JA. Hippocampal regulation of context-dependent neuronal activity in the lateral amygdala. Learning & Memory. 2007;14(4):318–324. 10.1101/lm.477007. [Europe PMC free article] [Abstract] [CrossRef] [Google Scholar]
- Maren S, Holt W. The hippocampus and contextual memory retrieval in Pavlovian conditioning. Behavioural brain research. 2000;110(1–2):97–108. [Abstract] [Google Scholar]
- Maren S, Quirk GJ. Neuronal signalling of fear memory. Nature reviews Neuroscience. 2004;5(11):844–852. 10.1038/nrn1535. [Abstract] [CrossRef] [Google Scholar]
- Mcnally GP, Johansen JP, Blair HT. Placing prediction into the fear circuit. Trends in neurosciences. 2011;34(6):283–292. 10.1016/j.tins.2011.03.005. [Europe PMC free article] [Abstract] [CrossRef] [Google Scholar]
- McSweeney FK, Swindell S. Common processes may contribute to extinction and habituation. The Journal of general psychology. 2002;129(4):364–400. [Abstract] [Google Scholar]
- Orsini CA, Maren S. Neural and cellular mechanisms of fear and extinction memory formation. Neuroscience and biobehavioral reviews. 2012;36(7):1773–1802. 10.1016/j.neubiorev.2011.12.014. [Europe PMC free article] [Abstract] [CrossRef] [Google Scholar]
- Orsini CA, Kim JH, Knapska E, Maren S. Hippocampal and prefrontal projections to the basal amygdala mediate contextual regulation of fear after extinction. Journal of Neuroscience. 2011;31(47):17269–17277. 10.1523/JNEUROSCI.4095-11.2011. [Europe PMC free article] [Abstract] [CrossRef] [Google Scholar]
- Pavlov IP. In: Conditioned reflexes: an investigation of the physiological activity of the cerebral cortex. Anrep GV, translator. New York: Dover Publications; 1927. [Europe PMC free article] [Abstract] [Google Scholar]
- Pearce JM. Similarity and discrimination: A selective review and a connectionist model. Psychological Review. 1994;101(4):587–607. 10.1037/0033-295X.101.4.587. [Abstract] [CrossRef] [Google Scholar]
- Pearce JM, Bouton ME. Theories of associative learning in animals. Annual Review of Psychology. 2001;52:111–139. 10.1146/annurev.psych.52.1.111. [Abstract] [CrossRef] [Google Scholar]
- Pearce J, Hall G. A model for Pavlovian learning: Variations in the effectiveness of conditioned but not of unconditioned stimuli. Psychological Review. 1980;87(6):532–552. [Abstract] [Google Scholar]
- Plendl W, Wotjak CT. Dissociation of within- and between-session extinction of conditioned fear. The Journal of neuroscience : the official journal of the Society for Neuroscience. 2010;30(14):4990–4998. 10.1523/JNEUROSCI.6038-09.2010. [Europe PMC free article] [Abstract] [CrossRef] [Google Scholar]
- Rescorla RA, Wagner AR. A theory of Pavlovian conditioning: Variations in the effectiveness of reinforcement and nonreinforcement. In: Black AH, Prokasy WF, editors. Classical conditioning II: Current Research and Theory. Vol. 2. New York: Appleton Century Crofts; 1972. pp. 64–99. [Google Scholar]
- Robbins SJ. Mechanisms underlying spontaneous recovery in autoshaping. Journal of Experimental Psychology: Animal Behavior Processes. 1990;16(3):235. [Google Scholar]
- Schmajuk NA, Gray JA, Lam YW. Latent inhibition: a neural network approach. Journal of Experimental Psychology: Animal Behavior Processes. 1996;22(3):321–349. [Abstract] [Google Scholar]
- Sokolov M. Higher nervous functions; the orienting reflex. Annual Review of Physiology. 1963;25:545–580. 10.1146/annurev.ph.25.030163.002553. [Abstract] [CrossRef] [Google Scholar]
- Vinogradova OS. Hippocampus as comparator: role of the two input and two output systems of the hippocampus in selection and registration of information. Hippocampus. 2001;11(5):578–598. 10.1002/hipo.1073. [Abstract] [CrossRef] [Google Scholar]
- Wagner A, Spear N, Miller R. Information processing in animals: memory mechanisms. Information processing in animals: memory mechanisms. 1981:5–47. [Google Scholar]
- Wessel JR, Danielmeier C, Morton JB, Ullsperger M. Surprise and error: common neuronal architecture for the processing of errors and novelty. The Journal of Neuroscience. 2012;32(22):7528–7537. [Europe PMC free article] [Abstract] [Google Scholar]
- Yoon T, Graham L, Kim J. Hippocampal lesion effects on occasion setting by contextual and discrete stimuli. Neurobiology of Learning and Memory. 2011;95(2):176–184. 10.1016/J.Nlm.2010.07.001. [Europe PMC free article] [Abstract] [CrossRef] [Google Scholar]
- Zelikowsky M, Pham DL, Fanselow MS. Temporal factors control hippocampal contributions to fear renewal after extinction. Hippocampus. 2011 10.1002/hipo.20954. [Europe PMC free article] [Abstract] [CrossRef] [Google Scholar]
Full text links
Read article at publisher's site: https://doi.org/10.1016/j.nlm.2013.06.004
Read article for free, from open access legal sources, via Unpaywall:
https://europepmc.org/articles/pmc3830723?pdf=render
Citations & impact
Impact metrics
Citations of article over time
Smart citations by scite.ai
Explore citation contexts and check if this article has been
supported or disputed.
https://scite.ai/reports/10.1016/j.nlm.2013.06.004
Article citations
Pavlovian safety learning: An integrative theoretical review.
Psychon Bull Rev, 21 Aug 2024
Cited by: 1 article | PMID: 39167292
Review
Thalamic nucleus reuniens coordinates prefrontal-hippocampal synchrony to suppress extinguished fear.
Nat Commun, 14(1):6565, 17 Oct 2023
Cited by: 8 articles | PMID: 37848425 | PMCID: PMC10582091
Reduced renewal of conditioned suppression following lesions of the dorsal hippocampus in male rats.
Behav Neurosci, 134(5):444-459, 11 Jun 2020
Cited by: 2 articles | PMID: 32525334 | PMCID: PMC7541573
Posterior parietal cortex mediates fear renewal in a novel context.
Mol Brain, 13(1):16, 05 Feb 2020
Cited by: 5 articles | PMID: 32024548 | PMCID: PMC7003400
Pavlovian Olfactory Fear Conditioning: Its Neural Circuity and Importance for Understanding Clinical Fear-Based Disorders.
Front Mol Neurosci, 12:221, 19 Sep 2019
Cited by: 6 articles | PMID: 31607858 | PMCID: PMC6761252
Review Free full text in Europe PMC
Go to all (28) article citations
Similar Articles
To arrive at the top five similar articles we use a word-weighted algorithm to compare words from the Title and Abstract of each citation.
Hippocampal inactivation disrupts contextual retrieval of fear memory after extinction.
J Neurosci, 21(5):1720-1726, 01 Mar 2001
Cited by: 289 articles | PMID: 11222661 | PMCID: PMC6762930
Hippocampal inactivation disrupts the acquisition and contextual encoding of fear extinction.
J Neurosci, 25(39):8978-8987, 01 Sep 2005
Cited by: 258 articles | PMID: 16192388 | PMCID: PMC6725608
Dorsal hippocampus inactivation impairs spontaneous recovery of Pavlovian magazine approach responding in rats.
Behav Brain Res, 269:37-43, 15 Apr 2014
Cited by: 6 articles | PMID: 24742862 | PMCID: PMC4094020
Hippocampal regulation of context-dependent neuronal activity in the lateral amygdala.
Learn Mem, 14(4):318-324, 12 Apr 2007
Cited by: 87 articles | PMID: 17522021 | PMCID: PMC2216537