Abstract
Free full text

Induction of innate lymphoid cell-derived interleukin-22 by the transcription factor STAT3 mediates protection against intestinal infection
Summary
Inhibitors of the transcription factor STAT3 target STAT3-dependent tumorigenesis but patients often develop diarrhea from unknown mechanisms. Here we showed that STAT3 deficiency increased morbidity and mortality after Citrobacter rodentium infection with decreased secretion of cytokines including IL-17 and IL-22 associated with the transcription factor RORγt. Administration of the cytokine IL-22 was sufficient to rescue STAT3-deficient mice from lethal infection. Although STAT3 was required for IL-22 production in both innate and adaptive arms, using conditional gene deficient mice we observed that STAT3 expression in RORγt+ innate lymphoid cells (ILC3s), but not T cells, was essential for the protection. However, STAT3 was required for RORγt expression in T helper cells, but not in ILC3s. Activated STAT3 could directly bind to the Il22 locus. Thus, cancer therapies that utilize STAT3 inhibitors increase the risk for pathogen-mediated diarrhea through direct suppression of IL-22 from gut ILCs.
INTRODUCTION
Signal transducer and activator of transcription 3 (STAT3) is a transcription factor that regulates many genes involved in apoptosis, proliferation, migration and survival in different cell types. STAT3 signaling is constitutively active in many types of tumor cells and tumor-associated immune cells during tumorigenesis, and this dysregulation promotes tumor growth and suppresses antitumor immune responses (Yu et al., 2007). Thus, STAT3 inhibitors have been explored in clinical trials for different cancer patients (Page et al., 2011). Sunitinib, an oral multitargeted tyrosine kinase inhibitor used for the treatment of several types of cancers including gastrointestinal tumors, also suppressed STAT3 activity in host immune cells (Xin et al., 2009). However, nearly half of the patients developed diarrhea after Sunitinib treatment with unclear pathogenesis (Schwandt et al., 2009). Mutations of STAT3 have been shown to be related to Hyper-immunoglobulin E syndrome patients with recurrent mucosal infections (Minegishi et al., 2007). Therefore, STAT3-related diarrhea could be linked with increased susceptibility to mucosal infections such as intestinal infections.
Citrobacter rodentium is a natural mouse extracellular enteric pathogen that mimics human Enterohaemorrhagic E. coli and Enteropathogenic E. coli, which are major causes of diarrheal disease worldwide that result in hundreds of thousands of deaths each year. Clearance of C. rodentium requires both the innate and adaptive immune responses (Bry and Brenner, 2004; Maaser et al., 2004). Both RORγt+ group 3 innate lymphoid cells (ILC3s) and T helper cells (Th17, Th22) are important for the host to control C. rodentium infection (Basu et al., 2012; Ivanov et al., 2009; Qiu et al., 2011; Tumanov et al., 2011). Transferring either wild-type ILC3s cells (Sonnenberg et al., 2011) or Th22 cells (Basu et al., 2012) protects the mice from C. rodentium infection. However, it is not clear whether the innate or adaptive RORγt+ lymphocytes are essential for protection against infection.
ILCs represent a family of immune cells with morphological characteristics of lymphocytes, yet lack rearranged antigen receptors. ILCs can produce an array of effector cytokines that correspond to the cytokine profiles of the T helper cell subsets; for example, IFN-γ by group 1 ILCs (NK cells and ILC1) and Th1 cells, IL-5 and IL-13 by group 2 ILCs (ILC2) and Th2 cells, and IL-17 and IL-22 by ILC3s (including LTi, NCR+ ILC3 and NCR− ILC3) and Th17 and Th22 cells (Spits et al., 2013; Spits and Cupedo, 2012; Spits and Di Santo, 2010). The current dogma is that development and function of innate and adaptive lymphoid cells are under the control of analogous transcription factors. T-bet is involved in the development of NK, ILC1 and Th1 cells (Fuchs et al., 2013; Gordon et al., 2012; Szabo et al., 2000), and GATA3 is critical for the development of both ILC2 and Th2 cells (Furusawa et al., 2013; Hoyler et al., 2012; Klein Wolterink et al., 2013; Mjosberg et al., 2012; Moro et al., 2010; Zheng and Flavell, 1997), whereas RORγt is required for the development of both ILC3s and Th17 cells (Eberl et al., 2004; Ivanov et al., 2006). STAT proteins are transcription factors involved in the differentiation of T helper cells (O’Shea et al., 2011). However, whether ILCs and T helper cells also share STAT protein for their development and downstream cytokines production is still unknown.
Previous studies have shown that deletion of STAT3 in Th17 cells impairs their expression of RORγt and development (Ivanov et al., 2006; Laurence et al., 2007; O’Shea et al., 2011; Veldhoen et al., 2008; Yang et al., 2007; Yang et al., 2008; Zhou et al., 2007), but its role in ILCs has not been examined yet. Because innate and adaptive IL-17 producers share many transcriptional networks, it is expected that STAT3 also regulates RORγt expression in ILCs, analogous to their adaptive counterparts. However, in contrast to the STAT3-dependent development of Th17 and Th22 cells, we revealed that STAT3 does not control RORγt expression on ILCs, but rather directly regulates their production of IL-22 in response to infection.
RESULTS
Sunitinib impairs the host defense against intestinal C. rodentium infection
To determine whether tyrosine kinase (including STAT3 pathway) inhibitor treatment increases the risk of gut infection, C. rodentium infected mice were orally administered Sunitinib at clinically relevant doses (Xin et al., 2009). After C. rodentium infection, the mice with high-dose Sunitinib (40 mg/Kg) treatment developed severe diarrhea and rapidly lost body weight, with nearly fifty percent mortality by 3 weeks; low-dose Sunitinib (4 mg/Kg) treated mice also developed diarrhea but lost less body weight, with few mortality by 3 weeks; however, neither weight loss nor death was observed in the control treatment mice (Figure 1A and 1B). Sunitinib treatment also resulted in increased bacterial titers in both feces and blood at day 8 and 11 post-infection (Figure 1C). Compared with control mice, the Sunitinib treated mice exhibited severe colonic pathology with severely disruption of the epithelial layer and infiltration of inflammatory cells (Figure S1). Together, these data indicate that oral Sunitinib administration could increase morbidity and mortality following intestinal C. rodentium infection.
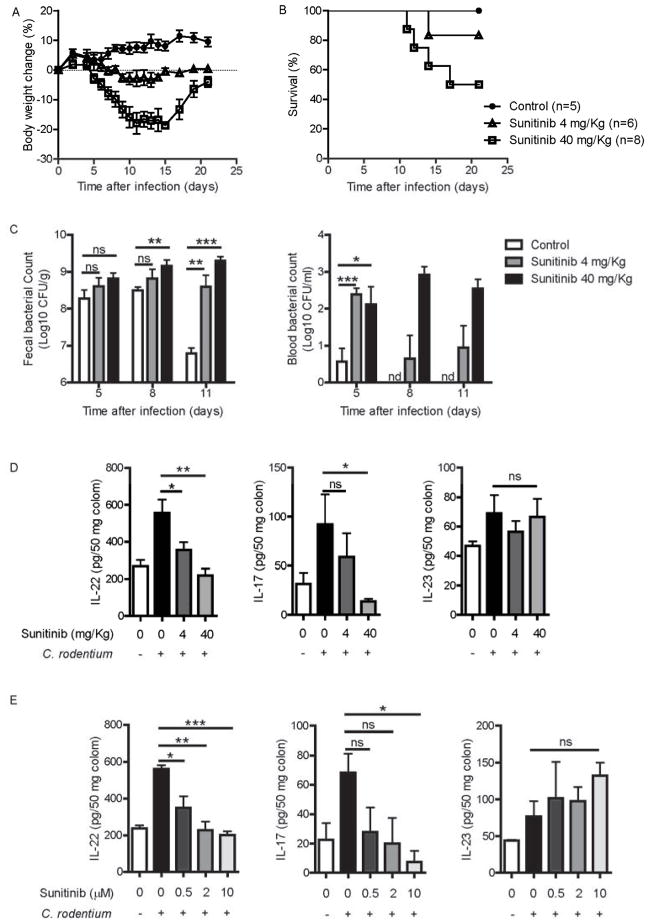
(A–C) 8 weeks old wild type mice were orally inoculated with 2 × 109 CFU of C. rodentium. Sunitinib (4 mg/Kg, n=6 or 40 mg/Kg, n=8) or control (n=5) was administered orally from day 0 to day 7, once daily. Body weight was measured at the indicated time points (A) and survival rates (B) are shown.
(C) Bacterial titers from blood and fecal homogenate cultures at indicated day post infection in the mice in A, B.
(D) IL-22, IL-17 and IL23 levels from the colon culture supernatants of Sunitinib or control treated mice at day 5 after infection (n=5 each group).
(E) The colons from untreated mice (n=3) were removed at day 5 after infection, and cultured with Sunitinib at indicated concentrations for 24 hours in vitro. IL-22, IL-17 and IL23 levels were examined in the colon culture supernatants.
*P<0.05, **P<0.01, ***P<0.001; ns, no significant difference (Student’s t-test); nd, nondetectable. Data are representative of two independent experiments (mean ± s.e.m.). See also Figure S1.
Previous studies have shown that the Th17 and Th22 cell-related cytokines, IL-17, IL-22 and IL-23, are important for the host defense against C. rodentium infection (Ishigame et al., 2009; Zheng et al., 2008). To determine whether the increased risk of intestinal infection by tyrosine kinase inhibition was due to impaired host immune responses, the colonic cytokine expression profile was examined at day 5 post infection. In contrast to Th1 and Th2 cell-related cytokines, the Th17 and Th22 cell-related cytokines (IL-17 and IL-22) were up-regulated after infection but were suppressed by both in vivo and in vitro Sunitinib treatment (Figure 1D, 1E and data not shown). Although IL-23 from DC or macrophages is a major inducer for IL-22 production, Sunitinib had no effect on IL-23 production (Figure 1D and 1E). Taken together, these data indicate that Sunitinib impairs the host defense against intestinal C. rodentium infection. It raises the possibility that STAT3 might contribute to IL-22-dependent protection.
STAT3 signaling from RORγt+ lymphocytes controls C. rodentium infection
IL-17 and IL-22 are known to be regulated by RORγt (encoded by Rorc) and are mainly produced by RORγt+ ILCs and T cells (Ivanov et al., 2006; Qiu et al., 2011). To determine whether Sunitinib’s adverse effect was due to STAT3 inhibition on ILC3s and T cells, Stat3-floxed mice were crossed with Rorc-cre transgenic mice to achieve specific deletion of STAT3 in RORγt-expressing cells (Rorc-cre-Stat3fl/fl). Because RORγt is transiently expressed at high levels at the double positive stage of T cell development, Rorc-cre-Stat3fl/fl mice not only lack STAT3 expression by RORγt+ ILC3s, but also most αβ T cells (Figure S2A–S2C).
We next tested whether STAT3 signaling is necessary for controlling the C. rodentium infection. After infection, Rorc-cre-Stat3fl/fl mice rapidly lost body weight and died around day 10, whereas no weight loss or death was observed in their littermate control wild-type (Stat3fl/fl) mice (Figure 2A and 2B) or Rorc-cre-Stat3fl/+ heterozygous mice (Figure S2D). To test whether the increased morbidity and mortality were associated with bacterial colitis, we checked bacterial titer and diarrhea status. Rorc-cre-Stat3fl/fl mice had 10–100 times higher bacterial titers in the feces compared to WT mice at day 5 and 8 post-infection (Figure 2C and S2E). Rorc-cre-Stat3fl/fl mice also showed increased bacterial titers in the blood, liver and spleen (Figure 2D and S2E), suggesting systemic dissemination of C. rodentium. The Rorc-cre-Stat3fl/fl mice exhibited severe diarrhea, colonic shortening, severe inflammation, and tissue injury in the colon and severely disruption of the epithelial layer compared with WT mice (Figure 2E, 2F and S2F). Together, these data indicate that STAT3 signaling in RORγt+ cells is essential for host defense against a mucosal bacterial pathogen.
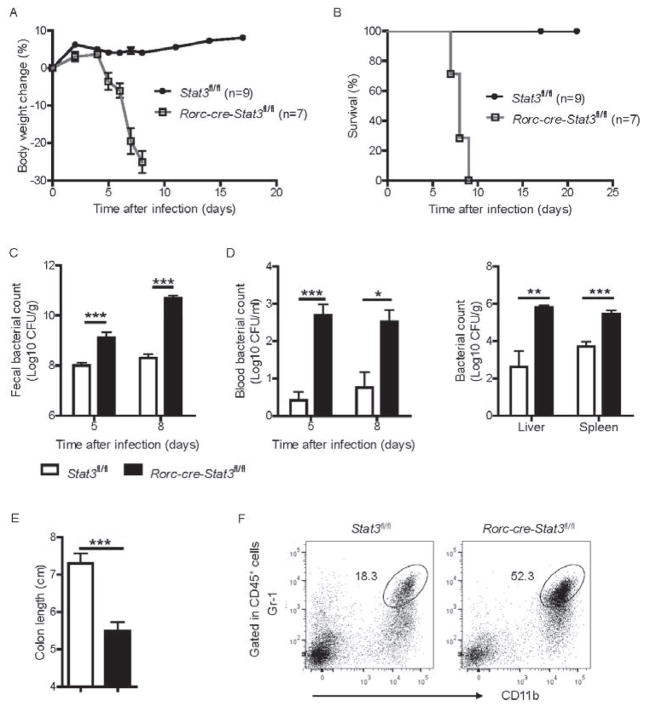
(A–F) 7 weeks old Rorc-cre-Stat3fl/fl (n = 7) and their littermate wild type Stat3fl/fl (n = 9) mice were orally inoculated with C. rodentium (2 × 109 CFU) and body weight was measured at the indicated time points. Body weight change (A) and survival rates (B) are shown.
(C) Bacterial titers from fecal homogenate cultures at indicated day post infection in the mice in A, B.
(D) Bacterial titers from blood and spleen, liver homogenate cultures at indicated day post infection are shown.
(E) Rorc-cre-Stat3fl/fl mice show colon shortening at day 8 post infection.
(F) Rorc-cre-Stat3fl/fl mice show more inflammatory monocytes or neutrophils infiltration in the colon. LPLs were isolated from colon and gated in CD45+ cells.
*P<0.05, **P<0.01, ***P<0.001 (Student’s t-test). Data are representative of three independent experiments (A–C; mean ± s.e.m.) or two independent experiments (D–E; mean ± s.e.m.). See also Figure S2.
STAT3 signaling from RORγt+ lymphocytes controls both IL-22 and IL-17 production during C. rodentium infection
We have shown that tyrosine kinase (including STAT3 pathway) inhibition suppressed both IL-22 and IL-17 expression after infection, which are required for the protection against C. rodentium infection (Ishigame et al., 2009; Ivanov et al., 2009; Wang et al., 2010; Zheng et al., 2008). We also measured IL-22 and IL-17 amounts in the colon of WT and Rorc-cre-Stat3fl/fl mice early after C. rodentium infection. Compared to WT mice, expression of IL-22 and IL-17 was significantly reduced in the colon of Rorc-cre-Stat3fl/fl mice on day 5 post infection (Figure 3A). RegIIIγ and RegIIIβ, two antimicrobial proteins dependent on IL-22 (Pickert et al., 2009; Zheng et al., 2008), were also reduced in the colon of Rorc-cre-Stat3fl/fl mice after infection (Figure 3A). These results suggest that the STAT3 signaling is essential for IL-22 and IL-17 production in the gut after mucosal bacterial infection.
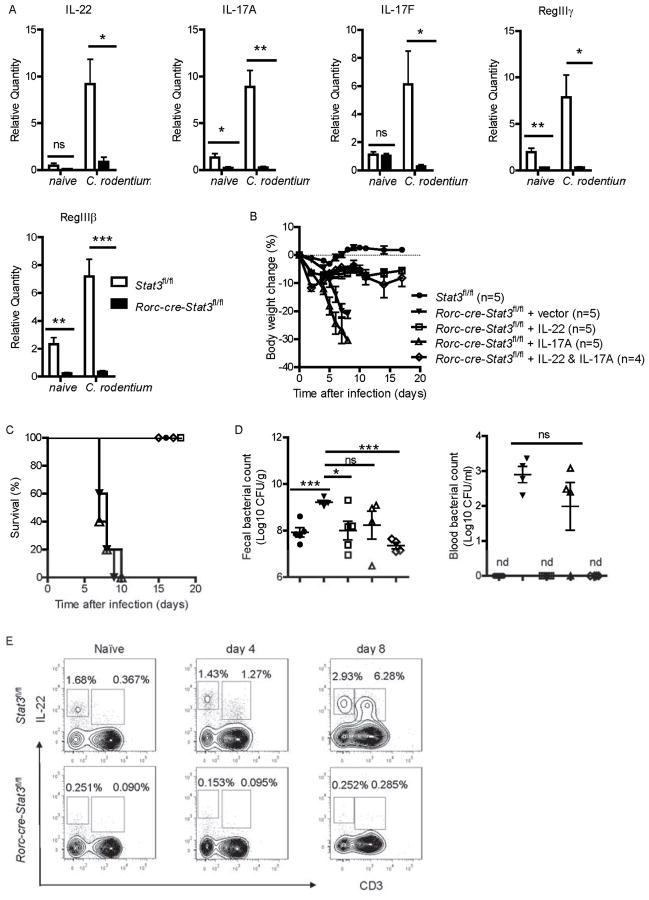
(A) Rorc-cre-Stat3fl/fl and wild type Stat3fl/fl littermate mice (n = 5 per group) were infected with 2 × 109 CFU of C. rodentium. The mRNA expression of IL-22, IL-17A, IL-17F and IL-22- dependent RegIIIγ and RegIIIβ antimicrobial proteins in the colon of naïve or 5 days infected mice were measured by real-time. *P<0.05, **P<0.01, ***P<0.001 (Student’s t-test). Data are representative of two independent experiments (A; mean ± s.e.m.).
(B–D) Stat3fl/fl (n = 5) and Rorc-cre-Stat3fl/fl mice were orally inoculated with 2 × 109 CFU of C. rodentium. 10 μg control vector (n = 5), IL-17 (n = 5), IL-22 (n = 5), or both IL-17 and IL-22 (n = 4) expressing plasmids were hydrodynamic injected into Rorc-cre-Stat3fl/fl mice through the tail vein 6 hr after C. rodentium infection. Body weight changes (B) and survival rates (C) were monitored at indicated time points. (D) CFU counts of C. rodentium in the fecal pellets and blood on day 5 after infection are shown. Data are representative of two independent experiments (mean ± s.e.m.). *P<0.05, ***P<0.001; ns, no significant difference (Student’s t-test). nd, nondetectable.
(E) STAT3 signaling is required for both innate and adaptive IL-22 production. IL-22 expression in CD3− and CD3+ cells were analyzed by intracellular cytokine staining followed by flow cytometry. Rorc-cre-Stat3fl/fl and wild type Stat3fl/fl littermate mice were infected with 2 × 109 CFU of C. rodentium. Intestinal LPLs were isolated from the colons of Stat3fl/fl or Rorc-cre-Stat3fl/fl mice at day 4 and day 8 post-infection. Cells were stimulated with IL-23 (25 ng/ml), PMA (50 ng/ml) and ionomycin (750 ng/ml) for 4 hours and gated in Thy1+ lymphocytes. Naïve mice were used as control. Data are representative of three independent experiments. See also Figure S3.
Exogenous IL-22 but not IL-17 rescues STAT3 deficiency mice during C. rodentium infection
Because both IL-17 and IL-22 are controlled by STAT3 signaling, we wondered whether administration of exogenous IL-17 or IL-22 is sufficient to rescue the death of Rorc-cre-Stat3fl/fl mice from C. rodentium infection. To address this question, IL-17A and IL-22 expressing plasmids were hydrodynamically injected into Rorc-cre-Stat3fl/fl mice at the same day of C. rodentium infection. All of the Rorc-cre-Stat3fl/fl mice treated with IL-22 survived after infection and lost weight only slightly, whereas vector and IL-17 alone treated mice rapidly lost body weight and succumbed to C. rodentium infection (Figure 3B and 3C). Accordingly, IL-22 treated Rorc-cre-Stat3fl/fl mice had reduced bacterial counts in their feces and blood compared to vector and IL-17 alone treated mice (Figure 3D). Collectively, these data demonstrate that exogenous IL-22 but not IL-17 is able to rescue Rorc-cre-Stat3fl/fl mice from a lethal C. rodentium infection and IL-22 is an essential and sufficient pathway downstream of STAT3 signaling to protect mice against mucosal bacterial pathogens.
STAT3 signaling is required for both innate and adaptive IL-22 production
Previous studies have shown that C. rodentium infection induces early wave of IL-22 production by ILC3s and second wave by CD4+ T cells (Th22) (Basu et al., 2012; Qiu et al., 2011; Tumanov et al., 2011). To test whether STAT3 control innate or adaptive IL-22 production, we examined cytokine expression in the intestinal lamina propria lymphocytes (LPLs) isolated from both naïve and infected Rorc-cre-Stat3fl/fl and wild type mice by intracellular cytokine staining. Consistent with the previous study (Basu et al., 2012; Qiu et al., 2011), CD3− innate LPLs were the main IL-22 producers compared to CD3+ T cells in naïve and infected WT mice at an early stage of infection (day 4), while there were more IL-22 producing T cells than ILCs at a later stage of infection (day 8) in WT mice (Figure 3E). However, Rorc-cre-Stat3fl/fl mice had severely decreased expression of IL-22 by intestinal LPLs, regardless of CD3− innate cells or CD3+ T cells (Figure 3E, S3A and S3B). Moreover, Sunitinib treatment also suppressed IL-22 production in innate LPLs from wild type mice (Figure S3E). In a similar manner as IL-22, the IL-17 production was also significantly reduced in both innate and adaptive lymphocytes (Figure S3C and S3D). Since PMA and ionomycin are not physiological stimuli, the LPLs were also stimulated with anti-CD3 and anti-CD28 to mimic TCR signaling. Similar to PMA and ionomycin treatment, STAT3 deficient T cells also showed little IL-22 and IL-17 production after anti-CD3 and anti-CD28 stimulation (Figure S3F). Together, our data indicate that STAT3 signaling is essential for IL-22 and IL-17 expression in both ILC3s and T cells.
STAT3 signaling and IL-22 from ILC3s but not from T cells are essential for the control of C. rodentium infection
Because STAT3 signaling regulates IL-22 expression in both innate and adaptive lymphocytes, we wondered whether innate or adaptive IL-22 is more important for the protection from C. rodentium infection. To test whether the STAT3 signaling from ILC3s is sufficient for the protection against C. rodentium infection in the transient absence of T cells, CD4 mAb was administered to infected Rorc-cre-Stat3fl/fl and WT mice to deplete the CD4+ T cells. To avoid depletion of CD4+ LTi cells, we used low dose of CD4 mAb to transiently remove CD4+ T cells but not CD4+ ILC3s. As shown in Figure S4A, 90% of peripheral CD4+ T cells were depleted, while there was no proportional reduction of ILC3s in the lamina propria after multiple CD4 mAb injection. Because CD4+ T cells are required for host adaptive immunity especially for antibody responses against C. rodentium (Bry et al., 2006), depletion of CD4+ T cells provided another model to study whether the innate immune response is sufficient against this pathogen in the absence of adaptive responses. Treatment with CD4 mAb resulted in reduction of C. rodentium specific IgG in WT mice and all of them died at 3 weeks after C. rodentium infection (Figure S4B, 4A and 4B), which is the same as Rag1−/− mice, suggesting that adaptive immunity is responsible for the late phase of protection. Treatment of Rorc-cre-Stat3fl/fl mice with additional CD4 mAb did not aggravate or ameliorate the C. rodentium infection compared with Rat IgG (control for CD4 mAb) treatment group. All of the Rorc-cre-Stat3fl/fl mice with or without depletion of CD4+ T cells still died on day 8 post-infection (Figure 4A–4C). These data suggest that STAT3 signaling and IL-22 from CD4+ T cells are not essential for protecting mice in the early phase of C. rodentium infection.
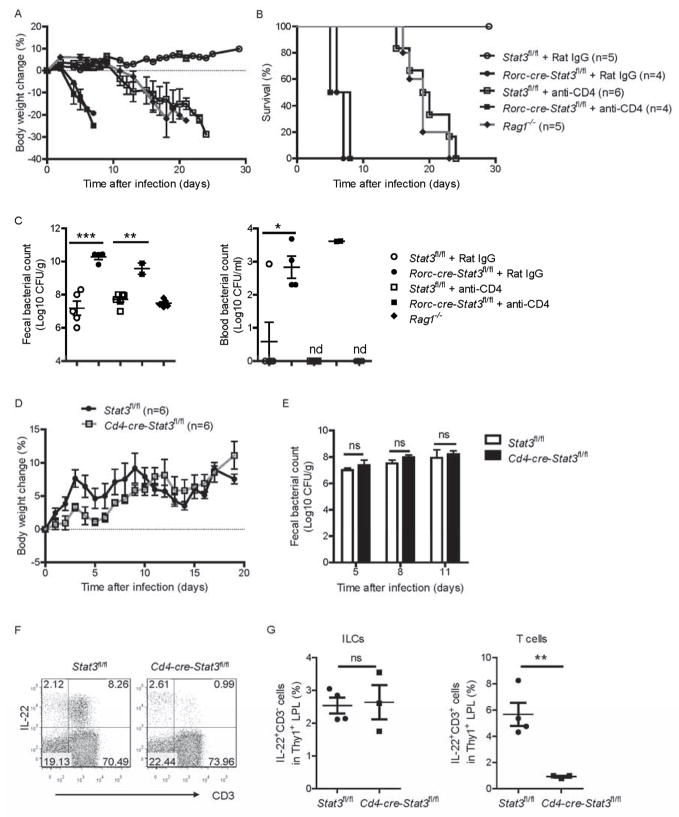
(A–C) Rorc-cre-Stat3fl/fl and wild type Stat3fl/fl mice were infected with 5 × 106 CFU of C. rodentium and injected intraperitoneally with mAb GK1.5 or Rat IgG (50 μg per mouse each time) at day −5, 0, 5, 10 and 15 post infection for depletion of CD4+ T cells (4 to 6 mice per group). Average body weight change (A) and survival rates (B) at the indicated time points are shown. (C) Bacterial titers from blood and fecal homogenate cultures at day 5 post infection. Each dot represents one individual mouse.
(D, E) Cd4-cre-Stat3fl/fl (n=6) and wild type Stat3fl/fl mice (n=6) were orally infected with 2 × 109 CFU of C. rodentium. (D) Body weight changes are shown at the indicated time points. (E) Bacterial titers from fecal homogenate cultures at indicated day post infection in the mice in D.
(F, G) IL-22 expression in CD3− and CD3+ cells were analyzed by intracellular cytokine staining followed by flow cytometry. Cd4-cre-Stat3fl/fl and wild type Stat3fl/fl littermate mice were infected with 2 × 109 CFU of C. rodentium. LPLs were isolated from the colons at day 8 post-infection. Cells were stimulated with IL-23 (25 ng/ml), PMA (50 ng/ml) and ionomycin (750 ng/ml) for 4 hours and gated in Thy1+ lymphocytes. Each dot represents one individual mouse.
*P<0.05, **P<0.01, ***P<0.001; ns, no significant difference (Student’s t-test). nd, nondetectable. Data are representative of two independent experiments (A–G; mean ± s.e.m.). See also Figure S4.
To further test whether STAT3 signaling in T cells is required for the later T and B cell response and prevention of chronic infection, we rescued Rorc-cre-Stat3fl/fl mice by exogenous IL-22 and examined the antibody production. IL-22-rescued Rorc-cre-Stat3fl/fl mice produced the same amount of C. rodentium specific IgG as WT mice (Figure S4C). Moreover, 60 days after first C. rodentium infection, IL-22-rescued Rorc-cre-Stat3fl/fl mice were challenged with high dose of C. rodentium again. Interestingly, none of the IL-22-rescued Rorc-cre-Stat3fl/fl mice showed diarrhea (data not shown) and weight loss (Figure S4D), suggesting IL-22-rescued Rorc-cre-Stat3fl/fl mice developed normal protection. Together, these data demonstrate that STAT3 signaling from CD4+ T cells is not essential for normal IgG production and protecting mice after C. rodentium infection.
To further test whether the STAT3 signaling from RORγt+ ILCs is sufficient for the protection against C. rodentium infection, we crossed the Stat3-floxed mice with Cd4-cre mice to generate mice lacking STAT3 only in T cells (Cd4-cre-Stat3fl/fl) but not in CD4+ RORγt+ ILCs (Eberl and Littman, 2004) (data not shown). Even after high dose of C. rodentium infection, the Cd4-cre-Stat3fl/fl mice did not lose body weight and all survived (Figure 4D). Furthermore, the fecal titers of C. rodentium were not significant higher in Cd4-cre-Stat3fl/fl mice than WT mice 5–11 days after infection (Figure 4E). Similar to the Rorc-cre-Stat3fl/fl mice, Cd4-cre-Stat3fl/fl mice also lacked IL-22 producing CD4+ T cells in the gut (Figure 4F and 4G). However, the IL-22 producing ILCs in Cd4-cre-Stat3fl/fl mice were not reduced compared with WT mice, which were different with Rorc-cre-Stat3fl/fl mice (Figure 3E, 4F and 4G). Furthermore, CD4 mAb was also administered to Cd4-cre-Stat3fl/fl mice and Stat3fl/fl mice. As shown in Figure S4E and S4F, both CD4 mAb treated Stat3fl/fl or Cd4-cre-Stat3fl/fl mice lost body weight and died around day 20 post infection, while untreated mice survived, suggesting that CD4+ T cells are important for the protection against C. rodentium infection, but STAT3 signaling from T cells is not essential. Together, these data demonstrate that STAT3 signaling and IL-22 from ILC3s, but not CD4+ T cells, is essential for protecting mice from death during C. rodentium infection.
CD90hiCD45lo ILC3s protect Rorc-cre-Stat3fl/fl mice during C. rodentium infection
Because we have shown that STAT3 signaling from ILC3s but not T cells is essential for the protection from C. rodentium infection, we wonder whether adoptive transfer of ILC3s could rescue Rorc-cre-Stat3fl/fl mice from infection. However, because of extremely low number of ILC3s and limited Rorcgfp/+ mice, it was difficult to get enough ILC3s. To find better cell surface marker to identify ILC3s, we screened a large panel of surface markers and found ILC3s could be strictly identified by CD45 and CD90. As shown in Figure 5A, after gating with CD45 and CD90, CD90+ LPLs from the large intestine and small intestine of Rag1−/− mice fell into three separate populations, CD90hiCD45lo, CD90loCD45int and CD90loCD45hi. Most of CD90hiCD45lo LPLs (96%) were RORγt+ ILC3s, and most of CD90loCD45int LPLs (95%) were NK1.1−RORγt− ILCs, whereas nearly three fourths of CD90loCD45hi were NK1.1+ NK cells. Similar to Rag1−/− mice, CD90+ LPLs from both large intestine and small intestine of C57BL/6 WT mice fell into two separate populations, CD90hiCD45lo and CD90loCD45hi. Most of CD90hiCD45lo LPLs (94%) were RORγt+ ILC3s, whereas CD90loCD45hi were CD3+ T cells and other RORγt− ILCs (Figure S5). These data suggest that CD90hiCD45lo are specific cell surface markers for intestinal RORγt+ ILC3s.
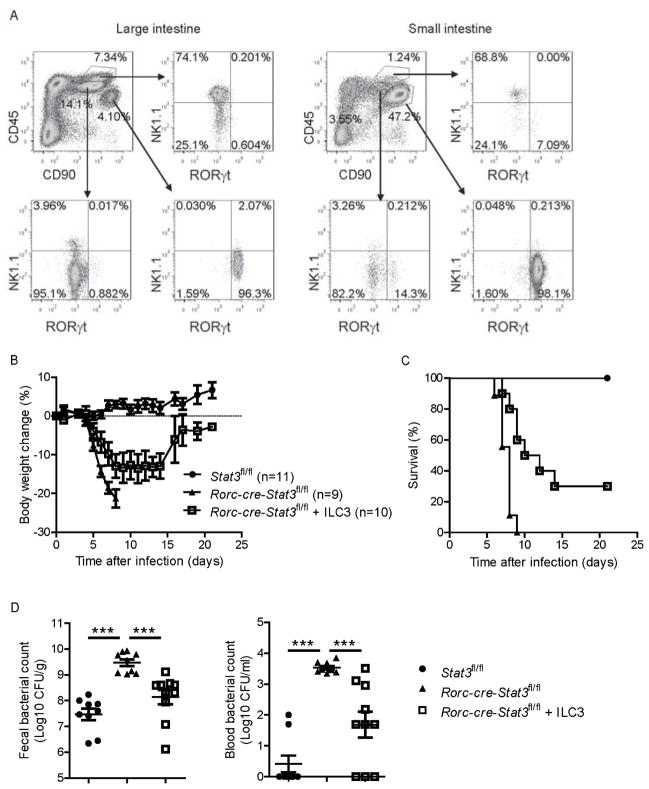
(A) Intestinal CD90hiCD45lo LPLs are RORγt+ ILCs. LPLs were isolated from both the large intestine and small intestine of Rag1−/− mice and gated by CD45 and CD90 expression. Three separate populations, CD90hiCD45lo, CD90loCD45int and CD90loCD45hi were further analyzed with the expression of NK1.1 and RORγt. Data are representative of two independent experiments.
(B–D) Rag1−/− mice were infected with 2 × 109 CFU of C. rodentium and CD90hiCD45lo ILC3s were sorted from both the colon and small intestine LPLs at day 1 post infection. Sorted ILC3s were stimulated with IL-23 (25 ng/ml) for 1 hour, then were transferred by i.v. injection (2 × 105 cells per mouse) into Rorc-cre-Stat3fl/fl recipients at day 0 and day 3 post inoculated with 5 × 106 CFU of C. rodentium. Untreated Rorc-cre-Stat3fl/fl and wild type Stat3fl/fl littermate mice were as control. Body weight changes (B) and survival rates (C) were monitored at indicated time points. (D) CFU counts of C. rodentium in the fecal pellets and blood on day 5 after infection are shown. Data are pooled from two independent experiments with nine to eleven mice per group (mean ± s.e.m.). ***P<0.001 (Student’s t-test). See also Figure S5.
Then we sorted CD90hiCD45lo ILC3s and transferred small number of them into Rorc-cre-Stat3fl/fl mice with C. rodentium infection. Compared with untreated Rorc-cre-Stat3fl/fl mice, ILC3s-transfered Rorc-cre-Stat3fl/fl mice lost less body weight and about one third of the mice survived and recovered from C. rodentium infection (Figure 5B and 5C). Accordingly, ILC3s-transferred Rorc-cre-Stat3fl/fl mice exhibited reduced bacterial counts in their feces and blood compared to untreated mice (Figure 5D). Collectively, these data demonstrate that CD90hiCD45lo ILC3s are sufficient to protect mice against C. rodentium infection.
The development of RORγt+ Th cells, but not RORγt+ ILC3s, requires STAT3 signaling
Because Rorc-cre-Stat3fl/fl mice showed reduced IL-22 in both ILCs and T cells, we next determined how STAT3 regulates IL-22 production. IL-22 is mainly produced in RORγt+ cells, and RORγt directly regulates IL-22 production at the transcriptional level. Previous studies have shown that STAT3 regulates the development of RORγt+ Th17 cells (Ivanov et al., 2006; Laurence et al., 2007; Yang et al., 2007). To determine whether STAT3 signaling is shared by both innate and adaptive colonic RORγt+ cells for their development, we examined the RORγt expression in the intestinal LPLs isolated from Rorc-cre-Stat3fl/fl mice and their littermate control wild-type mice. Consistent with Cd4-cre-Stat3fl/fl mice (Figure S6A), both the percentage and number of RORγt+ Th cells (CD3+CD4+RORγt+) in the colon were much lower in Rorc-cre-Stat3fl/fl mice compared with the WT mice (Figure 6A–6C and S6B). Unexpectedly, there were no differences in the percentages and numbers of the total RORγt+ ILC3s (Figure 6D–6F), the subsets of ILC3s (LTi, NCR+ ILC3 and NCR− ILC3; Figure 6D and S6C) or other ILCs (ILC1, ILC2; Figure S6C) between Rorc-cre-Stat3fl/fl and WT mice, suggesting that STAT3 was required for RORγt expression in T cells but not ILC3s or maintenance of ILC3s. Moreover, similar to naïve mice, there were no differences in percentages and total numbers of the ILC3s after C. rodentium infection between Rorc-cre-Stat3fl/fl and WT mice (Figure 6E and 6F). Taken together, our data indicate that STAT3 signaling is essential for the development of RORγt+ Th cells, but not ILC3s, and that STAT3-controlled IL-22 production is not through regulating RORγt expression in innate cells, which also suggests that RORγt is not sufficient to induce IL-22 production in the absence of STAT3 signaling.
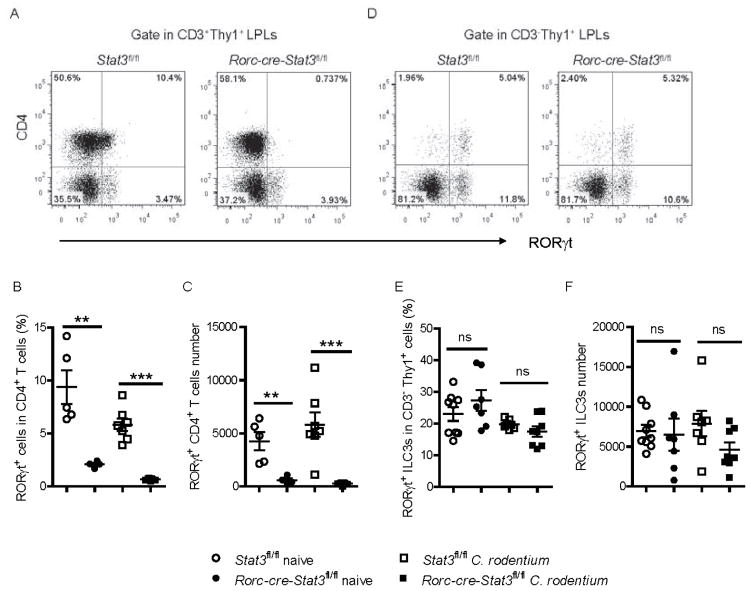
(A–F) Colonic LPLs were isolated from Stat3fl/fl and Rorc-cre-Stat3fl/fl littermate mice before or 5 days after C. rodentium infection.
(A, D) RORγt and CD4 expression were analyzed in colonic LPLs from naïve mice by flow cytometry after gating on Thy1+ CD3+ adaptive lymphocytes and Thy1+ CD3− innate lymphoid cells.
(B, C) Percentage of RORγt+ cells in the CD4+ CD3+ T cell population, as well as the absolute numbers of RORγt+ CD4+ T cells in the colons of naïve and C. rodentium infected Stat3fl/fl and Rorc-cre-Stat3fl/fl mice are shown.
(E, F) Percentage of RORγt+ cells in the CD3− Thy1+ ILCs population, as well as the absolute numbers of RORγt+ ILCs in the colons of naïve and C. rodentium infected Stat3fl/fl and Rorc-cre-Stat3fl/fl mice are shown.
**P<0.01, ***P<0.001; ns, no significant difference (Student’s t-test). Data are representative of three independent experiments (mean ± s.e.m.). Each dot represents one individual mouse (B, C, E, F). See also Figure S6.
STAT3 directly binds to the Il22 locus and regulates IL-22 production
Previous studies have shown that IL-23 is an inducer of IL-22 (Zheng et al., 2008). IL-23 interacts with the IL-23 receptor (IL-23R) and activates STAT3, which has multiple target genes, such as Il23r, Rorc, Ahr in T cells (Ghoreschi et al., 2010; Ivanov et al., 2006; Veldhoen et al., 2008). RORγt and Ahr can bind to the Il22 locus and directly promote IL-22 production (Qiu et al., 2011). As the expression of RORγt in ILCs was not reduced in Rorc-cre-Stat3fl/fl mice, we began to explore other pathways, such as IL23R or Ahr, defects in which could result in a defective response to IL-23 and subsequently reduced expression of IL-22. To test this hypothesis, we first examined the expression of these related genes in the colon of WT and Rorc-cre-Stat3fl/fl mice before or early after C. rodentium infection. Unexpectedly, there were no significant reductions of IL-23p19, p40, IL23R and Ahr in the Rorc-cre-Stat3fl/fl mice compared with WT mice, and the expression of RORγt was actually increased in the STAT3-deficient mice, likely as a secondary response to the increased infection (Figure 7A). Interestingly, IL-6 was increased in Rorc-cre-Stat3fl/fl mice after infection, indicating increased inflammation. Even with higher expression of RORγt, the IL-22 production was still impaired, suggesting that the regulation of IL-22 production by STAT3 signaling may not be mediated through these related pathways.

(A) Rorc-cre-Stat3fl/fl and Stat3fl/fl littermate mice (n = 5 per group) were infected with 2 × 109 CFU of C. rodentium. The mRNA expression of IL-23 p19, IL-23 p40, IL23R, IL-6, RORγt and Ahr in the colon were measured by real-time PCR before and 5 days after infection. Data are representative of two independent experiments (mean ± s.e.m.).
(B) RORγt+ ILCs were purified by flow cytometric sorting from colonic LPLs of Rorc-cre-Stat3fl/fl Rorcgfp/+ and Rorcgfp/+ mice and stimulated with or without IL-23 (25 ng/ml) for 1 hour. The mRNA expression of IL-22, IL-23R, RORγt and Ahr were measured by real-time PCR. Data are representative of two independent experiments (mean ± s.e.m.).
(C) STAT3 binding sites at the Il22 locus are shown.
(D) STAT3 binding at the Il22 locus in EL4 stable cell lines stably expressing either flag peptide (DFTC) or flag-tagged STAT3C (DFTC-STAT3C) was monitored using a ChIP assay. The anti-flag antibody immunoprecipitates were analyzed by real-time PCR. The fold enrichment of STAT3C binding at each locus was normalized to DFTC-empty EL4 cells. Ltbr and Il17 loci were used as negative and positive controls in the ChIP assays, respectively. Data are representative of three independent experiments (mean ± s.e.m. of triplicate samples of real-time PCR).
(E) CD90hiCD45lo ILC3s were sorted from Rag1−/− mice and stimulated with IL-23 (25 ng/ml) for 30 minutes. ChIP assays with anti-STAT3 mAb showed enhanced enrichment of STAT3 at the Il22 locus in primary ILC3s. Ltbr loci lacking STAT3 binging sites was used as negative controls. Data are representative of two independent experiments (mean ± s.e.m. of quadruplicate samples of real-time PCR).
*P<0.05, **P<0.01, ***P<0.001; ns, no significant difference (Student’s t-test).
To further study the mechanism of how STAT3 regulates IL-22, ILC3s were purified from both Rorcgfp/+ and Rorc-cre-Stat3fl/fl Rorcgfp/+ mice by flow cytometric sorting and stimulated with IL-23. Consistent with the previous results, STAT3 is required for IL-22 production in ILC3s after IL-23 stimulation. However, there were no significant differences in IL-23R, RORγt and Ahr between STAT3 deficient and WT ILC3s (Figure 7B). Together, these data suggest that STAT3 regulation of IL-22 is not through IL-23R, RORγt and Ahr.
STAT3 is a transcription factor that directly regulates IL-17 production (Yang et al., 2011). Therefore we wondered whether STAT3 can also directly regulate IL-22 production at the transcriptional level. Using an in silico approach, we found multiple STAT3 binding sites at the promoter and intron 1 of the Il22 gene (Hutchins et al., 2012), suggesting that the Il22 locus may be a direct target of STAT3 (Figure 7C). Indeed, using EL4 lymphoma cells stably expressing the double flag peptide (DTFC) and double flag-tagged STAT3C (DFTC-STAT3C), an active form of STAT3 (Zhou et al., 2007), a chromatin immunoprecipitation (ChIP) assay using anti-flag antibodies showed enhanced recruitment of STAT3 to the Il22 locus in cell lines stably expressing DFTC-STAT3C, suggesting that Il22 is a direct target gene of STAT3 (Figure 7D). To further confirm binding of STAT3 to the Il22 locus in primary cells, we sorted ILC3s from the intestinal LPLs of Rag1−/− mice. ChIP assay with anti-STAT3 antibody also showed enhanced recruitment of STAT3 to the Il22 locus in ILC3s (Figure 7E). Together, these data suggest that STAT3 could directly bind to the Il22 locus and promote IL-22 production in RORγt+ cells.
DISCUSSION
IL-22-producing innate lymphoid cells were shown to control the dissemination of commensal bacteria and prevent systemic inflammation in naïve mice, which correlates with chronic infectious diseases in humans, particularly in patients undergoing prolonged treatments that increase their risk for nosocomial infections of bacterial origins (Sonnenberg et al., 2012). However how IL-22 is regulated by various transcriptional factors is not fully understood. Our results show the production of IL-22 in both innate and adaptive lymphoid cells is dependent on STAT3, suggesting that STAT3 inhibition or deficiency may not only increase the chance of pathogen infection in the gut, but also result in the disruption of normal gut homeostasis.
Th22 cells are an important source of IL-22 for host protection against low dose C. rodentium infection (Basu et al., 2012). Here, we carefully utilized a complementary collection of conditional gene deficient mice combined with T cell-specific depletion to show that IL-22 from T cells is not necessary for conferring protection even after high doses of C. rodentium inoculation. Although adoptive transfer of IL-22 producing T cells rescues IL-22 deficient mice from lethal infection (Basu et al., 2012), this suggests that additional IL-22-producing T cells can sufficiently control infection but does not prove that Th22 is essential for the infection. Consistently, exogenous IL-22 can sufficiently rescue many different gene deficient mice from C. rodentium infection (Ota et al., 2011; Qiu et al., 2011; Tumanov et al., 2011; Zheng et al., 2008). Our data suggest that STAT3 and IL-22 from ILCs, but not Th22 cells, are essential for protecting the host in normal physiology state.
It is thought that the development and function of ILCs and Th cells are under the control of corresponding transcription factors (Hoyler et al., 2012; Zhou, 2012). However, our data suggest that the transcriptional regulation of ILC3s and Th17 and Th22 cells is complex, and may be distinct between innate and adaptive lymphocytes. In contrast to the essential role of controlling RORγt expression in T cells (Yang et al., 2007; Zhou et al., 2007), STAT3 has little impact on the development of RORγt+ ILC3s. This finding could explain the major differences in ontogeny between RORγt+ Th cells and ILC3s. ILC3s first appear as fetal LTi cells that are generated on E12.5 during ontogeny in the liver, while RORγt+ Th17 and Th22 cells are differentiated from naive CD4+ T cells after birth dependent on the commensal microbiota (Eberl, 2012). Germ-free mice mostly lack RORγt+ Th cells. Colonization of germ-free mice with segmented filamentous bacterium is sufficient to induce Th17 cells in the small intestinal (Ivanov et al., 2009). In contrast, the development of ILC3s is independent of gut flora, although there are debates about the influence of symbiotic microbiota on some subsets or function of ILC3s (Sanos et al., 2009; Satoh-Takayama et al., 2008; Sawa et al., 2011; Sonnenberg and Artis, 2012). Our data suggest that STAT3 signaling is necessary for the host to sense the environmental microbiota and subsequently generate RORγt+ Th cells, but not to “program” the expression of RORγt in microbiota-independent ILCs. Thus, the mechanisms underlying the differential regulation RORγt expression by STAT3 in ILCs and T cells remain to be defined.
STAT3 is one of the downstream adaptors of IL-23R signaling and regulates the expression of RORγt, Ahr, and IL-23R in T cells (Ghoreschi et al., 2010; Ivanov et al., 2006; Veldhoen et al., 2008). Presumably, IL-23 activates the STAT3 signaling, which induces RORγt, Ahr, and IL-23R expression, then subsequent induction of RORγt and Ahr regulates IL-22 production. However, our data show that the STAT3 deficiency in ILC3s has minimal impact on the expression of RORγt, Ahr and IL-23R, but rather activated STAT3 directly binds to the Il22 locus to regulate IL-22 transcription, suggesting that the expression of RORγt in ILC3s is not sufficient to promote IL-22 production in the absence of STAT3. Both RORγt and STAT3 are required for IL-22 production, and RORγt regulating IL-22 production is dependent on STAT3 signaling in ILCs, raising the questions whether RORγt controls STAT3 signaling or how STAT3 and RORγt cooperate on regulating IL-22 production in ILCs.
Together, our studies have demonstrated several important findings. 1) In contrast to the essential role of controlling the expression of RORγt in T cells, STAT3 has little impact on the development of RORγt+ ILC3s, but rather directly regulates the function of innate RORγt+ ILC3s, which is essential for gut immunity. 2) Although STAT3 regulates the function of both innate and adaptive RORγt+ cells, our data suggest that STAT3 signaling and IL-22 from innate RORγt+ ILC3s but not from adaptive T cells, are essential in the response to acute mucosal bacterial infections. 3) Previous studies have shown that STAT3 signaling controls IL-22 production by T cells through regulating RORγt expression. Our data show that STAT3 can directly control IL-22 production. 4) STAT3 inhibition in a major anti-tumor therapy also impairs the host protection from intestinal bacterial infection. Exogenous IL-22 administration may be beneficial for reducing side effects in the cancer patients undergoing STAT3 inhibitor treatment.
EXPERIMENTAL PROCEDURES
Mice
C57BL/6 and Rag1−/− mice were purchased from Harland Teklad. Cd4-Cre (Lee et al., 2001) mice were purchased from Taconic. Rorcgfp/+ (Eberl et al., 2004) mice were purchased from The Jackson Lab. Rorc-cre-Stat3fl/fl, Rorc-cre-Stat3fl/+, and Cd4-cre-Stat3fl/fl mice were generated by crossing Stat3-floxed mice (Takeda et al., 1998) with Rorc-cre (Eberl and Littman, 2004) and Cd4-cre transgenic mice, respectively. Rorc-cre-Stat3fl/fl Rorcgfp/+ mice were generated by crossing Rorc-cre-Stat3fl/fl mice with Rorcgfp/+ mice. All mice are on C57BL/6 background. Animal care and use were in accordance with institutional and National Institutes of Health guidelines and all studies were approved by the Animal Care and Use Committee of the University of Chicago and Northwestern University.
Infection with C. rodentium and Treatment
Mice were orally gavaged with C. rodentium strain DBS100 (ATCC 51459; American Type Culture Collection) and body weight, survival, colony-forming units (CFUs) counts, tissue histology were assessed as previously described (Tumanov et al., 2011; Wang et al., 2010). Colon culture was done as previously described (Zheng et al., 2008). Where indicated, Sunitinib (SU11248; SUTENT) or control was gavaged orally, once a day, at doses of 4 or 40 mg/Kg body weight after infection. Where indicated, mice were injected intraperitoneally with mouse IL-22-Ig fusion protein on 0, 2, 4 day after C. rodentium infection at a dose of 50 μg per mouse each time. Control groups received isotype control immunoglobulin. For depletion of CD4+ T lymphocytes, mice were injected intraperitoneally with mAb GK1.5 or Rat IgG on −5, 0, 5, 10, 15 day after C. rodentium infection at a dose of 50 μg per mouse each time. Where indicated, IL-17 and IL-22-expressing plasmids were introduced into mice using a hydrodynamic tail vein injection-based gene transfer technique (Tumanov et al., 2011).
Isolation of Intestinal LPLs
The isolation of intestinal lamina propria cells was done as previously described (Wang et al., 2010). In brief, mice were killed and colons were removed and placed in ice-cold PBS. The intestines were cut open longitudinally, thoroughly washed in ice-cold PBS, and cut into 1.5 cm pieces. Intestines were incubated with shaking in RPMI 1640 (Invitrogen) containing 3% FBS, 1mM DTT, 5 mM EDTA and 10 mM HEPES at 37°C for 30 min. After washing with RPMI 1640 containing 2 mM EDTA, the tissues were then digested in RPMI 1640 containing 0.05% DNase I (Sigma) and Liberase (Roche) (0.1 mg/ml) at 37°C for 30 min with slow rotation. The digested tissues were homogenized by vigorous shaking and passed through 100 μm cell strainer. Mononuclear cells were then harvested from the interphase of an 80% and 40% Percoll gradient after a spin at 2500 rpm for 20 min at room temperature.
Flow Cytometry, Antibodies and ELISA
Antibodies against CD3, CD4, CD8, CD45, CD90, Nkp46, Gr-1, CD11b, KLRG1, TCRβ, NK1.1 and Streptavidin-APC were purchased from BioLegend. Antibodies against RORγt and IL-17 were purchased from eBioscience. Antibodies against STAT3 and Rabbit IgG were purchased from Cell Signaling Technology. Anti-IL-22 antibody was a gift from Genentech. For nuclear staining, cells were fixed and permeabilized using a Transcription Factor Staining Buffer Set (eBioscience). For cytokine production, cells were stimulated ex vivo by IL-23 (25 ng/ml, R&D), PMA (50 ng/ml, Sigma) and ionomycin (750 ng/ml, Calbiochem) or anti-CD3 (plate coated, 1 μg/ml) and anti-CD28 (2 μg/ml) for 4 hr and Brefeldin A (10 μg/ml, eBioscience) was added 2 hr before cells were harvested for analysis. IC Fixation Buffer and Permeabilization Buffer were used according to the manufacturer’s instructions (eBioscience) for intracellular cytokine staining. Flow cytometry analysis was performed on FACSCanto (BD Biosciences) instruments and analyzed with FlowJo software (Tree Star Inc.). IL-22 (R&D Systems), IL-17, IL-23 (eBioscience) in supernatants were measured by ELISA according to the manufacturer’s recommendations.
Quantitative Real-Time RT-PCR
RNA from tissues was isolated with Trizol reagent (Invitrogen) and reverse transcribed with AMV Reverse Transcriptase (Promega). RNA from sorted cells was isolated with the RNeasy Micro Kit (Qiagen) and was reverse transcribed with Seniscript Reverse Transcription Kit (Qiagen). Real-time RT-PCR was performed using SSoFast EvaGreen supermix (Bio-Rad) and different primer sets (Table S1) on StepOne Plus (Applied Biosystems). Samples were normalized to HPRT and reported according to the 2−ΔΔCT method.
Adoptive Transfer Experiments
Rag1−/− mice were infected with C. rodentium. CD90hiCD45lo ILC3s were sorted from both the colon and small intestine LPLs on a FACS Aria III instrument (BD Bioscience) at day 1 post infection. Sorted ILC3s were stimulated with IL-23 (25 ng/ml) for 1 hour, then were transferred by i.v. injection (2 × 105 cells per mouse) into recipients inoculated with C. rodentium at day 0 and day 3.
Chromatin Immunoprecipitation
ChIP assays with EL4 cells were performed as previously described (Qiu et al., 2011). Briefly, 5×107 EL4 cells were first fixed in 1% formaldehyde in RPMI1640 media for 10 min at room temperature. Then the reaction was stopped by adding glycine solution. Fixed cells were washed with PBS and resuspended in lysis buffer. Chromatin was sheared by sonication on ice, and the insoluble fraction was removed by centrifugation. The lysate was precleared with Protein A beads (Santa Cruz Biotechnology) and then incubated with anti-Flag beads (Sigma) at 4°C for 5 hrs. After washing, precipitated chromatin fragments were eluted with flag peptide (Sigma). Then the samples were reverse cross-linked at 65°C by adding Tris-EDTA with 1% SDS. After proteinase K digestion, DNA was extracted using Phenol-Chloroform, and precipitated for quantitative real-time PCR analyses using specific primers. CD90hiCD45lo ILC3s were sorted from Rag1−/− mice and ChIP assays were performed with anti-STAT3 rabbit mAb (D3Z2G) or Rabbit IgG (Cell Signaling Technology). MegnaChIP protein A magnetic beads (Millipore) were used to isolate immune complexes. Crosslinks were reversed by heating at 65ting at 65rosslproteinase K treatment. DNA was purified with Qiaquick PCR purification kit (QIAGEN).
Statistical Methods
Statistical analysis was performed by two-tailed Student’s t test using GraphPad Prism 5.0 program. Data from such experiments are presented as mean values ± SEM; p < 0.05 was considered significant. For survival curves, statistics were done using the log rank (Mantel-Cox) test.
Acknowledgments
We are grateful to H. Yu (Beckman Research Institute of City of Hope, CA) for Stat3-floxed mice and Sunitinib (SU11248; SUTENT); D. Littman and I. Ivanov (New York University, NY) for Rorc-cre mice; W. Ouyang (Genentech, CA) for IL-22-expressing plasmid, IL-22-Ig and anti-IL-22 antibody; C. Dong (MD Anderson Cancer Center, TX) for IL-17 plasmid; V. Krishnamoorthy (University of Chicago, IL) for ChIP protocol; S. Kron and J. Cunningham (University of Chicago, IL) for sharing sonicator. The work was supported by the National Institutes of Health grants (AI 089954 and AI 091962 to L.Z., DK080736 and CA141975 to Y.X.F.), by a Pew Scholarship and a Cancer Research Institute Investigator Award (to L.Z).
Footnotes
Supplemental Information includes six figures, one table and Supplemental Experimental Procedures.
Publisher's Disclaimer: This is a PDF file of an unedited manuscript that has been accepted for publication. As a service to our customers we are providing this early version of the manuscript. The manuscript will undergo copyediting, typesetting, and review of the resulting proof before it is published in its final citable form. Please note that during the production process errors may be discovered which could affect the content, and all legal disclaimers that apply to the journal pertain.
References
- Basu R, O’Quinn DB, Silberger DJ, Schoeb TR, Fouser L, Ouyang W, Hatton RD, Weaver CT. Th22 cells are an important source of IL-22 for host protection against enteropathogenic bacteria. Immunity. 2012;37:1061–1075. [Europe PMC free article] [Abstract] [Google Scholar]
- Bry L, Brenner MB. Critical role of T cell-dependent serum antibody, but not the gut-associated lymphoid tissue, for surviving acute mucosal infection with Citrobacter rodentium, an attaching and effacing pathogen. J Immunol. 2004;172:433–441. [Abstract] [Google Scholar]
- Bry L, Brigl M, Brenner MB. CD4+-T-cell effector functions and costimulatory requirements essential for surviving mucosal infection with Citrobacter rodentium. Infect Immun. 2006;74:673–681. [Europe PMC free article] [Abstract] [Google Scholar]
- Eberl G. Development and evolution of RORgammat+ cells in a microbe’s world. Immunol Rev. 2012;245:177–188. [Abstract] [Google Scholar]
- Eberl G, Littman DR. Thymic origin of intestinal alphabeta T cells revealed by fate mapping of RORgammat+ cells. Science. 2004;305:248–251. [Abstract] [Google Scholar]
- Eberl G, Marmon S, Sunshine MJ, Rennert PD, Choi Y, Littman DR. An essential function for the nuclear receptor RORgamma(t) in the generation of fetal lymphoid tissue inducer cells. Nat Immunol. 2004;5:64–73. [Abstract] [Google Scholar]
- Fuchs A, Vermi W, Lee JS, Lonardi S, Gilfillan S, Newberry RD, Cella M, Colonna M. Intraepithelial Type 1 Innate Lymphoid Cells Are a Unique Subset of IL-12- and IL-15-Responsive IFN-gamma-Producing Cells. Immunity 2013 [Europe PMC free article] [Abstract] [Google Scholar]
- Furusawa J, Moro K, Motomura Y, Okamoto K, Zhu J, Takayanagi H, Kubo M, Koyasu S. Critical role of p38 and GATA3 in natural helper cell function. J Immunol. 2013;191:1818–1826. [Europe PMC free article] [Abstract] [Google Scholar]
- Ghoreschi K, Laurence A, Yang XP, Tato CM, McGeachy MJ, Konkel JE, Ramos HL, Wei L, Davidson TS, Bouladoux N, et al. Generation of pathogenic T(H)17 cells in the absence of TGF-beta signalling. Nature. 2010;467:967–971. [Europe PMC free article] [Abstract] [Google Scholar]
- Gordon SM, Chaix J, Rupp LJ, Wu J, Madera S, Sun JC, Lindsten T, Reiner SL. The transcription factors T-bet and Eomes control key checkpoints of natural killer cell maturation. Immunity. 2012;36:55–67. [Europe PMC free article] [Abstract] [Google Scholar]
- Hoyler T, Klose CS, Souabni A, Turqueti-Neves A, Pfeifer D, Rawlins EL, Voehringer D, Busslinger M, Diefenbach A. The Transcription Factor GATA-3 Controls Cell Fate and Maintenance of Type 2 Innate Lymphoid Cells. Immunity. 2012;37:634–648. [Europe PMC free article] [Abstract] [Google Scholar]
- Hutchins AP, Poulain S, Miranda-Saavedra D. Genome-wide analysis of STAT3 binding in vivo predicts effectors of the anti-inflammatory response in macrophages. Blood. 2012;119:e110–119. [Abstract] [Google Scholar]
- Ishigame H, Kakuta S, Nagai T, Kadoki M, Nambu A, Komiyama Y, Fujikado N, Tanahashi Y, Akitsu A, Kotaki H, et al. Differential roles of interleukin-17A and -17F in host defense against mucoepithelial bacterial infection and allergic responses. Immunity. 2009;30:108–119. [Abstract] [Google Scholar]
- Ivanov II, Atarashi K, Manel N, Brodie EL, Shima T, Karaoz U, Wei D, Goldfarb KC, Santee CA, Lynch SV, et al. Induction of intestinal Th17 cells by segmented filamentous bacteria. Cell. 2009;139:485–498. [Europe PMC free article] [Abstract] [Google Scholar]
- Ivanov II, McKenzie BS, Zhou L, Tadokoro CE, Lepelley A, Lafaille JJ, Cua DJ, Littman DR. The orphan nuclear receptor RORgammat directs the differentiation program of proinflammatory IL-17+ T helper cells. Cell. 2006;126:1121–1133. [Abstract] [Google Scholar]
- Klein Wolterink RG, Serafini N, van Nimwegen M, Vosshenrich CA, de Bruijn MJ, Fonseca Pereira D, Veiga Fernandes H, Hendriks RW, Di Santo JP. Essential, dose-dependent role for the transcription factor Gata3 in the development of IL-5+ and IL-13+ type 2 innate lymphoid cells. Proc Natl Acad Sci U S A. 2013;110:10240–10245. [Europe PMC free article] [Abstract] [Google Scholar]
- Laurence A, Tato CM, Davidson TS, Kanno Y, Chen Z, Yao Z, Blank RB, Meylan F, Siegel R, Hennighausen L, et al. Interleukin-2 signaling via STAT5 constrains T helper 17 cell generation. Immunity. 2007;26:371–381. [Abstract] [Google Scholar]
- Lee PP, Fitzpatrick DR, Beard C, Jessup HK, Lehar S, Makar KW, Perez-Melgosa M, Sweetser MT, Schlissel MS, Nguyen S, et al. A critical role for Dnmt1 and DNA methylation in T cell development, function, and survival. Immunity. 2001;15:763–774. [Abstract] [Google Scholar]
- Maaser C, Housley MP, Iimura M, Smith JR, Vallance BA, Finlay BB, Schreiber JR, Varki NM, Kagnoff MF, Eckmann L. Clearance of Citrobacter rodentium requires B cells but not secretory immunoglobulin A (IgA) or IgM antibodies. Infect Immun. 2004;72:3315–3324. [Europe PMC free article] [Abstract] [Google Scholar]
- Minegishi Y, Saito M, Tsuchiya S, Tsuge I, Takada H, Hara T, Kawamura N, Ariga T, Pasic S, Stojkovic O, et al. Dominant-negative mutations in the DNA-binding domain of STAT3 cause hyper-IgE syndrome. Nature. 2007;448:1058–1062. [Abstract] [Google Scholar]
- Mjosberg J, Bernink J, Golebski K, Karrich JJ, Peters CP, Blom B, te Velde AA, Fokkens WJ, van Drunen CM, Spits H. The transcription factor GATA3 is essential for the function of human type 2 innate lymphoid cells. Immunity. 2012;37:649–659. [Abstract] [Google Scholar]
- Moro K, Yamada T, Tanabe M, Takeuchi T, Ikawa T, Kawamoto H, Furusawa J, Ohtani M, Fujii H, Koyasu S. Innate production of T(H)2 cytokines by adipose tissue-associated c-Kit(+)Sca-1(+) lymphoid cells. Nature. 2010;463:540–544. [Abstract] [Google Scholar]
- O’Shea JJ, Lahesmaa R, Vahedi G, Laurence A, Kanno Y. Genomic views of STAT function in CD4+ T helper cell differentiation. Nat Rev Immunol. 2011;11:239–250. [Europe PMC free article] [Abstract] [Google Scholar]
- Ota N, Wong K, Valdez PA, Zheng Y, Crellin NK, Diehl L, Ouyang W. IL-22 bridges the lymphotoxin pathway with the maintenance of colonic lymphoid structures during infection with Citrobacter rodentium. Nat Immunol. 2011;12:941–948. [Abstract] [Google Scholar]
- Page BD, Ball DP, Gunning PT. Signal transducer and activator of transcription 3 inhibitors: a patent review. Expert Opin Ther Pat. 2011;21:65–83. [Abstract] [Google Scholar]
- Pickert G, Neufert C, Leppkes M, Zheng Y, Wittkopf N, Warntjen M, Lehr HA, Hirth S, Weigmann B, Wirtz S, et al. STAT3 links IL-22 signaling in intestinal epithelial cells to mucosal wound healing. J Exp Med. 2009;206:1465–1472. [Europe PMC free article] [Abstract] [Google Scholar]
- Qiu J, Heller JJ, Guo X, Chen ZM, Fish K, Fu YX, Zhou L. The aryl hydrocarbon receptor regulates gut immunity through modulation of innate lymphoid cells. Immunity. 2011;36:92–104. [Europe PMC free article] [Abstract] [Google Scholar]
- Sanos SL, Bui VL, Mortha A, Oberle K, Heners C, Johner C, Diefenbach A. RORgammat and commensal microflora are required for the differentiation of mucosal interleukin 22-producing NKp46+ cells. Nat Immunol. 2009;10:83–91. [Europe PMC free article] [Abstract] [Google Scholar]
- Satoh-Takayama N, Vosshenrich CA, Lesjean-Pottier S, Sawa S, Lochner M, Rattis F, Mention JJ, Thiam K, Cerf-Bensussan N, Mandelboim O, et al. Microbial flora drives interleukin 22 production in intestinal NKp46+ cells that provide innate mucosal immune defense. Immunity. 2008;29:958–970. [Abstract] [Google Scholar]
- Sawa S, Lochner M, Satoh-Takayama N, Dulauroy S, Berard M, Kleinschek M, Cua D, Di Santo JP, Eberl G. RORgammat+ innate lymphoid cells regulate intestinal homeostasis by integrating negative signals from the symbiotic microbiota. Nat Immunol. 2011;12:320–326. [Abstract] [Google Scholar]
- Schwandt A, Wood LS, Rini B, Dreicer R. Management of side effects associated with sunitinib therapy for patients with renal cell carcinoma. Onco Targets Ther. 2009;2:51–61. [Europe PMC free article] [Abstract] [Google Scholar]
- Sonnenberg GF, Artis D. Innate lymphoid cell interactions with microbiota: implications for intestinal health and disease. Immunity. 2012;37:601–610. [Europe PMC free article] [Abstract] [Google Scholar]
- Sonnenberg GF, Monticelli LA, Alenghat T, Fung TC, Hutnick NA, Kunisawa J, Shibata N, Grunberg S, Sinha R, Zahm AM, et al. Innate lymphoid cells promote anatomical containment of lymphoid-resident commensal bacteria. Science. 2012;336:1321–1325. [Europe PMC free article] [Abstract] [Google Scholar]
- Sonnenberg GF, Monticelli LA, Elloso MM, Fouser LA, Artis D. CD4(+) lymphoid tissue-inducer cells promote innate immunity in the gut. Immunity. 2011;34:122–134. [Europe PMC free article] [Abstract] [Google Scholar]
- Spits H, Artis D, Colonna M, Diefenbach A, Di Santo JP, Eberl G, Koyasu S, Locksley RM, McKenzie AN, Mebius RE, et al. Innate lymphoid cells--a proposal for uniform nomenclature. Nat Rev Immunol. 2013;13:145–149. [Abstract] [Google Scholar]
- Spits H, Cupedo T. Innate lymphoid cells: emerging insights in development, lineage relationships, and function. Annu Rev Immunol. 2012;30:647–675. [Abstract] [Google Scholar]
- Spits H, Di Santo JP. The expanding family of innate lymphoid cells: regulators and effectors of immunity and tissue remodeling. Nat Immunol. 2010;12:21–27. [Abstract] [Google Scholar]
- Szabo SJ, Kim ST, Costa GL, Zhang X, Fathman CG, Glimcher LH. A novel transcription factor, T-bet, directs Th1 lineage commitment. Cell. 2000;100:655–669. [Abstract] [Google Scholar]
- Takeda K, Kaisho T, Yoshida N, Takeda J, Kishimoto T, Akira S. Stat3 activation is responsible for IL-6-dependent T cell proliferation through preventing apoptosis: generation and characterization of T cell-specific Stat3-deficient mice. J Immunol. 1998;161:4652–4660. [Abstract] [Google Scholar]
- Tumanov AV, Koroleva EP, Guo X, Wang Y, Kruglov A, Nedospasov S, Fu YX. Lymphotoxin controls the IL-22 protection pathway in gut innate lymphoid cells during mucosal pathogen challenge. Cell Host Microbe. 2011;10:44–53. [Europe PMC free article] [Abstract] [Google Scholar]
- Veldhoen M, Hirota K, Westendorf AM, Buer J, Dumoutier L, Renauld JC, Stockinger B. The aryl hydrocarbon receptor links TH17-cell-mediated autoimmunity to environmental toxins. Nature. 2008;453:106–109. [Abstract] [Google Scholar]
- Wang Y, Koroleva EP, Kruglov AA, Kuprash DV, Nedospasov SA, Fu YX, Tumanov AV. Lymphotoxin beta receptor signaling in intestinal epithelial cells orchestrates innate immune responses against mucosal bacterial infection. Immunity. 2010;32:403–413. [Europe PMC free article] [Abstract] [Google Scholar]
- Xin H, Zhang C, Herrmann A, Du Y, Figlin R, Yu H. Sunitinib inhibition of Stat3 induces renal cell carcinoma tumor cell apoptosis and reduces immunosuppressive cells. Cancer Res. 2009;69:2506–2513. [Europe PMC free article] [Abstract] [Google Scholar]
- Yang XO, Panopoulos AD, Nurieva R, Chang SH, Wang D, Watowich SS, Dong C. STAT3 regulates cytokine-mediated generation of inflammatory helper T cells. J Biol Chem. 2007;282:9358–9363. [Abstract] [Google Scholar]
- Yang XO, Pappu BP, Nurieva R, Akimzhanov A, Kang HS, Chung Y, Ma L, Shah B, Panopoulos AD, Schluns KS, et al. T helper 17 lineage differentiation is programmed by orphan nuclear receptors ROR alpha and ROR gamma. Immunity. 2008;28:29–39. [Europe PMC free article] [Abstract] [Google Scholar]
- Yang XP, Ghoreschi K, Steward-Tharp SM, Rodriguez-Canales J, Zhu J, Grainger JR, Hirahara K, Sun HW, Wei L, Vahedi G, et al. Opposing regulation of the locus encoding IL-17 through direct, reciprocal actions of STAT3 and STAT5. Nat Immunol. 2011;12:247–254. [Europe PMC free article] [Abstract] [Google Scholar]
- Yu H, Kortylewski M, Pardoll D. Crosstalk between cancer and immune cells: role of STAT3 in the tumour microenvironment. Nat Rev Immunol. 2007;7:41–51. [Abstract] [Google Scholar]
- Zheng W, Flavell RA. The transcription factor GATA-3 is necessary and sufficient for Th2 cytokine gene expression in CD4 T cells. Cell. 1997;89:587–596. [Abstract] [Google Scholar]
- Zheng Y, Valdez PA, Danilenko DM, Hu Y, Sa SM, Gong Q, Abbas AR, Modrusan Z, Ghilardi N, de Sauvage FJ, et al. Interleukin-22 mediates early host defense against attaching and effacing bacterial pathogens. Nat Med. 2008;14:282–289. [Abstract] [Google Scholar]
- Zhou L. Striking similarity: GATA-3 regulates ILC2 and Th2 cells. Immunity. 2012;37:589–591. [Europe PMC free article] [Abstract] [Google Scholar]
- Zhou L, Ivanov II, Spolski R, Min R, Shenderov K, Egawa T, Levy DE, Leonard WJ, Littman DR. IL-6 programs T(H)-17 cell differentiation by promoting sequential engagement of the IL-21 and IL-23 pathways. Nat Immunol. 2007;8:967–974. [Abstract] [Google Scholar]
Full text links
Read article at publisher's site: https://doi.org/10.1016/j.immuni.2013.10.021
Read article for free, from open access legal sources, via Unpaywall:
http://www.cell.com/article/S1074761313005554/pdf
Citations & impact
Impact metrics
Citations of article over time
Alternative metrics
Smart citations by scite.ai
Explore citation contexts and check if this article has been
supported or disputed.
https://scite.ai/reports/10.1016/j.immuni.2013.10.021
Article citations
GPX4 restricts ferroptosis of NKp46+ILC3s to control intestinal inflammation.
Cell Death Dis, 15(9):687, 19 Sep 2024
Cited by: 0 articles | PMID: 39300068 | PMCID: PMC11413021
Sirtuin 6 inhibits group 3 innate lymphoid cell function and gut immunity by suppressing IL-22 production.
Front Immunol, 15:1402834, 26 Aug 2024
Cited by: 0 articles | PMID: 39253083 | PMCID: PMC11381250
Nucleophosmin 1 promotes mucosal immunity by supporting mitochondrial oxidative phosphorylation and ILC3 activity.
Nat Immunol, 25(9):1565-1579, 05 Aug 2024
Cited by: 0 articles | PMID: 39103576 | PMCID: PMC11362010
OLFM4 modulates intestinal inflammation by promoting IL-22+ILC3 in the gut.
Commun Biol, 7(1):914, 29 Jul 2024
Cited by: 0 articles | PMID: 39075283 | PMCID: PMC11286877
LGG promotes activation of intestinal ILC3 through TLR2 receptor and inhibits salmonella typhimurium infection in mice.
Virulence, 15(1):2384553, 30 Jul 2024
Cited by: 0 articles | PMID: 39080852 | PMCID: PMC11296546
Go to all (169) article citations
Data
Data behind the article
This data has been text mined from the article, or deposited into data resources.
BioStudies: supplemental material and supporting data
Similar Articles
To arrive at the top five similar articles we use a word-weighted algorithm to compare words from the Title and Abstract of each citation.
The aryl hydrocarbon receptor regulates gut immunity through modulation of innate lymphoid cells.
Immunity, 36(1):92-104, 15 Dec 2011
Cited by: 551 articles | PMID: 22177117 | PMCID: PMC3268875
Microbial flora drives interleukin 22 production in intestinal NKp46+ cells that provide innate mucosal immune defense.
Immunity, 29(6):958-970, 11 Dec 2008
Cited by: 755 articles | PMID: 19084435
DOCK8 regulates protective immunity by controlling the function and survival of RORγt+ ILCs.
Nat Commun, 5:4603, 05 Aug 2014
Cited by: 26 articles | PMID: 25091235 | PMCID: PMC4135384
Heterogeneity and diversity of group 3 innate lymphoid cells: new cells on the block.
Int Immunol, 28(1):29-34, 13 Oct 2015
Cited by: 9 articles | PMID: 26462712
Review
Funding
Funders who supported this work.
NCI NIH HHS (4)
Grant ID: P30 CA060553
Grant ID: R01 CA134563
Grant ID: R01 CA141975
Grant ID: CA141975
NIAID NIH HHS (4)
Grant ID: R01 AI089954
Grant ID: AI 091962
Grant ID: R21 AI091962
Grant ID: AI 089954
NIDDK NIH HHS (3)
Grant ID: R01 DK105562
Grant ID: R01 DK080736
Grant ID: DK080736