Abstract
Background/aims
Although indoor air pollution is a well-known risk factor for tuberculosis (TB), the possible link between outdoor air pollution and TB development has not been examined fully. We assessed the impact of outdoor air pollution on TB development in the Seoul metropolitan area, South Korea.Methods
The mean concentrations of ambient particulate matter (PM) with an aerodynamic diameter ≤ 10 µm (PM10), O3, CO, NO2, and SO2 levels in Seoul, between January 1, 1997 and December 31, 2006, were determined. Furthermore, their association with the risk of developing TB after adjusting for socioeconomic status, between January 1, 2002 and December 31, 2006, was investigated.Results
Between January 1, 2002 and December 31, 2006, a total of 41,185 TB cases were reported in Seoul. Concentrations of PM10, O3, CO, and NO2 were not associated with TB incidence in males or females. However, the interquartile increase in SO2 concentration was associated with a 7% increment in TB incidence (relative risk [RR], 1.07; 95% credible interval [CrI], 1.03 to 1.12) in males but not in females (RR, 1.02; 95% CrI, 0.98 to 1.07).Conclusions
Long-term exposure to ambient SO2 increased the risk of TB in males.Free full text

Impact of outdoor air pollution on the incidence of tuberculosis in the Seoul metropolitan area, South Korea
Abstract
Background/Aims
Although indoor air pollution is a well-known risk factor for tuberculosis (TB), the possible link between outdoor air pollution and TB development has not been examined fully. We assessed the impact of outdoor air pollution on TB development in the Seoul metropolitan area, South Korea.
Methods
The mean concentrations of ambient particulate matter (PM) with an aerodynamic diameter ≤ 10 µm (PM10), O3, CO, NO2, and SO2 levels in Seoul, between January 1, 1997 and December 31, 2006, were determined. Furthermore, their association with the risk of developing TB after adjusting for socioeconomic status, between January 1, 2002 and December 31, 2006, was investigated.
Results
Between January 1, 2002 and December 31, 2006, a total of 41,185 TB cases were reported in Seoul. Concentrations of PM10, O3, CO, and NO2 were not associated with TB incidence in males or females. However, the interquartile increase in SO2 concentration was associated with a 7% increment in TB incidence (relative risk [RR], 1.07; 95% credible interval [CrI], 1.03 to 1.12) in males but not in females (RR, 1.02; 95% CrI, 0.98 to 1.07).
Conclusions
Long-term exposure to ambient SO2 increased the risk of TB in males.
INTRODUCTION
Tuberculosis (TB) is an infectious disease caused by the bacillus Mycobacterium tuberculosis. It typically affects the lungs but can affect other sites. TB is one of the leading causes of mortality and morbidity worldwide. In 2010, there were 8.8 million cases of TB, 1.1 million deaths from TB among human immunodeficiency virus (HIV)-negative people, and an additional 0.35 million deaths from HIV-associated TB [1]. Despite rapid economic development in South Korea, TB remains an important health issue in the country. Nationwide, 33,167 TB cases were reported in 2010. The prevalence and annual incidence of TB in South Korea are currently estimated to be 151/100,000 and 97/100,000, respectively [1].
Indoor air pollution is a well-known risk factor for TB development. The association between indoor air pollution and TB was suggested as early as 1911. Observations between 1858 and 1902 in Paris that TB mortality was inversely associated with the number of windows per household were the first suggestion of a connection [2]. Since then, many studies showing an association between indoor air pollution and TB have been published. For example, among residents of Mexico City, cooking with a biomass stove was associated with a 2.4-times higher odds of developing TB [3]. One recent meta-analysis showed that indoor air pollution increased the incidence of pulmonary TB, with a relative risk (RR) of 1.95 [4].
Despite the well-established relationship between indoor air pollution and TB, the impact of outdoor air pollution on the development of TB has not been examined sufficiently. We assessed the impact of major air pollutants on TB development in the Seoul metropolitan area, South Korea, an intermediate TB burden country.
METHODS
Study design
The study protocol was approved by the Institutional Review Board (IRB) of Seoul National University Hospital. Because this study was performed retrospectively using anonymous data, obtaining informed consent from individual patients was waived by the IRB.
This was a retrospective cohort study involving the entire population of the Seoul metropolitan area. After adjusting for socioeconomic status, the mean concentrations of ambient particulate matter (PM) with an aerodynamic diameter ≤ 10 µm (PM10), O3, CO, NO2, and SO2 between January 1, 1997 and December 31, 2006, were tested as risk factors for the development of TB in Seoul between January 1, 2002 and December 31, 2006.
Population data
Mid-year population data for each year, grouped in 5-year age bands, for each of the 522 Seoul metropolitan area townships were obtained from the National Statistics Office [5]. For township-level socioeconomic status, deprivation indices using the Carstairs Index [6] were assayed for each townships across Seoul. Specifically, we analyzed the township-specific 1) proportion of overcrowded households (more than 1.5 persons/room), 2) percent of unemployment among males between 15 and 64 years, 3) percentage in manual occupations, and 4) lack of car ownership, based on 2005 National Census data. Finally, we generated a township-specific deprivation index by averaging the standardized z-scores for the four census-derived indicators. Subsequently, the townships were classified into five quintiles, based on the deprivation level.
Air pollution exposure estimate
Measurement of air pollutants were obtained from 27 monitoring stations, evenly scattered throughout Seoul and maintained by the Department of the Environment, through the period from January 1, 1997 to December 31, 2006. Each monitoring station provided hourly readings of PM10, O3, CO, NO2, and SO2 concentrations. Using the data from each monitoring station, we estimated the pollutant levels in each township using the ordinary kriging method [7]. We used the Spatial Analyst and Geospatial Analyst extensions of ArcGIS (ArcMap version 9.3, ESRI Inc., Redlands, CA, USA) using 1 × 1 km grids to partition each township for each pollutant. We extracted the concentrations of each pollutant using the central point of the kriging map for each township.
TB incidence
In Korea, physicians who diagnose TB in patients must report this electronically immediately to the Korean Institute of Tuberculosis [8]. The reporting form includes demographic information (e.g., age, gender, address) and clinical characteristics (e.g., past history of TB treatment, site of TB, smear positivity) of TB patients. Annual numbers of reported TB cases in each township were retrieved from that database. Incidences of township TB were calculated from the annual number of TB cases divided by the mid-year population of each township.
Statistical analyses
We calculated standardized incidence ratios (SIRs) for each township using data from January 1, 2002 to December 31, 2006. Expected cases were determined by multiplying the gender- and age-specific TB incidences in 5-year age bands by the corresponding gender- and age-specific population-years at risk in each township. The statistical assumption underlying these estimates is that the observed disease (i.e., TB) counts, yi, in each town area arise from a Poisson distribution with mean riEi, where Ei is the age- and gender-standardized expected number of TB cases in town area i and ri is the RR of disease in that area.
We used a hierarchical Bayesian method to estimate the pollution-specific RR of TB for each gender, adjusted for area deprivation level, using the intrinsic Gaussian conditional autoregressive model. This model controls the spatial autocorrelation component. Relative rates were estimated using Markov Chain Monte Carlo algorithms [9] with WinBUGS version 1.4.3 (MRC Biostatistics Unit, Cambridge, UK), which was called from 'R' version 2.12.0. Parameter means and 95% credible intervals (CrIs) were calculated from five independent chains of 50,000 iterations after a burn-in of the first 20,000 iterations. The convergence of posterior distribution was assessed with the Gelman-Rubin convergence diagnostic [10], and models were compared based on the deviance information criterion.
RESULTS
Air pollutant concentrations
Descriptive statistics for the air-quality variables are presented in Table 1. The average concentrations were 63.5 µg/m3 particulate matter (PM10), 16.0 ppb ozone (O3), 77.4 ppb carbon monoxide (CO), 34.4 ppb nitrogen dioxide (NO2), and 6.1 ppb sulfur dioxide (SO2). Of these, the annual averages of PM10 and NO2 were higher than the Korean national standard (PM10 ≤ 50 µg/m3 and NO2 ≤ 0.03 ppm) [11].
Table 1
Annual air pollutant concentrations from January 1, 1997 through December 2, 2006, in Seoul

National standards for each pollutant set by the Ministry of the Environment [11].
PM10 ≤ 50 µg/m3 (annual), O3 ≤ 0.6 ppm (over 8 hours), CO ≤ 9 ppm (over 8 hours), NO2 ≤ 0.03 ppm (annual), SO2 ≤ 0.02 ppm (annual). SD, standard deviation; Min, minimum; Max, maximum; IQR, interquartile range; PM10, particulate matter with an aerodynamic diameter ≤ 10 µm.
Numbers and characteristics of TB cases
Between January 1, 2002 and December 31, 2006, in Seoul, a total of 41,185 TB cases were reported to the Korean Institute of Tuberculosis. Of these, 24,952 (60.6%) were males. The mean age of the male patients was 44.2 years, and that of the female patients was 41.9 years. Of the male patients, 21,048 (84.4%) were new cases with no past history of TB treatment. Of the females, 14,547 (89.6%) were new cases. The proportion of smear-positive TB was 36.8% in males and 29.6% in females (Table 2).
Table 2
Demographic and clinical characteristics of reported tuberculosis cases 2002 to 2006, in Seoul
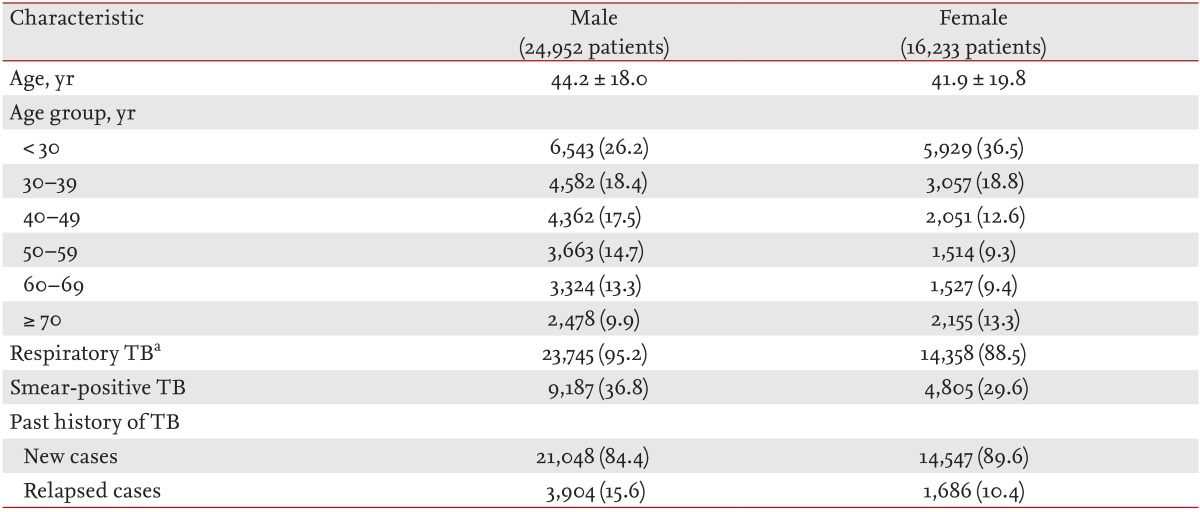
Values are presented as mean ± SD or number (%).
TB, tuberculosis.
aRespiratory TB includes pulmonary TB and TB pleuritis.
The mean male population of each township in 2005 was 9,714 and the mean female population was 9,755. The mean incidence of TB among males in each township was 47.8 and that of females was 31.1. Average SIRs of 522 Seoul metropolitan area towns were calculated as 1.03 for males and 1.02 for females (Table 3).
Table 3
Summary statistics of the distribution of the numbers and unsmoothed standardized incidence ratios of tuberculosis in males and females in Seoul from January 1, 2002 to December 31, 2006
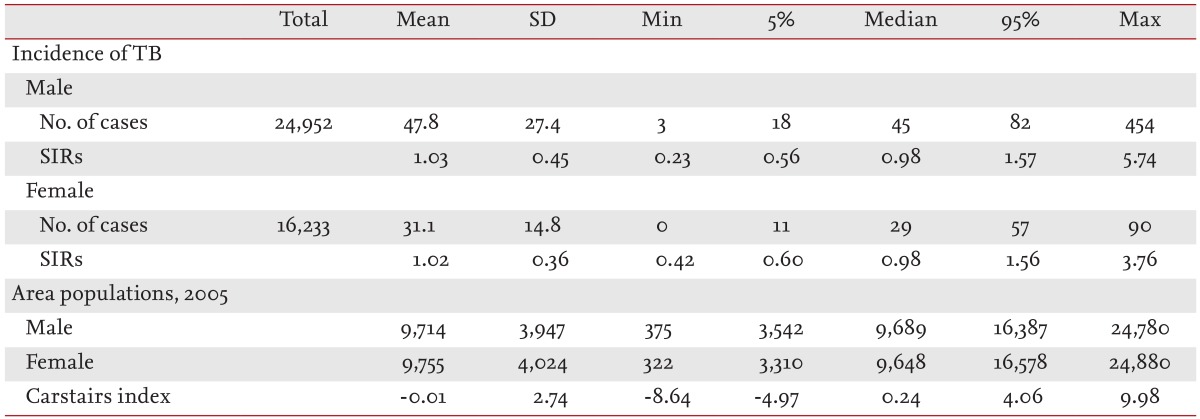
SD, standard deviation; Min, minimum; Max, maximum; TB, tuberculosis; SIRs, standardized incidence ratios.
Impact of air pollutant concentration on TB incidence
PM10, O3, CO, and NO2 concentrations were not associated with the incidence of TB in males or females. However, the interquartile increase in SO2 concentration was associated with a 7% increase in TB incidence (RR, 1.07; 95% CrI, 1.03 to 1.12) in males but not females (RR, 1.02; 95% CrI, 0.98 to 1.07) (Table 4).
Table 4
Impact of an interquartile increase in pollutant concentration on the incidence of tuberculosis

RR, relative risk; CrI, credible interval; PM10, particulate matter with an aerodynamic diameter ≤ 10 µm.
aRRs were adjusted for the quintiles of the Carstairs index as an indicator variable.
Geographic distribution of air pollutants and incidence of TB
Fig. 1 provides a map of the Seoul metropolitan area and the SO2 krigged values between January 1, 1997 and December 31, 2006, using 27 monitoring stations, categorized by deciles. The predicted SO2 concentrations of the central and northern townships were higher than those of other areas. Fig. 2 shows the unsmoothed and smoothed geographical distribution of the incidence of TB in males and females for the period January 1, 2002 to December 31, 2006. There was no spatial cluster of TB incidence; however, the incidence in the southern townships was generally higher than that in other areas.
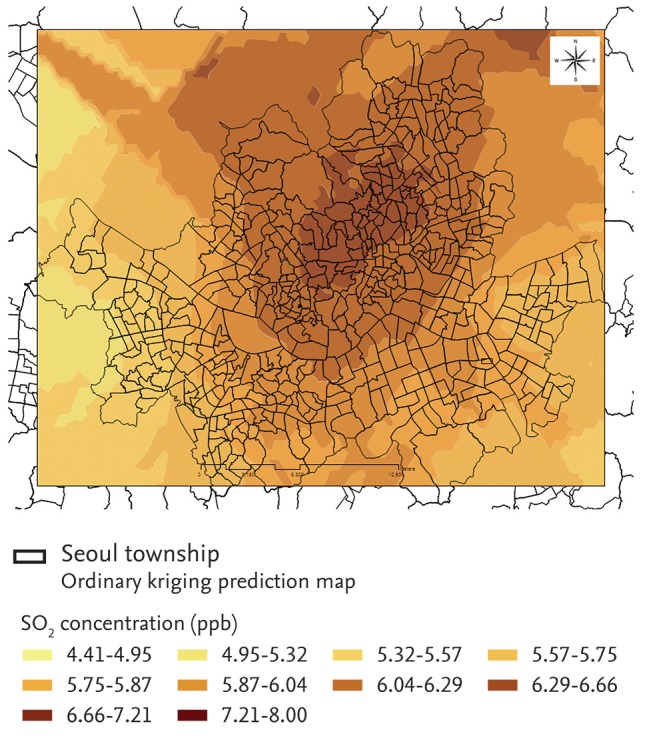
Estimated SO2 levels using air-monitoring data and kriging between January 1, 1997 and December 31, 2006, in the Seoul metropolitan area.
DISCUSSION
Despite the established relationship between indoor air pollution and TB, the impact of outdoor air pollution on the development of TB has not been determined. In this study, by analyzing air pollutant data and formally reported TB cases, we found that annual SO2 level is associated with an increased risk of TB in males in the Seoul metropolitan area, South Korea, a country with an intermediate TB burden.
The group of air pollutants collectively termed "sulfur oxides" comprises both gaseous and particulate chemical species. Four gas-phase compounds of sulfur oxide exist (SO, SO2, SO3, and S2O). Among them, only SO2 is present at sufficient concentrations in ambient air to be a public health concern [12]. Because of its high water solubility, SO2 can be readily scrubbed from inhaled air in the upper airways [13]. Penetration is more efficient during oral, rather than nasal, breathing and during physical activity. Upon contacting airway-covering fluid, SO2 dissolves quickly into the aqueous phase. Subsequently, it readily dissociates into bisulfite and sulfite ions, which can be transferred into the systemic circulation [12,13].
SO2 can affect various aspects of the pulmonary defenses, including alveolar macrophage function, mucociliary transport, and alveolar clearance. In a previous study, in vitro exposure to 12.5 ppm SO2 for 30 minutes induced the death of 62% of alveolar macrophages and caused a 63% decrease in the release of reactive oxygen species [14], which probably play a significant role in intracellular inhibition/killing of mycobacteria [15]. Another study indicated that in vitro exposure to SO2 decreased production or release of tumor necrosis factor-α (TNF-α) and interleukin-1 [16]. TNF-α is crucial for host defenses against M. tuberculosis because it plays a central role in the containment of tuberculous bacilli through granuloma formation [17]. Frequent reactivation of latent TB in patients using a TNF-neutralizing agent underscores the importance of TNF-α in defenses against M. tuberculosis [18]. Thus, the association between SO2 exposure and the development of active TB in our study may have been mediated by the negative effect of SO2 on reactive oxygen intermediate and TNF-α.
In this study, an association between SO2 exposure and TB was observed only in males. This may be due to gender differences in susceptibilities to air pollutants as well as to TB. Indeed, epidemiological studies on the effects of air pollutants on respiratory health have shown significant gender differences [19]. For example, SO2 and PM2.5 had a greater effect on forced expiratory volume in 1 second in males (199 mL) than females (87 mL) [20]. In addition, the association between SO2 exposure and evening peak expiratory flow rate was observed only in boys with asthma [21]. Hypotheses explaining these differences include anatomical/physiological differences in the airway [22], gender-linked hormonal status [23], and other factors (more household tasks performed by females may result in increased exposure to viral infection, indoor allergens, combustion exhaust, cleaning solvents, and aeroallergens) [24].
Furthermore, males seem to be more affected by TB than females, with a male/female ratio of 1.9 ± 0.6 for the worldwide case notification rate. In some countries, this ratio may reach values as high as 3 [25]. The possibility of under-notification of females [26] and poor quality of sputum samples collected from females [27] have been suggested to explain this. However, smoking, alcohol consumption, drug use, exposure to indoor dusts, sex steroid hormones, and the genetic makeup of the sex chromosomes may render males more susceptible to pulmonary TB than females [25]. The results of our study indicate that different susceptibility to outdoor air pollution, especially SO2, may also contribute to gender inequality in TB.
Given the greater prevalence of smoking in males compared to females in South Korea (47.3% vs. 3.1% in 2010) [28], our observation suggests the presence of synergistic effects between SO2 exposure and smoking, which is a risk factor for TB development [29]. In fact, such a synergistic effect on lung function has been reported in a Chinese population [30]. Impaired respiratory defense mechanisms that clear inhaled pollutants [31,32] and changes in respiratory responses [33] caused by smoking may increase the risk of TB development after inhaling M. tuberculosis. However, we could not prove the presence of synergy between SO2 exposure and smoking on the development of TB because of a lack of information on the smoking status of individual TB patients. This is a limitation of our study.
In conclusion, this study provides evidence of an association between outdoor air pollution and an increased risk of TB. Long-term exposure to ambient SO2 increases the risk of TB in males.
KEY MESSAGE
Acknowledgments
This study was supported by grant number 03-2009-0120 from the Seoul National University Hospital Research Fund. The funders had no role in study design, data collection and analysis, decision to publish, or preparation of the manuscript.
References
Articles from The Korean Journal of Internal Medicine are provided here courtesy of Korean Association of Internal Medicine
Full text links
Read article at publisher's site: https://doi.org/10.3904/kjim.2014.29.2.183
Read article for free, from open access legal sources, via Unpaywall:
https://www.kjim.org/upload/kjim-29-2-7_0-0.pdf
Citations & impact
Impact metrics
Article citations
Ecological-level factors associated with tuberculosis incidence and mortality: A systematic review and meta-analysis.
PLOS Glob Public Health, 4(10):e0003425, 15 Oct 2024
Cited by: 0 articles | PMID: 39405319 | PMCID: PMC11478872
The effects of meteorological factors and air pollutants on the incidence of tuberculosis in people living with HIV/AIDS in subtropical Guangxi, China.
BMC Public Health, 24(1):1333, 17 May 2024
Cited by: 1 article | PMID: 38760740
A systematic review and meta-analysis of the association between air pollutants and the incidence of tuberculosis.
Heliyon, 10(8):e28801, 04 Apr 2024
Cited by: 0 articles | PMID: 38638993 | PMCID: PMC11024561
Prevalence of latent tuberculosis infection in Asian nations: A systematic review and meta-analysis.
Immun Inflamm Dis, 12(2):e1200, 01 Feb 2024
Cited by: 3 articles | PMID: 38411377 | PMCID: PMC10898208
Review Free full text in Europe PMC
Impact of diesel exhaust particles on infections with Mycobacterium bovis BCG in in vitro human macrophages and an in vivo Galleria mellonella model.
Environ Pollut, 341:122597, 21 Sep 2023
Cited by: 0 articles | PMID: 37741543 | PMCID: PMC10804993
Go to all (37) article citations
Similar Articles
To arrive at the top five similar articles we use a word-weighted algorithm to compare words from the Title and Abstract of each citation.
Associations of ambient air pollutants with regional pulmonary tuberculosis incidence in the central Chinese province of Hubei: a Bayesian spatial-temporal analysis.
Environ Health, 19(1):51, 14 May 2020
Cited by: 13 articles | PMID: 32410699 | PMCID: PMC7226955
Association of social deprivation and outdoor air pollution with pulmonary tuberculosis in spatiotemporal analysis.
Int J Environ Health Res, 29(6):657-667, 30 Jan 2019
Cited by: 10 articles | PMID: 30698032
Effects of particulate air pollution on tuberculosis development in seven major cities of Korea from 2010 to 2016: methodological considerations involving long-term exposure and time lag.
Epidemiol Health, 42:e2020012, 12 Mar 2020
Cited by: 10 articles | PMID: 32164052 | PMCID: PMC7285441
Ambient Air Pollution Exposures and Newly Diagnosed Pulmonary Tuberculosis in Jinan, China: A Time Series Study.
Sci Rep, 8(1):17411, 27 Nov 2018
Cited by: 12 articles | PMID: 30479352 | PMCID: PMC6258663