Abstract
Free full text

Endothelin and Endothelin Antagonists in Chronic Kidney Disease
Abstract
The incidence and prevalence of chronic kidney disease (CKD), with diabetes and hypertension accounting for the majority of cases, is on the rise, with up to 160 million individuals worldwide predicted to be affected by 2020. Given that current treatment options, primarily targeted at the renin angiotensin system, only modestly slow down progression to end-stage renal disease, the urgent need for additional effective therapeutics is evident. Endothelin-1 (ET-1), largely through activation of endothelin A receptors, has been strongly implicated in renal cell injury, proteinuria, inflammation and fibrosis leading to CKD. Endothelin receptor antagonists (ERAs) have been demonstrated to ameliorate or even reverse renal injury and/or fibrosis in experimental models of CKD, while clinical trials indicate a substantial antiproteinuric effect of ERAs in diabetic and non-diabetic CKD patients even on top of maximal renin angiotensin system blockade. This review summarizes the role of ET in CKD pathogenesis and discusses the potential therapeutic benefit of targeting the ET system in CKD, with attention to the risks and benefits of such an approach.
Chronic kidney disease: A growing need for additional therapies
The global community is witnessing steadily increasing numbers of patients with chronic kidney disease (CKD), with diabetes and hypertension accounting for the majority of cases (1, 2). Up to 11% of the general population of the United States, Australia, Japan and Europe is currently affected, and numbers continue to increase in India, China, and Southeast Asia (3, 4). In view of the continuing obesity/diabetes pandemic and shifts towards older populations around the world, and given that current therapies only partially slow down progression to end-stage renal disease, the urgent need for additional, effective therapeutic agents lacking off-target effects is apparent (1, 4). While multiple potential drug targets are in the development pipeline, the endothelin (ET) system has received particularly high attention. As will be described, the renal ET system is activated in virtually all causes of CKD. In addition, blocking specific ET system pathways holds the promise to be of significant therapeutic benefit in slowing CKD progression. However, due to the potential for side effects, use of endothelin system blockers must be undertaken carefully and judiciously. Herein, we briefly describe the physiology and pathophysiology of the renal ET system, followed by review of clinical experience with ET blockers, their potential side effects, and finally discuss the future therapeutic potential of, and approach to, targeting the ET system in CKD.
The endothelin system in renal physiology
The ET family comprises three 21-amino acid peptides (ET-1, ET-2, and ET-3) of which ET-1 is the most biologically relevant to kidney function in health and disease. While ET-1 was originally described as an endothelium-derived vasoconstrictor (5), it is now evident that the peptide is produced by and acts upon virtually every cell type in the body (6). Endothelins bind to two receptor isoforms, ETA and ETB (6, 7). In general, under healthy conditions, binding to ETA promotes vasoconstriction, cell proliferation and matrix accumulation; ETB activation is vasodilatory, antiproliferative and antifibrotic, however under some pathological conditions, ETB can promote tissue injury and scarring (please see following sections). These effects of ET-1, whether in health or disease, are primarily exerted through local binding, i.e., the peptide acts in an autocrine and/or paracrine manner.
Endogenous renal ET is an important regulator of renal sodium and water excretion (7). Volume loading increases nephron ET-1 production which, largely through autocrine activation of thick ascending limb and collecting duct ETB (leading to production of nitric oxide as well as other signaling molecules), inhibits sodium and water reabsorption (7). Nephron, and particularly collecting duct, ETA also appears to exert a natriuretic effect (8, 9), however the mechanisms by which this occurs remain unclear. Blockade of ET receptors is associated with fluid retention and, as will be described, this side effect has had significant clinical impact. Endothelin receptor antagonists (ERAs) target ETA alone or both ETA and ETB (never just ETB); all clinically used ERAs cause fluid retention. Based on predicted ET-1 actions in the kidney, such fluid retention is perhaps not surprising. In support of a renal cause of fluid retention, recent studies in mice using two different relatively ETA-selective antagonists (atrasentan and ambrisentan) showed that the fluid retention was prevented by either nephron or collecting duct-specific deletion of ETA receptors (8).
Renal ET also modulates other aspects of renal physiology, including total and regional blood flow, mesangial contraction, podocyte function and acid/base handling. Endothelin involvement in renal acid secretion may take on particular relevance in CKD. Acid loading increases renal ET-1 production which, in turn, stimulates proximal and distal nephron proton secretion; blockade of the ET system impairs normal renal acid excretion (10). As will be discussed, acidemia that occurs in the setting of CKD promotes renal ET-1 production that, through promotion of pro-fibrotic pathways, may contribute to progressive deterioration of renal function.
The endothelin system in renal pathophysiology
Endothelin plays an important role in the development of proteinuria, fibrosis, and CKD progression (6). ET-1 promotes cell proliferation, hypertrophy, inflammation and extracellular matrix accumulation, all of which are important factors in progression of CKD (11, 12). Renal ET-1 production increases in conditions associated with renal disease progression, such as diabetes, insulin resistance, obesity, immune system activation, dyslipidemia, reactive oxygen species formation, nitric oxide deficiency and others (reviewed in (11), Figure 1). Infusion of non-pressor doses of ET-1 increases renal cortical inflammation (ICAM-1, MCP-1 and macrophages) (13) as well as podocyte effacement and urinary albumin excretion (14), effects that are largely prevented by concomitant treatment with an ETA antagonist (13, 14). Endothelin-1 also increases formation of other vasoconstrictors and growth factors (such as angiotensin II) (15). In turn, angiotensin II activates renal ET-1 formation (16), thereby creating a positive feedback loop. Of note, ET-1 also appears to be involved in the priming effect of acute ischemic renal injury on future CKD development, and this effect that can be largely prevented by blocking ETA (17).
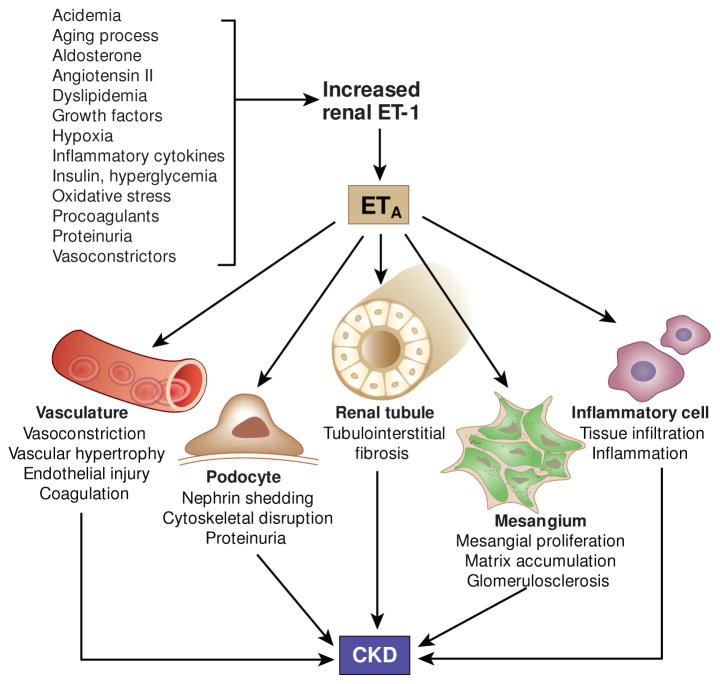
Pathophysiological role of endothelin in CKD development. Intrinsic (aging), physico-chemical (acidemia, hypoxia), biochemical (cytokines, oxidative stress, growth factors, procoagulants), metabolic (insulin, hyperglycemia, dyslipidemia), vasoactive (angiotensin II, aldosterone, vasoconstrictors), and pathological factors (proteinuria) enhance renal endothelin-1 (ET-1) production. CKD development is associated with increased formation of renal ET-1 which - primarily via ETA receptors – promotes renal injury and fibrosis through modulation of multiple renal cell types.
One aspect of ET activity in renal pathophysiology deserving of particular mention is podocyte involvement. Podocyte injury is a hallmark of proteinuric renal diseases and precedes the development of glomerulosclerosis (18, 19). Podocytes and neighboring cells synthesize ET-1, and podocytes express both ETA and ETB (18). In podocytes from humans and experimental animals, ETA has been primarily implicated in mediating cellular injury (18), although preliminary data from the Tharaux laboratory suggest that, at least in mice, activation of podocyte ETB might also cause podocyte dysfunction (20). The mechanisms by which ET-1 contributes to podocyte injury are not fully understood and are likely to be multifactorial. Exposure to ET-1 in vitro disrupts the podocyte actin cytoskeleton (21, 22), while treatment with ERAs prevents disruption of the podocyte actin cytoskeleton in experimental focal segmental glomerulosclerosis (FSGS) (18). Interestingly, exposure of podocytes to protein in vitro (mimicking proteinuria in vivo) induces synthesis of ET-1 and reduces glomerular permselectivity, an effect sensitive to ETA blockade (22, 23). Further, exogenous ET-1 chronically increases glomerular permeability via ETA-mediated mechanisms (14, 24).
Pathogenic role of the endothelin system in experimental chronic kidney disease
General considerations
A direct role for ET-1 in CKD was reported by Hocher et al. who observed that mice systemically overexpressing the human preproendothelin gene developed glomerulosclerosis in the absence of systemic hypertension (25). Benigni et al. first reported substantial reductions in proteinuria and glomerulosclerosis after ERA treatment in a rat renal mass reduction model (26). Since then, numerous preclinical ERA treatment studies have lent much support to the notion that endothelin, primarily via activation of ETA, contributes to renal disease progression under hypertensive as well as normotensive conditions (27).
Diabetic Nephropathy
Diabetes remains the predominant cause of CKD; the prevalence of diabetic nephropathy is likely to increase due to the obesity pandemic. Numerous factors contribute to increased renal ET-1 production in diabetic nephropathy, however hyperglycemia is a particularly strong inducer of ET-1 production (28). In podocytes, both hyperglycemia and endothelin cause disassembly of the podocyte actin cytoskeleton, apoptosis, and podocyte depletion (22, 29). These effects may be due, at least in part, to altered nephrin production since ET-1 triggers nephrin loss from human podocytes, an effect that is prevented by ETA antagonism (30). Several studies report a beneficial effect of ERAs on diabetic nephropathy in experimental animal models (31–34). Notably, the renoprotective effect of ERAs was not consistently associated with a fall in blood pressure (35), suggesting that ET-1 contributes to diabetic renal injury via non-hemodynamic effects.
Hypertensive nephropathy
Hypertensive nephropathy is associated with increased renal ET-1 production (35). A recent report observed a 45-fold increase in renal cortical ET-1 levels within 4 weeks of 5/6 nephrectomy in transgenic renin rats (36). Numerous preclinical studies have demonstrated nephroprotective effects of ERAs in various forms of hypertensive nephropathy, including angiotensin-II-dependent, renin-dependent, salt-loaded renin-dependent, aldosterone-induced, genetically salt-sensitive and deoxycorticosterone–salt-induced hypertension (35). ERAs caused regression of hypertension-associated vascular hypertrophy and glomerulosclerosis in a model of NO-deficient hypertension (37, 38). Vaneckova et al. demonstrated that ETA, but not combined ETA/B blockade, was antiproteinuric despite continued malignant hypertension (39, 40); ETA selective antagonists attenuate hypertensive nephropathy and increase renal NO synthase activity in Dahl salt-sensitive hypertension (41, 42). Recent work from the Chade laboratory has implicated ET-1 in CKD associated with renovascular hypertension. These investigators demonstrated that ETA blockade not only delays renovascular CKD progression in swine with renal artery stenosis, but that ETA (but not ETB) antagonism induces regression of glomerulosclerosis and albuminuria independent of hypertension; CKD regression was associated with improved renal blood flow and improved cortical microvessel structure in the stenotic kidney (43, 44).
Focal segmental glomerulosclerosis
Focal segmental glomerulosclerosis is a widely varying clinicopathological entity characterized by injury to the glomerular filtration barrier (19). Urinary excretion of ET-1 is increased in primary FSGS patients and glomerular ET-1 expression is enhanced in experimental FSGS (45). Podocyte-specific mechanisms have been proposed as underlying FSGS development (46, 47). In humans and rodents, aging is associated with spontaneous development of FSGS (46), the susceptibility for which has recently been linked to autophagy-related mechanisms controlling podocyte vacuolization. Aging-associated FSGS is associated with increased renal ET-1 expression (48, 49). Studies in rodents with aging-FSGS demonstrated that ETA-selective antagonism for 1 month caused blood pressure-independent regression of FSGS, proteinuria and GBM hypertrophy, partially restored podocyte morphology, and reduced podocyte autophagy (21) (Figure 2). Of note, ERA treatment markedly down-regulated p21waf1/cip1, a cell cycle inhibitor and inhibitor of cell growth that contributes to CKD progression in FSGS animals (21, 50, 51).
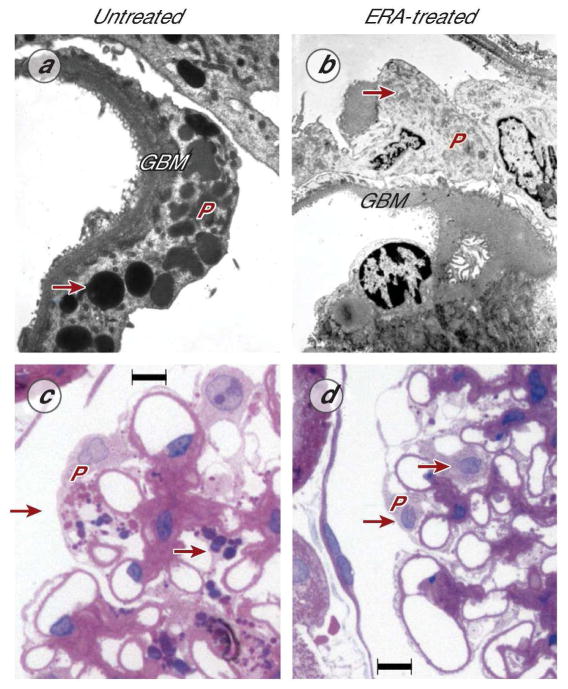
Ultrastructural and histological images demonstrating short-term effects of ERA treatment (darusentan) on aging-associated FSGS in the rat. a, Untreated kidney with FSGS, transmission electron microscopy of a podocyte (P) demonstrating hypertrophy of the glomerular basement membrane (GBM) and podocyte injury indicated by diffuse foot process effacement, podocyte hypertrophy, and autophagy-dependent vacuolar degeneration (arrow). b, Kidney with FSGS after 4 weeks of ERA treatment: ERA therapy caused regression of GBM hypertrophy and disappearance of podocyte vacuolization (arrow). c, light microscopy image (hematoxylin/eosin) of a glomerulus in an untreated kidney with FSGS, showing podocyte hypertrophy with enlarged nuclei, prominent nucleoles, and vacuolar degeneration, as well as hypertrophy of glomerular capillaries and matrix deposition/fibrosis (purple). d, Kidney with FSGS, ERA-treated for 4 weeks, showing normalization of podocyte size, virtually complete disappearance of vacuolar degeneration (arrows), as well as regression of glomerular capillary hypertrophy and matrix deposition. In this study (21), ERA treatment for 4 weeks induced regression of glomerulosclerosis by 55% and a 57% reduction in proteinuria. Panels adapted (21) and reproduced with permission of the publisher. Scale bar, 10 μm (c, d)
Human studies with endothelin antagonists in chronic kidney disease
Systemic and renal ET-1 production is increased in the setting of CKD, regardless of the underlying cause of the renal disease. Plasma ET-1 is increased by inflammation, vascular damage, lower renal clearance, acidosis and other factors, and directly correlates with urinary albumin excretion and the severity of CKD (52, 53). Plasma ET-1 is an independent predictor of vascular dysfunction in CKD patients, being associated with reduced flow-mediated brachial artery dilatation and increased carotid-femoral pulse wave velocity (PWV) (54). Urinary ET-1 excretion, which reflects renal ET-1 production, is increased in every cause of CKD in which it has been studied, including diabetes, hypertension, glomerulonephritides, polycystic kidney disease and others (55, 56). Acidemia also increases renal ET-1 production (10), while bicarbonate therapy decreases urinary ET-1 excretion and the rate of GFR decline in CKD patients with low or moderately reduced GFR (57, 58). In this regard, blockade of ET receptors increases urinary net acid excretion, renal ammoniagenesis, and serum bicarbonate in healthy human volunteers (59). Taken together with the preclinical studies, the above findings suggest that ERAs may be of therapeutic benefit in slowing CKD progression.
The effects of acute intravenous administration of ERAs on CKD patients have investigated in a series of very nice studies by Webb and colleagues (see Table 1 for discussion of this and other ET system inhibitors in CKD studies). Administration of an ETA-selective blocker, BQ123, augmented renal blood flow in hypertensive CKD patients, but not healthy volunteers, suggesting increased ETA activity in the renal vasculature in CKD patients (60). In this same study, BQ788, an ETB-selective antagonist, constricted the renal circulation and prevented BQ123-induced renal vasodilation, suggesting that ETB blockade may have deleterious effects on renal blood flow in CKD patients. Acute BQ123 administration also reduced proteinuria and PWV in 22 nondiabetic CKD patients to a greater extent than that seen with nifedipine (61); a subsequent sub-analysis determined that these BQ123 effects were greater in the setting of combined angiotensin converting inhibitors (ACEI) and angiotensin receptor blocker (ARB) administration, as compared to ACEI alone, suggesting the ETA blockade in CKD may be most effective in the setting of maximal renin-angiotensin system (RAS) blockade (62). Lastly, infusion of TAK-044 (~17-fold greater affinity for ETA as compared to ETB) to 7 nondiabetic CKD patients tended to increase renal plasma flow (63). These above studies collectively suggest that ERAs, and particularly ETA antagonists, might increase renal blood flow and reduce proteinuria in CKD patients.
Table 1
Completed and planned trials on ET system blockers in chronic kidney disease.
Disease | Study Type | Drug | Size | Outcome | Comments | Source |
---|---|---|---|---|---|---|
Hypertensive nephropathy | Acute infusion | BQ123 (ETA) BQ788 (ETB) | N=16 | BQ123 increased renal blood flow – prevented by BQ788 | BQ123 response seen in CKD, but not healthy patients. | (60) |
Non-diabetic CKD | Acute infusion | BQ123 (ETA) | N=22 | BQ123 reduced proteinuria and pulse wave velocity > nifedipine | Reduction in proteinuria and pulse wave velocity partly independent of blood pressure effects | (61) |
Non-diabetic CKD | Acute infusion | TAK-044 (ETA/B) | N=7 | TAK-044 tended to increase renal blood flow | Compared to placebo, TAK-044 reduced blood pressure and increased cardiac index | (63) |
Diabetic nephropathy | Phase 2 | Avosentan (ETA:B 50-300:1) 5, 10, 25, 50 mg/d | N=286 | Avosentan reduced UACR by ~21 – 30% from 5–50 mg/d vs. ~35% increased UACR in placebo | Baseline CrCl ~80 ml/min, UAER ~1500 mg/d. Fluid retention dose-dependent, ranging from 12–32% of patients. | (64) |
Diabetic nephropathy | Phase 3 (ASCEND) | Avosentan (ETA:B 50-300:1) 25, 50 mg/d | N=1392 | 44–49% reduction in UACR after ~4 months in avosentan group, 9% reduction in placebo. | Baseline median eGFR ~33 ml/min/1.73 m2, median UACR ~1500 mg/g. Trial terminated due to adverse events related to fluid retention | (65) |
Non-diabetic CKD | Phase 2 | Sitaxsentan (ETA) vs. nifedipine | N=27 | Sitaxsentan, but not nifedipine, reduced proteinuria after 6 weeks | CKD stages 1–4 | (66) |
Diabetic nephropathy | Phase 2a | Atrasentan (ETA) 0.25, 0.75, 1.75 mg/d | N=89 | Atrasentan reduced UACR ~35–40% at 2 highest doses vs. 11% decrease in placebo | Baseline UACR 350–515 mg/g and eGFR 48–61 ml/min/1.73 m2. Edema dose-dependent (14–46%) and generally mild. | (67) |
Diabetic nephropathy | Phase 2b (RADAR) | Atrasentan (ETA) 0.75, 1.25 mg/d | N=211 | Atrasentan reduced UACR ~35–39% vs. no change in placebo | Baseline eGFR 30–75 ml/min/1.73 m2, UACR 300–3500 mg/g, taking MTLD ACEI or ARB. Edema similar between groups. | (69) |
Diabetic nephropathy | Phase 3 (SONAR) | Atrasentan (ETA) 0.75 mg/d | Projected ~4150 | Actively enrolling. Primary endpoint - time to serum creatinine doubling or ESRD. | Baseline eGFR 25–75 ml/min/1.73 m2, UACR 300–5000 mg/g, taking MTLD ACEI or ARB. | (71) |
Diabetic nephropathy | Phase 2 | Daglutril (ECE inhibitor) | N=45 | No change UAER after 8 weeks | Baseline GFR ~70–90 ml/min, UAER 20–999 Dg/min. All taking losartan 100 mg/d. | (70) |
Primary FSGS | Phase 2 | RE-021 (dual ETA inhibitor and ARB) | N=72 | Not yet started. Primary endpoint – reduction in proteinuria. | Baseline eGFR >45 ml/min/1.73 m2, ages 8–50 years. | (72) |
Abbreviations: ARB – angiotensin receptor blocker; ACEI – angiotensin converting enzyme inhibitor; UACR – urinary albumin/creatinine ratio; CrCl – creatinine clearance; UAER – urinary albumin elimination rate; MTLD – maximal tolerated labelled dose. All diabetic nephropathy was type 2.
The first trial using ERAs in CKD evaluated the effect of 12 weeks of avosentan (ETA:B blockade ~ 50–300:1 on urinary mean albumin excretion rate (UAER) in 286 patients with diabetic nephropathy on RAS blockade with a creatinine clearance of ~80 ml/min and UAER of ~1500 mg/d (64). Avosentan decreased UACR by 20.9, 16.3, 25.0 and 29.9% at doses of 5, 10, 25, and 50 mg/d, respectively, while the placebo group had an 35.5% increase in UACR. The most significant side effect was fluid retention that was greatest at the highest avosentan dose (11.9, 21.1, 15.0, and 32.1% in the 5, 10, 25, and 50 mg/d groups, respectively). Thus, there was a possible small dose-dependent reduction in UACR of questionable significance, but potential adverse events associated with the 50 mg dose. The same company (Speedel) conducted a phase 3 trial (ASCEND, A Randomised, Double Blind, Placebo Controlled, Parallel Group Study to Assess the Effect of the Endothelin Receptor Antagonist Avosentan on Time to Doubling of Serum Creatinine, End Stage Renal Disease or Death in Patients With Type 2 Diabetes Mellitus and Diabetic Nephropathy) examining the effect of avosentan on renal disease progression or death in 1392 type II diabetic nephropathy patients (ACR ≥309 mg/g and a serum creatinine between ≥115 and 265 mmol/L (≥1.3 to 3.0 mg/dl) in men and between ≥106 and 265 mmol/L (≥1.2 to 3.0 mg/dl) in women) on RAS blockade (65). The median albumin to creatinine ratio (ACR) at baseline was ~1500 mg/g and the eGFR was ~33 ml/min/1.73 m2. Avosentan decreased ACR after a median follow-up of 4 months (44.3, 49.3, and 9.7% for the 25 mg/d, 50 mg/d and placebo groups, respectively), however the trial was prematurely terminated due to adverse cardiovascular events in the avosentan groups, including a 3-fold increase in the incidence of congestive heart failure. Importantly, another study published at the same time as the initial Phase 2 avosentan trial showed a clear dose-dependent reduction in fractional excretion of sodium between 0.5 and 50 mg of avosentan, and the authors concluded that the potential clinical benefits of avosentan should be investigated at doses ≤ 5 mg/d (Smolander, 2009). It was hypothesized that at the higher doses, avosentan may act on the ETB receptor to promote additional fluid retention (7). Taken together with the Phase 2 avosentan trial described above, it is apparent that the use of 25 and 50 mg avosentan doses in the ASCEND trial was ill-advised, particularly in patients with more advanced CKD who have a propensity towards fluid retention.
Given the failure of the ASCEND trial, subsequent trials examining ERAs in CKD were undertaken with very careful ERA dose and patient selection. In a phase 2 trial, sitaxsentan, a highly selective ETA blocker (ETA:B selectivity of ~ 6000:1) was given to 27 nondiabetic CKD (stages 1–4) subjects for 6 weeks (66). Sitaxsentan, but not the active comparator nifedipine, reduced proteinuria, while no clinically significant adverse events occurred. In another phase 2a study, the effect of atrasentan (ETA:B receptor selectivity of ~1200:1) for 8 weeks on ACR was assessed in 89 subjects with diabetic nephropathy (baseline ACR 350–515 mg/g and eGFR 48–61 ml/min/1.73 m2) receiving stable doses of RAS inhibitors (67). Atrasentan reduced ACR in the 0.75 mg/d and 1.75 mg/d groups as compared to placebo (−42%, −35% vs. 11% decreases, respectively), while the 0.25 mg/d dose was without effect. The ACR fell in the first week of treatment and was associated with reduced BP. This reduction in BP, and a possible consequent decrease in glomerular capillary pressure, however, is unlikely to fully explain the reduced ACR since the BP drop was not associated with a detectable decrease in eGFR; it raises the possibility that glomerular permeability was affected. As for previous trials, edema was dose-related: 9, 14, 18, and 46% in placebo and 0.25, 0.5 and 1.75 mg/d atrasentan groups, respectively (all the edema was mild to moderate). In a follow-up analysis of this study, mean UACR reduction in subjects receiving maximal labeled doses of RAS inhibitors (38% of the subjects) was similar to that seen overall (68). Based on these positive results, two Phase 2b trials (RADAR, Reducing Residual Albuminuria in Subjects With Diabetes and Nephropathy With AtRasentan), involving two identically-designed, parallel, multinational studies were undertaken examining the effect of atrasentan on residual albuminuria in a total of 211 subjects with type II diabetes, UACR between 300 – 3500 mg/g and eGFR between 30 – 75 ml/min/1.73 m2 who were receiving the maximal tolerated labeled dose of ACEI or ARB (69). Patients received atrasentan 0.75 mg/d (n=78) or 1.25 mg/d (n=83) or placebo (n=50) for 12 weeks. By repeated measures analysis, UACR did not change in the placebo group, but decreased by 35.5% and 38.6% over the 12 weeks in the 0.75 and 1.25 mg atrasentan groups, respectively. The UACR fell to its lowest value at the first time point (2 weeks of treatment) and this was associated with a concurrent fall in ambulatory BP in both groups (by ~5 mmHg); both BP and UACR normalized to baseline values 30 days after drug withdrawal, raising the possibility of involvement of a hemodynamic effect. The overall rate of new or worsening peripheral edema was 42%, 35% and 42% in placebo, 0.75 and 1.25 mg/d atrasentan groups, respectively, while the incidence of discontinuation of the study due to peripheral edema was 0%, 2.6% and 4.8% in the placebo, 0.75 and 1.25 mg/d atrasentan groups, respectively. The edema was generally mild and there was no difference in diuretic usage between groups. In this, as well as the other trials with atrasentan, patients were excluded if, among other criteria, they had a history of heart failure, were taking large doses of diuretics, or the BNP was elevated. Finally, both doses of atrasentan reduced total cholesterol (by ~18 mg/dl), LDL cholesterol (by ~ 15 mg/dl), and triglycerides (by 30 mg/dl 0.75 mg dose and 48 mg/dl in 1.25 mg dose) over 12 weeks of treatment and this was evident regardless of statin use; the mechanisms for such improvement in lipid profile are uncertain. In summary, Phase 2 trials using ETA-selective antagonists in patients with moderate CKD due to type II diabetes have shown significant reductions in albuminuria in the setting of maximal labeled-dose RAS blockade and at ERA doses that are generally well tolerated; an added benefit of improved lipid profile may also exist. These studies raise the possibility that ETA antagonists, particularly in the setting of a multi-drug approach involving maximal tolerated RAS blockade, may be beneficial in treating CKD progression.
Human studies with endothelin converting enzyme inhibition in chronic kidney disease
The effect of daglutril, an inhibitor of endothelin converting enzyme (ECE) and neutral endopeptidase (NEP) (the latter should increase natriuretic peptides, bradykinin and substance P), has now been assessed in type 2 diabetes patients with albuminuria (70). This crossover study was conducted on 45 Italian patients with urinary albumin excretion rates between 20–999 Pg/min who were taking losartan (100 mg/d). Patients received 8 weeks of placebo or daglutril (300 mg/d), followed by a 4-week washout and a second 8-week study period. The GFR was ~90 in the daglutril to placebo group and ~72 in the placebo to daglutril group; GFR did not change with treatment. Daglutril did not change 24-h urinary albumin excretion compared to placebo, but did reduce BP (24-h systolic BP fell by ~5 mmHg). Daglutril increased plasma big ET-1 levels, however plasma or urine ET-1 levels were not determined – the latter would be useful in determining how effectively ECE (which has several isoforms (11)) was inhibited. It is uncertain whether higher doses of daglutril would have manifested an antiproteinuric effect, however it is interesting that despite the BP reduction, no fall in albuminuria was observed; a comparable BP reduction was observed in the RADAR study wherein there was a substantial antiproteinuric effect. The reasons for these different effects of daglutril and ETA antagonists on proteinuria are unknown, but might relate to the fact that ECE reduces ET levels overall, so that both ETA and ETB activation are affected. Notably, no clinical studies have examined the effect of NEP or vasopeptidase inhibition without combined ECE blockade in the setting of CKD, hence it is not possible to know whether ECE blockade is problematic (or whether NEP/vasopeptidase inhibition is beneficial). In the final analysis, the current evidence suggests that ECE is not a promising target in treating CKD.
Ongoing and planned clinical trials with endothelin antagonists in chronic kidney disease
At least two trials involving ETA-selective ERAs in CKD are active or in the development stages. In a recently started phase 3 trial (SONAR, Study Of Diabetic Nephropathy With AtRasentan), 0.75 mg/d atrasentan or placebo will be given to subjects with type 2 diabetes receiving maximum tolerated labeled doses of a RAS inhibitor with an eGFR of 25–75 ml/min/1.73 m2, UACR 300–5,000 mg/g, and systolic BP of 110–160 mmHg (71). The primary endpoint is time to doubling of serum creatinine or the onset of ESRD. Patients will enter a 6-week enrichment period where they receive atrasentan. Responders (~3,150 subjects with UACR reduction ≥ 30% from baseline) and non-responders (~1000 subjects UACR < 30% reduction from baseline) will be randomized 1:1 into a double-blind treatment period for ~48 months. Another study, as yet in development, will examine the effect of RE-021, a dual ETA antagonist and ARB, on UAER in 72 patients with primary FSGS (eGFR >45 ml/min/1.73 m2, ages 8–50 years) (72). Finally, a study assessing the effect of one year of bosentan (ETA:B ~ 20:1) treatment on renal function in patients with scleroderma renal crisis was planned but suspended (73). The reasons for this are uncertain, although preliminary data in 6 patients did not show a benefit of bosentan over historical controls (74).
Adverse effects of endothelin receptor antagonists
Endothelin receptor antagonists have a risk profile that must be considered in all patients for whom therapy is contemplated (reviewed in (75, 76)). Sulfonamide-based ERAs can cause hepatotoxicity – although generally mild and avoided by appropriate dosing, one ERA (sitaxsentan) was removed from the market following two cases of fatal liver injury. Since both ETA and ETB are crucial for normal development, all ERAs are absolutely contraindicated during pregnancy. In addition, ERAs have been reported in drug company literature to potentially cause testicular toxicity, however significant testicular damage has not been reported in patients taking bosentan and ambrisentan, the only two ERAs currently licensed for clinical use (primarily for pulmonary hypertension). The most clinically relevant side effect of ERAs relates to fluid retention. As discussed earlier, this fluid retention occurs with either ETA-selective or combined ETA/B blockers and involves, at least in part, a primary effect on nephron sodium and water excretion. The key issue is that, regardless of the ERA used, appropriate dosing, concomitant diuretic use, and careful patient selection (avoiding patients with high risk of adverse effects of fluid retention such as those with severe CKD or congestive heart failure) are essential. Indeed, in the SONAR trial, diuretic use will be mandated – this is likely to be a key factor in the ultimate success of this class of drugs. Currently we do not know what level of CKD precludes ERA treatment, particularly if relatively low doses of ERAs are used, however it is unlikely that the risk-benefit ratio will merit use of this class of drugs in patients with an eGFR ≤ 20 ml/min/1.73 m2.
Conclusions and perspective
ET-1 is an important regulator of kidney function in health and disease (6, 7). Abnormal activation of the renal endothelin system can promote CKD progression; inhibition of primarily ETA receptors has been shown to ameliorate renal injury and fibrosis at multiple levels. Preclinical evidence and early phase clinical trials suggest that ERAs have potential therapeutic benefit as antiproteinuric and nephroprotective drugs for diabetic nephropathy, hypertensive nephropathy, FSGS and possibly other forms of CKD. The major limitation to ERA therapy is fluid retention which potentially can be prevented or minimized by careful patient selection, drug dosing and diuretic administration. Clinical trials are ongoing with ERAs in CKD; there is particular hope that a large Phase 3 trial in diabetic nephropathy will provide evidence for the clinical utility of this class of drugs. Given the dearth of new and effective therapies to retard CKD progression, continued examination of the biology of the renal ET system and the potential utility of agents that target renal ET receptors in CKD is highly warranted.
Acknowledgments
Sources of support: Part of the work presented in this review was supported by NIH grant P01 HL095499 (to D.E.K.) and SNSF grants 108 258 and 122 504 (to M.B.)
Footnotes
Disclosures
D.E.K. is a consultant to AbbVie and Retrophin, M.B. is a consultant to AbbVie.
References
Full text links
Read article at publisher's site: https://doi.org/10.1038/ki.2014.143
Read article for free, from open access legal sources, via Unpaywall:
http://www.kidney-international.org/article/S0085253815304087/pdf
Citations & impact
Impact metrics
Article citations
The Relationship between Vascular Biomarkers (Serum Endocan and Endothelin-1), NT-proBNP, and Renal Function in Chronic Kidney Disease, IgA Nephropathy: A Cross-Sectional Study.
Int J Mol Sci, 25(19):10552, 30 Sep 2024
Cited by: 0 articles | PMID: 39408883 | PMCID: PMC11476882
Prognostic Value of Plasma Endothelin-1 in Predicting Worse Outcomes in Patients with Prediabetes and Diabetes and Stable Coronary Artery Diseases.
Diabetes Metab J, 48(5):993-1002, 21 Aug 2024
Cited by: 0 articles | PMID: 39165112 | PMCID: PMC11449811
Sparsentan is superior to losartan in the gddY mouse model of IgA nephropathy.
Nephrol Dial Transplant, 39(9):1494-1503, 01 Aug 2024
Cited by: 4 articles | PMID: 38271614 | PMCID: PMC11361813
A new perspective on proteinuria and drug therapy for diabetic kidney disease.
Front Pharmacol, 15:1349022, 31 Jul 2024
Cited by: 1 article | PMID: 39144629 | PMCID: PMC11322372
Review Free full text in Europe PMC
Drug repurposing for glomerular diseases: an underutilized resource.
Nat Rev Nephrol, 20(11):707-721, 31 Jul 2024
Cited by: 2 articles | PMID: 39085415
Review
Go to all (137) article citations
Data
Data behind the article
This data has been text mined from the article, or deposited into data resources.
BioStudies: supplemental material and supporting data
Similar Articles
To arrive at the top five similar articles we use a word-weighted algorithm to compare words from the Title and Abstract of each citation.
Therapeutic potential of endothelin receptor antagonism in kidney disease.
Am J Physiol Regul Integr Comp Physiol, 310(5):R388-97, 23 Dec 2015
Cited by: 13 articles | PMID: 26702154
Review
Endothelin-targeted new treatments for proteinuric and inflammatory glomerular diseases: focus on the added value to anti-renin-angiotensin system inhibition.
Pediatr Nephrol, 36(4):763-775, 17 Mar 2020
Cited by: 7 articles | PMID: 32185491
Review
Endothelin Receptor Antagonists: New Hope for Renal Protection?
Curr Hypertens Rep, 17(7):57, 01 Jul 2015
Cited by: 4 articles | PMID: 26068660
Review
Dual inhibition of renin-angiotensin-aldosterone system and endothelin-1 in treatment of chronic kidney disease.
Am J Physiol Regul Integr Comp Physiol, 310(10):R877-84, 23 Mar 2016
Cited by: 46 articles | PMID: 27009050 | PMCID: PMC4896079
Review Free full text in Europe PMC
Funding
Funders who supported this work.
NHLBI NIH HHS (1)
Grant ID: P01 HL095499
National Institutes of Health (1)
Grant ID: P01 HL095499
Swiss National Science Foundation (2)
Grant ID: 108 258
Grant ID: 122 504