Abstract
Free full text
Enhancement of oxidative and drought tolerance in Arabidopsis by overaccumulation of antioxidant flavonoids
Associated Data
Abstract
The notion that plants use specialized metabolism to protect against environmental stresses needs to be experimentally proven by addressing the question of whether stress tolerance by specialized metabolism is directly due to metabolites such as flavonoids. We report that flavonoids with radical scavenging activity mitigate against oxidative and drought stress in Arabidopsis thaliana. Metabolome and transcriptome profiling and experiments with oxidative and drought stress in wild-type, single overexpressors of MYB12/PFG1 (PRODUCTION OF FLAVONOL GLYCOSIDES1) or MYB75/PAP1 (PRODUCTION OF ANTHOCYANIN PIGMENT1), double overexpressors of MYB12 and PAP1, transparent testa4 (tt4) as a flavonoid-deficient mutant, and flavonoid-deficient MYB12 or PAP1 overexpressing lines (obtained by crossing tt4 and the individual MYB overexpressor) demonstrated that flavonoid overaccumulation was key to enhanced tolerance to such stresses. Antioxidative activity assays using 2,2-diphenyl-1-picrylhydrazyl, methyl viologen, and 3,3′-diaminobenzidine clearly showed that anthocyanin overaccumulation with strong in vitro antioxidative activity mitigated the accumulation of reactive oxygen species in vivo under oxidative and drought stress. These data confirm the usefulness of flavonoids for enhancing both biotic and abiotic stress tolerance in crops.
Introduction
Environmental stresses cause significant loss of plant productivity. Abiotic stresses, e.g. light, UV, temperature, drought, soil salinity, air pollution, and mechanical damage, directly affect crop production (Vickers et al., 2009). For example, the US drought of 2012, which was the most severe in at least 25 years, had a serious impact on production and yields of corn and soybean, leading to economic damage (Gilbert, 2012). This observation strongly suggests that a better understanding of the stress response is critically important for agricultural and economic performance to improve the tolerance of crops against such environmental stresses using phytochemical genomics and crop breeding (Varshney et al., 2012).
Flavonoids are a representative group of secondary metabolites that have been recently called ‘specialized metabolites’ because plants synthesize species-specific metabolites (Harborne and Williams, 2000). A series of flavonoid subgroups such as flavonols, (iso)flavones, flavanones, flavan-3-ols, proanthocyanidins, and anthocyanins has been recognized in over 500 food items by the US Department of Agriculture as dietary flavonoids with consistent evidence of beneficial effects in humans. Such metabolites are expected to be useful for crop breeding because they play a role in allelopathy, which has positive or negative effects on the growth of target organisms such as fungi, insects, and plants (Harborne and Williams, 2000; Bais et al., 2003; Field et al., 2006).
Biotic and abiotic environmental stresses induce the accumulation of flavonoids in plants (Dixon and Paiva, 1995). Under the influence of abiotic stresses such as phosphate, nitrogen, light, temperature, UV, and drought, flavonoids accumulate in the model plant Arabidopsis thaliana (Arabidopsis) (Rowan et al., 2009; Stracke et al., 2010a; Catala et al., 2011; Koops et al., 2011; Kusano et al., 2011; Lei et al., 2011; Tohge et al., 2013). A common factor with abiotic stress is the accumulation of reactive oxygen species (ROS) such as hydroxyl radicals ·OH, superoxide radicals , and hydrogen peroxide H2O2. Therefore, it can be questioned whether flavonoids effectively scavenge ROS. Arabidopsis can be utilized to address this question as its flavonoid biosynthetic pathway has been elucidated at the metabolite and gene levels. Fifty-four flavonoids accumulate in an organ-specific manner in Arabidopsis (Saito et al., 2013), and 35 genes encoding biosynthetic enzymes or transcription factors are responsible for aglycone formation and subsequent tailoring modifications to produce these flavonols and anthocyanins (Yonekura-Sakakibara et al., 2008). The flavonol regulators MYB12/PFG1 (PRODUCTION OF FLAVONOL GLYCOSIDES1), MYB11/PFG2, and MYB111/PFG3 regulate expression of the four early biosynthetic genes chalcone synthase (CHS), chalcone isomerase (CHI), flavanone 3-hydroxylase (F3H), flavonoid 3′-hydroxylase (F3′H), and flavonol synthase (FLS) (Stracke et al., 2007, 2010b), whereas the anthocyanin regulators MYB75/PAP1 (PRODUCTION OF ANTHOCYANIN PIGMENT1), MYB90/PAP2, MYB113, and MYB114 control the expression of the late biosynthetic genes dihydroflavonol reductase (DFR) and leucoanthocyanidin dioxygenase/anthocyanidin synthase (LDOX/ANS) (Borevitz et al., 2000; Gonzalez et al., 2008; Dubos et al., 2010).
Manipulating the expression of flavonoid biosynthetic genes alters the accumulation of flavonoids in Arabidopsis and other plants (Shirley et al., 1995; Borevitz et al., 2000; Butelli et al., 2008, 2012). Subsequent omics profiling using these genetically engineered plants has led to the identification of new functions of biosynthetic genes and the clarification of biosynthetic pathways (Fukushima et al., 2009; Saito and Matsuda, 2010). Although a few reports have discussed the interaction between flavonoid accumulation and tolerance to abiotic stresses such as UV-B irradiation (Stracke et al., 2010a; Kusano et al., 2011), flavonoid function remains to be determined experimentally. In the present study, we report flavonoids as in vitro ROS scavengers that lead to enhanced oxidative and drought tolerance in vivo using metabolome and transcriptome profiling in the wild type, MYB12/PAP1 overexpressors, transparent testa4 (tt4) as a flavonoid-deficient mutant, and flavonoid-deficient MYB12 or PAP1 overexpressors.
Results
Visible phenotype is conferred by co-expression of the flavonoid-specific transcription factors MYB12 and PAP1
The WOX1 (double overexpressor1 of both MYB12 and PAP1) lines were prepared by crossing MYB12OX (MYB12 overexpressor) (Mehrtens et al., 2005) and pap1-D (production of anthocyanin pigment1-Dominant) (Borevitz et al., 2000) to address the function of flavonoids during environmental stress (Figure(Figure1a).1a). Both MYB12 and PAP1 were concurrently overexpressed in WOX1 lines (Figure(Figure1b).1b). MYB12/PAP1 overexpression did not result in significant differences in plant size in the MYB12OX, pap1-D, or WOX1 lines compared with that in the wild type Columbia-0 (Col-0) under our growth conditions (Figure S1 in Supporting Information). During the seedling stage, the pap1-D and WOX1 lines exhibited purple pigmentation due to the accumulation of anthocyanin in petioles, hypocotyls, and roots. In contrast, the MYB12OX line had apparently the same phenotype as that of the wild type (Figure(Figure1a).1a). Purple pigmentation was observed in the adult stage, particularly in the veins, petioles, and stems of the pap1-D and WOX1 lines (Figure S1).

Phenotypes of the wild type, MYB12OX, pap1-D, and WOX1 lines.(a) Phenotypes of seeds and aerial parts. The red arrows indicates coloration from anthocyanin accumulation in 3-week-old plants.(b) Steady-state MYB12 and PAP1 mRNA levels by real-time PCR. The aerial parts of 3-week-old plants grown on growth medium were used in this analysis. Steady-state MYB12 and PAP1 mRNA levels in MYB12OX and pap1-D were both considered as 100%. Standard deviations (error bars) were calculated from the results of three biological replicates.
The quantity and diversity of flavonoids is increased by overexpressing MYB12/PAP1
The dominant flavonoids in MYB12OX and pap1-D are flavonol glycosides and anthocyanins (Mehrtens et al., 2005; Tohge et al., 2005a; Stracke et al., 2010b). Flavonoid-targeted profiling was performed by liquid chromatography photodiode array mass spectrometry (LC PDA MS) to determine the differences in the quantity and profiles of flavonols and anthocyanins between the wild type and MYB overexpressors. The LC PDA MS procedure provided UV spectra for deciphering the peaks of flavonol glycosides and anthocyanins in addition to MS spectra (Yonekura-Sakakibara et al., 2008). The level of total anthocyanins in all parts increased significantly in the pap1-D and WOX1 lines (approximately a 20-fold increase), whereas that of total flavonols increased slightly in the MYB12OX and WOX1 lines compared with that in the wild type (approximately a two-fold increase) (Figure(Figure2a2a and Figure S2). The WOX1 lines accumulated enhanced levels of the glycosides of kaempferol, quercetin, and cyanidin compared with each single overexpressed line (Figure S3).
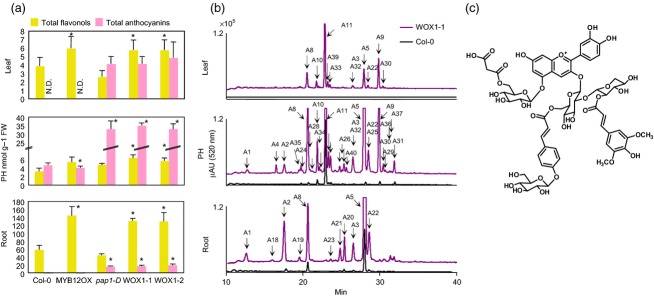
Flavonoid contents of the wild type and MYB overexpressors grown under general conditions.(a) Total flavonols and anthocyanins in each part of 3-week-old plants. Differences between the wild-type and other plants analyzed using Student's t-test were statistically significant (*P < 0.05). Differences and standard deviations (error bars) were calculated from the results of three biological replicates. PH, petiole and hypocotyl; N.D., not detected.(b) Representative chromatogram of the wild type and WOX1-1 at 520 nm. The black line chromatogram represents the wild type, and the purple line chromatogram represents WOX1-1. See Tables S1 and S2 for details of the detected peaks. Such anthocyanins were also detected in pap1-D. L, leaf; PH, petiole and hypocotyl; R, root.(c) Structure of A11.
Twenty-three anthocyanins (A18–A40), probably including isomers of the major anthocyanins (Rowan et al., 2009), were assigned as minor anthocyanins in the pap1-D and WOX1 lines during profiling (Figure(Figure2b).2b). In addition to the major anthocyanin A11 (Figure(Figure2c)2c) and its intermediates (A1–A10) (Table S1) (Nakabayashi et al., 2009), most of the minor anthocyanins accumulated in petioles and hypocotyls (PH). Of those, the five minor anthocyanins (A21, A22, A28, A30, and A39) with caffeoyl or feruloyl moieties (Table S2) were assigned on the basis of UV (approximately λmax 520 nm) and MS spectra (difference and fragment ion). This metabolic plasticity revealed the dynamic nature of specialized metabolism in plants, thus suggesting an adaptive response to biosynthetic diversity.
Flavonol overaccumulation in the MYB12OX and WOX1 lines was not prominent (Figure(Figure2a).2a). Thus, the effect of MYB12 overexpression on flavonol accumulation was examined under light-shielding conditions using black paper (Figure S4). The effects of MYB12 overexpression were more clearly observed by comparing the wild type and MYB overexpressors grown under the light-shielding conditions because flavonols and anthocyanins did not accumulate in the roots of Arabidopsis wild-type plants grown under similar light-shielding and soil conditions (Hemm et al., 2004). Growth under the light-shielding conditions revealed that flavonols accumulated significantly in all parts of the MYB12OX and WOX1 lines compared with the wild type (greater than seven-fold increase) (Figure(Figure3a).3a). Accumulation of major flavonols (f1, f2, f3, f5, f6, and f8) increased significantly in the hypocotyls and transient zone (HTz) and roots of the MYB12OX and WOX1 lines (Figure(Figure3b,c).3b,c). This result indicates that MYB12 overexpression increases flavonol biosynthesis systemically in the plants.

Flavonoid contents of the wild type and MYB overexpressors grown under the light-shielding condition.(a) Total flavonols and anthocyanins in each part of 2-week-old plants. Differences between the wild type and other plants analyzed using Student's t-test were statistically significant (*P < 0.05). Differences and standard deviations (error bars) were calculated from the results of three biological replicates. HTz, hypocotyl and transient zone; N.D., not detected.(b) Representative chromatogram of the wild type and WOX1-1 at 340 nm. Details of the detected peaks are presented in Table S1.(c) Structure of flavonols.
The concentration of abscisic acid (ABA) is unaffected by increased flavonoid biosynthesis
Plant hormone analysis of the aerial parts was performed using LC tandem mass spectrometry (LC MS/MS) to test the hypothesis that both MYB overexpression and flavonoid accumulation affect the levels of plant hormones that play an important role in stress signaling and the expression of stress-related genes (Figure S5 and Method S1). The analysis revealed similar ABA concentrations in the wild-type and MYB-overexpressing lines, ruling out a role for stress-related hormones downstream of the MYB gene. The analysis also revealed that trans-zeatin (tZ) increased significantly in the MYB overexpressors compared with the wild type, whereas N6-(Δ2-isopentenyl) adenine (iP) decreased.
Stress-related gene expression is unaffected by increased flavonoid biosynthesis
We further prepared a tt4 mutant line lacking CHS (Shirley et al., 1995) and the flavonoid-deficient MYB overexpressors MYB12OX/tt4 and pap1-D/tt4 that were obtained by crossing MYB12OX or pap1-D with tt4, respectively (Figure(Figure4).4). This was done to explore the effect of MYB overexpression on the accumulation of a wide range of metabolites including flavonoids by untargeted metabolome profiling of whole plants using LC quadrupole time-of-flight MS (LC QTOF MS) (Matsuda et al., 2010). Principal component analysis (PCA) of the processed data suggested that accumulation of flavonols and anthocyanins was the main contributor to the separation in the scatterplot (Figure(Figure5a).5a). The results of hierarchical cluster analysis (HCA) revealed that MYB12 and PAP1 overexpression primarily induced overaccumulation of flavonols and anthocyanins (Figure(Figure5b).5b). Flavonols and anthocyanins were not detected in tt4, MYB12OX/tt4, or pap1-D/tt4.

Phenotype of wild-type, tt4, the flavonoid-deficient MYB overexpressors, and the MYB overexpressors.Upper: Phenotype of seeds and 3-week-old plants.Lower: Relative MYB12 and PAP1 expression in the aerial parts of 3-week-old plants grown on GM plates. Steady-state MYB12 and PAP1 mRNA levels in MYB12OX and pap1-D were considered 100%, respectively. Standard deviations (error bars) were calculated from the results of 3 biological replicates.

Metabolome profiling using liquid chromatography quadrupole time-of-flight mass spectrometry.(a) Metabolo-type of the wild-type, tt4, flavonoid-deficient MYB overexpressors, and MYB overexpressors. Whole parts of 3-week-old plants grown on growth medium plates in a growth chamber were analyzed (three biological replicates). Processed data including 380 metabolite peaks after alignment, deisotoping, noise cutting, and normalization were used for principal component analysis (PCA) using SIMCA-P 11.5 software. The plot of principal component 1 (PC1, 35.1%) vs. principal component 2 (PC2, 17.8%) is presented. Gray and brown dotted circles represent the flavonoid-deficient and flavonoid-overaccumulating plants, respectively. Col-0, black squares; tt4, orange circles; MYB12OX/tt4, green triangles; pap1-D/tt4, purple squares; MYB12OX, red circles; pap1-D, blue diamonds; WOX1-1, pink crosses; and WOX1-2, yellow triangles. (b) Hierarchical cluster analysis using the processed metabolome data. Pearson's correlation analysis was used. Relative log2-transformed values of signal intensities were calculated for the wild-type. The single arrow indicates the cluster of flavonols, and the double arrow indicates the cluster of anthocyanins. Lower: Enlarged view of the flavonol and anthocyanin clusters. Bold indicates assigned monoisotopic ion, and asterisk indicates flavonoid-derived ion.
Transcriptome profiling using DNA microarrays of the aerial parts of the wild type and other plants was performed to examine whether MYB overexpression or flavonoid overaccumulation were responsible for the expression of stress-related genes involved in both the biotic and abiotic stress response (Figure(Figure6,6, upper part). Profiling revealed that the expression of 907 genes was significantly induced in the mutants compared with the wild type (P < 0.05; log2 fold change ≥1.0) (Dataset S1). Of these genes, 245 were categorized into the Gene Ontology term ‘response to biotic or abiotic stimulus’ in the Gene Ontology annotations of TAIR10. However, of those, only six genes (A5GlcMalT, F3H, A5GlcT, TT19, DFR, and A3G2″XylT) were commonly induced in the PAP1-overexpressing pap1-D/tt4, pap1-D, and WOX1 lines, whereas no stress-related genes were induced in the anthocyanin-overaccumulating pap1-D and WOX1 lines (Figure(Figure6,6, lower part). No stress-related genes were commonly induced in the MYB12-overexpressing MYB12OX/tt4, MYB12OX, and WOX1 lines or in the flavonol-overaccumulating MYB12OX and WOX1 lines. In addition, untargeted profiling using the 907 genes was performed to investigate the expression of genes annotated as ‘unknown’. The expression of six additional genes was identified as commonly altered in the PAP1-overexpressing lines (Dataset S1). However, those genes, including three flavonoid biosynthetic genes, appeared to be unrelated to the improvement of stress tolerance. These results suggest that enhanced MYB expression and flavonoid accumulation have no effect on the expression of stress-related genes.
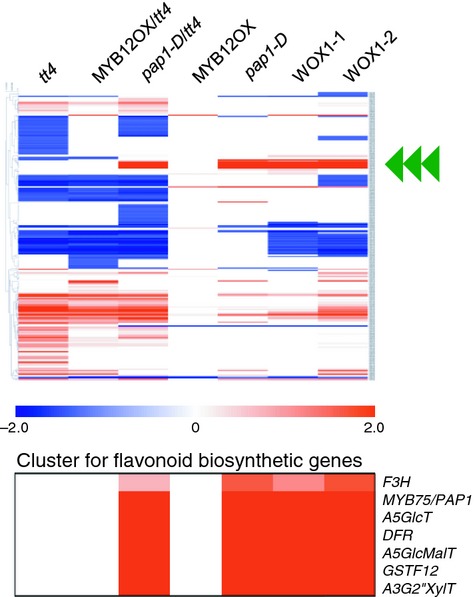
Transcriptome profiling using a DNA microarray.The processed transcriptome data including 245 stress-related genes were used for hierarchical cluster analysis. Pearson's correlation analysis was used. Relative log2-transformed signal intensity values were calculated for the wild type. The triple arrow indicates the cluster of flavonoid-related biosynthetic genes. The lower part shows an enlarged view of the flavonoid gene cluster. F3H, flavanone 3-hydroxylase (At3 g51240); A5GlcT, anthocyanin 5-O-glucosyltransferase (At4 g14090); DFR, dihydroflavonol reductase (At5 g42800); A5GlcMalT, anthocyanin malonyltransferase (At3 g29590); GSTF12, glutathione S-transferase12 (At5 g17220); A3G2″XylT, anthocyanin 3-O-glucoside: 2″-O-xylosyltransferase (At5 g54060).
Enhanced tolerance of abiotic stress by overaccumulation of flavonols and anthocyanins
The radical scavenging activity of flavonoids found in Arabidopsis was assayed using 2,2-diphenyl-1-picrylhydrazyl (DPPH) to investigate the contribution of flavonoid accumulation to stress tolerance. The DPPH assay is an easy method for evaluating the activity of flavonoids on the basis of the established reaction mechanism of DPPH and flavonoids (Seyoum et al., 2006). Quercetin 3-O-glucoside (Q3G) and cyanidin 3-O-glucoside (C3G) exhibited approximately 10-fold higher activity than kaempferol 3-O-glucoside (Figure(Figure7a),7a), suggesting that the highly modified flavonoid glycosides detected in this study have radical scavenging activities. Thus, the radical scavenging activity of the crude extract from each plant was examined. Extracts from the pap1-D and WOX1 lines exhibited greater than two-fold higher activity than those from the wild type (Figure(Figure7b),7b), providing compelling evidence that the highly modified flavonoids in Arabidopsis are responsible for radical scavenging activity in vitro. All plants were exposed to oxidative stress using methyl viologen, which generates and accumulates ROS in plants (Fujita et al., 2012). As a result, the pap1-D and WOX1 lines exhibited a tolerance to oxidative stress three times greater than the wild-type (Figure(Figure77c).

Enhancement of oxidative tolerance by flavonoid overaccumulation.(a) Radical scavenging activity of kaempferol 3-O-glucoside (K3G), quercetin 3-O-glucoside (Q3G), isorhamnetin 3-O-glucoside (I3G), and cyanidin 3-O-glucoside (C3G) against 2,2-diphenyl-1-picrylhydrazyl (DPPH). Trolox, which is a vitamin E analog, was the positive control. Differences and standard deviations (error bars) were calculated from the results of three replicates.(b) Relative radical scavenging activity of the acidic methanol–water extracts from the wild type, tt4, flavonoid-deficient MYB overexpressors, and MYB overexpressors against DPPH. Relative activity values for the wild type were calculated for this analysis. Differences between the wild type and other plants analyzed using Student's t-test were statistically significant (*P < 0.05). Differences and standard deviations (error bars) were calculated from the results of three biological replicates.(c) Relative chlorophyll levels in the wild type, tt4, flavonoid-deficient MYB overexpressors, and MYB overexpressors after oxidative stress using methyl viologen. Relative values of the wild-type chlorophyll level were calculated. Differences between the wild type and other plants analyzed using Student's t-test were statistically significant (*P < 0.05). Differences and standard deviations (error bars) were calculated from the results of three biological replicates.
The wild-type and mutants were exposed to drought stress, which also increases the production and accumulation of ROS (Rivero et al., 2007; Hernandez et al., 2009). The number of PAP1-overexpressing lines that survived was more than two times higher than that of the wild type, which survived stochastically (Figure(Figure8a8a and Figure S6). Untargeted profiling using LC QTOF MS was performed using samples harvested after rewatering to check the contribution of anthocyanin accumulation to drought tolerance (Figure(Figure8b).8b). Accumulation of the major anthocyanin A11 most prominently contributed to the separation of the pap1-D and WOX1 lines on PC1 in PCA (Figure(Figure88c).
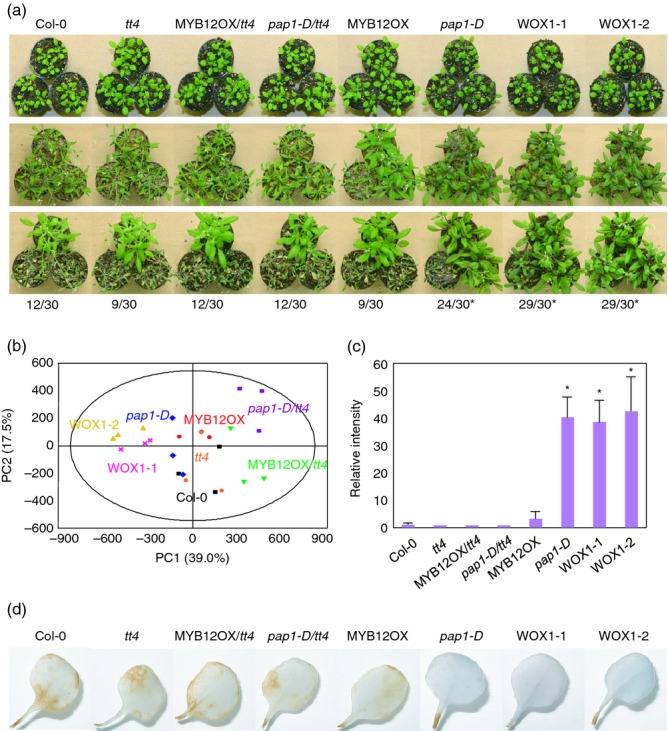
Enhancement of drought tolerance by flavonoid overaccumulation.(a) Phenotype of the wild type, tt4, flavonoid-deficient MYB overexpressors, and MYB overexpressors under drought stress. Top: three plants were grown on soil in one pot. Ten pots were prepared in each experiment. Middle: a difference in wilting was observed among the MYB overexpressors and other lines after 14 days; Bottom: all plants were rewatered after 16 days. Images were obtained, after repositioning the pots for photography, 3 days after rewatering. The total number of surviving plants is shown in the experiment with 30 plants. Differences in average numbers of surviving plants in the pots between the wild type and other plants analyzed using Student's t-test were statistically significant (*P < 0.05). The reproducible results were obtained from two independent experiments.(b) Metabolo-type of the samples after rewatering in the drought stress experiment. The freeze-dried samples were analyzed by untargeted profiling using liquid chromatography quadrupole time-of-flight mass spectroscopy. Processed data, including 2084 metabolite peaks after alignment, de-isotoping, noise cutting, and normalization were used for PCA using simca-P 11.5 software. The plot of PC1 (39.0%) versus PC2 (17.5%) is presented. Col-0, black squares; tt4, orange circles; MYB12OX/tt4, green triangles; pap1-D/tt4, purple squares; MYB12OX, red circles; pap1-D, blue diamonds; WOX1-1, pink crosses; and WOX1-2, yellow triangles.(c) Relative intensity of A11 after drought stress and rewatering. Differences of the intensities between the wild type and other plants were analyzed using Student's t-test (*P < 0.05). Differences and standard deviations (error bars) were calculated from the results of three biological replicates.(d) H2O2 generation in the wild type, tt4, flavonoid-deficient MYB overexpressors, and MYB overexpressors after drought stress. H2O2 production was detected by 3,3′-diaminobenzidine polymerization. The representative results are shown, and the reproducible results were obtained from three biological replicates.
To investigate the flavonoids in Arabidopsis that mitigate ROS in vivo, 3,3′-diaminobenzidine (DAB) staining, which is a marker of H2O2 accumulation in the presence of peroxidase (Thordal-Christensen et al., 1997), was performed using the detached leaves of 3-week-old plants incubated under drought conditions. The DAB staining patterns showed that greater anthocyanin accumulation mitigated H2O2 accumulation (Figure(Figure88d).
The relative water content in whole plants and detached leaves of the MYB12OX, pap1-D, and WOX1 lines showed a slightly greater decreasing trend in the water loss experiments than that of the wild type (Figure(Figure9a,b),9a,b), suggesting a positive correlation between flavonoid accumulation and the prevention of water loss.
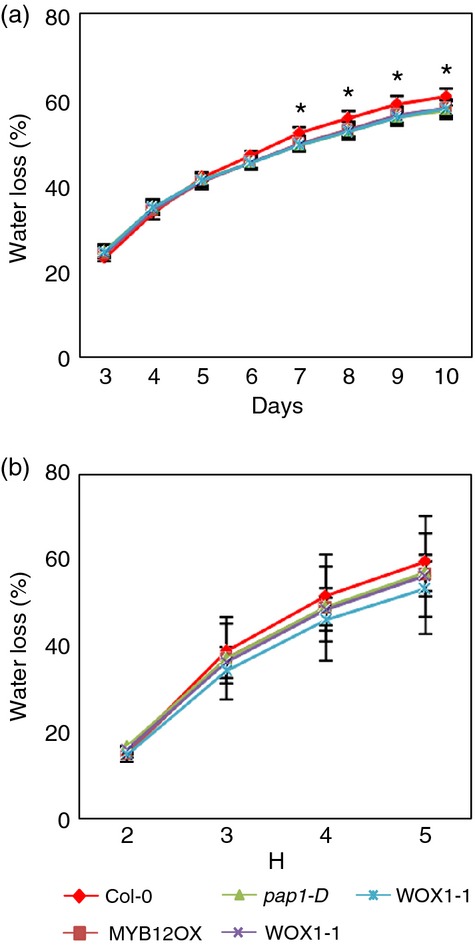
Water loss in the wild type, MYB12OX, pap1-D, WOX1-1, and WOX1-2.(a) Water loss in plants grown on soil during drought stress. Five plants were grown in a pot. The weight of the pot was calculated for water loss. Differences and standard deviations (error bars) were calculated from the results of six biological replicates. Differences between the wild type and other plants were analyzed using Student's t-test. The star indicates statistical significance (P < 0.05) between the wild type and MYB12OX, pap1-D, and WOX.(b) Water loss in detached leaves (10 leaves) of 3-week-old plants. Differences and standard deviations (error bars) were calculated from the results of three biological replicates. Differences between the wild type and other plants were analyzed using Student's t-test. There was no statistically significant difference between the wild type and other plants.
Discussion
There is growing interest in the functions of specialized metabolites in plants because such functions may be beneficial for agriculture (Varshney et al., 2012). Metabolomics and transcriptomics are powerful tools for understanding cellular responses under a given condition and for identifying the functions of genes and metabolites. Many responses to environmental stresses are common among plant species, including transcriptional activation of stress-related genes for acquisition of stress tolerance, accumulation of compatible solutes to retain water in cells and of antioxidants to protect cells against environmental stresses. However, no direct experimental evidence links flavonoids and stress tolerance. In general, wild-type, flavonoid-deficient mutant(s) and flavonoid-specific MYB overexpressor(s) are useful for discussing stress tolerance due to flavonoid accumulation. However, the bottleneck in the experimental design is to precisely evaluate the effect of flavonoid accumulation or MYB overexpression in a given result. Therefore, a series of Arabidopsis lines overaccumulating and lacking flavonoids under MYB overexpression was required to identify flavonoid function. In this study, the effects of both MYB overexpression and flavonoid overaccumulation on oxidative and drought tolerance were distinguished by comparing the wild-type, tt4, the flavonoid-deficient MYB overexpressors MYB12OX/tt4 and pap1-D/tt4, the single MYB overexpressors MYB12OX and pap1-D, and the double MYB overexpressors WOX1-1 and WOX1-2. We showed that flavonoid accumulation, which results in radical scavenging activity in vitro, leads to the enhancement of oxidative and drought tolerance in vivo. We also showed that flavonoid accumulation tends to prevent water loss.
Data from metabolome, transcriptome, and plant hormone analyses under the unstressed growth condition suggested that overexpressing MYB and flavonoid overaccumulation had no effect on the ABA level (Figure S5), which is a representative plant hormone involved in signaling in abiotic stress responses and in the expression of ABA biosynthetic genes and stress-related genes excluding the six flavonoid biosynthetic genes (Figure(Figure6).6). The MYB-overexpressing plants did not show enhanced drought tolerance through ABA-induced abiotic stress response mechanisms under the unstressed condition. EDL3, encoding an F-box protein, functions positively during ABA signaling and negatively during anthocyanin biosynthesis (Koops et al., 2011). EDL3 expression did not change significantly in the transcriptome and real-time PCR analyses, indicating that the stress signaling pathway including ABA and EDL3 for both the flavonoid biosynthetic pathway and abiotic stress response was not affected by the induction of flavonoid biosynthesis. Increased levels of the plant hormones ABA and cytokinins play a key role in drought tolerance in tobacco (Nicotiana tabacum) (Rivero et al., 2007), suggesting that the slight increase in cytokinin accumulation in the MYB overexpressors was unrelated to enhanced drought tolerance (Figure S5). The relationship between flavonoid and cytokinin biosynthesis remains to be clarified and further research is necessary.
Our results suggest that anthocyanin overaccumulation is directly associated with oxidative and drought tolerance, given that the anthocyanin-overaccumulating plants showed such tolerance whereas the flavonoid-deficient overexpressing plants exhibited apparently the same phenotype as the wild type and tt4 (Figures(Figures7c7c and and8a).8a). Cyclic single electron reduction/oxidation of the methyl viologen reagent is a critical event (redox cycling). Redox cycling is linked to two important events related to the development of toxicity: depletion of NADPH and generation of ROS (Winterbourn, 1981; Bus and Gibson, 1984). Mitigation of ROS accumulation by anthocyanin overaccumulation resulted in oxidative tolerance in the pap1-D and WOX1 lines (Figure(Figure7c).7c). Drought stress induces the accumulation of ROS in plants (Vickers et al., 2009). Staining with DAB, which is a marker of H2O2 accumulation in vivo (Thordal-Christensen et al., 1997), clearly showed that anthocyanin overaccumulation reduced the accumulation of H2O2 in vivo (Figure(Figure8d).8d). A common factor between oxidative and drought stress is the accumulation of ROS, which are mainly produced in chloroplasts and peroxisomes (Kim et al., 2008). Reactive oxygen species play an important role as signaling molecules that initiate stress responses in plants. However, overaccumulation of ROS causes a chain reaction of ROS production. Cytosolic H2O2 enters the vacuoles and subsequently initiates a chain reaction resulting in excess ROS accumulation (Bolouri-Moghaddam et al., 2010; Agati et al., 2012). The increased generation of ROS in the cellular components is attributed to a disturbance in cellular homeostasis such as programmed cell death (Vickers et al., 2009) or death by cytotoxicity (Kim et al., 2008). Our results suggest that anthocyanins, which generally accumulate in the vacuoles (Hernandez et al., 2009), prevent oxidative damage, making it possible for the anthocyanin-overaccumulating plants to exhibit oxidative and drought tolerance. The function of anthocyanins against ROS can be inferred from their strong antioxidative activity in vitro. Cyanidin-type anthocyanins and quercetin-type flavonols were responsible for the strong radical scavenging activity in the in vitro DPPH assay (Figure(Figure7)7) (Seyoum et al., 2006). The presence of hydroxyl group(s) on the aglycone moiety is important for scavenging DPPH, whereas the sugar and acyl moieties do not appear to be essential (Tohge et al., 2005b; Seyoum et al., 2006). The mechanism of mitigation of ROS by such anthocyanins remains to be clarified. Therefore for the next step it will be important to reveal what type of ROS can be mitigated by such anthocyanins. Partial evidence for flavonoids as antioxidants in vivo is accumulating gradually. Flavonoid deficiency increases H2O2 in Arabidopsis (Zhang et al., 2012). Anthocyanins are suitably located to account for the enhanced rates of H2O2 production (Chalker-Scott, 2002). In addition to anthocyanins, other specialized metabolites such as hydroxycinnamate esters, which are believed to be radical scavengers (Ferreres et al., 2011), also accumulate in vacuoles. Overaccumulation of hydroxycinnamate ester confers UV-B tolerance in Arabidopsis (Jin et al., 2000). These results suggest that specialized metabolites play a role in scavenging ROS in vacuoles to enhance stress tolerance.
It has been suggested that anthocyanins as antioxidants are likely to be osmoregulators that maintain water homeostasis (Do and Cormier, 1990; Chalker-Scott, 2002; Hughes et al., 2010, 2013; Hughes, 2011; Sperdouli and Moustakas, 2012), together with compatible solutes such as sugars (e.g. mannitol, sorbitol, and trehalose) and amino acids (e.g. proline), which help to adjust homeostasis and scavenge ROS in various plant species (Chen and Murata, 2002). We revealed that flavonoid overaccumulation prevented water loss from whole plants and detached leaves (Figures(Figures8a8a and and9).9). Our data suggest that anthocyanins in Arabidopsis may play a role in water homeostasis by scavenging ROS into vacuoles under drought stress. Stomatal closure reduces plant water loss through transpiration during drought stress (Hirayama and Shinozaki, 2010). A previous study in tobacco with an altered level of the antioxidant ascorbic acid reported a change in stomatal closure, leading to the prevention of wilting and water loss. In comparative analysis with the wild type, plants overexpressing or suppressing dehydroascorbate reductase (DHAR) revealed that increasing the redox state of ascorbic acid resulted in an increase in the transpiration rate and stomatal conductance under normal growth conditions. Furthermore, guard cells with a higher redox state of ascorbic acid were less responsive to H2O2 or ABA signaling, and the plants exhibited increased water loss after drought conditions were initiated (Chen and Gallie, 2004). Taken together with previous results and our data, there may be no direct association between anthocyanins, which do not accumulate in substantial amounts, guard cells (Gallagher and Smith, 2000), and stomatal closure.
Flavonoids, which have various useful in vitro activities (Harborne and Williams, 2000), are useful in crop production. Increased flavonoid accumulation was achieved by crossing MYB12OX and pap1-D. As expected, the major difference between the other lines was flavonoid accumulation (Figure(Figure5a).5a). In fact, the WOX1 lines accumulated higher levels of flavonols and anthocyanins than each single overexpressing line. The profile of flavonols and anthocyanins in the WOX1 lines was identical to that in the MYB12OX or pap1-D lines. The enhanced flavonoid overaccumulation in the WOX lines was probably initiated independently by MYB12 and PAP1. The Arabidopsis triple mutant myb11myb12myb111 suggests the independent function of MYB12 and PAP1 (Stracke et al., 2010b). Ectopic overexpression of single MYB transcription factors resulted in the induction of flavonoid biosynthesis without significantly inhibiting plant growth (Figure S1). The combined overexpression of MYB12 and PAP1 also resulted in no significant difference in the WOX1 lines. Furthermore, the flavonoid-deficient MYB12OX/tt4 and pap1-D/tt4 lines were generated by crossing tt4 and MYB12OX or pap1-D, respectively, with no or minimal growth penalty. A recent report revealed that MYB12OX is UV-B tolerant (Stracke et al., 2010a), and MYB12-overexpressing tobacco is tolerant against insects such as Spodoptera litura and Helicoverpa armigera because of flavonoid overaccumulation (Misra et al., 2010). Purple tomato fruit, which ectopically overexpresses two transcription factors Delila and Rosea1 from snapdragons, has increased shelf-life and reduced susceptibility to Botrytis cinerea, an important post-harvest pathogen (Zhang et al., 2013). These reports suggest that flavonoid overaccumulation can be a key to concurrently enhancing tolerance to multiple stressors. Our results also suggest a useful indication of how to generate promising novel crop varieties with enhanced stress tolerance. Cross-fertilization using varieties containing high amounts of flavonoids will provide new insights into tolerance to biotic and abiotic stresses, potentially leading to increased crop production. Flavonoids are hypothetically produced as antioxidants in the late response against UV-B stress (Kusano et al., 2011). Insights into biotic and abiotic stress tolerance due to flavonoid accumulation in crops will be elicited during long-term stress exposure.
Methods
Plant materials and growth conditions
Arabidopsis thaliana (Col-0) plants were used as the wild type in this study. MYB12OX and pap1-D were described previously (Borevitz et al., 2000; Mehrtens et al., 2005). Homozygous WOX1-1 and WOX1-2 were prepared by crossing MYB12OX and pap1-D, followed by self-fertilization of the F1 generation. Two progenies, exhibiting both Basta and kanamycin tolerance in the F2 and F3 generations, were obtained and named WOX1-1 and WOX1-2. The homozygous MYB12OX/tt4 and pap1-D/tt4 lines were prepared by crossing MYB12OX or pap1-D with tt4, respectively. Progenies that exhibited both Basta and kanamycin tolerance (F2 and F3 generations) were obtained. Sterilized seeds were kept for 2 days at 4°C in the dark and were sown on plates of growth medium (GM) agar containing 1% sucrose (Valvekens et al., 1988). Black paper was used for wrapping the plates used for the light-shielding condition. All plates were set in a growth chamber at 22°C and a relative humidity of approximately 60% under a 16-h light (approximately 40 μmol sec−1 m−2)/8-h dark cycle. The drought stress experiment was conducted at 21°C and 40 ± 5% relative humidity under 16 h/8 h light/dark conditions in a plant growth room. Harvested plant samples were immediately frozen in liquid nitrogen and stored at −80°C until use.
Flavonoid-targeted profiling by LC PDA MS
This analysis was performed as described previously (Tohge et al., 2005a), except that the column was changed to Atlantis® T3 (5 μm, 4.6 mm × 150 mm; Waters, http://www.waters.com/).
Untargeted profiling by LC QTOF MS
Frozen or freeze-dried samples were extracted with 5 or 50 μl of 80% methanol (MeOH) containing 2.5 μm lidocaine per milligram fresh or dry weight, respectively, which was an internal standard for positive mode analysis, using a mixer mill (MM300; Retsch, http://www.retsch.com/) together with one zirconia bead per tube for 10 min at 20 Hz. After centrifugation at 15 000 g for 10 min and filtration using an Oasis HLB 96-well μElution Plate (Waters), the extracts (1 μl) were analyzed by LC QTOF MS (LC, Waters Acquity UPLC system; MS, Waters Xevo G2 Q-Tof). The analytical conditions were as follows. For LC: column, Acquity bridged ethyl hybrid (BEH) C18 (1.7 μm, 2.1 mm × 100 mm, Waters); solvent system, solvent A (water including 0.1% formic acid) and solvent B (acetonitrile including 0.1% formic acid); gradient program, 99.5% A/0.5% B at 0 min, 99.5% A/0.5% B at 0.1 min, 20% A/80% B at 10 min, 0.5% A/99.5% B at 10.1 min, 0.5% A/99.5% B at 12.0 min, 99.5% A/0.5% B at 12.1 min, and 99.5% A/0.5% B at 15.0 min; flow rate, 0.3 ml min−1; column temperature, 40°C. For MS: capillary voltage, +3.0 keV, cone voltage, 25.0 V; source temperature, 120°C; desolvation temperature, 450°C; cone gas flow, 50 L h−1; desolvation gas flow, 800 L h−1; collision energy, 6 V; mass range, m/z 100–1500; scan duration, 0.1 sec; interscan delay, 0.014 sec; mode, centroid; polarity, positive; lockspray (leucine enkephalin); scan duration, 1.0 sec; interscan delay, 0.1 sec. The data matrix was aligned using MassLynx ver. 4.1 (Waters). After alignment, de-isotoping, and cutoff of the low-intensity peaks (<500 counts) and the intensity values of the remaining peaks were divided by those of lidocaine ([M + H]+, m/z 235.1804) for normalization. The processed data were used for PCA using SIMCA-P 11.5. The log2-transformed value was used for HCA using MeV 4.8 (http://mev.tm4.org/). All metabolome data acquired by LC QTOF MS were deposited at DROP Met in PRIMe (http://prime.psc.riken.jp/) and are freely available. These data were also deposited in MetaboLights (accession number MTBLS57) (Steinbeck et al., 2012; Salek et al., 2013) and MetabolomeExpress (Carroll et al., 2010).
Transcriptome profiling by DNA microarray
Total RNA samples were extracted using an RNeasy Plant Mini Kit (Qiagen, http://www.qiagen.com/). Transcriptome profiling using Affymetrix ATH1 GeneChips® (24K) was performed according to the manufacturer's instructions. The r package from Bioconductor (http://www.bioconductor.org/) was used for the normalization (MAS5) of the CELL files. After filtering using Student's t-test (P < 0.05) between the wild type and other plants, all genes were then filtered with a fold change of ≥2.0 in the expression level between the wild type and each mutant. Only 907 genes in the other lines were considered. The fold change value was calculated as log2 (Dataset S1). Gene Ontology analysis (TAIR10; http://www.arabidopsis.org/) was performed to annotate 245 biotic and abiotic stress-related genes. The log2-transformed value was used for HCA using MeV 4.8. The data discussed here were also deposited in NCBI's Gene Expression Omnibus (Edgar et al., 2002) and are accessible through the GEO Series accession number GSE 51215.
Real-time PCR
Total RNA was extracted using the RNeasy Plant Mini Kit (Qiagen) and cDNA was synthesized using the SuperScript III First-Strand Synthesis System for RT-PCR (Invitrogen, http://www.invitrogen.com/) according to the manufacturer's instructions. The primers for MYB12 and PAP1 were used as described previously (Stracke et al., 2007; Gonzalez et al., 2008). The PCR programme for amplification was as follows: 95°C for 20 sec, 95°C for 3 sec, and 60°C for 30 sec (40 cycles).
Assay of radical scavenging activity
Radical scavenging activity was measured using DPPH as described previously (Nakajima et al., 2004; Tohge et al., 2005b).
Chemicals
Trolox and flavonoid standard compounds were purchased from Kanto Chemical (http://www.kanto.co.jp/english/) and Extrasynthese (http://www.extrasynthese.com/). DPPH was purchased from Wako Pure Chemical Industries (http://www.wako-chem.co.jp/english/).
Assay of standard compounds
Each standard solvent (2 mm) and DPPH solvent (200 μm) was prepared in a solvent mixture (MeOH:H2O:CH3COOH = 9:10:1) for this assay. After allowing the mixture of each standard solvent (10 μl) and DPPH solvent (390 μl) to sit for 5 min, DPPH absorbance was measured at 517 nm.
Assay of the acidic MeOH–water extracts of wild type, tt4, flavonoid-deficient MYB overexpressors, and MYB overexpressors
Whole parts of 3-week-old plants grown on GM plates in the growth chamber were extracted with 5 μl solvent (MeOH:H2O:CH3COOH = 9:10:1) per milligram fresh weight (FW). After allowing a mixture of each extraction solvent (50 μl) and the DPPH solvent (450 μl) to sit for 5 min, DPPH absorbance was measured at 517 nm.
Oxidative stress treatment using methyl viologen
Three-week-old plants grown on GM plates were transferred to GM plates containing 10 μm methyl viologen (Wako Pure Chemical Industries) and were incubated for 21 days. After the stress treatment, frozen aerial parts were extracted with 20 μl MeOH per milligram fresh weight (FW). The UV absorption of the remaining chlorophylls (663 nm) was measured.
Drought stress treatment
This experiment was conducted using Arasystem (Beta-Tech BVBA, http://www.arasystem.com/). Seeds of each line were sown in soil [Pro-Mix BX (Premier Tech Horticulture Inc., http://www.pthorticulture.com/): vermiculite = 2:1, supplemented with fertilizer] and were grown in the plant growth room. After germination, three plants per pot were used for the drought stress experiments. The plants were last watered on day 20 after germination. Three-week-old plants were exposed to drought stress by stopping watering. Trays with pots were turned 90° every day. The plants were rewatered after 16 days. The number of surviving plants was counted 3 days after watering.
3,3′-Diaminobenzidine staining
Three-week-old plants were incubated in 2-ml tubes with 2 ml of water for 3 days in the growth chamber to assess drought stress. The detached leaves of the incubated plants were used for DAB staining (Thordal-Christensen et al., 1997).
Water loss experiment
Two-week-old plants were transferred from agar plates to soil conditions (five plants per pot). After a 1-week conditioning period, the plants were subjected to drought stress by withholding water. The weight of the pots was calculated at each time point. Another experiment was performed using detached leaves. The weight of 10 leaves from 3-week-old plants grown on GM medium was calculated at each time point.
Acknowledgments
We thank Professor Bernd Weisshaar and Dr Ralf Stracke (Bielefeld University, Germany) for providing MYB12OX, Dr Richard A. Dixon (University of North Texas, USA) for providing pap1-D, and Professor Hiromitsu Takayama, Dr Mariko Kitajima (Chiba University, Japan), Dr Rie Nishiyama (RIKEN CSRS), and Dr Makoto Suzuki (Kobe University) for useful comments. We also thank Tomoko Narisawa, Tamiko Asano, Toshiyo Motojima, Masami Nanri, Kiyoko Morohoshi, Chieko Komori, and Tetsuya Mori (RIKEN CSRS) for excellent technical assistance. This work was supported, in part, by: CREST, JST; Grants-in-aid from the Ministry of Education, Culture, Sports, Science and Technology, Japan; Japan Advanced Plant Science Network; Strategic International Research Cooperative Program (SICP), JST; and Strategic International Collaborative Research Program (SICORP), JST.
Supporting Information
Additional Supporting Information may be found in the online version of this article.
Figure S2
Plant samples used for flavonoid-targeted profiling using liquid chromatography photodiode array mass spectrometry.
Figure S3
Breakdown of total flavonoids detected in flavonoid-targeted profiling using liquid chromatography photodiode array mass spectrometry.
Figure S5
Concentration of plant hormones and their derivatives in the wild type and MYB overexpressors.
Figure S6
Phenotype of the wild type, tt4, flavonoid-deficient MYB overexpressors, and MYB overexpressors after rewatering following drought stress.
Table S1
Characterization of known metabolites detected during flavonoid-targeted profiling using liquid chromatography photodiode array mass spectrometry.
Table S2
Characterization of new anthocyanins detected during flavonoid-targeted profiling using liquid chromatography photodiode array mass spectrometry.
Dataset S1
Biotic and abiotic stress-related genes induced in tt4, flavonoid-deficient MYB overexpressors, and MYB overexpressors compared with the wild type.
Method S1
Plant hormone analysis by liquid chromatography tandem mass spectrometry.
References
- Agati G, Azzarello E, Pollastri S, Tattini M. Flavonoids as antioxidants in plants: location and functional significance. Plant Sci. 2012;196:67–76. [Abstract] [Google Scholar]
- Bais HP, Vepachedu R, Gilroy S, Callaway RM, Vivanco JM. Allelopathy and exotic plant invasion: from molecules and genes to species interactions. Science. 2003;301:1377–1380. [Abstract] [Google Scholar]
- Bolouri-Moghaddam MR, Le Roy K, Xiang L, Rolland F, Van den Ende W. Sugar signalling and antioxidant network connections in plant cells. FEBS J. 2010;277:2022–2037. [Abstract] [Google Scholar]
- Borevitz JO, Xia YJ, Blount J, Dixon RA, Lamb C. Activation tagging identifies a conserved MYB regulator of phenylpropanoid biosynthesis. Plant Cell. 2000;12:2383–2393. [Abstract] [Google Scholar]
- Bus JS, Gibson JE. Paraquat: model for oxidant-initiated toxicity. Environ. Health Perspect. 1984;55:37–46. [Europe PMC free article] [Abstract] [Google Scholar]
- Butelli E, Titta L, Giorgio M, et al. Enrichment of tomato fruit with health-promoting anthocyanins by expression of select transcription factors. Nat. Biotechnol. 2008;26:1301–1308. [Abstract] [Google Scholar]
- Butelli E, Licciardello C, Zhang Y, Liu J, Mackay S, Bailey P, Reforgiato-Recupero G, Martin C. Retrotransposons control fruit-specific, cold-dependent accumulation of anthocyanins in blood oranges. Plant Cell. 2012;24:1242–1255. [Abstract] [Google Scholar]
- Carroll AJ, Badger MR, Harvey Millar A. The MetabolomeExpress Project: enabling web-based processing, analysis and transparent dissemination of GC/MS metabolomics datasets. BMC Bioinformatics. 2010;11:376. [Europe PMC free article] [Abstract] [Google Scholar]
- Catala R, Medina J, Salinas J. Integration of low temperature and light signaling during cold acclimation response in Arabidopsis. Proc. Natl. Acad. Sci. USA. 2011;108:16475–16480. [Europe PMC free article] [Abstract] [Google Scholar]
- Chalker-Scott L. Do anthocyanins function as osmoregulators in leaf tissues? Adv. Bot. Res. 2002;37:103–127. [Google Scholar]
- Chen Z, Gallie DR. The ascorbic acid redox state controls guard cell signaling and stomatal movement. Plant Cell. 2004;16:1143–1162. [Abstract] [Google Scholar]
- Chen TH, Murata N. Enhancement of tolerance of abiotic stress by metabolic engineering of betaines and other compatible solutes. Curr. Opin. Plant Biol. 2002;5:250–257. [Abstract] [Google Scholar]
- Dixon RA, Paiva NL. Stress-induced phenylpropanoid metabolism. Plant Cell. 1995;7:1085–1097. [Abstract] [Google Scholar]
- Do CB, Cormier F. Accumulation of anthocyanins enhanced by a high osmotic potential in grape (Vitis Vinifera L.) Cell-Suspensions. Plant Cell Rep. 1990;9:143–146. [Abstract] [Google Scholar]
- Dubos C, Stracke R, Grotewold E, Weisshaar B, Martin C, Lepiniec L. MYB transcription factors in Arabidopsis. Trends Plant Sci. 2010;15:573–581. [Abstract] [Google Scholar]
- Edgar R, Domrachev M, Lash AE. Gene Expression Omnibus: NCBI gene expression and hybridization array data repository. Nucleic Acids Res. 2002;30:207–210. [Europe PMC free article] [Abstract] [Google Scholar]
- Ferreres F, Figueiredo R, Bettencourt S, et al. Identification of phenolic compounds in isolated vacuoles of the medicinal plant Catharanthus roseus and their interaction with vacuolar class III peroxidase: an H2O2 affair? J. Exp. Bot. 2011;62:2841–2854. [Abstract] [Google Scholar]
- Field B, Jordan F, Osbourn A. First encounters–deployment of defence-related natural products by plants. New Phytol. 2006;172:193–207. [Abstract] [Google Scholar]
- Fujita M, Fujita Y, Iuchi S, Yamada K, Kobayashi Y, Urano K, Kobayashi M, Yamaguchi-Shinozaki K, Shinozaki K. Natural variation in a polyamine transporter determines paraquat tolerance in Arabidopsis. Proc. Natl Acad. Sci. USA. 2012;109:6343–6347. [Europe PMC free article] [Abstract] [Google Scholar]
- Fukushima A, Kusano M, Redestig H, Arita M, Saito K. Integrated omics approaches in plant systems biology. Curr. Opin. Chem. Biol. 2009;13:532–538. [Abstract] [Google Scholar]
- Gallagher K, Smith LG. Roles for polarity and nuclear determinants in specifying daughter cell fates after an asymmetric cell division in the maize leaf. Curr. Biol. 2000;10:1229–1232. [Abstract] [Google Scholar]
- Gilbert N. Drought devastates US crops. Nature. 2012 . 10.1038/nature.2012.11065. [Google Scholar]
- Gonzalez A, Zhao M, Leavitt JM, Lloyd AM. Regulation of the anthocyanin biosynthetic pathway by the TTG1/bHLH/Myb transcriptional complex in Arabidopsis seedlings. Plant J. 2008;53:814–827. [Abstract] [Google Scholar]
- Harborne JB, Williams CA. Advances in flavonoid research since 1992. Phytochemistry. 2000;55:481–504. [Abstract] [Google Scholar]
- Hemm MR, Rider SD, Ogas J, Murry DJ, Chapple C. Light induces phenylpropanoid metabolism in Arabidopsis roots. Plant J. 2004;38:765–778. [Abstract] [Google Scholar]
- Hernandez I, Alegre L, Van Breusegem F, Munne-Bosch S. How relevant are flavonoids as antioxidants in plants? Trends Plant Sci. 2009;14:125–132. [Abstract] [Google Scholar]
- Hirayama T, Shinozaki K. Research on plant abiotic stress responses in the post-genome era: past, present and future. Plant J. 2010;61:1041–1052. [Abstract] [Google Scholar]
- Hughes NM. Winter leaf reddening in ‘evergreen’ species. New Phytol. 2011;190:573–581. [Abstract] [Google Scholar]
- Hughes NM, Reinhardt K, Feild TS, Gerardi AR, Smith WK. Association between winter anthocyanin production and drought stress in angiosperm evergreen species. J. Exp. Bot. 2010;61:1699–1709. [Europe PMC free article] [Abstract] [Google Scholar]
- Hughes NM, Carpenter KL, Cannon JG. Estimating contribution of anthocyanin pigments to osmotic adjustment during winter leaf reddening. J. Plant Physiol. 2013;170:230–233. [Abstract] [Google Scholar]
- Jin H, Cominelli E, Bailey P, Parr A, Mehrtens F, Jones J, Tonelli C, Weisshaar B, Martin C. Transcriptional repression by AtMYB4 controls production of UV-protecting sunscreens in Arabidopsis. EMBO J. 2000;19:6150–6161. [Europe PMC free article] [Abstract] [Google Scholar]
- Kim CH, Meskauskiene R, Apel K, Laloi C. No single way to understand singlet oxygen signalling in plants. EMBO Rep. 2008;9:435–439. [Europe PMC free article] [Abstract] [Google Scholar]
- Koops P, Pelser S, Ignatz M, Klose C, Marrocco-Selden K, Kretsch T. EDL3 is an F-box protein involved in the regulation of abscisic acid signalling in Arabidopsis thaliana. J. Exp. Bot. 2011;62:5547–5560. [Europe PMC free article] [Abstract] [Google Scholar]
- Kusano M, Tohge T, Fukushima A, et al. Metabolomics reveals comprehensive reprogramming involving two independent metabolic responses of Arabidopsis to UV-B light. Plant J. 2011;67:354–369. [Abstract] [Google Scholar]
- Lei MG, Liu YD, Zhang BC, Zhao YT, Wang XJ, Zhou YH, Raghothama KG, Liu D. Genetic and genomic evidence that sucrose is a global regulator of plant responses to phosphate starvation in Arabidopsis. Plant Physiol. 2011;156:1116–1130. [Abstract] [Google Scholar]
- Matsuda F, Hirai MY, Sasaki E, Akiyama K, Yonekura-Sakakibara K, Provart NJ, Sakurai T, Shimada Y, Saito K. AtMetExpress development: a phytochemical atlas of arabidopsis development. Plant Physiol. 2010;152:566–578. [Abstract] [Google Scholar]
- Mehrtens F, Kranz H, Bednarek P, Weisshaar B. The Arabidopsis transcription factor MYB12 is a flavonol-specific regulator of phenylpropanoid biosynthesis. Plant Physiol. 2005;138:1083–1096. [Abstract] [Google Scholar]
- Misra P, Pandey A, Tiwari M, et al. Modulation of transcriptome and metabolome of tobacco by arabidopsis transcription factor, AtMYB12. Leads to Insect Resistance. Plant Physiol. 2010;152:2258–2268. [Abstract] [Google Scholar]
- Nakabayashi R, Kusano M, Kobayashi M, Tohge T, Yonekura-Sakakibara K, Kogure N, Yamazaki M, Kitajima M, Saito K, Takayama H. Metabolomics-oriented isolation and structure elucidation of 37 compounds including two anthocyanins from Arabidopsis thaliana. Phytochemistry. 2009;70:1017–1029. [Abstract] [Google Scholar]
- Nakajima J, Tanaka I, Seo S, Yamazaki M, Saito K. LC/PDA/ESI-MS profiling and radical scavenging activity of anthocyanins in various berries. J. Biomed. Biotechnol. 2004;5:241–247. [Europe PMC free article] [Abstract] [Google Scholar]
- Rivero RM, Kojima M, Gepstein A, Sakakibara H, Mittler R, Gepstein S, Blumwald E. Delayed leaf senescence induces extreme drought tolerance in a flowering plant. Proc. Natl Acad. Sci. USA. 2007;104:19631–19636. [Europe PMC free article] [Abstract] [Google Scholar]
- Rowan DD, Cao MS, Lin-Wang K, et al. Environmental regulation of leaf colour in red 35S:PAP1 Arabidopsis thaliana. New Phytol. 2009;182:102–115. [Abstract] [Google Scholar]
- Saito K, Matsuda F. Metabolomics for functional genomics, systems biology, and biotechnology. Annu. Rev. Plant Biol. 2010;61:463–489. [Abstract] [Google Scholar]
- Saito K, Yonekura-Sakakibara K, Nakabayashi R, Higashi Y, Yamazaki M, Tohge T, Fernie AR. The flavonoid biosynthetic pathway in Arabidopsis: structural and genetic diversity. Plant Physiol. Biochem. 2013;72:21–34. [Abstract] [Google Scholar]
- Salek RM, Haug K, Conesa P, et al. The MetaboLights repository: curation challenges in metabolomics. Database (Oxford) 2013;2013:bat029. [Europe PMC free article] [Abstract] [Google Scholar]
- Seyoum A, Asres K, El-Fiky FK. Structure-radical scavenging activity relationships of flavonoids. Phytochemistry. 2006;67:2058–2070. [Abstract] [Google Scholar]
- Shirley BW, Kubasek WL, Storz G, Bruggemann E, Koornneef M, Ausubel FM, Goodman HM. Analysis of Arabidopsis mutants deficient in flavonoid biosynthesis. Plant J. 1995;8:659–671. [Abstract] [Google Scholar]
- Sperdouli I, Moustakas M. Interaction of proline, sugars, and anthocyanins during photosynthetic acclimation of Arabidopsis thaliana to drought stress. J. Plant Physiol. 2012;169:577–585. [Abstract] [Google Scholar]
- Steinbeck C, Conesa P, Haug K, Mahendraker T, Williams M, Maguire E, Rocca-Serra P, Sansone SA, Salek RM, Griffin JL. MetaboLights: towards a new COSMOS of metabolomics data management. Metabolomics. 2012;8:757–760. [Europe PMC free article] [Abstract] [Google Scholar]
- Stracke R, Ishihara H, Barsch GHA, Mehrtens F, Niehaus K, Weisshaar B. Differential regulation of closely related R2R3-MYB transcription factors controls flavonol accumulation in different parts of the Arabidopsis thaliana seedling. Plant J. 2007;50:660–677. [Europe PMC free article] [Abstract] [Google Scholar]
- Stracke R, Favory JJ, Gruber H, Bartelniewoehner L, Bartels S, Binkert M, Funk M, Weisshaar B, Ulm R. The Arabidopsis bZIP transcription factor HY5 regulates expression of the PFG1/MYB12 gene in response to light and ultraviolet-B radiation. Plant, Cell Environ. 2010a;33:88–103. [Abstract] [Google Scholar]
- Stracke R, Jahns O, Keck M, Tohge T, Niehaus K, Fernie AR, Weisshaar B. Analysis of PRODUCTION OF FLAVONOL GLYCOSIDES-dependent flavonol glycoside accumulation in Arabidopsis thaliana plants reveals MYB11-, MYB12- and MYB111-independent flavonol glycoside accumulation. New Phytol. 2010b;188:985–1000. [Abstract] [Google Scholar]
- Thordal-Christensen H, Zhang ZG, Wei YD, Collinge DB. Subcellular localization of H2O2 in plants. H2O2 accumulation in papillae and hypersensitive response during the barley-powdery mildew interaction. Plant J. 1997;11:1187–1194. [Google Scholar]
- Tohge T, Nishiyama Y, Hirai MY, et al. Functional genomics by integrated analysis of metabolome and transcriptome of Arabidopsis plants over-expressing an MYB transcription factor. Plant J. 2005a;42:218–235. [Abstract] [Google Scholar]
- Tohge T, Matsui K, Ohme-Takagi M, Yamazaki M, Saito K. Enhanced radical scavenging activity of genetically modified Arabidopsis seeds. Biotechnol. Lett. 2005b;27:297–303. [Abstract] [Google Scholar]
- Tohge T, Watanabe M, Hoefgen R, Fernie AR. The evolution of phenylpropanoid metabolism in the green lineage. Crit. Rev. Biochem. Mol. Biol. 2013;48:123–152. [Abstract] [Google Scholar]
- Valvekens D, Vanmontagu M, Vanlijsebettens M. Agrobacterium tumefaciens-mediated transformation of Arabidopsis thaliana root explants by using kanamycin selection. Proc. Natl Acad. Sci. USA. 1988;85:5536–5540. [Europe PMC free article] [Abstract] [Google Scholar]
- Varshney RK, Ribaut JM, Buckler ES, Tuberosa R, Rafalski JA, Langridge P. Can genomics boost productivity of orphan crops? Nat. Biotechnol. 2012;30:1172–1176. [Abstract] [Google Scholar]
- Vickers CE, Gershenzon J, Lerdau MT, Loreto F. A unified mechanism of action for volatile isoprenoids in plant abiotic stress. Nat. Chem. Biol. 2009;5:283–291. [Abstract] [Google Scholar]
- Winterbourn CC. Production of hydroxyl radicals from paraquat radicals and H2O2. FEBS Lett. 1981;128:339–342. [Abstract] [Google Scholar]
- Yonekura-Sakakibara K, Tohge T, Matsuda F, Nakabayashi R, Takayama H, Niida R, Watanabe-Takahashi A, Inoue E, Saito K. Comprehensive flavonol profiling and transcriptome coexpression analysis leading to decoding gene-metabolite correlations in Arabidopsis. Plant Cell. 2008;20:2160–2176. [Abstract] [Google Scholar]
- Zhang Q, Su LJ, Chen JW, Zeng XQ, Sun BY, Peng CL. The antioxidative role of anthocyanins in Arabidopsis under high-irradiance. Biol. Plantarum. 2012;56:97–104. [Google Scholar]
- Zhang Y, Butelli E, De Stefano R, et al. Anthocyanins double the shelf life of tomatoes by delaying overripening and reducing susceptibility to gray mold. Curr. Biol. 2013;23:1094–1100. [Europe PMC free article] [Abstract] [Google Scholar]
Citations & impact
Impact metrics
Citations of article over time
Alternative metrics
Article citations
Integrated Analysis of Transcriptome and Metabolome Provides Insights into Flavonoid Biosynthesis of Blueberry Leaves in Response to Drought Stress.
Int J Mol Sci, 25(20):11135, 17 Oct 2024
Cited by: 0 articles | PMID: 39456917 | PMCID: PMC11508776
Physiological and differential protein expression analyses of the calcium stress response in the <i>Drynaria roosii</i> rhizome.
Heliyon, 10(19):e38260, 21 Sep 2024
Cited by: 0 articles | PMID: 39386768 | PMCID: PMC11462351
Impact of Abiotic Stress on Rice and the Role of DNA Methylation in Stress Response Mechanisms.
Plants (Basel), 13(19):2700, 26 Sep 2024
Cited by: 0 articles | PMID: 39409570 | PMCID: PMC11478684
Review Free full text in Europe PMC
Multi-omics analyses reveal the mechanisms underlying the responses of Casuarina equisetifolia ssp. incana to seawater atomization and encroachment stress.
BMC Plant Biol, 24(1):854, 12 Sep 2024
Cited by: 0 articles | PMID: 39266948 | PMCID: PMC11391710
Transcriptome dynamics in Artemisia annua provides new insights into cold adaptation and de-adaptation.
Front Plant Sci, 15:1412416, 29 Aug 2024
Cited by: 0 articles | PMID: 39268001 | PMCID: PMC11390472
Go to all (476) article citations
Other citations
Wikipedia
Data
Data behind the article
This data has been text mined from the article, or deposited into data resources.
BioStudies: supplemental material and supporting data
MetaboLights - Metabolomics experiments and derived information
- (1 citation) MetaboLights - MTBLS57
Similar Articles
To arrive at the top five similar articles we use a word-weighted algorithm to compare words from the Title and Abstract of each citation.
Drastic anthocyanin increase in response to PAP1 overexpression in fls1 knockout mutant confers enhanced osmotic stress tolerance in Arabidopsis thaliana.
Plant Cell Rep, 35(11):2369-2379, 25 Aug 2016
Cited by: 16 articles | PMID: 27562381
Alternation of flavonoid accumulation under drought stress in Arabidopsis thaliana.
Plant Signal Behav, 9(8):e29518, 01 Jan 2014
Cited by: 68 articles | PMID: 25763629 | PMCID: PMC4203635
Double-stranded RNA-binding protein DRB3 negatively regulates anthocyanin biosynthesis by modulating PAP1 expression in Arabidopsis thaliana.
J Plant Res, 130(1):45-55, 19 Dec 2016
Cited by: 9 articles | PMID: 27995376
Multiple Functions of MYB Transcription Factors in Abiotic Stress Responses.
Int J Mol Sci, 22(11):6125, 07 Jun 2021
Cited by: 129 articles | PMID: 34200125 | PMCID: PMC8201141
Review Free full text in Europe PMC