Abstract
Free full text

D-TRANSPOSITION OF THE GREAT ARTERIES: Hot Topics in the Current Era of the Arterial Switch Operation
Abstract
Objectives
This paper aims to update clinicians on “Hot Topics” in the management of patients with d-transposition of the great arteries (D-TGA) in the current surgical era.
Background
The arterial switch operation (ASO) has replaced atrial switch procedures for D-TGA and 90% of patients now reach adulthood.
Methods
The Adult Congenital and Pediatric Cardiology Council (ACPC) of the American College of Cardiology assembled a team of experts to summarize current knowledge on genetics, prenatal diagnosis, surgical timing, balloon atrial septostomy (BAS), prostaglandin therapy (PGE), intraoperative techniques, imaging, coronary obstruction, arrhythmias, sudden death, aortic dilation and regurgitation (AR), neurodevelopmental (ND) issues and lifelong care of D-TGA patients.
Results
In simple D-TGA, 1) familial recurrence risk is low; 2) children diagnosed prenatally have improved cognitive skills compared with those diagnosed postnatally; 3) echocardiography helps to identify risk factors; 4) routine use of BAS and PGE may not be beneficial in some cases; 5) early ASO improves outcomes and reduces costs with a low mortality. Single or intramural coronary arteries remain risk factors; 6) post-ASO arrhythmias and cardiac dysfunction should raise suspicion of coronary insufficiency; 7) coronary insufficiency and arrhythmias are rare but associated with sudden death; 8) early and late-onset ND abnormalities are common; 9) AR and aortic root dilation are well tolerated; and 10) the aging ASO patient may benefit from “exercise-prescription” rather than restriction.
Conclusions
Significant strides have been made in understanding risk factors for cardiac, ND and other important clinical outcomes after ASO.
INTRODUCTION
The arterial switch operation (ASO) has now replaced the atrial switch procedures developed by Mustard and Senning in the management of d-transposition of the great arteries (D-TGA) [1, 2]. Since Jatene performed the first successful ASO in 1975 [3], survival rates have improved with refinement of surgical techniques and improved medical management. Currently, most treated patients live to adulthood, with a 20-year survival of nearly 90%.
This paper aims to update clinicians on the management of patients with D-TGA and an intact (or virtually intact) ventricular septum who undergo the ASO, utilizing a “time-line” approach (Figure 1).
GENETICS
D-TGA accounts for 5 – 7% of all congenital heart defects (CHD) with a prevalence of 0.2 per 1000 live births and male preponderance [4–6]. Sibling recurrence rates of 0.27 and 2%, respectively, have been noted in simple and complex forms associated with a functional single ventricle or heterotaxy [6–8]. Among parents and siblings, mean recurrence risk for CHD is 1.4% [7, 8]. Causative mutations have been reported in the Nodal signaling pathway (Table 1) [9, 10].
TABLE 1
D-TGA Pathways and Genes.
Final Common Pathways TGA
|
TGA-Causing Genes
|
L-R = left – right asymmetry; TGA = Transposition of the Great Arteries.
Mutations in ZIC3, initially known to cause X-linked heterotaxy, have also been shown to cause sporadic and familial D-TGA [9–11]. Abnormalities in other Nodal pathway genes, such as CFC1 and FoxH1, have also been associated with isolated or syndromic D-TGA [9–11]. In addition, PROSIT240 (also termed THRAP2 or MED13L) mutations have been identified in patients with D-TGA with or without mental retardation [12]. Neural crest induction has long been considered a major target in the development of D-TGA and other conotruncal defects [13], supporting the concept that genetic variants or susceptibility alleles within one or more developmental pathways may dysregulate signaling in a synergistic fashion.
Clinical genetic testing is currently not widely available for D-TGA, with Comparative Genomic Hybridization (CGH) and Fluorescence in-situ hybridization (FISH) reserved for patients with multiple congenital anomalies and syndromes.
PREOPERATIVE CARE
Prenatal Diagnosis
Accurate prenatal diagnosis minimizes maternal and infant risk as well as improves outcomes. Relying solely on first and early second trimester transvaginal fetal echocardiography is not recommended. [14].
Hypermobility of the atrial septum and reverse diastolic patent ductus arteriosus (PDA) flow have been associated with a need for urgent BAS [15]. However, as prenatal prediction of the adequacy of the interatrial communication is imperfect, it is recommended that neonates with D-TGA be delivered in a center performing BAS. Children with D-TGA diagnosed prenatally have better early complex cognitive skills, particularly executive function, as compared with those diagnosed postnatally, in whom preoperative acidosis and profound hypoxemia are more common [16].
Timing and Location of Delivery
Once fetal diagnosis is made, a multi-disciplinary team approach helps improve outcomes and shorten time to intervention [17]. Barring obstetric complications, delivery at 39–40 weeks is preferred [18] as the last 6 weeks of gestation are a critical period of growth and development, particularly of the brain and lungs. Disturbance of the intrauterine milieu during the latter part of gestation may impact long-term neurodevelopmental (ND) and respiratory outcome [19]. In late gestation, the transposed fetal circulation results in lower substrate delivery to the rapidly developing brain, which may account for the higher than expected incidence of microcephaly, white matter injury and cortical immaturity seen on neonatal MRI scans. Current data suggests that ND outcome will be better if children can be delivered at term [20–23]. It is recommended that coordination of maternal transfer begins soon after the fetal diagnosis is made for institutions that do not perform the ASO. (24)
Pulse oximetry
Pulse oximetry was added to the Uniform Screening Panel for newborns in 2011, but it is still not universally utilized. [25]. Screening may result in timely diagnosis of newborns with D-TGA who may have eluded prenatal detection.
Imaging
Trans-thoracic echocardiography is used in D-TGA to confirm the prenatal diagnosis and obtain details of the anatomy. Malaligned commissures and a single coronary ostium have been associated with late coronary events and death after ASO [26].
Prostaglandin therapy
Most hypoxemic neonates with D-TGA will benefit solely from early institution of prostaglandin E1 (PGE). However, neonates with inadequate intracardiac mixing due to a restrictive foramen ovale will not improve on PGE alone. In this setting, the markedly increased pulmonary blood flow from the PDA may lead to deleterious left atrial hypertension, pulmonary congestion, and low cardiac output requiring urgent BAS.
With medical management, ~ 90% of neonates with D-TGA are relatively stable and undergo electively-timed ASO; however, preoperative management and the exact timing of surgery vary by institution. Clinicians are confronted with a decision-analysis of competing risks in which one must weigh the relative risks and benefits of: 1) BAS, 2) PGE and a resultant PDA, and 3) timing of neonatal surgery.
Risks of BAS
BAS may be associated with vascular trauma, atrial arrhythmias, atrial perforation and tamponade, with conflicting data regarding an increased risk of stroke. Some reports suggest that embolic brain injury is related to BAS [27, 28] although this has been refuted by others [29–31].
Risks of PGE
PGE can cause apnea, hypotension and fever (especially in lower birth weight neonates). There may be a false sense of security in the preoperative neonate on PGE who appears to have “adequate” oxygen saturations. While awaiting ASO, neonates remain at risk for mechanical ventilation, infection, medical errors, paradoxical emboli, increased cost and a longer hospital stay [32].
A reassuring peripheral oxygen saturation may be associated with paradoxically low cerebral oxygen delivery. Cerebral venous oxygen saturation is significantly lower than predicted from the arterial or mixed venous oxygen saturation in neonates with a run-off lesion [33, 34]. Mean cerebral oxygen saturations in children with a PDA and normal systemic oxygen saturations range in the low 50s and are likely lower in D-TGA [33]. Central nervous system injury, specifically to the white matter, has been associated with even a few days delay in ASO, particularly if accompanied by significant hypoxemia [30]. Earlier elimination of hypoxemia may contribute to improved motor outcomes and brain growth in certain subgroups [35].
Timing of surgery
Waiting several days after birth before an ASO has potential benefits: 1) transition from fetal to neonatal circulation; 2) reduction in pulmonary vascular resistance; 3) kidney and liver function improvement; 4) initiation of enteral nutrition; 5) evaluation for other congenital anomalies; and 6) family preparation for surgery. However, risks relating to BAS, prolonged exposure to PGE and PDA physiology, longer duration of hypoxemia, lower cerebral oxygen delivery and paradoxical emboli, may counteract the benefits of “elective” delay.
Earlier ASO may have a ND benefit [30] and may reduce hospital morbidity, complications and cost. One study suggests that 3 days of age is the ideal time for an ASO [24]. Others suggest the ASO can be safely performed within hours of birth using autologous umbilical cord blood to prime the bypass circuit [36, 37]. These recommendations require longer follow-up and multi-institutional validation, but suggest that delays in performing the ASO should be minimized. It is becoming increasingly common to schedule ASO within 2 to 4 days of diagnosis while the decision to perform a BAS, as well as when or whether to discontinue PGE after BAS, remains institution-specific.
Timing the ASO is particularly challenging in premature and low-birth weight neonates as the surgical risk and morbidity of BAS and PGE therapy are higher in smaller infants [38, 39]. Although not specifically investigated in D-TGA, among heterogeneous groups of CHD, the relationship of risk to weight is non-linear with an inflection point at ~ 2.0 to 2.2 kg [40].
SURGICAL CHALLENGES OF THE ASO
Simple in concept, the ASO remains one of the most complex neonatal operations. The great arteries are divided and a Lecompte maneuver is performed. (Figure 2A) The coronary arteries are transferred, the great arteries are reconnected to the proper ventricle and any intracardiac communication is closed (Figure 2B). Mortality risk factors include: smaller institutional and surgeon experience, smaller patient size, side-by-side great vessel arrangement, LV hypoplasia, LV outlet obstruction, and arch abnormalities, as well as intramural and single coronary artery [41–50]. Early mortality is almost always due to difficulty with coronary artery transfer resulting in myocardial ischemia [41, 51]. Table 2 summarizes surgical series focusing on survival and risk factors for mortality. Two recent studies demonstrate hospital survival of > 98% [24, 52]. Data from the United Kingdom’s National Institute for Cardiovascular Outcomes (NICOR) shows a nationwide 30-day mortality for the ASO at < 3% with a 1-year survival of > 96% [53].
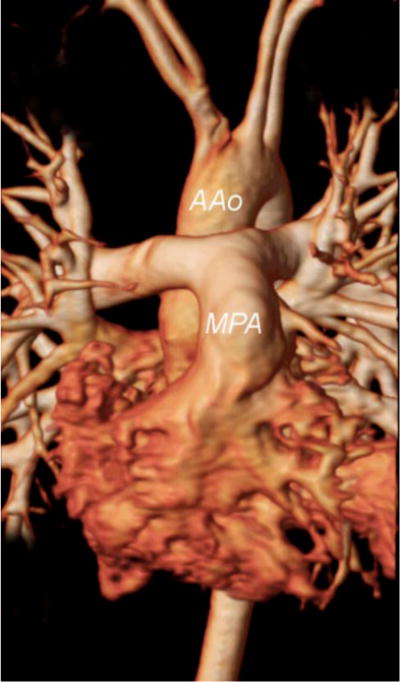
Magnetic resonance angiogram in a patient with D-TGA after the ASO with the Lecompte maneuver. Note the position of the neo-main pulmonary artery (MPA) and branches anterior to the ascending aorta (AAo)
AAo = ascending aorta; MPA = main pulmonary artery.
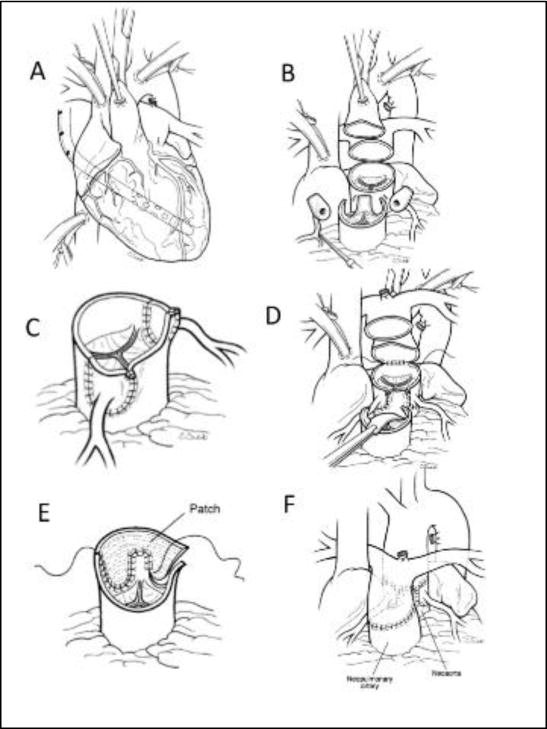
A) Typical anatomy and cannulation for surgery. The sites for implantation of the coronary arteries have been marked on the pulmonary root. B) The great vessels have been divided above the sinotubular junction and the coronary buttons excised and mobilized. C) The coronary buttons have been implanted into the pulmonary root with medially based trap-door incisions. D) The pulmonary artery bifurcation has been moved anterior to the aorta. The “Lecompte maneuver” and the anastomosis of the ascending aorta to the proximal neoaortic root has been initiated. E) The defect in the aorta created by excising the coronary buttons is being repaired with a single “pantaloon” patch creating the neo-pulmonary root. F) The pulmonary artery has been anastomosed to the neopulmonary root.
TABLE 2
Outcome and predictors of early mortality of the arterial switch operation for TGA with IVS publications during the last decade.
Author, year | Inclusive years | n | % IVS | Early Survival For TGA IVS % | 5 Year Survival% | 10 Year Survival% | Coronary anatomic risk factors | Other predictors of early mortality |
---|---|---|---|---|---|---|---|---|
Sarris, 2006* | 1998–2000 | 613 | 70 | 97 | NA | NA | Single coronary (univariate analysis only) | Open sternum |
Lalezari, 2011 | 1977–2007 | 332 | 60.8 | 88.6 | 85.8† | 85.2† | Not a risk factor for early mortality | Technical problems with coronary transfer |
Fricke, 2012 | 1983–2009 | 618 | 64 | 98.2 | 98 | 97 | Not a risk factor for early mortality | Weight < 2.5 kg ECMO |
Khairy, 2013 | 1983–1999 | 400 | 59.5 | 93.5† | NA | 92.7† | Single right coronary artery | Postoperative heart failure |
Cain, 2014 | 2000–2011 | 70 | 100 | 98.6 | NA | NA | None identified | No predictors of early mortality but earlier repair < 4 days of age was associated with decrease resource utilization |
Anderson, 2014 | 2003–2012 | 140 | 75 | 98.6 | NA | NA | None identified | No predictors of early mortality but earlier repair < 4 days of age was associated with decrease resource utilization |
ECMO = extracorporeal membrane oxygenation; IVS = intact ventricular septum; TGA = transposition of the great arteries with intact septum.
Transfer of the coronary artery origins is key to a successful ASO. After the aorta is cross-clamped and divided, the sinus aorta surrounding the coronary ostia (the so-called “coronary button”) is excised. The boundary of the button is 1–2 millimeters of sinus aorta surrounding the coronary ostium. If the ostium is adjacent to a commissure, the commissure should be taken down to ensure the button is large enough for coronary transfer. After button excision, the proximal coronary is mobilized to allow the vessel to be implanted into the pulmonary root. Several techniques for coronary artery implantation have been described with no clear advantage of one technique over another [54]. Assessment of coronary anatomy and adequate filling after transfer is essential. Depressed ventricular function or inability to be weaned from cardiopulmonary support may be considered coronary insufficiency until proven otherwise.
Elective delayed sternal closure
In two large series of the ASO, a quarter to one-third of patients had delayed sternal closure, which was associated with worse outcome [43, 45]. In a recent retrospective study, elective delayed sternal closure afforded no benefit [55]. Although delayed sternal closure is used commonly and may be necessary in patients who have had a prolonged and complicated operation, routine delayed sternal closure cannot be recommended.
Hemodynamic and neurological monitoring
Following an uncomplicated ASO, the postoperative course is typically uneventful with the opportunity to rapidly “de-intensify” the patient. Standard monitoring includes ECG, invasive arterial and central venous pressure, pulse oximetry and capnography. Transthoracic left atrial lines, while standard in the past [56], are not generally used unless there are concerns of potential LV dysfunction. Venous saturation monitoring or pulmonary artery catheters may be considered in select cases [56–58].
Near infrared spectroscopy (NIRS) is considered both a hemodynamic and neurological monitor, with increasing reports of the predictive validity of low cerebral NIRS values and later ND disabilities [58–60]. Some centers advocate continuous electroencephalography, transcranial Doppler, strict glycemic control and measurement of biomarkers such as S100β to identify infants at risk for ND abnormalities. These newer techniques cannot currently be recommended as standard therapy as results have been disparate [61–63]. A recent systematic review suggested that the only strategy that could be “recommended” was avoidance of extreme hemodilution during the ASO [64].
Timing of Extubation
In the past, postoperative care following the ASO frequently included deep sedation and neuromuscular blockade [57]. However, it is increasingly recognized that prolonged mechanical ventilation is associated with an increased risk of pneumonia, a longer hospital length of stay and increased cost [65]. In the absence of significant hemodynamic instability or bleeding, one recent meta-analysis of a heterogeneous patient group suggests that early extubation (< 6 hours after surgery) can be achieved safely with a more rapid pace of recovery and fewer complications [66].
Early post-operative evaluation
In most infants with early post-operative ischemia, global and/or regional ventricular dysfunction is seen by echocardiography [49, 67]. Unexplained profound ventricular dysfunction, low cardiac output syndrome or hemodynamically significant arrhythmias, including supraventricular tachycardia, junctional ectopic tachycardia or ventricular tachycardia, should raise suspicion of early coronary insufficiency [41, 46, 51, 68, 69]. Cardiac catheterization and angiography is the preferred method to evaluate coronary obstruction in the unstable neonate. Computed tomography (CT) post-ASO is limited by rapid neonatal heart rate and high radiation doses. Cardiac magnetic resonance (CMR) is limited by tachycardia and low spatial resolution.
THE “LATE” ASO
Although neonatal ASO is ideal, it demands early diagnosis and rapid access to a surgical center [24]. In much of the world these may not be available and one must choose between a late ASO, with or without some form of preparation of the LV, or an atrial switch. In the 1980s and early 1990s, preparation of the LV by banding the pulmonary artery was used as an alternative to a Senning or Mustard procedure, albeit with significant risk. Primary ASO may be used in patients older than 2 months of age with only slight increase in early morbidity and mortality, with 5.7% requiring postoperative mechanical assistance in one study [70]. Other studies show comparable outcomes of the late ASO in children up to 8 years of age, with only a mild increase in morbidity and resource consumption [71–74]. Those undergoing the two-stage ASO fared the worst. Long term data are limited, and there is a need for a multicenter study in order to define risk factors and the ability of the LV to meet expected demands [75]. A recent study from China reports that children with D-TGA older than 6 months of age at the time of ASO had quality-of-life scores indistinguishable from their normal peers [76].
TECHNICAL PERFORMANCE SCORE
The ASO is a technically challenging operation with many components that require perfection for optimal outcomes. The technical performance score (TPS) is a tool that assesses the adequacy of a repair as: Class 1 (optimal, no residua), Class 2 (adequate, minor residua) or Class 3 (inadequate, major residua or unplanned reintervention or placement of a permanent pacemaker prior to discharge), based on specific echocardiographic and clinical criteria [77]. TPS correlates with pre-discharge outcomes such as duration of ventilatory support, postoperative length of stay and complications (Figure 3), as well as post-discharge outcomes, such as unplanned reinterventions (Figure 4). Thus, TPS may help identify which patients are likely to require increased surveillance.
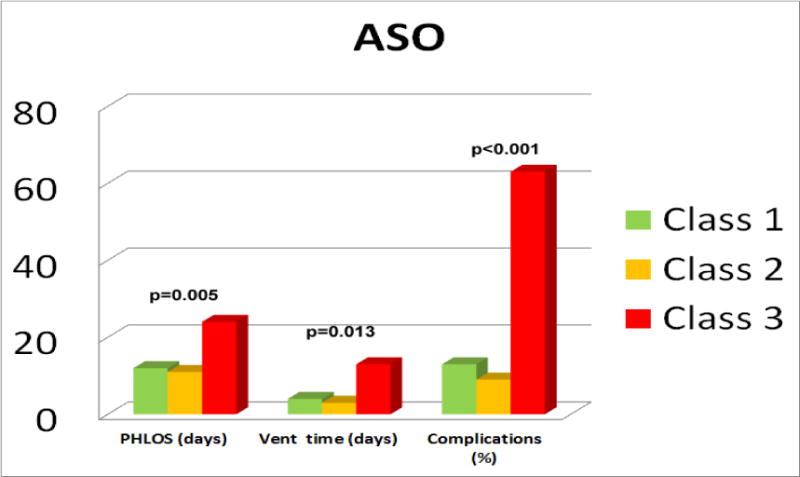
Postoperative hospital length of stay, ventilation time and complications across TPS classes in a 2011 multicenter cohort that includes both ASO and ASO/VSD. The X axis represents days for length of stay and ventilation time, and % for complications. Class 3 patients fared worse when compared to Class 1 after adjusting for age, prematurity and presence of chromosomal anomalies, as well as center and cardio-pulmonary bypass times.
Class 1 = No residua; Class 2 = minor residual defects; Class 3 = Major residual defects or unplanned reintervention in anatomic area of repair; PHLOS =Post-operative hospital length of stay; Vent = postoperative duration on ventilator.
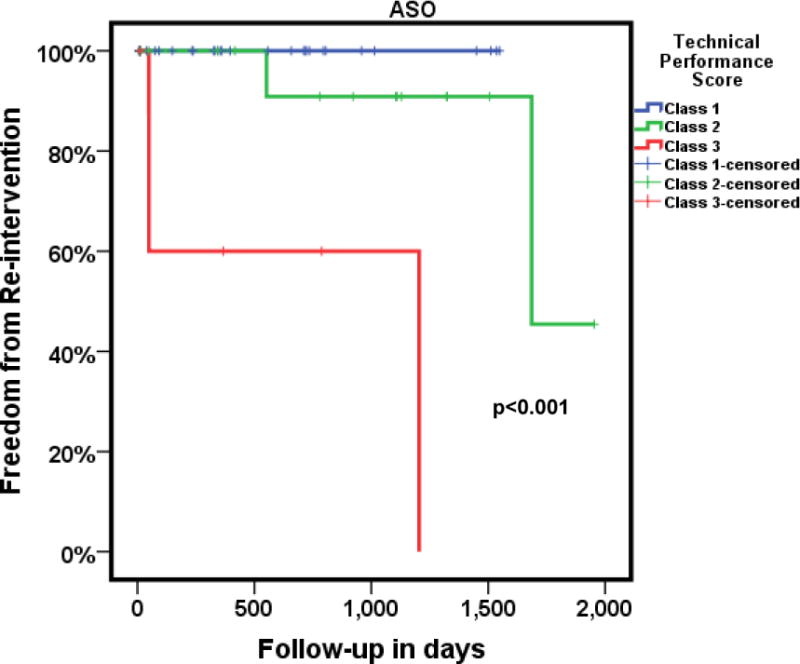
Kaplan-Meier estimate of freedom from reintervention in a cohort of 70 subjects following ASO for D-TGA/IVS followed over 4 years. Class 3 patients (bold red line) had significantly higher reinterventions when compared to Class 1 (bold blue line) patients. The small hatch marks in each of the lines represents the time up to which each patient had follow-up.
ASO = arterial switch operation; Class 1 = No residua; Class 2 = minor residual defects; Class 3 = Major residual defects or unplanned reinterventions in anatomic area of repair, D-TGA/IVS = d-transposition of great arteries with intact ventricular septum.
Pediatric Cardiac Care Consortium (PCCC)
The Pediatric Cardiac Care Consortium (PCCC), a registry founded to support quality improvement in cardiac surgery, has collected event outcome data from 45 small to mid-size centers in North America. The registry has a total of 1,835 patients with D-TGA who underwent ASO between 1982 and 2007. Since 1984, over 1700 neonatal ASOs have been reported with a mean patient age of 7 days at repair and an in-hospital and 30-day overall mortality of 7.8% and 6.6% respectively. The number of neonatal procedures and mortality by year is presented in Figure 4. Postoperative mortality dropped to 2.9% in the last 5 years. Overall mortality in children with a late ASO (median 49 days, range 29–232 days, n = 43) was 5.4%.
QUALITY METRICS
To address lack of consensus regarding appropriate follow-up for D-TGA after ASO, the American College of Cardiology Adult Congenital and Pediatric Cardiology Council used the RAND methodology [78] to develop quality measures for ambulatory care. Quality measures include: 1) postoperative echocardiography between 3 and 12 months of age; 2) neurodevelopmental assessment; 3) screening lipid profile by 11 years of age; and 4) documentation of transition plan to adult providers at 18 years of age.
Post-operative management for D-TGA after ASO was the first condition to be included in an innovative learning system known as Standardized Clinical Assessment and Management Plans (SCAMPs) to optimize care delivery [79]. Initial analyses using TGA/ASO SCAMP algorithms reduced projected clinic visits and echocardiograms compared to controls [80]. Subsequent iterations resulted in cost reductions by eliminating testing with low clinical benefit and limiting expensive tests such as CMR to high-risk patients.
LIFE-LONG FOLLOW-UP CARE
After the ASO, patients may have residual lesions and/or sequelae from interventions that require lifelong surveillance and care. Effective transition to ACHD care is essential [81, 82]. Recommended practices to help facilitate this transition have been published [83] but are not yet universally employed [84].
Survival into adulthood after ASO is common and the risk of reoperation is low [85]. However, ASO has life-long consequences, some of which may still be unrecognized, requiring ongoing medical surveillance. To date, neo-pulmonary stenosis, neo-aortic regurgitation, neo-aortic root dilation and coronary artery disease have been noted. Chronotropic incompetence, exercise intolerance and sudden cardiac death (SCD) are additional concerns. Life-long management of the ASO population poses several challenges: 1) absence of current consensus regarding the appropriate interval and modality for surveillance imaging; 2) there is no defined management strategy when subclinical anatomic or physiologic abnormalities are identified; 3) symptoms attributable to potential complications, especially coronary obstruction, are rare and therefore practitioners must be vigilant for classic and atypical presentations; and 4) the effects of acquired coronary artery disease superimposed on manipulated coronary arteries remain unknown. To date, almost 40 years after the initial ASO, we have identified a number of long-term consequences (Table 3).
TABLE 3
Postoperative Sequelae Following the Arterial Switch Operation.
Long Term Post-Operative Sequelae | Incidence |
---|---|
Supravalvular pulmonary stenosis * | ~ 10% |
Supravalvular aortic stenosis * | ~ 5% |
Neoaortic root dilation | Nearly universal |
Neoaortic regurgitation | Most (moderate or severe in < 10%) |
Asymptomatic coronary occlusion | 2–7% |
Sudden cardiac death | < 1% |
Arrhythmia | 2–10% |
Aortic dissection or rupture | Unknown |
Modified from Wernovsky et al. Congenit Heart Dis 2006;1:10–26 [219].
Myocardial Ischemia
Obstructed coronary arteries are present in 5–7% of survivors [41, 86–89] and remain the most common cause of morbidity and mortality following ASO. The incidence of myocardial ischemia, infarction and death is most prevalent in the first 3 months after ASO. When ostial stenosis is identified in childhood, annual electrocardiogram and echocardiography are recommended with advanced imaging if indicated.
Coronary obstruction late after the ASO is uncommon and more frequently anatomic than physiologic. In one long-term study, freedom from coronary events was 88.1 ± 6.4% at 22 years [80]. Selective coronary angiography has been considered the gold standard for anatomic evaluation of the coronary lumen [90]. Intimal thickening may be detected by intravascular ultrasound [91]. Modern ECG-gated CT angiography provides excellent spatial resolution to evaluate the coronary arteries and is considered by many the study of choice for morphologic assessment in young adults with ASO but is associated with exposure to radiation [92, 93]. CMR angiography has lower spatial resolution but can provide additional functional information (e.g., myocardial viability, aortic regurgitation) [94]. Detection of subclinical myocardial ischemia after the ASO remains a challenge. Clinical evaluation, electrocardiography, and echocardiography have a low sensitivity for detecting coronary insufficiency [87].
Exercise testing is the preferred modality for physiologic assessment, but pharmacologic stress with dobutamine or a coronary vasodilator (e.g., adenosine) may also be used. Stress echocardiography and CMR can detect regional wall motion abnormalities. Single-photon emission computed tomography (SPECT), positron emission tomography (PET) or first pass perfusion CMR may detect perfusion defects. Exercise-induced myocardial perfusion defects are present by SPECT in 5–10% of patients, while symptoms and ST segment depression are rare and of unclear significance [95–97]. SPECT should be reserved for high-risk patients including those with exercise-induced symptoms suggestive of ischemia, atypical coronary variants, intramural coronaries, those who had coronary button re-implantation, or when electrocardiographic markers of ischemia are unreliable.
Neo-aortic root dilation and aortic regurgitation
Neo-aortic valve regurgitation (AR) is a long-term consequence of the ASO. Freedom from AR of Grades IV, III and II at 23 years was 90.2 ± 6.6, 70.9 ± 9.6 and 20.3 ± 5.5%, respectively. Older age at time of ASO, presence of VSD, bicuspid pulmonic valve, previous PA banding, perioperative mild AR and higher neo-aortic root/ascending aorta ratio are risk factors for AR [98–100]. Neo-aortic root dilation is also a consequence of ASO [101, 102], especially if there is size discrepancy of the great arteries [103]. Fortunately, aortic root dilation has not been significantly progressive during the current duration of follow-up and has not been associated with acute aortic dissection or increased mortality [104].
RE-OPERATION LATE AFTER ASO
A single-center study reporting the outcomes of 400 subjects revealed that although 75% of patients with good functional status were free from intervention at 25 years of follow-up, between 3 and 10% developed moderate neo-aortic or neo-pulmonary regurgitation and stenosis [63]. Two to 8% of patients may require intervention, including balloon angioplasty, transcatheter stenting or surgical patch arterioplasty [99, 105, 106]. The ASO reoperation study revealed that pulmonary artery reconstruction was required earlier than neo-aortic intervention (5.4 ± 6.8 vs 13.8 ± 7.7 years, p < 0.001) [107]. Freedom from pulmonary stenosis (PS) of ≥ 36 and ≥ 20 mmHg at 23 years was 34.8 ± 18.0 and 17.7 ± 9.6%, respectively, but incidence may be steadily declining [106]. Coronary grafting is indicated in a very small group of patients [86, 99, 108].
LATE ARRHYTHMIAS AND CHRONOTROPIC IMPAIRMENT
Normal sinus node function is present in 96–99% of patients after the ASO [41]. Chronotropic impairment due to abnormal autonomic function from sympathetic denervation is a consistent finding post ASO [41, 50, 69, 109]. The incidence of late arrhythmia is 2.4 – 9.6% [41, 50, 69, 110, 111]. Most late postoperative arrhythmias occurring beyond 30 days are associated with residual hemodynamic lesions or coronary artery abnormalities, especially occlusion. Late postoperative supraventricular tachycardia, especially atrial flutter or fibrillation, is associated with RVOT obstruction and/or significant tricuspid regurgitation [49, 69, 110]. Late ventricular tachycardia may be scar-related or secondary to ischemia and is typically amenable to ablation therapy [111, 112].
Sudden Cardiac Death (SCD)
Most deaths occur 1–5 years after the ASO, although some occur later, possibly related to exercise-induced external compression of unusually distributed coronary arteries [41]. Studies have reported a 0.3–0.8% incidence of SCD thought to be related to primary arrhythmia, myocardial ischemia or infarction [41, 50, 85, 110, 111, 113]. Early SCD is often associated with technical difficulties during coronary transfer [87]. Ventricular fibrillation and late SCD are usually associated with myocardial ischemia or infarction from coronary obstruction or intimal proliferation. Whether these are associated with atypical or intramural coronary arteries are unclear [51, 69, 85, 114, 115]. High-risk patients with a history of atypical, intramural or problematic coronary re-implantation may require in depth screening prior to engaging in high level physical activity [96, 116, 117].
ANTICIPATORY CARE: LIFESTYLE CHOICES, CHOLESTEROL, HYPERTENSION AND EXERCISE
Neonatal coronary manipulation, potential endothelial stress and ongoing aortic root pathology make coronary risk factor management integral to life-long care [118]. Obesity, dyslipidemia, hypertension, inactivity and smoking are risk factors for the development of coronary artery disease [119]. Recent data suggests HMG CoA reductase inhibitors should be used electively in at-risk patients regardless of LDL number. A recent study characterized obese ASO teenagers as having higher blood pressure, higher LV mass index, lower brachial artery reactivity (suggestive of endothelial abnormality), higher triglyceride with lower high-density lipoprotein levels, and increased carotid intimal medial thickness as compared to normal weight individuals [119]. Unfortunately, the incidence of obesity after the ASO is high [120] making this a potential significant future morbidity. These individuals have limited aerobic capacity on exercise testing [121]. Additionally, atypical coronary anatomy, pulmonary artery stenosis and ventilatory inefficiency are associated with decreased aerobic capacity [119, 122, 123].
LV function may be preserved despite decreased aerobic capacity, implicating the afore mentioned non-cardiac etiologies, including physical deconditioning and obesity. In the presence of normal LV function, the aging ASO population may benefit from increased physical activity and conditioning (i.e.: exercise “prescription” rather than “restriction”). European recommendations support active lifestyles including recreational and competitive sports for most [124].
CENTRAL NERVOUS SYSTEM AND ND OUTCOMES
Much of what we know about ND outcomes in CHD has been learned from children with D-TGA [125–129]. Given the high frequency of ND abnormalities in this population [18, 130, 131], all D-TGA patients should have a ND evaluation, ideally in early childhood, in keeping with the recent recommendations of the American Heart Association and American Academy of Pediatrics’ Scientific Statement [132]. There is emerging evidence that therapies directed toward improving maternal well-being as well as early therapies for the infant may improve long-term outcomes [133–135].
Most reports document a correlation between perioperative events, such as significant hypoxemia, acidosis, prolonged cardiopulmonary bypass, low cardiac output, and adverse ND and behavioral testing, some of which are modifiable or preventable [136–138]. The landmark prospective “Boston Circulatory Arrest Study” reported follow-up in D-TGA survivors at 1, 4, 8 and 16 years of age [118, 139–143]. Throughout follow-up, the group as a whole under-performed in many aspects of their ND evaluations. Behavior, speech, and language delays were detected at 4 and 8 years with significant deficits in visual-spatial and visual-memory skills, as well as in components of executive functioning such as working memory, hypothesis generation, sustained attention, and higher-order language skills. The intelligence quotients for the cohort were close to normal. However, additional concerns became apparent: executive dysfunction and “theory of mind” abnormalities were prevalent, patients were 4 times more likely to be taking psychotropic medications compared to cardiovascular medications, and 65% were receiving behavioral therapies and/or additional help at school. One-third had brain abnormalities detected on MRI. These abnormalities have been confirmed in multiple centers worldwide [144–146].
Academic and behavioral challenges may translate to occupational challenges in adulthood where studies are still lacking. Despite these challenges, quality of life has been good thus far in adolescents [147, 148].
PREGNANCY
The specific risks for the ASO population depend on existence and type of long-term complications. Fortunately, AR, the most common consequence of the ASO, is well-tolerated in pregnancy. Significant aortic root dilation or coronary obstruction require multi-disciplinary pre-conception evaluation. Established risk factors include impaired LV systolic function, ventricular tachycardia and mechanical systemic aortic valve thrombosis [149]. Research involving registries are underway to better understand pregnancy risks and outcomes post-ASO [150].
Vertical Transmission of Transposition of D-TGA
Genetic counseling to elucidate vertical transmission in D-TGA patients should take place prior to pregnancy. Diglio et al. identified two families where a mother with CHD had one or more D-TGA children [6]. Interestingly, of the 370 families with D-TGA offspring, none of the parents had D-TGA. Fesslova et al. also demonstrated a very low vertical transmission rate [151]. Furthermore, disease discordance was typical as none of the offspring of D-TGA parents had the same disease.
INSURABILITY/EMPLOYABILITY
For many adults with CHD, physical and psychological factors may affect both insurability and employability. Cognitive intervention in early childhood may result in improved employment status in adulthood [152]. Patients with ASO who emerge from early adulthood with reasonable cognitive function have positive trajectories [152].
In addition to the Americans with Disabilities Act of 1990, the Affordable Care Act has further supported the care of adults with CHD in the US by guaranteeing fair coverage for pre-existing conditions, increasing the age young adults can stay on their parents’ plan, and eliminating lifetime dollar caps on covered services.
SUMMARY
The long-term outcomes of patients with D-TGA have improved dramatically in the current ASO era with advances in prenatal diagnosis, BAS, PGE, surgical technique and postoperative management.
Ten key statements regarding this topic are: 1) The familial recurrence risk is low; 2) Children diagnosed prenatally have improved cognitive skills when compared with those diagnosed postnatally. Delivery at term is advantageous; 3) Echocardiography helps to identify risk factors such as commissural misalignment, restrictive PFO, coronary abnormalities and acute LV dysfunction before and after ASO; 4) BAS and PGE carry risks and their routine use may be minimized by performing the ASO in the first few days of life; 5) Early ASO improves outcomes and reduces costs. Mortality is low despite atypical coronary anatomy but single or intramural coronary arteries remain risk factors; 6) Early post-ASO arrhythmias and cardiac dysfunction should raise suspicion of coronary insufficiency or obstructive lesions; 7) Arrhythmias and coronary obstruction are rare but associated with SCD; 8) Early and late-onset ND abnormalities are common. Perioperative events such as pre-term delivery, BAS, prolonged PGE therapy, hypoxemia, paradoxical embolism and acidosis are preventable incriminating factors; 9) AR and aortic root dilation are common but, to date, are well-tolerated during pregnancy and, to our knowledge, not associated with dissection; and 10) The aging ASO patient should benefit from “exercise-prescription” rather than restriction.
CONCLUSION AND FUTURE DIRECTIONS
In the current era, the previously grim natural course of the neonate with D-TGA has been replaced by a very low mortality. Most treated patients live to adulthood, with a 20-year survival rate of almost 90%. The ASO is a great success story. However, there remains a number of uncertainties. Comorbidities such as ND abnormalities, low aerobic capacity, obesity and coronary disease remain challenging. Transfer of the coronary arteries in the neonate may result in ischemia. Longer follow-up data are needed to understand the severity and frequency of late myocardial ischemia, the need for re-intervention, and whether lifestyle and dietary modifications in childhood may reduce late-onset risks. Increasing duration of follow-up may unmask a higher prevalence of neo-aortic dilation and AR. The life-long, as opposed to “long-term”, consequences of this landmark surgical procedure remain speculative and will require the tincture of time to test our current management.
Acknowledgments
We wish to acknowledge the secretarial help of Debbie Metz from Children’s Heart Specialists, PSC, as well as the administrative support of Stephanie Mitchell from the American College of Cardiology.
ABBREVIATIONS
ACHD | adult with congenital heart disease |
AR | neo-aortic regurgitation |
ASO | arterial switch operation |
BAS | balloon atrial septostomy |
CHD | congenital heart defects |
CMR | cardiac magnetic resonance |
CT | computed tomography |
D-TGA | d-ventricular loop transposition of the great arteries |
LV | left ventricle |
ND | neurodevelopmental |
NIRS | near infrared spectroscopy |
PFO | patent foramen ovale |
PDA | patent ductus arteriosus |
PET | positron emission tomography |
PGE | prostaglandin E1 |
RVOTO | right ventricular outflow tract obstruction |
SCD | sudden cardiac death |
SPECT | single-photon emission computed tomography |
TPS | technical performance score |
VSD | ventricular septal defect |
Footnotes
Juan Villafane has disclosed his relationship with Biomedical. James Tweddell has disclosed his relationship with CorMatrix. Tal Geva has disclosed his relationship with Medtronic. Meena Nathan has disclosed her grant support through the Thoracic Surgery Foundation for Research and Education. Lazaros Kochilas has disclosed his support by NIH/HNLBI (1R01 HL122392-01). Kathy Jenkins has disclosed her grant support through Johns Hopkins Medical Center, Medtronic and NuMed. Robert H. Beekman, III has disclosed his relationship with St. Jude Medical. The remaining authors have no relationships to disclose.
References
. [Abstract] [Google Scholar]
Thorac Cardiovasc Surg. 2010;139(2):349–53. [Abstract] [Google Scholar]
Citations & impact
Impact metrics
Article citations
Effects of Prostaglandin E1 and Balloon Atrial Septostomy on Cerebral Blood Flow and Oxygenation in Newborns Diagnosed with Transposition of the Great Arteries.
Biomedicines, 12(9):2018, 04 Sep 2024
Cited by: 0 articles | PMID: 39335532 | PMCID: PMC11428714
Integrated prenatal and postnatal management for neonates with transposition of the great arteries: thirteen-year experience at a single center.
Ital J Pediatr, 50(1):153, 22 Aug 2024
Cited by: 0 articles | PMID: 39175080 | PMCID: PMC11340064
Pathogenesis and Surgical Treatment of Dextro-Transposition of the Great Arteries (D-TGA): Part II.
J Clin Med, 13(16):4823, 15 Aug 2024
Cited by: 1 article | PMID: 39200964 | PMCID: PMC11355351
Review Free full text in Europe PMC
Live-Birth Incidence of Isolated D-Transposition of Great Arteries-The Shift in Trends Due to Early Diagnosis.
Diagnostics (Basel), 14(11):1185, 05 Jun 2024
Cited by: 0 articles | PMID: 38893711 | PMCID: PMC11171914
Pulmonary, aorta, and coronary arteries post-arterial switch in transposition of great arteries: intermediate-term surveillance utilizing conventional echocardiography and cardiac multislice computed tomography.
Ital J Pediatr, 50(1):122, 26 Jun 2024
Cited by: 0 articles | PMID: 38926831 | PMCID: PMC11210087
Go to all (103) article citations
Similar Articles
To arrive at the top five similar articles we use a word-weighted algorithm to compare words from the Title and Abstract of each citation.
Management of the Adult with Arterial Switch.
Methodist Debakey Cardiovasc J, 15(2):133-137, 01 Apr 2019
Cited by: 5 articles | PMID: 31384376 | PMCID: PMC6668747
Review Free full text in Europe PMC
Elective Non-Urgent Balloon-Atrial Septostomy in Infants with d-Transposition of the Great Arteries Does Not Eliminate the Need for PGE1 Therapy at the Time of Arterial Switch Operation.
Pediatr Cardiol, 42(3):597-605, 25 Jan 2021
Cited by: 4 articles | PMID: 33492430
Earlier arterial switch operation improves outcomes and reduces costs for neonates with transposition of the great arteries.
J Am Coll Cardiol, 63(5):481-487, 30 Oct 2013
Cited by: 48 articles | PMID: 24184243
Development of quality metrics for ambulatory pediatric cardiology: Transposition of the great arteries after arterial switch operation.
Congenit Heart Dis, 13(1):52-58, 02 Oct 2017
Cited by: 0 articles | PMID: 28971577
Review
Funding
Funders who supported this work.
Johns Hopkins Medical Center
Medtronic
NHLBI NIH HHS (1)
Grant ID: R01 HL122392