Abstract
Free full text

Diversity and Evolution of the Class A Chromosomal Beta-Lactamase Gene in Klebsiella pneumoniae
Abstract
We investigated the diversity of the chromosomal class A beta-lactamase gene in Klebsiella pneumoniae in order to study the evolution of the gene. A 789-bp portion was sequenced in a panel of 28 strains, representative of three phylogenetic groups, KpI, KpII, and KpIII, recently identified in K. pneumoniae and of different chromosomal beta-lactamase variants previously identified. Three groups of sequences were found, two of them corresponding to the families SHV (pI 7.6) and LEN (pI 7.1), respectively, and one, more heterogeneous, corresponding to a new family that we named OKP (for other K. pneumoniae beta-lactamase). Levels of susceptibility to ampicillin, cefuroxime, cefotaxime, ceftazidime, and aztreonam and inhibition by clavulanic acid were similar in the three groups. One new SHV variant, seven new LEN variants, and four OKP variants were identified. The OKP variants formed two subgroups based on nucleotide sequences, one with pIs of 7.8 and 8.1 and the other with pIs of 6.5 and 7.0. The nucleotide sequences of the housekeeping genes gyrA, coding for subunit A of gyrase, and mdh, coding for malate dehydrogenase, were also determined. Phylogenetic analysis of the three genes studied revealed parallel evolution, with the SHV, OKP, and LEN beta-lactamase families corresponding to the phylogenetic groups KpI, KpII, and KpIII, respectively. This correspondence was fully confirmed for 34 additional strains in PCR assays specific for the three beta-lactamase families. We estimated the time since divergence of the phylogenetic groups KpI and KpIII at between 6 and 28 million years, confirming the ancient presence of the beta-lactamase gene in the genome of K. pneumoniae.
Beta-lactam antibiotics are the most commonly prescribed antibacterial agents, and production of beta-lactamases is the most common and important resistance mechanism of pathogenic bacteria against these agents. The recent history of beta-lactams and beta-lactamases is archetypical of the successive waves of rapid emergence of broader-spectrum resistance in response to introductions of antimicrobials with broadened activities (10, 23, 34, 52). The introduction into clinical practice of the oxyimino-cephalosporins for treatment of serious infections due to gram-negative bacteria was soon followed by emergence of the so-called extended-spectrum beta-lactamases (ESBLs). These enzymes are mostly plasmid-encoded derivatives of TEM-1, TEM-2, and SHV-1 and confer resistance to third-generation cephalosporins, as well as to monobactams, in addition to broad-spectrum penicillins and narrow-spectrum cephalosporins (10, 34).
Most of the beta-lactamases described to date (1, 2, 12-14) are plasmid-encoded enzymes from clinical isolates. However, analysis of clinical strains only may not provide a thorough picture of the evolution of resistance, since many resistance mechanisms have evolved in response to the selective pressure of ancient antimicrobial compounds produced in natural ecosystems (4, 5, 35). Thus, the origins of a substantial fraction of the extant diversity of resistance genes predate the clinical use of antibiotics. Knowledge of the diversity and evolution of antimicrobial resistance genes in natural populations is important for an understanding of the origin of resistance and of the evolution of, e.g., beta-lactamase catalytic activity (6).
Evidence that the diversity of resistance genes is ancient was provided by early studies comparing beta-lactamases and penicillin-binding proteins (29) and, more recently, by phylogenetic analysis of beta-lactamase gene families in comparison with the phylogenies of the bacterial species harboring them (4, 5). Also, the class A beta-lactamases of the OXY group seem to have diversified in parallel with housekeeping genes in Klebsiella oxytoca (17, 18). Sequence surveys of beta-lactamase genes in various bacterial species have revealed the putative evolutionary origins of some beta-lactamase families (27, 47). The ancestor of the plasmid-encoded SHV-1, which itself is believed to be the origin of a substantial proportion of ESBLs found in clinical infections (52), was suggested to be an allelic variant of the chromosomal class A beta-lactamase gene of Klebsiella pneumoniae (15, 20). The SHV family of ESBLs now includes SHV-2 to SHV-51, which differ from SHV-1 at one or a few amino acid positions (http://www.lahey.org/studies/webt.htm). Most of these variants have been described as plasmid-encoded enzymes that are often found in Escherichia coli and Klebsiella spp., but also in other gram-negative bacteria, such as Pseudomonas aeruginosa.
Several different alleles of the species-specific beta-lactamase gene of K. pneumoniae were identified in 1997 by Hœggman et al. (20) when they looked at a 187-bp region of the gene in fecal-carriage isolates from Swedish neonates. The alleles clustered into two major groups, one including the SHV-1 plasmid gene (26, 38) and another including the previously described chromosomal LEN-1 gene (3, 26, 38). All variants of the chromosomal beta-lactamase of K. pneumoniae confer resistance to ampicillin, amoxicillin, carbenicillin, and ticarcillin, but not to extended-spectrum beta-lactams (3, 15, 20, 33, 34; G. S. Babini and D. M. Livermore, Letter, Antimicrob. Agents Chemother. 44:2230, 2000).
The presence of a chromosomal gene at high frequency in a bacterial species does not necessarily mean that the gene was anciently present in the particular species. Recent horizontal introduction of the gene followed by a rapid rise in frequency due to selective pressure is an alternative explanation. To determine whether beta-lactamase diversification occurred as a natural, long-term evolutionary process within K. pneumoniae, we investigated the nucleotide diversity and evolution of the chromosomal beta-lactamase gene in comparison with two housekeeping genes, gyrA, coding for subunit A of gyrase, and mdh, coding for malate dehydrogenase. The gyrA sequence had previously been used by Brisse and Verhoef as a reliable marker of the phylogenetic diversity of K. pneumoniae (11).
MATERIALS AND METHODS
Strains.
Twenty-eight of the K. pneumoniae strains used in this study are listed in Table Table1.1. Seven of the strains were representatives of the three previously described K. pneumoniae phylogenetic groups KpI, KpII, and KpIII (11). Twenty of the strains were previously shown to carry chromosomal beta-lactamase gene variants identical or related either to blaSHV-1 (encoding pI 7.6 beta-lactamase) or to blaLEN-1 (encoding pI 7.1 beta-lactamase) (20). The blood isolate SB30, from a patient in Germany, was added to the study because it showed a peculiar gyrA sequence among >200 K. pneumoniae strains analyzed for their gyrA gene (S. Brisse, unpublished data). The fecal isolate OR95:2, from a Swedish neonate, was included because it carried a chromosomal beta-lactamase gene that did not cluster with blaSHV-1- or blaLEN-1-like alleles (20). Two environmental K. pneumoniae strains from plants (SB95 and SB96; kindly provided by P. A. D. Grimont, Institut Pasteur, Paris, France) were also included.
TABLE 1.
K. pneumoniae strains studied and their characteristics
Strain name(s) | K. pneumoniae subspecies | Groupa,b | pIb,c | Source | Reference(s) | MIC (μg/ml)d
| bla allelee | BLA amino acid sequencef | Beta- lactamaseg | gyrA allelee | mdh allelee | GenBank accession no. | ||||
---|---|---|---|---|---|---|---|---|---|---|---|---|---|---|---|---|
AMP | CRX | CTX | CAZ | AZT | ||||||||||||
ATCC 13883T, SB132 | pneumoniae | KpI | 7.6 | Pneumonia, The Netherlands | 11, 20 | 64 | 4 | 0.064 | 0.250 | 0.125 | 1 | 1 | SHV-1 | 4 | 5 | AJ635407 |
ATCC 13884T | rhinoscleromatis | KpI | 7.6* | Nose, Indonesia | 11, 20 | 32 | 0.5 | 0.032 | 0.125 | 0.064 | 2 | 4 | New SHV variant | 3 | 10 | AJ635411 |
ATCC 11296T, NCTC 5050, SB176 | ozaenae | KpI | 7.6 | Nose, Indonesia | 11, 20 | 16 | 0.5 | 0.016 | 0.032 | 0.016 | 3 | 2 | SHV-11 | 4 | 5 | AJ635408 |
1976 E | pneumoniae | KpI* | 7.6 | Not known | 20 | >256 | 32 | 0.125 | 0.250 | 0.064 | 4 | 1 | SHV-1 | 4 | 9 | AJ635413 |
ES694:2 | pneumoniae | KpI* | 7.6 | Feces, Sweden | 20 | 128 | 8 | 0.250 | 0.500 | 0.125 | 5 | 1 | SHV-1 | 4 | 8 | AJ635412 |
HU653:4 | pneumoniae | KpI* | 7.6* | Feces, Sweden | 20 | 16 | 2 | 0.032 | 0.125 | 0.032 | 6 | 3 | SHV-33 | 4 | 5 | AJ635410 |
MA207:1 | pneumoniae | KpI* | 7.6* | Feces, Sweden | 20 | 16 | 2 | 0.032 | 0.125 | 0.032 | 6 | 3 | SHV-33 | 4 | 5 | AJ635409 |
UD580:1 | pneumoniae | KpI* | 7.6 | Feces, Sweden | 20 | 32 | 4 | 0.064 | 0.125 | 0.016 | 7 | 2 | SHV-11 | 4 | 5 | AJ635415 |
UD890:1 | pneumoniae | KpI* | 7.6 | Feces, Sweden | 20 | 16 | 8 | 0.125 | 0.064 | 0.064 | 8 | 2 | SHV-11 | 4 | 9 | AJ635414 |
UD1001:4 | pneumoniae | KpI* | 7.6 | Feces, Sweden | 20 | 32 | 2 | 0.032 | 0.125 | 0.032 | 7 | 2 | SHV-11 | 4 | 5 | AJ635416 |
SB18 | pneumoniae | KpII | 7.8* | Blood, France | 11 | 16 | 2 | 0.032 | 0.064 | 0.032 | 9 | 14 | New OKP variant | 8 | 3 | AJ635401 |
SB59 | pneumoniae | KpII | 8.1* | Blood, Spain | 11 | 64 | 2 | 0.064 | 0.125 | 0.064 | 10 | 15 | New OKP variant | 7 | 4 | AJ635404 |
LEN-1 | pneumoniae | KpIII* | 7.1 | Feces, Japan | 20 | 8 | 1 | 0.032 | 0.064 | 0.016 | 11 | 5 | LEN-1 | 1 | 1 | AJ635405 |
SB1 | pneumoniae | KpIII | 7.1* | Blood, Austria | 11 | 16 | 2 | 0.032 | 0.064 | 0.032 | 12 | 7 | New LEN variant | 1 | 1 | AJ635400 |
SB31 | pneumoniae | KpIII | 7.1* | Blood, Germany | 11 | 16 | 2 | 0.064 | 0.125 | 0.032 | 13 | 8 | New LEN variant | 2 | 1 | AJ635403 |
SB95 (Kleb PP) | pneumoniae | KpIII* | 7.1* | Plant, France | This study | 4 | 1 | 0.016 | 0.125 | 0.016 | 11 | 5 | LEN-1 | 2 | 1 | AJ635405 |
SB96 (Kleb 701) | pneumoniae | KpIII* | 7.1* | Plant, France | This study | 16 | 2 | 0.032 | 0.064 | 0.032 | 14 | 9 | New LEN variant | 2 | 1 | AJ635406 |
JO436:1 | pneumoniae | KpIII* | 7.1 | Feces, Sweden | 20 | 8 | 2 | 0.064 | 0.125 | 0.064 | 15 | 7 | New LEN variant | 2 | 1 | AJ635421 |
JO757:2 | pneumoniae | KpIII* | 7.1 | Feces, Sweden | 20 | 16 | 8 | 0.064 | 0.250 | 0.064 | 16 | 7 | New LEN variant | 2 | 1 | AJ635422 |
KK427:2 | pneumoniae | KpIII* | 7.1 | Feces, Sweden | 20 | 16 | 8 | 0.250 | 0.125 | 0.064 | 17 | 5 | LEN-1 | 2 | 11 | AJ635423 |
OR803:4 | pneumoniae | KpIII* | 7.1 | Feces, Sweden | 20 | 16 | 2 | 0.032 | 0.125 | 0.032 | 18 | 10 | New LEN variant | 2 | 1 | AJ635417 |
SO661:2 | pneumoniae | KpIII* | 7.1 | Feces, Sweden | 20 | 8 | 4 | 0.064 | 0.064 | 0.064 | 19 | 11 | New LEN variant | 1 | 1 | AJ635419 |
UD711:2 | pneumoniae | KpIII* | 7.1 | Feces, Sweden | 20 | 16 | 4 | 0.064 | 0.125 | 0.064 | 20 | 6 | LEN-2 | 2 | 6 | AJ635418 |
VA680:2 | pneumoniae | KpIII* | 7.1 | Feces, Sweden | 20 | 4 | 2 | 0.016 | 0.032 | 0.016 | 20 | 6 | LEN-2 | 2 | 6 | AJ635426 |
UD827:1 | pneumoniae | KpIII* | 7.1 | Feces, Sweden | 20 | 8 | 2 | 0.032 | 0.125 | 0.032 | 21 | 12 | New LEN variant | 2 | 1 | AJ635424 |
UD892:1 | pneumoniae | KpIII* | 7.1 | Feces, Sweden | 20 | 8 | 2 | 0.064 | 0.125 | 0.064 | 22 | 13 | New LEN variant | 2 | 1 | AJ635425 |
SB30 | pneumoniae | KpII* | 7.0* | Blood, Germany | This study | 32 | 4 | 0.064 | 0.250 | 0.125 | 23 | 16 | New OKP variant | 6 | 2 | AJ635402 |
OR95:2 | pneumoniae | KpII* | 6.5* | Feces, Sweden | 20 | 32 | 4 | 0.064 | 0.125 | 0.125 | 24 | 17 | New OKP variant | 5 | 7 | AJ635420 |
An additional 34 clinical isolates, previously assigned to any of the three phylogenetic groups based on their gyrA genes (11), were included in order to study, by PCR, the correspondence between the phylogenetic groups and the chromosomal beta-lactamase gene variants. Twenty-one of the isolates belonged to group KpI, six belonged to KpII, and seven belonged to KpIII.
Beta-lactamase analysis and antibiotic susceptibility testing.
Analytical isoelectric focusing of crude cell-free sonicates was performed by the method of Matthew and Harris (36) on polyacrylamide gels (Ampholine PAGplate [pH 3.5 to 9.5]; Amersham Biosciences) in a Multiphor II system (Amersham Biosciences). The beta-lactamase bands were visualized by staining them with nitrocefin (Oxoid). The MICs of ampicillin, cefuroxime, cefotaxime, ceftazidime, and aztreonam were determined by Etest (AB Biodisk, Stockholm, Sweden) according to the manufacturer's instructions. MICs were also determined in the presence of clavulanic acid (2 μg/ml; SmithKline Beecham Pharmaceuticals). Transfer of ampicillin resistance was tested by conjugation experiments as described previously (20).
Nucleotide sequence determination.
The sequence of a 789-bp portion of the 861-bp chromosomal beta-lactamase gene of K. pneumoniae was determined after PCR amplification performed using the primers SHVseq1 (5′-ATG CGT TAT ATT CGC CTG TG) (positions 1 to 20 of the SHV-1 coding sequence; GenBank M59181) and SHVseq3a (5′-TTA GCG TTG CCA GTG CTC) (positions 861 to 844; GenBank M59181). The primers used for sequence determination included the two PCR primers and the internal primers SHVseq2 (5′-CCG CAG GAT TGA CTG CC) (positions 422 to 438) and SHVseq4 (5′-AGG CGG GTG ACG TTG TC) (positions 473 to 457). The sequence of a 383-bp portion of gyrA, starting at position 163 (codon 55) of the gene, was determined as previously described (11). The sequence of a 342-bp portion (codons 31 to 144) of the 939-bp malate dehydrogenase (mdh) gene was determined using PCR and the sequencing primers Pmdh-4 (5′-CCT TTC AGT TCC GCA ACA AAG) and Pmdh-5 (5′-AAC TGC CTT CAG GTT CAG AAC). These primers corresponded to fully conserved regions on an alignment of mdh genes from E. coli (GenBank accession no. M24777) (53) and K. oxytoca strain SB77 (Brisse, unpublished). Sequence determination of PCR products was carried out with the Big Dye Terminator Cycle Sequencing Ready Reaction kit (Perkin-Elmer) on an ABI 3700 or 3100 automated capillary DNA sequencer.
Specific PCR amplification of beta-lactamase gene families.
PCR primer pairs were designed to specifically amplify beta-lactamase genes of the SHV and LEN families and of a new third family named OKP (see Results): SHVfor (5′-GAG CGA AAG ATC CAC TAT CG) (positions 262 to 281) and SHVrev (5′-GGT ATC CCG CAG ATA AAT CA) (positions 786 to 767), LEN.for (5′-AAC TAC TGA CCG CGC AGC AT) (positions 563 to 582) and LEN.rev (5′-ATT ACG CTC GGC CAT ACT CG) (positions 810 to 791), and OKP-1F (5′-GCG GGC CGS GTG GGC TAT GTT) (positions 109 to 129) and OKP-2R (5′-MGC GGG GCC GCC RAC GCT CTT) (positions 426 to 406). PCR mixture conditions were as described previously (11). PCR amplification was achieved by submitting samples to an initial denaturation step (1 min at 94°C), followed by 35 amplification cycles (94°C for 1 min; 30 s at the annealing temperature of 60°C for SHV primers, 65°C for OKP primers, or 52°C for LEN primers; 72°C for 90 s) and a final elongation step of 5 min at 72°C. The seven phylogenetic-group reference strains were used as controls.
Sequence data analysis.
To determine the model of nucleotide substitution that best fit the data for each gene, the program Modeltest version 3.06 (49) was used to compare the maximum-likelihood scores of 56 different models computed using PAUP* version 4.0b10 (51), as described in the instructions for the Modeltest program. Phylogenetic trees were obtained using PAUP* version 4.0b10 and the neighbor-joining method, with distance options corresponding to the best model found for each gene. These models were HKY+G (21) for the beta-lactamase gene, the Jukes-Cantor (28) model for gyrA, and the Kimura two-parameter K80 model (31) for the mdh gene. Bootstrap analysis was performed with 1,000 replicates. Trees generated by PAUP* were saved and drawn using TreeView version 1.6.6 (44). The McDonald-Kreitman test of neutral evolution (37) was performed using DNASP version 3.53 (49a). Levels of synonymous substitution per synonymous site (Ks) were estimated using DNASP version 3.53, which uses the Nei and Gojobori method (39) after Jukes-Cantor correction for multiple substitutions. Computation of the Ks value for the divergence between E. coli and Salmonella enterica was performed by comparing pairwise values for one representative sequence of each E. coli phylogenetic group and all subspecies of S. enterica (9).
Nucleotide sequence accession numbers.
The beta-lactamase gene sequences and the gyrA and mdh sequences have been submitted to the GenBank-EMBL databases under accession numbers AJ635372 to AJ635426 (Table (Table11).
RESULTS
Isoelectric focusing analysis and antibiotic susceptibility testing.
Analytical isoelectric focusing revealed a single beta-lactamase band in all 28 strains, most of which were pI 7.6 or 7.1, i.e., SHV-1- or LEN-1-like, respectively (Table (Table1).1). The MICs of ampicillin, cefuroxime, cefotaxime, ceftazidime, and aztreonam showed the broad-spectrum penicillinase activity typical of class A beta-lactamases in all strains (Table (Table1).1). The ampicillin MICs were lowered by clavulanic acid (data not shown). No ESBL activity was detected. All attempts to transfer ampicillin resistance from the study strains to E. coli by conjugation failed, whereas plasmid-bearing strains used as positive controls for the transfer successfully donated their plasmids to the recipient strain.
Molecular phylogeny of the chromosomal beta-lactamase gene.
The nucleotide sequence of a 789-bp portion of the chromosomal beta-lactamase gene was established in the 28 strains. The sequence corresponds to 92% of the gene length and starts at position 37 of blaSHV-1 (codon 13) and ends 33 nucleotides before the stop codon. All sequences were aligned without insertion-deletion events, and there were no missing or ambiguous data. The sample contained 24 distinct bla alleles (Table (Table1;1; see GenBank accession numbers for nucleotide substitutions). Alignment of the 24 nucleotide sequences showed 156 polymorphic sites, of which 14 were present in only one sequence and 142 were present in at least two sequences and thus phylogenetically informative.
The phylogenetic analysis revealed three groups of nucleotide sequences (Fig. (Fig.1).1). The first group included the three reference strains of the phylogenetic group KpI (ATCC 13883T, ATCC 13884T, and ATCC 11296T/NCTC 5050T); the SHV-1 reference strain, 1976E; and all six fecal isolates expressing SHV-1-like beta-lactamases. The second group included the two reference strains of group KpIII, the LEN-1 reference strain, the two environmental isolates, and the nine fecal isolates expressing LEN-1-like beta-lactamases. The third group comprised the two KpII reference strains and strains SB30 and OR95:2. The nucleotide sequence divergence was much lower within all three Kp groups than between any pair of them (Table (Table2),2), as illustrated by the long branches leading to the ancestral node of each group compared to the short branches inside the groups (Fig. (Fig.1).1). Of the three groups of bla sequences, the third was the most heterogeneous and showed two distinct subgroups, one comprising the two KpII reference strains SB18 and SB59 and one comprising strains SB30 and OR95:2 (Fig. (Fig.11).
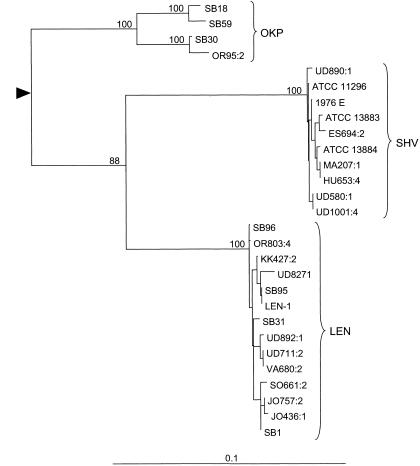
Phylogeny of the chromosomal beta-lactamase gene of K. pneumoniae. The tree was obtained by the neighbor-joining method using the HKY85+G model of nucleotide substitution (see Materials and Methods). The root was determined using the sequence of beta-lactamase TEM-1, the closest known relative to the chromosomal beta-lactamase of K. pneumoniae. Because of the long branch leading to the TEM-1 sequence, the root position is represented by a triangle in order to increase the scale of the figure so that the relationships among K. pneumoniae beta-lactamase sequences are clearly visible. The scale indicates the proportion of dissimilar nucleotides among sequences after correction for multiple substitutions. Three major groups of sequences are visible, corresponding to the SHV, LEN, and OKP families of beta-lactamase genes. The values at the nodes correspond to the percent confidence obtained after 1,000 bootstrap replicates. Bootstrap values inside the two groups corresponding to the SHV and LEN families were well below 50% for most nodes and are not given.
TABLE 2.
Sequence divergence for K. pneumoniae genes within and between the phylogenetic groups KpI, -II, and -III and between the two KpII subgroups
Comparison | Divergencea
| |||||
---|---|---|---|---|---|---|
bla
| gyrA
| mdh
| ||||
Mean within groupsb | Mean between groupsc | Mean within groupsb | Mean between groupsc | Mean within groupsb | Mean between groupsc | |
KpI vs KpII | 0.53 ± 0.05; 3.46 ± 0.91 | 12.30 ± 3.04 | 0.26 ± 0.13; 0.61 ± 0.16 | 1.24 ± 0.51 | 0.78 ± 0.23; 1.27 ± 0.35 | 3.36 ± 1.08 |
KpI vs KpIII | 0.53 ± 0.05; 0.60 ± 0.07 | 9.70 ± 1.42 | 0.26 ± 0.13; 0.26 ± 0.13 | 1.31 ± 0.68 | 0.78 ± 0.23; 0.39 ± 0.13 | 4.73 ± 1.69 |
KpII vs KpIII | 3.46 ± 0.91; 0.60 ± 0.07 | 11.38 ± 2.30 | 0.61 ± 0.16; 0.26 ± 0.13 | 2.16 ± 0.88 | 1.27 ± 0.35; 0.39 ± 0.13 | 3.12 ± 1.11 |
SB18 + SB59 vs SB30 + OR952 | 1.14 ± 0.57; 0.87 ± 0.44 | 4.69 ± 2.43 | 0.26 ± 0.13; 0.26 ± 0.13 | 0.78 ± 0.43 | 0.29 ± 0.15; 0.29 ± 0.15 | 1.75 ± 0.91 |
There was excellent correspondence between the sequences of the chromosomal beta-lactamase genes and the pIs of the encoded enzymes (Table (Table1).1). Indeed, the first group of sequences were all from strains producing pI 7.6 (SHV-1-like) beta-lactamases, and the second group included all sequences from pI 7.1 (LEN-1-like) strains. Also, the divergence seen within the third group of sequences corresponded to the pI values. The four strains in this group had beta-lactamases with different pIs (SB18, pI 7.8; SB59, pI 8.1; SB30, pI 7.0; and OR95:2, pI 6.5). None of the sequences in the third group could be closely matched to sequences in public databases. The closest matches corresponded to blaSHV (88% similarity to blaSHV-1) and blaLEN (89% similarity to blaLEN-1). Therefore, we chose to assign them to a new beta-lactamase gene family, which we designated blaOKP (for other K. pneumoniae beta-lactamases).
Correspondence between the phylogenies of the chromosomal beta-lactamase gene and gyrA.
The sequence of a 383-bp portion of the gyrA gene was established for 21 strains under study and compared to the gyrA sequences obtained previously for the seven reference strains of the K. pneumoniae phylogenetic groups KpI, KpII, and KpIII (11). All sequences were aligned without insertion or deletion, and no site had ambiguous or missing data. Eight distinct alleles were found among the 28 sequences (Table (Table1;1; see GenBank accession numbers for nucleotide substitutions). Eleven sites were polymorphic, two of which were singletons and nine of which were phylogenetically informative. Ten changes occurred in the third codon position, and one occurred in the first position. No change was observed at the amino acid level. The biggest pairwise difference was seen between alleles 1 and 7, with 3% (10 of 383) nucleotide changes.
The phylogenetic relationships among the gyrA sequences, depicted in Fig. Fig.2,2, corresponded fully to the phylogeny obtained for the chromosomal beta-lactamase gene seen in Fig. Fig.1.1. Indeed, all strains with blaSHV sequences fell into group KpI, all strains with blaLEN sequences fell into KpIII, and strains SB30 and OR95:2 formed a third branch, together with the KpII reference strains SB18 and SB59. Similar to the result observed based on their chromosomal beta-lactamase gene, strains SB30 and OR95:2 clustered in a distinct subgroup, different from the subgroup formed by the KpII reference strains. The nucleotide sequence divergence of gyrA within and between the groups KpI, -II, and -III is given in Table Table22.
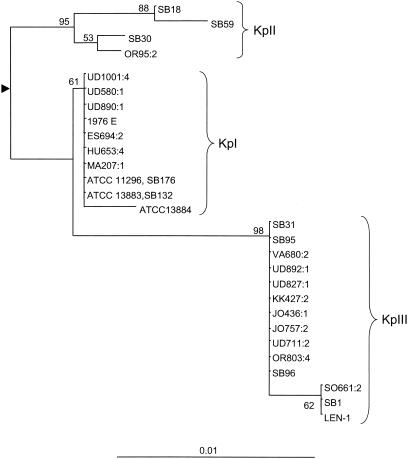
Phylogeny of the gyrA gene of K. pneumoniae. The tree was obtained by the neighbor-joining method using the Jukes-Cantor model of nucleotide substitution (see Materials and Methods). The tree was rooted at the midpoint of the branch leading from group KpII to the other two groups. The scale indicates the proportion of dissimilar nucleotides among sequences after correction for multiple substitutions. Three major groups of sequences are visible, corresponding to the K. pneumoniae phylogenetic groups KpI, KpII, and KpIII defined previously (11).
The correspondence between the chromosomal beta-lactamase gene sequences and the phylogenetic groups based on gyrA sequences was verified when 34 additional clinical strains, previously assigned to different phylogenetic groups, were analyzed by PCR (data not shown). The blaSHV gene was detected only in the 21 KpI strains tested, and the blaLEN gene was detected only in the seven KpIII strains tested. The PCR assays performed with primers OKP-1F and OKP-2R were positive only in the six KpII strains tested. The gyrA sequences of these strains showed that they were more closely related to KpII reference strains than they were to strains SB30 and OR95:2.
Diversity of chromosomally encoded beta-lactamase.
Among the 24 distinct nucleotide sequences obtained from the 28 strains, there were 54 codon differences that implied amino acid changes. In total, 17 distinct amino acid sequences were found (Tables (Tables11 and and3).3). Among the 10 KpI strains (all producing SHV beta-lactamases), one of the amino acid changes observed occurred at a site (residue 252) where variation had not been described previously (http://www.lahey.org/studies/webt.htm). The strain carrying this blaSHV allele, K. pneumoniae subsp. rhinoscleromatis ATCC13884T, thus produces an SHV variant with a leucine at position 252 instead of the proline found in previously described SHV enzymes. The other KpI strains showed deduced amino acid sequences identical to that of SHV-1, SHV-11 (the Leu35Gln variant, which was described as non-ESBL) (41), or SHV-33 (the Pro226Ser variant, which does not confer resistance to broad-spectrum cephalosporins) (15).
TABLE 3.
Polymorphic amino acid sites found in the 17 distinct beta-lactamases from this study
Amino acid sequence no.a | Beta-lactamase | Amino acid at position numberb
| |||||||||||||||||||||||||||||||||||||||||||||||||||||
---|---|---|---|---|---|---|---|---|---|---|---|---|---|---|---|---|---|---|---|---|---|---|---|---|---|---|---|---|---|---|---|---|---|---|---|---|---|---|---|---|---|---|---|---|---|---|---|---|---|---|---|---|---|---|---|
1 | 1 | 1 | 1 | 1 | 1 | 1 | 1 | 1 | 1 | 1 | 1 | 1 | 1 | 2 | 2 | 2 | 2 | 2 | 2 | 2 | 2 | 2 | 2 | 2 | 2 | 2 | 2 | 2 | 2 | ||||||||||||||||||||||||||
1 | 1 | 2 | 2 | 2 | 2 | 3 | 3 | 3 | 3 | 4 | 4 | 4 | 5 | 5 | 5 | 6 | 6 | 6 | 7 | 8 | 9 | 9 | 9 | 1 | 1 | 2 | 3 | 4 | 4 | 5 | 6 | 6 | 7 | 8 | 9 | 9 | 9 | 0 | 1 | 2 | 2 | 2 | 3 | 4 | 5 | 5 | 5 | 5 | 6 | 6 | 7 | 7 | 8 | ||
7 | 8 | 2 | 4 | 6 | 8 | 3 | 4 | 5 | 8 | 1 | 6 | 7 | 2 | 6 | 8 | 3 | 8 | 9 | 5 | 8 | 2 | 4 | 6 | 4 | 9 | 9 | 5 | 0 | 1 | 0 | 1 | 8 | 7 | 8 | 6 | 7 | 8 | 5 | 5 | 3 | 6 | 7 | 3 | 3 | 2 | 3 | 4 | 6 | 2 | 8 | 0 | 7 | 0 | ||
1 | SHV-1 | A | T | A | H | S | Q | I | K | L | S | S | M | I | A | T | T | D | M | M | V | D | E | K | H | A | V | M | A | A | T | A | R | E | A | A | S | Q | R | R | R | S | P | A | D | A | P | N | N | A | I | P | S | Q | G |
2 | SHV-11 | . | . | . | . | . | . | . | . | Q | . | . | . | . | . | . | . | . | . | . | . | . | . | . | . | . | . | . | . | . | . | . | . | . | . | . | . | . | . | . | . | . | . | . | . | . | . | . | . | . | . | . | . | . | . |
3 | SHV-33 | . | . | . | . | . | . | . | . | . | . | . | . | . | . | . | . | . | . | . | . | . | . | . | . | . | . | . | . | . | . | . | . | . | . | . | . | . | . | . | . | . | S | . | . | . | . | . | . | . | . | . | . | . | . |
4 | New SHV variant | . | . | . | . | . | . | . | . | . | . | . | . | . | . | . | . | . | . | . | . | . | . | . | . | . | . | . | . | . | . | . | . | . | . | . | . | . | . | . | . | . | . | . | . | . | L | . | . | . | . | . | . | . | . |
5 | LEN-1 | . | . | V | Y | G | . | . | . | Q | . | . | . | V | . | . | A | . | . | V | L | L | D | R | . | V | I | L | G | . | . | . | . | A | . | . | A | . | H | Q | . | A | . | P | . | . | . | D | G | P | . | . | . | H | . |
6 | LEN-2 | . | . | . | D | G | . | . | . | Q | . | . | . | V | . | . | A | . | . | V | L | V | D | R | . | V | I | L | G | . | . | . | . | A | . | . | A | . | H | Q | . | A | . | P | . | . | . | D | G | P | . | . | . | H | . |
7 | New LEN variant 1 | . | . | . | Y | G | . | . | . | Q | . | . | . | V | . | . | A | . | . | V | L | L | D | R | . | T | I | L | G | . | . | . | . | A | . | . | A | . | H | Q | . | A | . | P | . | . | . | D | G | P | . | . | . | H | . |
8 | New LEN variant 2 | . | . | . | D | G | . | . | . | Q | . | . | . | V | . | . | A | . | . | V | L | L | D | R | . | V | I | L | G | . | . | . | . | A | . | . | A | . | H | Q | . | A | . | P | . | . | . | D | G | P | L | . | . | H | . |
9 | New LEN variant 3 | . | . | . | Y | G | . | . | . | Q | . | . | . | V | . | . | A | . | . | V | L | L | D | R | . | V | I | L | G | . | . | . | . | A | . | . | A | . | H | Q | . | A | . | . | . | . | . | D | G | P | . | . | . | H | . |
10 | New LEN variant 4 | . | . | . | D | G | . | . | . | Q | . | . | . | V | . | . | A | . | . | V | L | L | D | R | . | V | I | L | G | . | . | . | . | A | . | . | A | . | H | Q | . | A | . | . | . | . | . | D | G | P | . | . | . | H | . |
11 | New LEN variant 5 | . | . | . | D | G | . | . | . | Q | . | . | . | V | . | . | A | . | . | V | L | L | D | R | . | T | I | L | G | . | . | . | . | A | . | . | A | . | H | Q | . | A | . | P | . | . | . | D | G | P | L | . | . | H | . |
12 | New LEN variant 6 | . | . | V | Y | G | . | . | . | Q | . | P | . | V | . | . | A | . | . | V | L | L | D | R | . | V | I | L | G | . | . | . | H | A | . | . | A | . | H | Q | . | A | . | P | . | . | . | D | G | P | . | . | . | H | . |
13 | New LEN variant 7 | . | . | . | D | G | . | . | . | Q | . | . | . | V | . | . | A | . | . | V | L | V | D | R | . | T | I | L | G | . | . | . | . | A | . | . | A | . | H | Q | . | A | . | P | . | . | . | D | G | P | . | . | . | H | . |
14 | New OKP variant 1 | . | A | . | F | . | P | . | T | R | . | A | Y | V | V | . | A | N | L | . | L | . | D | R | R | . | . | . | G | K | S | . | . | . | V | . | . | H | T | Q | Q | A | . | . | E | S | . | . | G | . | . | . | . | . | R |
15 | New OKP variant 2 | . | A | . | F | . | . | V | T | R | . | A | Y | V | . | . | A | S | L | . | L | . | D | R | R | . | . | . | G | K | S | . | . | . | V | . | . | H | T | Q | Q | A | . | . | . | S | . | . | G | . | . | . | T | . | R |
16 | New OKP variant 3 | . | A | . | F | . | . | . | . | I | G | A | Y | V | . | M | A | S | L | . | L | . | D | R | . | . | . | . | G | K | S | . | . | . | V | T | T | P | S | Q | . | A | . | . | . | S | . | D | G | . | . | A | T | . | . |
17 | New OKP variant 4 | T | A | . | F | . | . | . | . | I | G | A | Y | V | . | M | A | S | L | . | L | . | D | R | . | . | . | . | G | K | S | T | . | . | V | T | T | P | S | Q | Q | A | . | . | . | S | . | D | G | . | . | A | T | . | . |
Among the 14 KpIII strains (all producing LEN beta-lactamases), one plant isolate and one fecal isolate had amino acid sequences identical to that of the LEN-1 enzyme (3). Two other fecal isolates had beta-lactamase amino acid sequences that were indistinguishable from that of the LEN-2 enzyme (15). The remaining nine KpIII strains showed seven distinct amino acid sequences differing from LEN-1 by two to four amino acids (Table (Table3).3). These new variants are also different from LEN-2 and a recently described variant LEN enzyme (GenBank AF452105). They thus represent seven new LEN variants.
The deduced amino acid sequences of the OKP beta-lactamases of the KpII reference strains SB18 and SB59 differed by six residues (Table (Table3).3). The other two OKP beta lactamases, found in strains SB30 and OR95:2, differed by 3 amino acids, and they differed from the beta-lactamase sequence of strain SB18 by 18 and 19 amino acids, respectively (Table (Table3).3). Along the 263-codon portion that was sequenced, 28 to 32 amino acid differences (87.8 to 89% similarity) were observed among the four OKP beta-lactamases and SHV-1, and 27 to 33 amino acid differences were observed between the OKP beta-lactamases and LEN-1 (87.5 to 89.7% similarity).
The deduced amino acid sequences of all beta-lactamase variants identified in this study were conserved at all positions considered to be important for enzymatic activity (25, 32). The Asp-104 residue, suspected to be responsible for the very slight differences in the substrate profiles of SHV-1 and TEM-1 (32), was conserved in all enzyme variants. Changes associated with clinically relevant phenotypic resistance, in particular the change Gly238Ser associated with the majority of ESBL SHV enzymes, were not observed.
To test whether the evolution of the chromosomal beta-lactamase gene has been subjected to natural selection, we performed the McDonald-Kreitman test of neutral evolution (37). This test compares the synonymous and nonsynonymous variation between and within groups. Under neutrality, the ratio of synonymous to nonsynonymous substitutions that are fixed between groups should be the same as the ratio of synonymous to nonsynonymous polymorphisms within groups. The test, performed for the pairwise comparison of the three K. pneumoniae groups KpI, -II, and -III, did not indicate any evidence of selection on the beta-lactamase gene (Table (Table44).
TABLE 4.
Results of McDonald-Kreitman tests of neutral evolution of the chromosomal beta-lactamase gene of K. pneumoniae
Group 1 | Group 2 | No. of fixed differences between group 1 and group 2
| No. of polymorphisms observed within groups 1 and 2
| Ga | Pb | ||
---|---|---|---|---|---|---|---|
Synonymous | Nonsynonymous | Synonymous | Nonsynonymous | ||||
KpI | KpII | 48 | 27 | 30 | 27 | 1.71 | 0.19 |
KpI | KpIII | 42 | 23 | 17 | 14 | 0.82 | 0.36 |
KpII | KpIII | 38 | 27 | 32 | 31 | 0.75 | 0.39 |
TEM-1c | KpI + KpII + KpIII | 144 | 85 | 98 | 59 | 0.008 | 0.93 |
Ancient presence of the chromosomal beta-lactamase gene in K. pneumoniae.
In order to determine whether the chromosomal beta-lactamase gene was anciently present in K. pneumoniae, we estimated the time since the divergence of the K. pneumoniae phylogenetic groups based on the molecular-clock hypothesis, according to which the rate of nucleotide substitution along evolutionary lineages is roughly constant over time. To this end, we chose to analyze the mdh gene. Indeed, the nucleotide sequence of this housekeeping gene was determined previously for representatives of all E. coli phylogenetic groups and S. enterica subspecies (9). Since the divergence time between E. coli and S. enterica was estimated by several independent approaches (24, 40, 43, 45), the rate of evolution of the mdh gene could be calibrated.
The nucleotide sequence of a 342-bp portion of the mdh gene was established in the 28 strains under study. The 11 sequences found (Table (Table1)1) differed at 28 sites, 21 of which were phylogenetically informative. Two changes were observed in the second position of codons, and three were observed in the first position. Only these five changes induced amino acid replacements; the rest were synonymous. Table Table22 shows the levels of nucleotide sequence divergence found for the mdh gene within and between the K. pneumoniae phylogenetic groups KpI, -II, and -III. Similar to what was observed for both bla and gyrA, the divergence was higher within the KpII group than within KpI and KpIII, with a clear distinction between SB18 and SB59 on the one hand and strain SB30 and OR95:2 on the other hand.
Under the molecular-clock hypothesis, sequence variation at synonymous sites can be used to estimate the time since the existence of the last common ancestor (30, 42). For the mdh gene, the level of sequence divergence at synonymous sites, Ks, was estimated for the pairwise comparisons of the three K. pneumoniae groups. The estimated mean Ks values (with standard deviations) were 0.132 (0.011) for KpI vs. KpII, 0.179 (0.009) for KpI vs. KpIII, and 0.115 (0.012) for KpII vs. KpIII. In order to calibrate the rate of substitution, we used the two extreme values of various estimates proposed by other authors for the time since the divergence of E. coli and S. enterica: 140 (43) and 30 (40, 45) million years (Myr). The Ks value for the corresponding segment of the mdh gene for E. coli vs. S. enterica was 0.875 (0.073). Using the estimated ages of 30 or 140 Myr for E. coli and S. enterica would mean a rate of synonymous-site divergence of 6.25 × 10−9 or 1.34 × 10−9 substitutions per year, respectively. These rates would give an estimated time since the divergence of KpI and KpIII, the two groups showing the highest pairwise mdh sequence divergence, of 6 and 28 Myr, respectively.
DISCUSSION
In order to study the evolution of the chromosomal beta-lactamase gene in K. pneumoniae, we analyzed the diversity of the gene in strains representing the known range of K. pneumoniae genetic diversity. This approach revealed a close correspondence between the chromosomal beta-lactamase gene sequences and the previously defined phylogenetic groups of K. pneumoniae. It also made it possible to identify a new family of beta-lactamase variants.
Phylogenetic analyses of the bla, gyrA, and mdh sequences were all in agreement, showing three major groups. Our results firmly demonstrated the correspondence between the phylogenetic group KpI and the SHV family, as well as between group KpIII and the LEN family. Also, the pI values of the beta-lactamases paralleled the grouping; thus, pI 7.6 (SHV-like) was seen for all KpI strains and pI 7.1 (LEN-like) was seen for all KpIII strains.
Strain SB30 and strain OR95:2, a strain previously shown to harbor a chromosomal beta-lactamase gene different from both blaSHV and blaLEN alleles (20), clustered together with the two KpII reference strains, SB18 and SB59, based on the sequence data of bla, gyrA, and mdh, and we assigned their beta-lactamases to a new family that we named OKP. However, these four strains consistently formed two separate subgroups based on the three genes sequenced, one including strains SB18 and SB59 and the other including strains SB30 and OR95:2. Based on the strains characterized so far, the two subgroups in the new beta-lactamase family, OKP, exhibit distinct pI ranges, and their amino acid sequences differ by ~7%. It is possible that the study of more strains producing OKP-like enzymes will unravel additional beta-lactamase diversity both in pI values and in sequences and that the apparently clear-cut separation between the two subgroups will become blurred as intermediate variants are found. Alternatively, the phylogenetic discontinuity between the two subgroups may be confirmed in the future, in which case the two subgroups of beta-lactamases may be distinguished as OKP-A (for the variants harbored by strains SB18 and SB59) and OKP-B (for the variants harbored by strains SB30 and OR95:2). The susceptibility profiles of strains harboring OKP beta-lactamases were similar to those of strains harboring SHV and LEN beta-lactamases, suggesting similar activities of the chromosomal enzymes in the three families, as is known for the SHV and LEN families (34).
Interestingly, the pIs of the OKP beta-lactamases differ from the pIs observed for the chromosomal SHV (pI 7.6) and LEN (pI 7.1) enzymes. Therefore, the pIs of the three phylogenetic groups, if consistently found to be nonoverlapping, would represent a useful way to identify the beta-lactamase families of K. pneumoniae. Given the correspondence between the beta-lactamases and the phylogenetic groups, pI determination would also be useful for a presumptive identification of the phylogenetic group. For example, a K. pneumoniae beta-lactamase with a pI of 8.1 that was previously reported as an atypical chromosomal penicillinase (7) might be identified as the natural enzyme of a KpII strain.
Our results provide a new understanding of the distribution of beta-lactamases in K. pneumoniae. Indeed, the correspondence between the phylogenetic group KpI and SHV provides an answer to the currently debated question of the possible ubiquity of a chromosomal SHV gene in K. pneumoniae (Babini and Livermore, letter). Although we did not fully demonstrate the chromosomal locations of all of our beta-lactamase gene sequences, the presence of a single pI band, the failure of conjugation experiments, and the coevolution of bla and the two housekeeping genes gyrA and mdh, together with the well-documented chromosomal location of SHV and LEN genes (3, 15, 20), all support the notion of a chromosomal location of the beta-lactamase genes studied.
The fact that an SHV gene was found in all KpI strains investigated but in none of the KpII and KpIII strains indicates that blaSHV is generally present on the chromosomes of KpI strains but not on the chromosomes of KpII or KpIII strains, which instead harbor blaOKP or blaLEN, respectively. As KpI appears to be the most common K. pneumoniae group, at least in Europe (it is found in ~90% of K. pneumoniae clinical isolates studied from European hospitals [Brisse, unpublished]), the chromosomal SHV beta-lactamase is common, but not ubiquitous, in K. pneumoniae. A numerical dominance of SHV (68 strains) over LEN (14 strains) and other variants (16 strains) was also seen in the study of fecal isolates from neonates in Swedish special-care units (20).
Because the phylogeny of beta-lactamase genes is concordant with the phylogenies of the housekeeping genes gyrA and mdh, by far the most likely evolutionary origin of the three K. pneumoniae beta-lactamase families is diversification from a common ancestor, in parallel with the evolutionary divergence of the phylogenetic groups. In addition, data for the blaOKP alleles of the two subgroups within the phylogenetic group KpII suggest that diversification of the beta-lactamase genes also follows that of housekeeping genes between the two subgroups of KpII. In population genetics terms, the congruence of gene phylogenies indicates that clonal evolution is the major source of nucleotide variation in K. pneumoniae, at least for long-term divergence among phylogenetic groups. In other words, horizontal transfer among groups is limited to such an extent that it does not disrupt the genetic association of chromosomal loci, and the phylogeny based on a given gene reflects the overall phylogenetic relationships among strains. This finding parallels the findings of the population structure of E. coli and S. enterica, the closest evolutionary neighbors of K. pneumoniae, which have been studied in this respect and were found to exhibit a clonal population structure reflecting limited genetic exchange among strains. It is remarkable that the congruence among gene phylogenies is retained even for the beta-lactamase gene, as one could expect natural selection to raise to detectable levels the frequencies of strains that would have received an advantageous allele through a horizontal-transfer event, even if these events are very rare. Horizontal transfer is observed, for example, in E. coli and S. enterica for loci subjected to intense diversifying selection, such as those coding for flagellar or somatic antigens (8, 50). However, there was no evidence for selective events in the evolutionary history of the beta-lactamase gene. Also, the susceptibility data show that the three families of K. pneumoniae chromosomal beta-lactamases confer similar levels of resistance to broad-spectrum penicillins, so exchange of chromosomal beta-lactamase genes within the species would probably be of little selective advantage for the recipient strain. Recently, correspondence between beta-lactamase and housekeeping gene diversities was also observed in K. oxytoca (17, 18).
The stable association of beta-lactamase with the K. pneumoniae chromosome raises the question of the biological factors that would put the loss of the enzyme at a selective disadvantage. A likely explanation is that the natural habitat of K. pneumoniae would contain inhibitory amounts of beta-lactam antibiotics against which the chromosomal beta-lactamase confers resistance. Although K. pneumoniae is nowadays mostly known as a nosocomial pathogen, it is considered to be ubiquitous (19, 46). Its primary habitat may be soil or, for phylogenetic group KpIII, association with plants, as suggested by the fact that the two plant-associated strains in this study belonged to KpIII and that nitrogen-fixing strains associated with maize belong to KpIII (16). Several microorganisms known to produce penicillins and cephalosporins are present in the environment (48). The hypothesis of such an ecological role for the K. pneumoniae chromosomal beta-lactamase would imply a rapid epidemiological turnover whereby K. pneumoniae strains in human carriage would be regularly replaced by environmental strains. Otherwise, circulation among the human population for long enough periods would remove the apparent strict requirement for K. pneumoniae strains to maintain and express a beta-lactamase. Alternatively, the beta-lactamase could fulfill another physiological function in K. pneumoniae, besides conferring resistance to beta-lactams, as was suggested for the AmpC enzyme of E. coli (22).
Irrespective of the ecological or evolutionary factors favoring the maintenance of the class A chromosomal beta-lactamase in K. pneumoniae, this stable association with the chromosome resulted in diversification along K. pneumoniae phylogenetic lineages for a long evolutionary period. The range of time estimates for the last common ancestor of K. pneumoniae groups is wide, mainly due to the uncertainty about the E. coli-S. enterica split, but it shows that the diversification of the K. pneumoniae chromosomal beta-lactamase gene is ancient. This result parallels those, for example, of Barlow and Hall (4, 5), which indicate that the major part of the extant diversity of other families of beta-lactamases results from ancient evolutionary events rather than being the consequence of recent selective pressure by beta-lactam agents used in medicine. Exploring the diversity of resistance genes in bacteria representative of the phylogenetic diversity of bacterial groups, as we did here within K. pneumoniae, should be useful to uncover new beta-lactamases in those organisms in which these enzymes evolve in parallel with housekeeping genes. This is expected to be the case in clonal organisms harboring resistance genes in their chromosomes.
Acknowledgments
We thank L. G. Burman for contributions to early steps of this project and L. Gezelius for technical assistance with isoelectric focusing and MIC determinations. We are grateful to P. A. D. Grimont for providing the two environmental strains.
REFERENCES
Articles from Antimicrobial Agents and Chemotherapy are provided here courtesy of American Society for Microbiology (ASM)
Full text links
Read article at publisher's site: https://doi.org/10.1128/aac.48.7.2400-2408.2004
Read article for free, from open access legal sources, via Unpaywall:
https://europepmc.org/articles/pmc434173?pdf=render
Citations & impact
Impact metrics
Citations of article over time
Article citations
Diversity, functional classification and genotyping of SHV β-lactamases in <i>Klebsiella pneumoniae</i>.
Microb Genom, 10(10), 01 Oct 2024
Cited by: 0 articles | PMID: 39432416 | PMCID: PMC11493186
Review Free full text in Europe PMC
The Impact of Bamboo Consumption on the Spread of Antibiotic Resistance Genes in Giant Pandas.
Vet Sci, 10(11):630, 24 Oct 2023
Cited by: 1 article | PMID: 37999453 | PMCID: PMC10675626
Tetrandrine Derivatives as Promising Antibacterial Agents.
ACS Omega, 8(31):28156-28164, 26 Jul 2023
Cited by: 0 articles | PMID: 37576675 | PMCID: PMC10413380
Genetic Diversity of Polymyxin-Resistance Mechanisms in Clinical Isolates of Carbapenem-Resistant Klebsiella pneumoniae: a Multicenter Study in China.
Microbiol Spectr, e0523122, 27 Feb 2023
Cited by: 7 articles | PMID: 36847569 | PMCID: PMC10100843
Whole Genome Sequencing Reveals Presence of High-Risk Global Clones of Klebsiella pneumoniae Harboring Multiple Antibiotic Resistance Genes in Multiple Plasmids in Mwanza, Tanzania.
Microorganisms, 10(12):2396, 02 Dec 2022
Cited by: 2 articles | PMID: 36557648 | PMCID: PMC9785957
Go to all (92) article citations
Data
Data behind the article
This data has been text mined from the article, or deposited into data resources.
BioStudies: supplemental material and supporting data
Nucleotide Sequences (Showing 31 of 31)
- (2 citations) ENA - AJ635405
- (2 citations) ENA - AJ635426
- (2 citations) ENA - M59181
- (1 citation) ENA - AJ635401
- (1 citation) ENA - AJ635423
- (1 citation) ENA - AJ635424
- (1 citation) ENA - AJ635402
- (1 citation) ENA - AJ635421
- (1 citation) ENA - AJ635400
- (1 citation) ENA - AJ635422
- (1 citation) ENA - AJ635420
- (1 citation) ENA - AJ635409
- (1 citation) ENA - AJ635407
- (1 citation) ENA - AJ635408
- (1 citation) ENA - AJ635406
- (1 citation) ENA - AJ635403
- (1 citation) ENA - AJ635425
- (1 citation) ENA - AJ635404
- (1 citation) ENA - M24777
- (1 citation) ENA - AJ635372
- (1 citation) ENA - AJ635412
- (1 citation) ENA - AJ635413
- (1 citation) ENA - AJ635410
- (1 citation) ENA - AJ635411
- (1 citation) ENA - AJ635418
- (1 citation) ENA - AJ635419
- (1 citation) ENA - AJ635416
- (1 citation) ENA - AJ635417
- (1 citation) ENA - AJ635414
- (1 citation) ENA - AJ635415
- (1 citation) ENA - AF452105
Show less
Similar Articles
To arrive at the top five similar articles we use a word-weighted algorithm to compare words from the Title and Abstract of each citation.
Variants of the Klebsiella pneumoniae OKP chromosomal beta-lactamase are divided into two main groups, OKP-A and OKP-B.
Antimicrob Agents Chemother, 49(12):5149-5152, 01 Dec 2005
Cited by: 42 articles | PMID: 16304190 | PMCID: PMC1315974
An allelic variant of the chromosomal gene for class A beta-lactamase K2, specific for Klebsiella pneumoniae, is the ancestor of SHV-1.
Antimicrob Agents Chemother, 41(12):2705-2709, 01 Dec 1997
Cited by: 25 articles | PMID: 9420042 | PMCID: PMC164192
Molecular identification of (bla)SHV, (bla)LEN and (bla)OKP beta-lactamase genes in Klebsiella pneumoniae by bi-directional sequencing of universal SP6- and T7-sequence-tagged (bla)SHV-PCR amplicons.
Mol Cell Probes, 23(3-4):195-200, 01 Jun 2009
Cited by: 8 articles | PMID: 19496249
Phenotypic and genotypic characterization of β-lactam resistance in Klebsiella pneumoniae isolated from swine.
Vet Microbiol, 149(1-2):139-146, 07 Oct 2010
Cited by: 16 articles | PMID: 21035968