Abstract
Free full text

Microglial and macrophage polarization-new prospects for brain repair
Abstract
The historical view of the adult brain as a static organ has shifted in the last few decades. We now know that the mature brain remains plastic and has some regeneration capacity after injury. The injured brain engages microglia/macrophages to clear cellular debris and fine-tune neurorestorative processes. However, microglia/macrophage activation can also hinder central nervous system (CNS) repair and expand tissue damage. One explanation for this dualistic role of microglia/macrophages in neurological recovery is their polarization into different phenotypes at different stages of injury. This perspective article highlights the specific roles of polarized microglia/macrophages in CNS repair after acute injuries. We propose that therapeutic approaches targeting cerebral inflammation should be shifted from complete microglia/macrophage suppression toward a subtler titration of the balance between various phenotypes. Recent breakthroughs in the identification of regulatory molecules that control this dramatic phenotypic shift are accelerating the pace of research towards curing brain disorders.
For many decades, the adult central nervous system (CNS) was considered extremely limited in its capacity for neuroplasticity and regeneration. This traditional view has been considerably revised as it gradually became clear that diseases or injuries can trigger manifold repair processes in the adult brain, including neurogenesis, axonal sprouting, synaptogenesis, angiogenesis, oligodendrogenesis, and remyelination.1 Although these spontaneous responses may not lead to complete brain repair,2 the regenerative efforts of the adult CNS provide a novel therapeutic opportunity to boost natural defenses in compromised tissue and to restore a significant fraction of the neurovascular network.3, 4
Microglia and macrophages are among the most potent modulators of CNS repair/regeneration.5 However, these cells appear to be double-edged swords in the battle for neurological recovery. On the one hand, microglia/macrophage activation fosters brain recovery by clearing cell debris, resolving local inflammation, and releasing a plethora of trophic factors.5–8 On the other hand, microglia/macrophage activation can also hinder CNS repair and expand tissue damage.8–10 We propose that these seemingly contradictory functions of microglia/macrophage reflect their acquisition of distinct phenotypes in response to different microenvironmental cues. In in vitro settings, “classically activated” M1 microglia/macrophages typically release destructive pro-inflammatory mediators. In contrast, "alternatively activated" M2 phenotypes clear cellular debris through phagocytosis and release numerous protective/trophic factors11, 12 (Figure 1). However, recent studies have shown that the M1/M2 dichotomy is an oversimplified conceptual framework that only represents two extreme activation states. The status of microglia/macrophages in vivo, is much more complicated than in vitro and may include a spectrum of different but overlapping functional phenotypes. For example, a growing body of evidence reveals diversity in M2 phenotype subpopulations, such as M2a, M2b, M2c and Mox, each with unique physiological features and distinct biological functions.11–13 The current studies in CNS injuries have not yet characterized these subpopulations of M2 cells. The broad M1 and M2 classification has nevertheless persisted as a useful concept to enhance our understanding of microglia/macrophage functional status during injury progression and to help us explore new therapeutic strategies.11, 14 Emerging evidence now supports M1/M2 microglia/macrophage polarization in several types of acute CNS injuries, including stroke,15 traumatic brain injury (TBI),16 and spinal cord injury (SCI).17
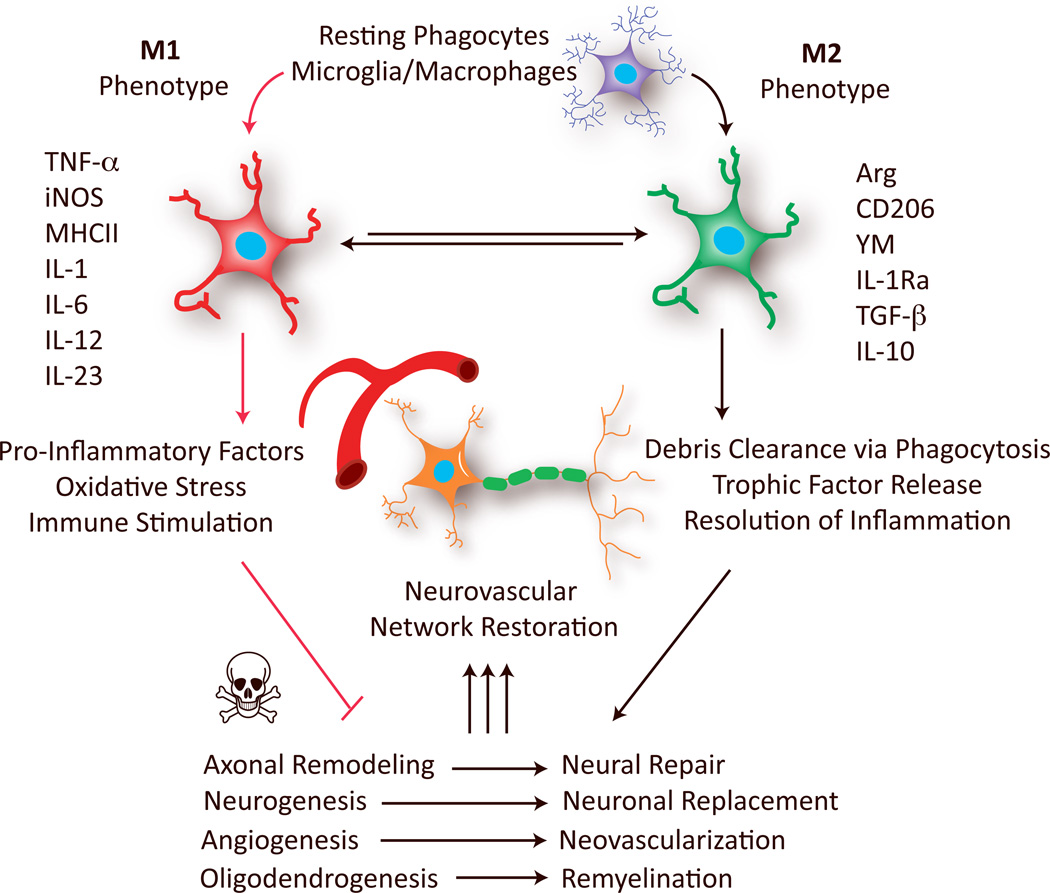
Microglia/macrophages polarize toward M1 or M2 phenotypes at various stages of stroke and other CNS injuries. M1 microglia/macrophages, characterized by the expression of M1 signatures genes (eg. TNFα, iNOS and IL-6), release pro-inflammatory factors and free radicals that impair brain repair/regeneration. In contrast, M2 microglia/macrophages, characterized by the expression of M2 signatures genes (eg. Arg, CD206 and IL-10), improve brain repair/regeneration by enhancing phagocytosis, releasing tropic factors, and resolving cerebral inflammation. Arg: arginase; IL: interleukin; IL-1Ra: IL-1 receptor antagonist; iNOS: inducible nitric oxide synthase; MHCII: major histocompatibility complex II; TGF-β, transforming growth factor-β; TNFα: tumor necrosis factor-α.
In this perspective, we discuss microglia/macrophage phenotypes in the context of CNS remodeling in response to acute injuries. A considerable body of literature suggests that activated microglia/macrophages with distinct phenotypes promote or interfere with neurological recovery after stroke or other injuries. In particular, the M2 phenotype is known to promote restorative processes, including neurogenesis, axonal remodeling, angiogenesis, oligodendrogenesis, and remyelination (Figure 1). Considering these evolutionarily adaptive functions of microglia/macrophages, we envision that future therapeutic approaches targeting cerebral inflammation will shift from total microglia/macrophage suppression to a subtler titration of the balance between different phenotypes. Recent findings on regulatory molecules that control phenotype switching, including extracellular stimulating factors and intracellular signaling molecules may accelerate the pace of research towards that goal.
Phenotypic dynamics
Microglia/macrophages are among the first responders to CNS injuries; they are mobilized within an hour18 and continue to accumulate for over a month.19 These cells do not remain static at the site of injury. Instead, they alter their morphology and exert a variety of important functions. Recent studies in various CNS injury models show that the majority of newly recruited microglia/macrophages at the site of injury express M2 signature genes, whereas microglia/macrophages expressing M1 genes dominate the landscape about 1 week after injury15–17, 20 This phenotype shift from M2-dominant to M1-dominant may result from a M2-to-M1 conversion within activated microglia/macrophages, as well as the continuing recruitment of pro-inflammatory M1 microglia/macrophages to the site of injury. It should be noted that the M2-to-M1 transition in microglia/macrophages after CNS injuries is distinct from the phenotypic changes observed in macrophage in non-CNS tissues, which usually shift from an initial pro-inflammatory M1 phenotype towards an inflammation-resolving M2 phenotype.21 The precise reasons for this difference are not yet clear. However, the inherent differences between microglia and macrophages might play a role.22, 23 In addition, the difference might be attributed at least partially to the existence of a blood brain barrier (BBB) and the formation of glial scar, both of which restrict the recruitment of “fresh” M2 macrophages and microglia to the site of injury during the recovery phase. Given the evidence in favor of a role for M2 microglia/macrophages in CNS regeneration, M2-to-M1 phenotypic switches may create an unfavorable microenvironment for CNS repair, which usually peaks several days after injury and lasts for a few weeks.24, 25
Microglia/macrophage polarization in CNS injury might be affected by many factors in addition to the timing after insults. For example, microglia/macrophages in the core of the ischemic zone are phenotypically different from those in the penumbra,15, 20 suggesting that the degree of injury influences microglia/macrophage polarization. In addition, although both white and grey matter exhibit the M2-to-M1 switch after injury16 or with aging,26 the temporal patterns of the switch differ between the two types of tissue and more prominent phenotypic changes occur in white matter.
Aging is another key factor affecting microglia/macrophage phenotype.27 The traditional view holds that aged microglia/macrophage are primed towards M1, dictating profound cytotoxicity and contributing to age-related deficits.27 However, this concept was recently challenged by findings of age-related microglial priming towards M2.28 This was accompanied by down-regulation of neurotoxic pathways and up-regulation of neuroprotective pathways. Further studies on the effects of aging on microglia/macrophage polarization will be critical for establishing therapeutics in age-related injuries such as stroke.
Although microglia are known as “brain macrophages” and share many functions with their peripheral macrophage cousins, many differences have been noted in the polarization of these two cell types. Polarized microglia are distinguished from polarized macrophages by protein expression, phagocytic capacity, and response to injuries.22, 23 Furthermore, M2 microglia seem to be more protective than M2 macrophages in vitro and show a greater tendency to maintain M2 status.22, 23 Validation of these observations in vivo has been challenging due to the paucity of markers to distinguish microglia from macrophages. The construction of bone marrow chimeras is the only currently available tool to tackle this question. Thus far, in vivo studies using this technique have confirmed that resident microglia and bone marrow-derived macrophages are both involved in phenotype switching,8 but may contribute differentially to CNS repair.29
Functions in brain repair
Neurogenesis
Recent evidence indicates that microglia/macrophage phenotype can exert positive or negative effects on adult neurogenesis after injury. For example, M1-activated microglia impair basal neurogenesis.9, 30 Pro-inflammatory microglia/macrophages that secrete destructive factors have also been shown to hinder neurogenesis and aggravate long-term neurological deficits after injury.31 In contrast, M2-activated microglia promote basal neurogenesis.30, 32 Following brain injuries, microglia/macrophages that produce specific trophic factors can enhance neural precursor cell (NPC) proliferation, neuroblast migration, and functional integration into existing neural circuitry.33, 34 Although distinct effects of M1 and M2 microglia/macrophages on neurogenesis have been demonstrated in vitro,30 pro-neurogenic and anti-neurogenic phenotypes are not clearly demarcated in vivo. Indeed, overlapping functions of different phenotypes may even co-exist. In line with this notion, chronic but delayed treatment with minocycline, a selective inhibitor of M1 microglia,35 promotes post-stroke neurogenesis and functional recovery.10 However, sustained microglial inhibition by minocycline beginning immediately after stroke actually suppresses neurogenesis.36 These seemingly conflicting results suggest that M1 microglia exert some pro-neurogenic functions after all, such as clearance of cell debris soon after brain injury. These findings suggest that future therapeutic strategies will need to boost the correct phenotype at the right time to improve stroke outcomes.
Axonal regeneration
Axonal regeneration after injury occurs to a limited degree in the adult CNS.37 Not surprisingly, M1-activated microglia/macrophages hinder axonal regeneration.38, 39 For example, microglia and macrophages activated by LPS or γ inhibit neurite outgrowth39 and induce axonal retraction in dystrophic neurons in vitro.38 Macrophage infiltration also correlates with axonal dieback in vivo.38 Although the untimely activation/infiltration of microglia/macrophages might be detrimental to injured axons, M2 macrophages are critical for axonal regeneration.40 Specialized subsets of macrophages that secrete protective molecules such as IL-10 and oncomodulin have been shown to promote axonal regrowth and recovery after SCI.7, 40 Similarly, in the damaged optic nerve, macrophage-derived oncomodulin promotes axonal regeneration.41, 42 In these various axonal regeneration models, macrophages appear to play a major role while microglia exert minor, if any, effects.40 These observations demonstrate that macrophages and microglia occupy distinct niches in the injured CNS.
Synaptic plasticity
Beyond their effects on axonal regeneration, microglia are known to regulate synaptic plasticity not only during development but also after CNS injuries.43, 44 Recent studies demonstrated that microglia directly modulate synaptic activities.45, 46 Whether microglial phenotypes differentially affect synapses is still unknown. However, intriguing studies revealed that the complement C1q complex, which can shift microglia toward the pro-inflammatory M1 phenotype, regulates synaptic pruning by tagging the to-be-eliminated synapses for microglial phagocytosis.47, 48 In addition, low levels of TNFα, a classic M1 inflammatory cytokine, are known to promote synaptic connectivity under physiological conditions.49 These studies all suggest that M1 microglia may possess a normal adaptive role in the regulation of synaptic homeostasis and plasticity. However, it remains to be elucidated whether and how the surge of these M1-related mediators after CNS injuries influences synapse remodeling.
White matter integrity
Distinct roles of polarized microglia/macrophages in oligodendrocyte (OL) renewal and white matter recovery have been documented. A switch from M1 to M2-dominant phenotypes was observed at the initiation of remyelination in models of multiple sclerosis.8 Selective depletion of M1 or M2 phenotypic cells has shown that the M2 phenotype promotes, while the M1 phenotype impairs OL regeneration.8 The exact function of polarized microglia/macrophages in white matter damage/repair after CNS injuries is still unknown. Our recent study of microglia/macrophages in TBI highlighted an M2-to-M1 phenotype shift at late stages of injury in the corpus callosum, the largest white matter structure in the brain.16 Notably, the number of M1 microglia/macrophages in the injured brain is strongly correlated with the severity of white matter injury.16 Several in vitro studies have revealed multitier effects of polarized microglia/macrophages on OLs. First, specific phenotypic characteristics of microglia/macrophages are known to influence OL survival. In an in vitro model of hypoxia/ischemia, M1 microglia-conditioned media exacerbated OL death induced by oxygen/glucose deprivation.16 Second, M1 and M2 microglia are thought to exert opposite effects on oligodendrogenesis. M1-activated microglia impair oligodendrogenesis in a TNF-α-dependent manner. In contrast, M2-activated microglia support oligodendrogenesis, at least partly through release of insulin-like growth factor-1 (IGF-1).30 In addition, M2 cell-conditioned media promotes oligodendrocyte precursor differentiation into mature OLs.8 These findings strongly suggest that microglia/macrophage polarization helps determine OL cell fate and white matter integrity after CNS injuries.
Angiogenesis and vascular repair
The angiogenic function of macrophages is already established in vascular repair during wound healing.50, 51 M2 macrophages produce robust pro-angiogenic factors such as vascular endothelial growth factor (VEGF)52 and IL-8.50 M2 macrophages also release pro-matrix metalloproteinase-9 (pro-MMP-9) in a unique, tissue inhibitor of metalloproteinases-1 (TIMP-1)-free form to enhance their angiogenic capacity.51 The phenotype-specific roles of microglia in angiogenesis have not yet been addressed. Nevertheless, the activation state of microglia has been reported to regulate brain endothelial cell proliferation, a key step for BBB repair and angiogenesis.53 Interestingly, the M1-type cytokine TNF-α and the M2-type cytokine TGF-β are known to exhibit distinct effects on endothelial proliferation. It is therefore possible that the polarization state of microglia is a key regulator of angiogenesis and BBB repair after CNS injury. The exact role of microglia/macrophage polarization in angiogenesis after CNS injuries awaits further investigation.
In summary, last decade has witnessed an explosion in research supporting phenotype-specific roles of microglia/macrophages in multiple steps of CNS repair/regeneration. This research, however, is mainly based on in vitro experiments or in vivo depletion of microglia/macrophages. To date, there is very little direct in vivo evidence demonstrating the involvement of microglia/macrophages in CNS repair. However, recent advances in in vivo imaging methods have provided novel, powerful tools to noninvasively observe microglia/macrophages in live animals (Box 1).54–56 Further identification of new markers of various phenotypes for in vivo imaging will lend additional insight into the precise role of microglia/macrophage polarization in CNS repair and may guide future therapeutic interventions targeting phenotype switching.
Modulation of phenotypes
In response to distinct microenvironmental cues, a fully polarized M1 or M2 subpopulation can reverse its phenotype and function.57 With increasing numbers of studies confirming the differential contributions of M1 and M2 microglia/macrophages to CNS repair, identification of the extracellular signals that trigger phenotypic shifts and the molecular switches inside microglia/macrophages that control such a dramatic change may lead to novel therapeutic strategies.
Extracellular signals
Microglia/macrophage phenotype shifts can be triggered by molecular cues from injured tissue.15, 58 For example, extracellular matrix proteins such as chondroitin sulfate proteoglycan (CSPG) increase substantially after SCI and promote M2 macrophage polarization.58 Inhibition of CSPG production by xyloside immediately after SCI shifts macrophage polarization toward M1, increases tissue loss, and impairs functional motor recovery. Furthermore, conditioned media collected from ischemic neurons primes microglial polarization toward M1,15 suggesting that injured neurons release soluble factors and/or shed their components to drive the M2-to-M1 shift. Compromised brain endothelial cells also release multiple cytokines that mediate microglia/macrophage polarization.59 In addition, infiltrating peripheral immune cells, particularly T lymphocytes, can interact with microglia/macrophages, inducing them to adopt either M1 or M2 phenotypes.60 For example, passive transfer of regulatory T lymphocytes from early disease-stage amyotrophic lateral sclerosis (ALS) mice into end-stage ALS mice results in sustained M2 microglial polarization and prolongs survival, supporting the importance of antigen-specific regulatory T cells in M2 induction.60 In general, Th1-derived supernatant that is abundant in IFN-γ, TNF-α and IL-2 induces M1 polarization. In contrast, Th2 and regulatory T cells that secrete IL-4 divert microglial polarization toward M2.60, 61 Because T cells are known to infiltrate into the injured CNS, it is possible that T cell-derived factors drive microglia/macrophage phenotype changes after CNS injuries. However, precisely which extracellular signals induce phenotype shifting after CNS injuries is not yet clear. Several phenotype inducers, such as TNF-α, IFN-γ, IL-6, lipocalin-2, TGF-β, galectin-1, bone morphogenetic protein-7 (BMP-7), substance-P, and IL-2562–67 are expressed in the CNS and may be upregulated after injury (Figure 2). It is possible that several of these molecules work synergistically or differentially at specific times after CNS injury to regulate phenotype shifts.
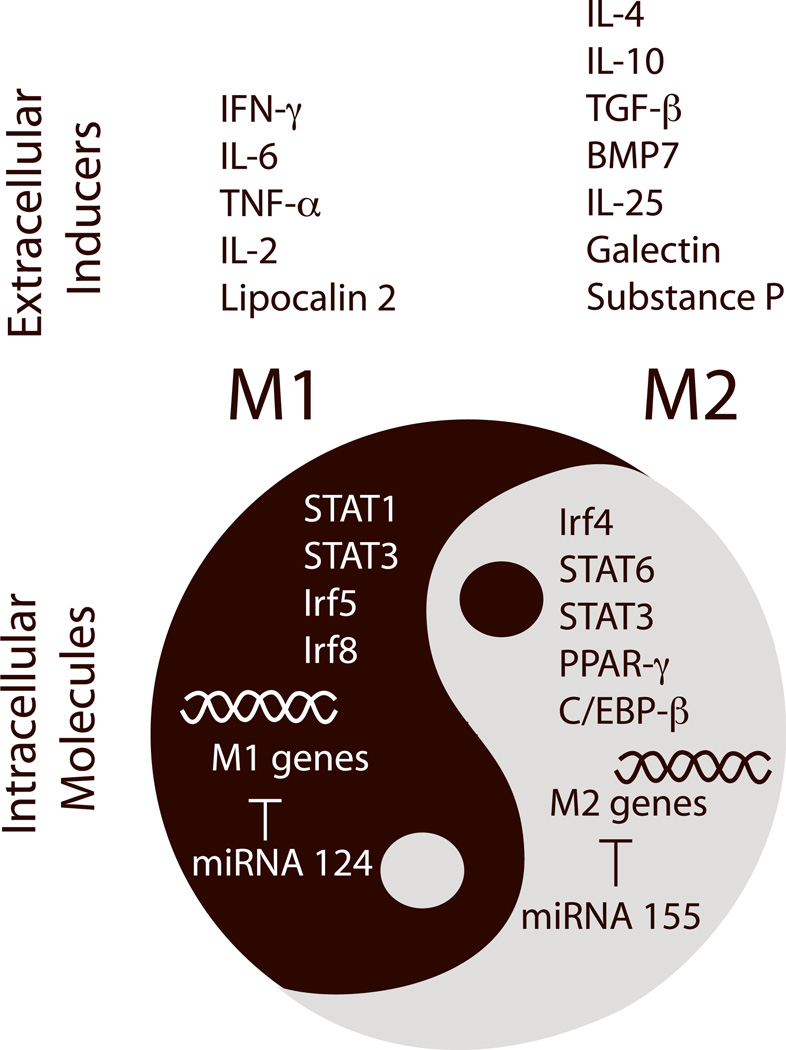
Recent studies have identified several intrinsic molecular switches that control phenotypic changes in microglia/macrophages. Targeting these molecular switches may lead to novel therapies that boost the repair function of microglia/macrophages and promote neurovascular network restoration after stroke and other CNS injuries. C/EBP-β: CCAAT-enhancer-binding protein-β; CREB: cyclic AMP response element binding protein; Irf: interferon response factor; miRNA: microRNA; PPAR-γ: peroxisome proliferator-activated receptor gamma-γ; STAT: signal transducer and activator of transcription.
Intracellular molecular switches
Breakthrough research on macrophages has revealed several transcriptional regulators that serve as central switches to turn on a group of the M1 or M2 genes and achieve polarization (Figure 2). The signal transducer and activator of transcription (STAT) family of transcription factors are well-known for their roles in cytokine action and immune regulation. In general, STATs are activated by Janus kinase (JAK) and suppressed by feedback inhibitors named suppressor of cytokine signaling (SOCS). Many members of the STAT family, including STAT1, STAT3, and STAT6, are involved in microglia/macrophage phenotype switching.68 For example, STAT1 enhances M1 polarization after IFN-γ exposure.69 STAT6 is important in establishing the M2 phenotype upon IL-13 and IL-4 stimulation.70, 71 The functions of STAT3 are diverse, as they involve both IL-10-stimulated M2 polarization72 and IL-6-stimulated M1 polarization.73 In addition to these cytokines, other wide-ranging factors such as heme oxygenase-1 and high density lipoproteins also influence macrophage phenotype through the SOCS/JAK/STAT pathway.74, 75
The nuclear hormone receptor peroxisome proliferator-activated receptor-γ (PPAR-γ) is another transcriptional factor that long been known to regulate microglia/macrophage phenotype. PPAR-γ mediates monocyte polarization towards alternative M2 macrophages with anti-inflammatory properties.76 As one might expect, PPAR-γ agonists (rosiglitazone and pioglitazone) are capable of inducing M2 phenotype switching in models of CNS diseases related to inflammation.77, 78
Several recent discoveries also highlight the importance of other transcription factors in phenotype modulation. For example, different subtypes of interferon response factors (Irf) exert distinct roles in macrophage polarization: Irf4 is a key transcription factor controlling M2 polarization,79 whereas Irf5 and Irf8 control M1 polarization.80, 81 The CCAAT-enhancer-binding protein-β (C/EBP-β) was also shown to induce M2 macrophage-specific gene expression, such as Arginase-1 and IL-10.82 Further characterization of these factors and their signaling pathways may provide an exciting array of potential therapeutic targets for CNS recovery.
Recent research has also defined a role for microRNA (miRNA) in microglia/macrophage polarization.83, 84 (Figure 2) M1- and M2-polarized microglia/macrophages exhibit distinct miRNA profiles.83 It is well accepted that miRNA-155 expression promotes M1 polarization by suppressing M2-signature genes85, 86 and that miRNA-124 enhances the M2 phenotype by targeting M1 genes.87 With the fast pace of miRNA research, increasing numbers of miRNAs have been associated with specific microglia/macrophage phenotypes.88, 89 However, further studies are required to confirm the specific function of these miRNAs in phenotype switching.
Our current knowledge about the intracellular molecules that regulate phenotype switching is mainly derived from studies in peripheral macrophages. The importance of these molecular switches in microglia/macrophage polarization after CNS injuries has not been verified. Nevertheless, several recent reports have documented changes in expression of these switch molecules and their possible involvement in CNS injuries (Table 1). For example, it has been shown that expression of Irf8 gradually decreases within 3d after stroke while the expression of Irf4 increases.90, 91 These trends are consistent with the peak in M2 microglia/macrophages 3–5d after stroke.15 Furthermore, Irf8 knockout protects against ischemic brain injury and ameliorates post-stroke inflammation.91 Although these two studies focused on the roles of neuronal Irf4 and Irf8, it is possible that the expression of these two molecules in microglia/macrophages regulates their phenotypes. This would explain, at least in part, the detrimental and protective changes after stroke observed in Irf4 and Irf8 knockout mice, respectively. In addition, some chemicals that are able to regulate the activity of phenotype switches (such as miRNA-124, PPAR-γ and STAT-1) have been shown to determine injury outcomes through microglia/macrophage-related mechanisms.78, 92, 93 In Table 1, we list several molecular switches that are related to CNS injuries. Further studies of the role of these molecules in microglia/macrophage polarization after CNS injuries and their involvement in CNS repair are highly warranted.
Table 1
Intrinsic phenotype switches involved in CNS injuries
Factors | Injury model | Increase or decrease | Effect | Refs |
---|---|---|---|---|
M1 switches | ||||
Irf8 | Mouse transient middle cerebral artery occlusion (tMCAO) | Gradually decreases within 3d after MCAO | Exacerbates brain injury | 91 |
STAT1 | Rat tMCAO | Increases late after MCAO (4, 7, 15d but not 6 and 24h) | STAT1 inhibitor | 92, 106 |
Mouse spinal cord injury (SCI) | __ | STAT1 inhibitor reduces inflammation and improves locomotor function after SCI | 107 | |
miR155 | Rat permanent middle cerebral artery occlusion (pMCAO), brain hemorrhage, kainate-induced seizure | Decreases soon after CNS injuries (within 24h) | __ | 108 |
M2 switches | ||||
Irf4 | Mouse tMCAO | Gradually increases within 3d after MCAO | Reduces brain injury | 90 |
PPAR-γ | Mouse/Rat intracerebral hemorrhage | __ | PPAR-γ agonist inhibits microglial inflammation and enhances phagocytosis | 78 |
Mouse tMCAO | __ | PPAR-γ agonist inhibits microglial inflammation | 109 | |
STAT-6 | Mouse tMCAO | Increases soon after tMCAO (1,3,12h after MCAO) | __ | 110 |
miRNA-124 | Intraplantar IL-1β-induced hyperalgesia | Decreases from 1–14d after injection | miRNA-124 enhances M2 polarization | 93 |
Dual effect on M1 and M2 polarization | ||||
STAT3 | Mouse neonatal hypoxia/ischemia (H/I) | Increases in microglia 1–2d after ischemia and lasts until 7d | Related to inflammatory responses after H/I | 111 |
Therapeutic perspectives
Our rapidly expanding knowledge on microglia/macrophage phenotypes offers novel therapeutic approaches to CNS remodeling. As M2 microglia/macrophages promote regeneration, transplantation of ex vivo activated M2 cells to counteract the M2-to-M1 shift at late stages of injuries is a promising cell-based regenerative strategy. However, a recent animal study demonstrated that M2 macrophage administration at subacute stages after middle cerebral occlusion (MCAO) failed to improve stroke outcomes after 2 weeks.94 Although this study is inconclusive due to the lack of a dose-response curve and long-term endpoints, it raises several important concerns about M2 microglia/macrophage therapy. What is the optimal route of administration? When should the cells be administered? Is CNS penetration essential for therapeutic effects? An additional concern is that microglia/macrophages are highly sensitive and may encounter unexpected extracellular cues during cell preparation and after transplantation, resulting in the reduction or loss of their protective M2 features. The application of genetically-engineered instead of ex vivo-induced M2 microglia/macrophage may help solve this problem. Although more comprehensive animal studies are required to determine the clinical potential of this cellular therapy, there are reasons to remain hopeful, as the vast majority of studies to date support the benefits of a carefully timed M1-to-M2 shift.8, 78 Furthermore, M2 transplantation has been shown to be effective in promoting neurological recovery in a model of multiple sclerosis.95
Besides cell transplantation, the protective factors produced by M2 microglia/macrophages represent additional opportunities to increase our armamentarium of restorative treatments (Table 2). Many of these factors, such as IGF-1 and oncomodulin, have already been tested in CNS injury models and exhibited efficacy.41, 96 Of course, the application of a single protective factor cannot fully replicate the beneficial effects of M2 microglia/macrophages, which involve the additive or synergistic actions of multiple protective molecules. In this regard, the identification of extracellular signals and intracellular molecular switches that control phenotypic changes provide two promising methods for microglia/macrophage-targeted regenerative therapy. The first approach is to turn on the molecular switch toward M2 or turn down the switch toward M1 activation. For example, nanoparticle-delivered siRNA can silence Irf5 in macrophages and improve the repair of myocardial infarcts and cutaneous wounds.97, 98 As nanomaterials cross the BBB with ease, this provides a promising avenue to deliver phenotype switching molecules to injured CNS tissue. In addition, inhibitors and inducers of key switch molecules have been identified,77,99 providing additional strategies for intervention. Therapies that target phenotype-related miRNAs or inhibitors of miRNAs are also under recent development. For example, cationic liposomes can deliver miRNAs into macrophages.87 The application of this approach in CNS injuries would depend on the capability of such liposomes to penetrate the compromised BBB. The second approach for phenotypic regulation is to supplement the microenvironment with M2-stimulating factors. For example, IL-4 is effective in reducing acute ischemic brain damage.100 As it has strong M2-inducing properties, its effect on long-term recovery deserves further investigation. Additionally, lentiviral delivery of M2 inducers, such as IL-10, into microglia/macrophages may induce M2 polarization and prevent the M2-to-M1 phenotype shift in inflammatory environments.101
Table 2
M2 microglia/macrophage-derived factors that promote neurovascular restoration.
Factors | Effect | Model | Refs |
---|---|---|---|
Activin A | Promotes oligodendrocyte differentiation and remyelination in corpus callosum | Lysolecithin-induced focal demyelination | 8 |
BDNF | Stimulates axonal regeneration and functional recovery | Spinal cord injury and surgical striatal injury | 112, 113 |
CXCL12 | Facilitates synaptic inputs into newly developing neurons upon their arrival at the ischemic lesion border zone | tMCAO in mouse | 114 |
Galectin-3 | Increases proliferation of NPCs in subventricular zone and enhances angiogenesis in ischemic striatum | tMCAO in spontaneously hypertensive rat | 33 |
GDNF | Stimulates axonal sprouting | Surgical striatal injury | 113 |
IGF-1 | Increases proliferation of NPCs in subgranular zone; enhances neurogenesis and oligodendrogenesis | Pilocarpine-induced status epilepticus in mouse | 30, 34 |
IL-10 | Promotes functional recovery after SCI | Spinal cord injury | 40 |
oncomodulin | Promotes neurite growth from dorsal root ganglion (DRG) or retinal ganglion (RG) neurons. | DRG or RG neuron culture Optic nerve crush in rat | 7, 41 |
Osteopontin | Attracts lateral migration of neuroblasts from the ipsilateral subventricular zone to the ischemic striatum | tMCAO in spontaneously hypertensive rat | 115 |
Protease serine 2 | Promotes the generation of neurons from optic nerve cells | Optic nerve cell culture | 32 |
Although the microglia/macrophage phenotypic switch offers a promising avenue for promoting CNS regeneration, clinical translation is a daunting task because our picture of microglia/macrophage phenotypes is still incomplete. As mentioned above, we have only begun to explore the dynamics of microglia/macrophage polarization. Further characterization of microglia/macrophage phenotypic behavior, especially its temporal and spatial evolution, will be critical in deciding the clinical treatment regimen. Furthermore, the action of many phenotype inducers is not a simple all-or-none phenomenon, but a function of timing and dose. An instructive example of additional challenges lying ahead is the proteoglycan CSPG, which has both beneficial and destructive properties in injured tissue. Although CSPG can induce the M2 phenotype switch during acute phases of SCI, it is a major component of scar tissue and known to hinder axonal regeneration.58 Caution against eliciting poorly timed M1-to-M2 phenotype switches in the clinic is also advisable because additional beneficial roles for M1 microglia may yet be discovered. It is unlikely that mammals evolved an M1 microglial/macrophage phenotype that only serves to exacerbate injury and exerts no positive role whatsoever. For example, as mentioned above, M1 microglia seem to contribute to synaptic remodeling and may also drive the clearance of cellular debris early after acute brain injuries. In addition, several factors that are known to be important in CNS repair, such as MMP-9,102 can be released not only from M2 but also from M1 microglia/macrophages.103 In this context, both pro-inflammatory and inflammation-resolving cells might be important for effective tissue repair at different time points after CNS injuries. Another potential concern is that the long-term maintenance of M2 phenotypes may negatively affect immune defense and result in serious health consequences, such as tumorigenesis.104 Therefore, we must be careful to boost the correct phenotype at the right time to enhance natural repair processes after CNS injuries.
Finally, we must also concede that most of our knowledge on microglia/macrophage polarization is derived from rodents. Unfortunately, there are many differences between rodent microglia/macrophages and their human counterparts.105 Although some in vitro studies suggest that human microglia and macrophages might be similarly polarized,23 whether all the features of polarization in rodent cells are truly applicable to humans still remains an important question. Additional studies using human biological systems,105 such as induced pluripotent stem cells, will therefore be essential for the ultimate translation of microglia/macrophage phenotype research into clinical practice.
Acknowledgements
Dr. Xiaoming Hu is supported by the American Heart Association (13SDG14570025) and the Ethyl Vincent pilot grant in multiple sclerosis from Department of Neurology, University of Pittsburgh. Dr. Rehana Leak is supported by a Commonwealth Universal Research Enhancement (C.U.R.E.) Award from the Pennsylvania Department of Health and a Michael J. Fox Foundation Innovation Award. Dr. Yanqin Gao is supported by the Chinese Natural Science Foundation grants (81171149 and 81371306). Dr. Jun Chen is supported by the National Institutes of Health Grants (NS45048, NS62157, NS59806, and NS36736), Chinese Natural Science Foundation grants (81228008) and a VA Merit Review.
References
Full text links
Read article at publisher's site: https://doi.org/10.1038/nrneurol.2014.207
Read article for free, from open access legal sources, via Unpaywall:
https://www.ncbi.nlm.nih.gov/pmc/articles/PMC4395497
Citations & impact
Impact metrics
Citations of article over time
Alternative metrics
Smart citations by scite.ai
Explore citation contexts and check if this article has been
supported or disputed.
https://scite.ai/reports/10.1038/nrneurol.2014.207
Article citations
STING orchestrates microglia polarization via interaction with LC3 in autophagy after ischemia.
Cell Death Dis, 15(11):824, 13 Nov 2024
Cited by: 0 articles | PMID: 39537618 | PMCID: PMC11560960
Microglia Signatures: A Cause or Consequence of Microglia-Related Brain Disorders?
Int J Mol Sci, 25(20):10951, 11 Oct 2024
Cited by: 0 articles | PMID: 39456734 | PMCID: PMC11507570
Review Free full text in Europe PMC
Neuroprotective effects of all-trans-retinoic acid are mediated via downregulation of TLR4/NF-κB signaling in a rat model of middle cerebral artery occlusion.
Neurosciences (Riyadh), 29(4):276-283, 01 Oct 2024
Cited by: 0 articles | PMID: 39379083 | PMCID: PMC11460776
Combined Analysis of Human and Experimental Rat Samples Identified Biomarkers for Ischemic Stroke.
Mol Neurobiol, 26 Sep 2024
Cited by: 0 articles | PMID: 39325100
Lactobacilli Cell-Free Supernatants Modulate Inflammation and Oxidative Stress in Human Microglia via NRF2-SOD1 Signaling.
Cell Mol Neurobiol, 44(1):60, 17 Sep 2024
Cited by: 0 articles | PMID: 39287687 | PMCID: PMC11408562
Go to all (733) article citations
Similar Articles
To arrive at the top five similar articles we use a word-weighted algorithm to compare words from the Title and Abstract of each citation.
Microglia in the TBI brain: The good, the bad, and the dysregulated.
Exp Neurol, 275 Pt 3:316-327, 03 Sep 2015
Cited by: 360 articles | PMID: 26342753 | PMCID: PMC4689601
Review Free full text in Europe PMC
Macrophage and microglial plasticity in the injured spinal cord.
Neuroscience, 307:311-318, 02 Sep 2015
Cited by: 65 articles | PMID: 26342747
Review
Functions and mechanisms of microglia/macrophages in neuroinflammation and neurogenesis after stroke.
Prog Neurobiol, 142:23-44, 07 May 2016
Cited by: 329 articles | PMID: 27166859
Review
Activated macrophage/microglial cells can promote the regeneration of sensory axons into the injured spinal cord.
Exp Neurol, 148(2):433-443, 01 Dec 1997
Cited by: 165 articles | PMID: 9417823
Funding
Funders who supported this work.
NINDS NIH HHS (8)
Grant ID: R01 NS036736
Grant ID: R01 NS062157
Grant ID: NS45048
Grant ID: NS59806
Grant ID: R01 NS045048
Grant ID: NS36736
Grant ID: NS62157
Grant ID: P01 NS059806
RRD VA (1)
Grant ID: I01 RX000420