Abstract
Free full text

PIN1 Inhibition Suppresses Osteoclast Differentiation and Inflammatory Responses
Abstract
Inflammatory responses and osteoclast differentiation play pivotal roles in the pathogenesis of osteolytic bone diseases such as periodontitis. Although overexpression or inhibition of peptidyl-prolyl cis/trans isomerase NIMA-interacting 1 (PIN1) offers a possible therapeutic strategy for chronic inflammatory diseases, the role of PIN1 in periodontal disease is unclear. The aim of the present study was to evaluate PIN1 expression in periodontitis patients as well as the effects of PIN1 inhibition by juglone or PIN1 small-interfering RNA (siRNA) and of PIN1 overexpression using a recombinant adenovirus encoding PIN1 (Ad-PIN1) on the inflammatory response and osteoclastic differentiation in lipopolysaccharide (LPS)- and nicotine-stimulated human periodontal ligament cells (PDLCs). PIN1 was up-regulated in chronically inflamed PDLCs from periodontitis patients and in LPS- and nicotine-exposed PDLCs. Inhibition of PIN1 by juglone or knockdown of PIN1 gene expression by siRNA markedly attenuated LPS- and nicotine-stimulated prostaglandin E2 (PGE2) and nitric oxide (NO) production, as well as cyclooxygenase-2 (COX-2) and inducible nitric oxide synthase (iNOS) expression, whereas PIN1 overexpression by Ad-PIN1 increased it. LPS- and nicotine-induced nuclear factor (NF)-κB activation was blocked by juglone and PIN1 siRNA but increased by Ad-PIN1. Conditioned medium prepared from LPS- and nicotine-treated PDLCs increased the number of tartrate-resistant acid phosphatase–stained osteoclasts and osteoclast-specific gene expression. These responses were blocked by PIN1 inhibition and silencing but stimulated by Ad-PIN1. Furthermore, juglone and PIN1 siRNA inhibited LPS- and nicotine-induced osteoclastogenic cytokine expression in PDLCs. This study is the first to demonstrate that PIN1 inhibition exhibits anti-inflammatory effects and blocks osteoclastic differentiation in LPS- and nicotine-treated PDLCs. PIN1 inhibition may be a therapeutic strategy for inflammatory osteolysis in periodontal disease.
Introduction
Both the periodontal microflora and the host response play critical roles in the initiation and progression of periodontal diseases (Albandar 2002). Lipopolysaccharide (LPS) from gram-negative bacteria is thought to be an important pathogenic factor and to contribute to alveolar bone resorption by inducing inflammatory cytokines, such as interleukin (IL)–1β, tumor necrosis factor (TNF)–α, and prostaglandin E2 (PGE2), in macrophages and neutrophilic leukocytes (Guiglia et al. 2013). Moreover, inducible nitric oxide synthase (iNOS)–derived nitric oxide (NO) and cyclooxygenase-2 (COX-2)–derived PGE2 levels play an important role in periodontitis by mediating inflammatory reactions in periodontal tissues (Leitão et al. 2005; Kurtis et al. 2007). LPS reportedly increases osteoclast formation by stimulating the receptor activator of nuclear factor κ-light-chain-enhancer of activated B cells (NF-κB)/receptor activator of NF-κB ligand (RANK/RANKL) signaling system (Sato et al. 2004). Thus, potential therapeutic strategies for the treatment of chronic inflammatory diseases (e.g., periodontitis) have been proposed; these include suppressing inflammatory responses and blocking osteoclast differentiation (Jimi et al. 2004).
Tobacco smoking may be an important risk factor for the development and severity of periodontitis (Johnson and Guthmiller 2007). Nicotine, one of the most abundant components of tobacco smoke, has been detected in the saliva and gingival crevicular fluid of smokers (McGuire et al. 1989). Thus, periodontal ligament cells (PDLCs) treated with nicotine plus LPS might be an in vitro model relevant to periodontitis in smokers with poor oral hygiene (Jeong GS et al. 2009; Pi et al. 2010; Jeong et al. 2011; Kim et al. 2012; Lee et al. 2013). The combination of nicotine and LPS exerts a synergistic effect on the expression and production of inflammatory mediators, including NO and PGE2, and on the expression of iNOS and COX-2 in PDLCs (Jeong GS et al. 2009; Pi et al. 2010; Kim et al. 2012). Conditioned medium (CM) from nicotine and LPS-treated Saos-2 osteoblasts stimulates the formation of osteoclast-like cells by increasing macrophage colony-stimulating factor (M-CSF) and PGE2, compared with that produced by nicotine treatment alone (Tanaka et al. 2006). Our recent study demonstrated that nicotine and LPS treatment up-regulate the mRNA expression of osteoclast markers, including RANKL and M-CSF in PDLCs (Lee et al. 2013). These results suggest that alveolar bone resorption in smokers is increased further by insufficient mouth cleaning.
Protein phosphorylation is a key cellular signaling mechanism that induces changes in protein conformation (Lu and Zhou 2007). Peptidyl-prolyl cis/trans isomerase NIMA-interacting 1 (PIN1) has been identified as a regulator of phosphorylation signaling that plays a part in the regulation of cell growth, genotoxic and other stress responses, and differentiation (Lu and Zhou 2007; Lee YM et al. 2014). It was recently demonstrated that PIN1 may be a therapeutic target in the treatment or prevention of inflammatory disease. In vivo inhibition of PIN1 by juglone reduced eosinophilic pulmonary inflammation in rat asthma (Esnault et al. 2007), rat allergic lung fibrosis (Shen et al. 2008), kidney fibrosis in rat ureteral occlusion (Reese et al. 2010), and inflammation in mice with type II collagen-induced rheumatoid arthritis (RA; Jeong HG et al. 2009). In vitro inhibition of PIN1 by juglone and PIN1 small-interfering RNA (siRNA) blocked ultraviolet A radiation-stimulated COX-2 expression and PGE2 production in mouse epidermal cells (Quyen et al. 2013) and decreased COX-2 expression in primary cultured chondrocytes from RA patients (Jeong HG et al. 2009). However, PIN1 knockdown in endothelial cells increased COX-2 induction and PGE2 production by LPS/interferon (Liu et al. 2009). In contrast, PIN1 overexpression by a PIN1 adenovirus (Ad-PIN1) attenuated carbon tetrachloride–induced acute liver injury in mice by modulating apoptotic signals and increasing NF-κB activity (Risal et al. 2012). Furthermore, PIN1-/- mice showed markedly worse liver injury after ischemia/reperfusion (Kuboki et al. 2009), significant bone loss with aging (Lee et al. 2009), reduced bone mineral density and trabecular bone volume (Shen et al. 2013), and larger osteoclasts without changes in the osteoclast number (Islam et al. 2014).
The transcription factor NF-κB regulates the expression of inflammatory genes, such as COX-2 and iNOS (Pi et al. 2010). PIN1 binds to the phosphorylated Thr254-Pro motif of p65 and subsequently inhibits IκBα binding, which increases the nuclear localization and activity of NF-κB (Ryo et al. 2003). Moreover, PIN1-knockout mice had reduced hepatic NF-κB activation and more liver injury after ischemia/reperfusion injury than did wild-type mice (Kuboki et al. 2009). We hypothesized that PIN1 may play a role in osteoclastogenesis and inflammatory response, as PIN1 is expressed in bone tissue, and primary cultured osteoblasts and osteoclasts (Shen et al. 2013), and PIN1 inhibition or overexpression exerts anti-inflammatory effects in various disease models.
The aim of the present study was to (1) investigate whether PIN1 is up-regulated in patients with periodontitis and in nicotine- and LPS-stimulated human PDLCs, (2) assess whether PIN1 inhibition or overexpression suppresses the nicotine- and LPS-induced inflammatory response in PDLCs and osteoclastic differentiation in mouse bone marrow–derived macrophages (BMMs), and (3) identify the underlying mechanisms in human PDLCs.
Materials and Methods
Gene Expression Profiling
To compare PIN1 mRNA expression between normal and diseased periodontal tissues, publicly available gene expression data sets for human samples were retrieved from the National Center for Biotechnology Information (NCBI) Gene Expression Omnibus (GEO) database (accession No. GSE7321, http://www.ncbi.nlm.nih.gov/geo/). GSE7321 is a microarray data set obtained from 32 patients at the Dental Medical School of the University of Göttingen (12 men, 20 women, aged 18–72 y) from March 2005 to December 2005 (Gersdorff et al. 2008). Determination of healthy and chronically inflamed PDLC groups was performed using clinical and radiological parameters (i.e., clinical attachment loss, increase in probing depth, and radiographic bone loss). Teeth with healthy PDL had no clinical signs of inflammation (no bleeding on probing), exhibited a probing depth of 3.5 mm, and had no detectable radiographic bone loss. In contrast, teeth with generalized chronic inflammation exhibited obvious clinical signals of inflammation, including a probing depth of >4 mm and radiographic evidence of bone loss (1–5 mm).
Patients and Biopsies
Gingival biopsies were obtained from 15 subjects undergoing periodontal surgery for disease-related or non–disease-related (e.g., esthetic) reasons at the Department of Periodontology, Kyung Hee University Dental Hospital (Seoul, Korea). Biopsies were harvested from single teeth and divided into 3 groups as follows: (1) healthy group (systemically and periodontally healthy subjects, with a high standard of oral hygiene, no bleeding on probing, and probing depth ≤3 mm in the selected sites; n = 5), (2) periodontitis group (subjects who had never smoked, were systemically healthy, and were clinically diagnosed with severe chronic periodontitis [e.g., probing depth ≥
7 mm and bleeding on probing]; n = 5), and (3) smoking group (systemically healthy subjects clinically diagnosed with severe chronic periodontitis [e.g., probing depth ≥7 mm and bleeding on probing] who had smoked ≥1 pack per day for at least 10 y; n = 5). Informed consent to use the tissues was obtained at the time of surgery.
Cell Culture
Immortalized human dental periodontal ligament cells transfected with the human telomerase catalytic component (hTERT) were kindly provided by Professor Takashi Takata (Hiroshima University, Japan; Kitagawa et al. 2006). Cells were cultured at 37 °C in Minimal Essential Medium α (α-MEM) supplemented with 10% fetal bovine serum (FBS), 100 U/mL penicillin, and 100 µg/mL streptomycin in a humidified atmosphere of 5% CO2.
Preparation of Recombinant PIN1 Adenovirus
An adenovirus encoding PIN1 (kindly provided by Professor Byung-Hyun Park, Jeonbuk National University and Republic of Korea) was created using the ViraPower Adenovirus Expression System according to the manufacturer’s instructions (Invitrogen, Carlsbad, CA, USA). The adenovirus was prepared and amplified as described previously (Zhou et al. 2013). The virus (MOI 100) was added to cells.
PIN1 siRNA Transfection
Cells were incubated in 6-well plates for 24 h and then transfected with control siRNA, PIN1 siRNA, or NF-κB siRNA (Bioneer Corporation, Daejeon, South Korea) using Lipofectamine 2000 (Gibco; Invitrogen Ltd., Paisley, UK) according to the manufacturer’s instructions.
Isolation of RNA and Reverse Transcription–Polymerase Chain Reaction
Total RNA was extracted from cells and tissues using TRIzol reagent (Life Technologies, Gaithersburg, MD, USA) according to the manufacturer’s instructions. cDNA synthesis was performed using an AccuPower RT PreMix kit (Bioneer Corporation) and oligo-dT primers. Primer sequences used for reverse transcription–polymerase chain reaction (RT-PCR) analysis were listed in our previous studies (Lee et al. 2013; Lee YM et al. 2014). The PCR products were resolved on a 1.2% agarose gel and stained with ethidium bromide.
Western Blot Analysis
Cells were solubilized in ice-cold 1% Triton X-100 lysis buffer. Proteins (20 µg) were mixed with an equal volume of 2× sodium dodecyl sulfate (SDS) sample buffer, boiled for 5 min, resolved by SDS-polyacrylamide gel electrophoresis (PAGE; 12% acrylamide), and transferred to nitrocellulose membranes. Protein bands were visualized by enhanced chemiluminescence (ECL) according to the manufacturer’s instructions and exposed to X-ray film.
Immunofluorescence Confocal Microscopy
Treated cells were fixed with cold 4% paraformaldehyde and blocked by treatment with 2% bovine serum albumin in Tris-buffered saline for 1 h, then incubated with rabbit anti–NF-κB p65 antibody (1:200; Santa Cruz Biotechnology, Santa Cruz, CA, USA). Cells were further incubated with a 1:1,000 dilution of DyLight 488 goat anti-rabbit immunoglobulin (Ig)G (Abcam, Cambridge, MA, USA) for 1 h. The samples were counterstained with 5 µg/mL propidium iodide for 15 min to identify the nuclei and analyzed by confocal microscopy (Carl Zeiss, Jena, Germany).
Quantification of NO and PGE2 Levels
Total NO production was determined as the nitrite concentration in the culture medium using a spectrophotometric assay based on the Griess reaction. The concentrations of PGE2 in culture supernatants were determined using an enzyme-linked immunosorbent assay kit (R&D Systems, Minneapolis, MN, USA), according to the manufacturer’s recommendations.
Osteoclast Differentiation Assays
Mouse BMMs obtained from 5-wk-old female ICR mice (Charles River Laboratories, Seoul, Korea) were used as osteoclast precursor cells for in vitro osteoclastogenesis experiments. CM was prepared from PDLCs treated with nicotine plus LPS, juglone, PIN1 siRNA, and Ad-PIN1 for 24 h. Mouse BMMs were incubated overnight in culture dishes with α-MEM supplemented with 10% FBS. After attached cells were discarded, floating cells were further cultured for 3 d in the presence of M-CSF (30 ng/mL). Upon 2-d treatment of BMMs with 30 ng/mL M-CSF and 100 ng/mL RANKL, >80% of the cells became mononuclear tartrate-resistant acid phosphatase (TRAP)–positive cells (preosteoclasts). To evaluate the osteoclastogenic activity of CM from PDLCs, we treated preosteoclasts with the CM mixture (60% CM plus 40% fresh α-MEM without M-CSF and RANKL) and further cultured the cells for up to 4 d to achieve mature osteoclast differentiation, as described previously (Lee et al. 2013; Lee SI et al. 2014). After 4 d of culture, cells were stained for TRAP activity with a leukocyte acid phosphatase kit (Sigma, St. Louis, MO, USA).
Co-immunoprecipitation
Stimulated cells were lysed in protein extraction solution (cat. No. 17081; iNtRON Biotechnology, Korea) containing protease and phosphatase inhibitor cocktail (Pierce Biotechnology, Rockford, IL, USA) and then centrifuged at 12,000 g for 15 min at 4 °C. Following protein isolation, 500 µg of total protein per sample was diluted with co-immunoprecipitation buffer and precleaned with normal mouse IgG and proteinA/G-plus beads (Santa Cruz Biotechnology) and then immunoprecipitated with an anti-PIN1 (Santa Cruz Biotechnology) antibody, respectively, overnight at 4 °C. After washing, the beads were boiled in 20 µL of SDS sample buffer, and 10 µL of the eluate was fractionated by 12% SDS-PAGE followed by Western blotting with anti-PIN1 or anti-p65 antibody. The reactive bands were detected with an ECL system (Amersham, Amersham, UK), used according to the manufacturer’s instructions.
RNA Extraction and RT-PCR
Total RNA was isolated using Trizol (Invitrogen) and reverse-transcribed to cDNA with RT Master Mix (Bioneer Corporation). The primers used to amplify PIN1 were 5′-AGG ACT TTG AGT CTC TGG CCT CAC-3′ (forward; F) and 5′-AGG CGT CTT CAA ATG GCT TCT G-3′ (reverse; R). The primers used to amplify β-actin cDNA were 5′-CAT GGA TGA TGA TAT CGC CGC G-3′ (F) and 5′ACA TGA TCT GGG TCA TCT TCT CG-3′ (R). Quantitative real-time PCR (qRT-PCR) was performed using SYBR Green PCR Master Mix (Roche Diagnostics, Basel, Switzerland) using the LightCycler (Roche Molecular Biochemicals, Indianapolis, IN, USA). Samples were run in triplicate, and the efficiency of each primer was calculated using an internal standard control. All values were normalized to that of β-actin.
Statistical Analysis
Data are expressed as the means ± standard deviation. Comparisons were performed using a 1-way analysis of variance (SPSS version 22.0, Chicago, IL, USA). All experiments were repeated at least 3 times, and results from representative experiments are shown.
Results
mRNA Expression of PIN1 in Healthy Controls and Periodontitis Patients
To determine the role of PIN1 in periodontal disease, we searched expression data for PIN1 in public expression databases and for probe set 200879_s_at on the Affymetrix U133 2.0+ platform in GEO (NCBI) for normal and diseased periodontal tissues. According to the expression data sets, mRNA expression of PIN1 was 39.97% higher in chronically inflamed PDLCs from patients with periodontitis compared with healthy PDLCs (Fig. 1A).

Expression of peptidyl-prolyl cis/trans isomerase NIMA-interacting 1 (PIN1) in periodontitis patients and nicotine plus lipopolysaccharide (LPS)-stimulated human periodontal ligament cells (PDLCs). (A) PIN1 gene expression in PDLCs from patients with chronic periodontitis and from healthy subjects. Data were derived from a microarray analysis study in a public expression database. (B) Comparison of relative PIN1 gene expression among healthy, nonsmoker periodontitis patients and smoker periodontitis patients. (C) Effects of LPS and nicotine on protein expression of PIN1. (D, E, F) Effects of the PIN1 inhibitor juglone on LPS- plus nicotine-induced PIN1, inducible nitric oxide (NO) synthase (iNOS), and cyclooxygenase-2 (COX-2) protein expression (C) and on NO and prostaglandin E2 (PGE2) secretion (D, E). Cells were incubated for 24 h with the indicated concentrations of nicotine and LPS (B). Cells were pretreated with juglone for 1h and then stimulated with LPS (1 µg/mL) plus nicotine (5 mM) for an additional 24 h (C, D, E). Production of NO and PGE2 was measured using the Griess assay and enzyme-linked immunosorbent assay (ELISA), respectively. Protein expression was determined by Western blot analysis. Western blot data representative of 3 independent experiments with similar results are shown. *P < 0.05 versus control; #P < 0.05 versus nonsmoker periodontitis or versus LPS plus nicotine (n = 4).
To verify the mRNA expression of PIN1 in periodontal tissue harvested from periodontal patients, gingival biopsies were taken from healthy and periodontally diseased individuals (Fig. 1B). PIN1 mRNA expression was significantly higher in patients with periodontitis than in periodontally healthy subjects. Furthermore, smoker subjects with periodontitis had a statistically significantly higher PIN1 expression than did nonsmoker periodontitis patients (P < 0.05).
Effects of Nicotine and LPS on PIN1 Protein Expression in Human PDLCs
To evaluate the effects of nicotine and LPS on PIN1 expression, human PDLCs were treated with various concentrations of nicotine and LPS for 24 h (Fig. 1C), which resulted in a concentration-dependent up-regulation of PIN1 protein compared with treatment of LPS or nicotine alone. Maximal induction of PIN1 was observed when the cells were treated with 1 µg/mL LPS plus 5 mM nicotine for 24 h.
Effects of PIN1 Inhibition and Overexpression on the Nicotine- and LPS-Induced Inflammatory Response in PDLCs
To understand the role of PIN1 in an in vitro model of periodontal disease, a series of functional studies was performed by inhibiting PIN1 with juglone, knocking down PIN1 expression with PIN1 siRNA, and overexpressing PIN1 with a PIN1-expressing adenovirus (Ad-PIN1) in nicotine- and LPS-stimulated PDLCs. Pretreatment with juglone concentration-dependently inhibited nicotine- and LPS-induced PIN1 expression, protein expression of inflammatory mediators such as COX-2 and iNOS, and production of NO and PGE2 (Fig. 1D–F). As shown in Figure 2, PIN1 siRNA knockdown significantly attenuated the effects of nicotine and LPS on COX-2 and iNOS protein expression, as well as NO and PGE2 production, whereas a control siRNA showed no effect in PDLCs. Moreover, adenovirus-mediated PIN1 overexpression accentuated nicotine- and LPS-induced COX-2 and iNOS up-regulation as well as production of NO and PGE2 (Fig. 2A–C).
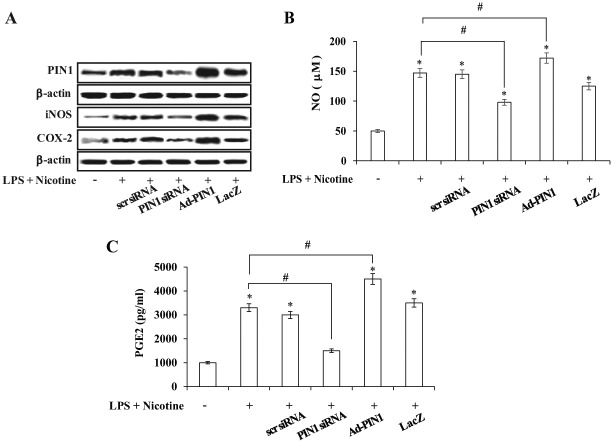
Effects of small-interfering RNA (siRNA)–mediated peptidyl-prolyl cis/trans isomerase NIMA-interacting 1 (PIN1) silencing and recombinant adenovirus encoding PIN1 (Ad-PIN1)–mediated PIN1 overexpression on nicotine- plus lipopolysaccharide (LPS)–induced PIN1, inducible nitric oxide synthase, and cyclooxygenase-2 expression (A) and on production of nitric oxide (NO) (B) and prostaglandin E2 (PGE2) (C) in periodontal ligament cells. Cells were pretreated with Ad-PIN1 and control adenovirus (Ad-LacZ) for 5 h or transfected with PIN1 siRNA or control siRNA (30 nM) for 12 h and then incubated with 5 mM nicotine and 1 µg/mL LPS for 24 h. Protein expression was assessed by Western blot analysis (A). Production of NO and PGE2 was measured using the Griess assay and enzyme-linked immunosorbent assay, respectively. *P < 0.05 versus control; #P < 0.05 versus LPS plus nicotine (n = 4).
Effects of PIN1 Inhibition and Overexpression on Nicotine- and LPS-Induced NF-κB Activation
The effects of PIN1 inhibition and activation on NF-κB signaling were assessed by Western blotting and confocal microscopy (Fig. 3A, ,B).B). Treatment with a PIN1 inhibitor and PIN1 siRNA blocked LPS- and nicotine-induced degradation of IκBα, phosphorylation of IκBα, and nuclear translocation of p65. By contrast, pretreatment with Ad-PIN1 increased NF-κB activation. In an immunofluorescence assay, NF-κB/p65 nuclear translocation in LPS- and nicotine-stimulated PDLCs was decreased by PIN1 inhibition but increased by Ad-PIN1 (Fig. 3B).
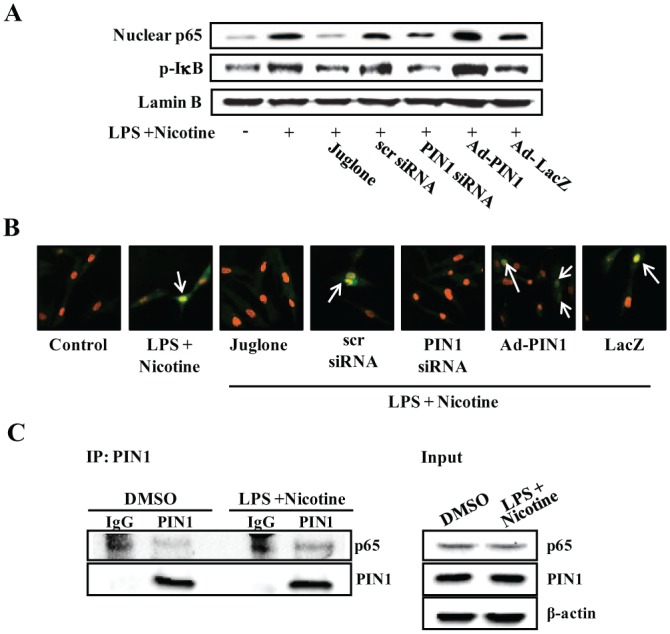
Effect of peptidyl-prolyl cis/trans isomerase NIMA-interacting 1 (PIN1) inhibition and overexpression on nicotine- and lipopolysaccharide (LPS)–induced nuclear factor kappa–light-chain-enhancer of activated B cells (NF-κB) activation in periodontal ligament cells (PDLCs). Cells were pretreated with adenovirus encoding PIN1 for 5 h or juglone for 1 h and transfected with PIN1 small-interfering RNA (siRNA) or control siRNA (30 nM) for 12 h. Cells were then incubated with 5mM nicotine and 1 µg/mL LPS for 60 min. NF-κB signaling was assessed by Western blot analysis (A) and immunofluorescent staining (B). A change in the nucleus color from red to yellow (due to co-localization of green fluorescein isothiocyanate fluorescence and red propidium iodide fluorescence, arrows) was indicative of NF-κB translocation in the cells. (C) Physical interaction between PIN1 and p65. Cells were treated with or without nicotine plus LPS for 1 h. Cells were collected, and total protein was extracted. PIN1 binding to p65 was confirmed by immunoprecipitation against PIN1 using a PIN1-specific antibody prior to immunoblotting for p65 and PIN1. The data are representative of 3 independent experiments. This figure is available in color online at http://jdr.sagepub.com.
To determine whether a p65-PIN1 interaction occurs in PDLCs, co-immunoprecipitation experiments were performed. Immunoprecipitation was then carried out using an anti-PIN1 antibody, followed by immunoblotting for p65 and PIN1 (Fig. 3C). Endogenous PIN1 and p65 interacted in the unstimulated state, which was enhanced upon nicotine and LPS stimulation. This suggests that physical interaction between PIN1 and p65 could increase the stability and nuclear translocation of p65 through inhibition of IκBα binding to p65.
Effects of PIN1 Inhibition and Overexpression on Osteoclastogenesis
To examine whether PIN1 inhibition and overexpression affected nicotine and LPS-mediated osteoclastogenic molecules, PDLCs were either transfected with PIN1 siRNA or Ad-PIN1 and pretreated with juglone, following 24-h exposure to nicotine and LPS. Juglone and PIN1 siRNA blocked nicotine- and LPS-induced up-regulation of RANKL. However, Ad-PIN1 enhanced nicotine- and LPS-stimulated RANKL expression (Fig. 4A).
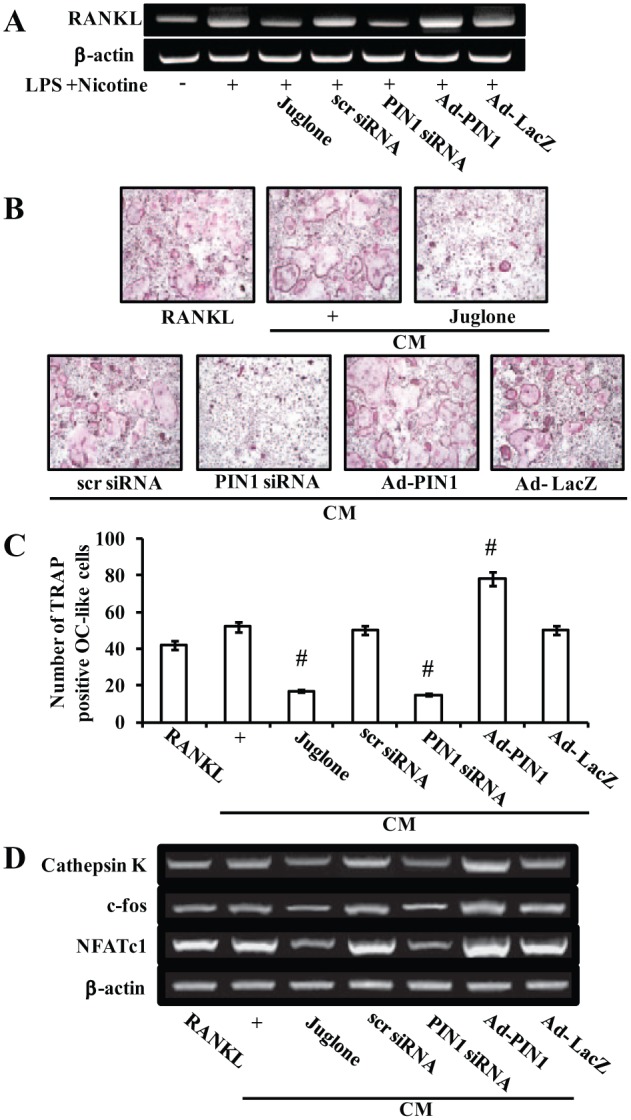
Effects of peptidyl-prolyl cis/trans isomerase NIMA-interacting 1 (PIN1) inhibition and overexpression on osteoclastogenesis. Effects of PIN1 inhibition and overexpression on nicotine- and lipopolysaccharide (LPS)–induced receptor activator of NF-κB ligand (RANKL) and osteoprotegerin (OPG) expression in periodontal ligament cells (PDLCs) (A). Effect of PIN1 inhibition and overexpression on the osteoclastogenic effects of conditioned medium (CM) from nicotine- and LPS-treated PDLCs, as assessed using an in vitro osteoclast differentiation system (B–D). PDLCs were pretreated with adenovirus encoding PIN1 for 5 h, juglone for 1 h, and PIN1 small-interfering RNA for 12 h and then incubated with 5 mM nicotine and 1 µg/mL LPS for 24 h (A). PDLCs were cultured in the presence of 5 mM nicotine and 1 µg/mL LPS for 1 d, and CM was prepared as described in the Materials and Methods section. At the end of culture, cells were stained for tartrate-resistant acid phosphatase activity (B). The numbers of osteoclasts per well were counted (C). mRNA expression of osteoclast-specific marker genes was assessed by reverse transcription–polymerase chain reaction (D). Similar data were obtained from 3 independent experiments. #P < 0.05 versus CM collected from nicotine- and LPS-treated PDLCs.
To elucidate the effects of PIN1 inhibition and activation on osteoclastogenesis in vitro, preosteoclasts were allowed to differentiate into mature osteoclasts in CM prepared from PDLCs treated with nicotine plus LPS, juglone, PIN1 siRNA, or Ad-PIN. TRAP staining revealed that osteoclast formation was increased in PIN1-overexpressing cells but decreased by juglone and PIN1 siRNA (Fig. 4B, ,C).C). RANKL-induced expression of osteoclastogenesis marker genes, such as cathepsin K, c-fos, and NFATc1, were up-regulated by PIN1 overexpression but down-regulated by PIN1 inhibition (Fig. 4D).
To identify possible destructive mediators of LPS- and nicotine-stimulated PIN1 expression, a broad range of proinflammatory cytokines implicated in osteoclast differentiation was examined. PIN1 inhibition attenuated LPS- and nicotine-induced TNF-α, IL-1β, IL-6, IL-11, IL-17, and IL-23 mRNA expression, whereas PIN1 activation had the opposite effect. IL-15 was not affected by PIN1 inhibition or overexpression (Fig. 5A).
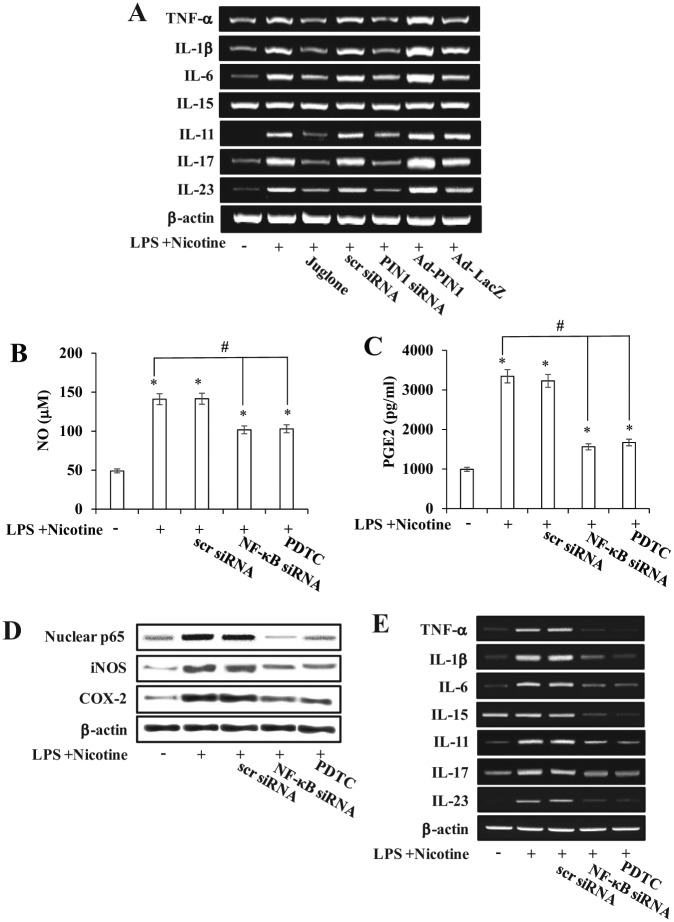
Effects of peptidyl-prolyl cis/trans isomerase NIMA-interacting 1 (PIN1) inhibition and overexpression on inflammatory response. Effects of PIN1 inhibition and overexpression on nicotine- and lipopolysaccharide (LPS)–induced up-regulation of osteoclastogenic cytokines (A). Effects of the NF-κB inhibitor pyrrolidine dithiocarbamate (PDTC) or NF-κB small-interfering RNA (siRNA) on nicotine- plus LPS-induced inducible nitric oxide synthase and cyclooxygenase-2 (D), osteoclastogenic cytokine expression (E), and production of nitric oxide (NO) (B) and prostaglandin E2 (PGE2) (C) in periodontal ligament cells (PDLCs). Cells were pretreated with PDTC (1mM) for 1 h or NF-κB siRNA (30 nM) for 12 h (B-E). PDLCs were treated with Ad-PIN1 for 5 h, juglone for 1 h, and PIN1 siRNA for 12 h and then incubated with 5 mM nicotine and 1 µg/mL LPS for 24 h (A–E). mRNA and protein expression was determined by reverse transcription–polymerase chain reaction and Western blot analysis, respectively (A, D, E). Production of NO and PGE2 was measured using the Griess assay and enzyme-linked immunosorbent assay, respectively (B, C). Data were obtained from 3 independent experiments.
To confirm that the NF-κB pathway was involved in the LPS- and nicotine-induced production of inflammatory mediators in PDLCs, we used an NF-κB inhibitor, pyrrolidine dithiocarbamate (PDTC), or NF-κB gene silencing. PDTC and NF-κB siRNA decreased the nicotine- and LPS-induced expression of iNOS and COX-2 proteins as well as NO and PGE2 production (Fig. 5B–D). In addition, LPS- and nicotine-mediated TNF-α, IL-1β, IL-6, IL-11, IL-17, and IL-23 mRNA induction were inhibited by PDTC and NF-κB siRNA (Fig. 5E).
Discussion
In the present study, we used a PDLC line immortalized by hTERT transfection (Kitagawa et al. 2006), which retains the features of their original primary cells (i.e., PDLCs). Thus, this immortalized cell line is a helpful tool for studying the biology and mechanisms of PDLCs.
PIN1 mRNA or protein up-regulation was detected in vivo in periodontitis patients based on publicly available microarray data (Gersdorff et al. 2008) and our real-time PCR data, as well as in vitro in PDLCs stimulated with the major periodontopathogens, nicotine, and LPS. Up-regulation of PIN1 was also observed in radiation-exposed mouse skin tissues and JB6 Cl41 epidermal cells (Han et al. 2011) and in chondrocytes, lymphocytes, and fibroblasts from RA lesions in mice with type II collagen-induced RA (Jeong HG et al. 2009). Since PIN1 is up-regulated in periodontitis patients and nicotine- and LPS-stimulated PDLCs, we evaluated PIN1 as a potential target for periodontitis.
Juglone, 5-hydroxy-1,4-naphthoquinone, inhibits members of the parvulin peptidyl-prolyl isomerase (PPIase) family and functions by modifying sulfhydryl groups (Hennig et al. 1998). The compound irreversibly inhibits the enzymatic activity of PIN1 by inactivating a unique cysteine residue in the PIN1 active site (Hennig et al. 1998). It has been suggested that juglone inhibits transcription in vitro by inhibiting preinitiation complex formation by modifying sulfhydryl groups of RNA polymerase II (Chao et al. 2001). Juglone is frequently used to explore the relevance of PIN1 function in in vivo and in vitro, particularly since it often phenocopies the effects of PIN1 dominant-negative mutants or PIN1 knockdown (Esnault et al. 2007; Reese et al. 2010). PIN1 transcription and its protein levels fluctuate during cell cycle progression in nontransformed cells (Ryo et al. 2002). Moreover, juglone or PIN1 siRNA blocks cell cycle progression and triggers cell death (Wiegand et al. 2009). In line with these reports, we found that treatment with juglone and PIN1 siRNA down-regulated protein and mRNA expression of PIN1 in a concentration-dependent manner (Fig. 1C; Appendix Fig.). In addition, juglone inhibits PIN1 function by suppressing mRNA expression of PIN1 (Appendix Fig.). These results suggest that juglone specifically affects PIN1 transcription, at least in PDLCs.
Our results revealed that juglone, a chemical inhibitor of PIN1, and PIN1 siRNA blocked the LPS- and nicotine-induced increases in iNOS and COX-2 expression, as well as NO and PGE2 production. By contrast, PIN1 activation by Ad-PIN1 accentuated the LPS- and nicotine-induced inflammatory responses. These data suggest that PIN1 inhibition exerts anti-inflammatory effects in PDL cells in vitro. Our results are consistent with data showing that juglone reduced RA progress in a collagen-induced RA mouse model and suppressed COX-2 expression in human primary RA chondrocytes (Jeong HG et al. 2009).
Our results indicate that nicotine- and LPS-induced NF-κB activation was blocked by juglone and PIN1 siRNA and increased by PIN1 overexpression. Since the expression of these proinflammatory mediators is modulated by NF-κB, our findings suggest that the inhibition of proinflammatory mediator production by juglone and PIN1 siRNA was due to blockade of the NF-κB signaling pathway. These results are in agreement with a previous report that PIN1 knockdown decreased TNF-α–induced NF-κB activation in U251-MG cells, resulting in inhibition of IL-8 expression (Atkinson et al. 2009). Similarly, NF-κB activation in PIN1-overexpressing chondrocytes is associated with overproduction of proinflammatory mediators in RA progression (Jeong HG et al. 2009).
It was recently reported that Pin1-/- mice exhibit a defect in bone morphogenetic protein signaling in osteoblasts but normal osteoclast function (Shen et al. 2013). However, Islam et al. (2014) demonstrated wide variation in the sizes of osteoclasts in Pin1-/- mice, with very high numbers of large osteoclasts. Since Pin1-/- mice show significant bone loss with aging (Lee et al. 2009), we examined whether PIN1 is involved in osteoclast differentiation as well as up-regulation of osteoclastogenic cytokine expression in BMMs (the culmination of this process is periodontal bone destruction). Osteoclast formation and expression of osteoclastogenic genes, such as cathepsin K, c-fos, and NFATc1, was inhibited by CM from juglone- and PIN1 siRNA-treated cells but increased by Ad-Pin1 CM. To our knowledge, this is the first demonstration that PIN1 mediates the inhibitory effect of nicotine and LPS on osteoclast differentiation.
It was recently demonstrated that periodontitis with inflammation or destruction of periodontal tissue and resorption of alveolar bone results in increased production of several osteoclastogenic cytokines, such as IL-1, IL-6, and TNF-β (Kobayashi et al. 2000; Kim et al. 2010). In the present study, inhibition of PIN1 by juglone or PIN1 siRNA blocked the nicotine- and LPS-stimulated expression of TNF-α, IL-1β, IL-6, IL-11, IL-17, and IL-23, whereas PIN1 overexpression accentuated it. Our results suggest that induction of the expression of osteoclastogenic cytokines such as TNF-α, IL-1β, IL-6, IL-11, IL-17, and IL-23 by PIN1 modulation leads to osteoclast differentiation.
In summary, this study is the first to demonstrate that PIN1 inhibition blocks nicotine- and LPS-induced inflammatory responses and osteoclast differentiation, possibly through osteoclastogenic cytokines. PIN1 inhibition is a potential therapeutic strategy in osteolytic bone diseases such as periodontal disease.
Author Contributions
Y.-A. Cho, S.-S. Jue, contributed to conception, design, data acquisition, analysis, and interpretation, drafted and critically revised the manuscript; W.-J. Bae, contributed to design, data acquisition, drafted and critically revised the manuscript; S.-H. Heo, contributed to conception, data acquisition, and analysis, drafted and critically revised the manuscript; S.-I. Shin, contributed to design, data acquisition, and analysis, drafted and critically revised the manuscript; I.-K. Kwon, S.-C. Lee, contributed to conception and data interpretation, drafted and critically revised the manuscript; E.-C. Kim, contributed to conception, design, data analysis, and interpretation, drafted and critically revised the manuscript. All authors gave final approval and agree to be accountable for all aspects of the work.
Footnotes
A supplemental appendix to this article is published electronically only at http://jdr.sagepub.com/supplemental.
This work was supported by a grant from the National Research Foundation of Korea (NRF) funded by the Korean government (MSIP; 2012R1A5A2051384), the Mid-Career Researcher Program through an NRF grant funded by the Ministry of Education, Science and Technology (2012004117), and Kyung Hee University in 2014 (KHU-20140680).
The authors declare no potential conflicts of interest with respect to the authorship and/or publication of this article.
References
- Albandar JM. 2002. Global risk factors and risk indicators for periodontal diseases. Periodontol 2000. 29:177–206. [Abstract] [Google Scholar]
- Atkinson GP, Nozell SE, Harrison DK, Stonecypher MS, Chen D, Benveniste EN. 2009. The prolyl isomerase Pin1 regulates the NF-κB signaling pathway and interleukin-8 expression in glioblastoma. Oncogene. 28(42):3735–3745. [Abstract] [Google Scholar]
- Chao SH, Greenleaf AL, Price DH. 2001. Juglone, an inhibitor of the peptidyl-prolyl isomerase Pin1, also directly blocks transcription. Nucleic Acids Res. 29(3):767–773. [Europe PMC free article] [Abstract] [Google Scholar]
- Esnault S, Rosenthal LA, Shen ZJ, Sedgwick JB, Szakaly RJ, Sorkness RL, Malter JS. 2007. A critical role for Pin1 in allergic pulmonary eosinophilia in rats. J Allergy Clin Immunol. 120(5):1082–1088. [Abstract] [Google Scholar]
- Gersdorff N, Miró X, Roediger M, Geffers R, Toepfer T, Huels A, Miosge N. 2008. Gene expression analysis of chronically inflamed and healthy human periodontal ligament cells in vivo. Dent Res J. 5(1):5–11. [Google Scholar]
- Guiglia R, Di Fede O, Lo Russo L, Sprini D, Rini GB, Campisi G. 2013. Osteoporosis, jawbones and periodontal disease. Med Oral Patol Oral Cir Bucal. 18(1):93–99. [Europe PMC free article] [Abstract] [Google Scholar]
- Han CY, Hien TT, Lim SC, Kang KW. 2011. Role of Pin1 in UVA-induced cell proliferation and malignant transformation in epidermal cells. Biochem Biophys Res Commun. 410(1):68–74. [Abstract] [Google Scholar]
- Hennig L, Christner C, Kipping M, Schelbert B, Rücknagel KP, Grabley S, Küllertz G, Fischer G. 1998. Selective inactivation of parvulin-like peptidyl-prolyl cis/trans isomerases by juglone. Biochemistry. 37(17):5953–5960. [Abstract] [Google Scholar]
- Islam R, Bae HS, Yoon WJ, Woo KM, Baek JH, Kim HH, Uchida T, Ryoo HM. 2014. Pin1 regulates osteoclast fusion through suppression of the master regulator of cell fusion DC-STAMP. J Cell Physiol. 229(12):2166–2174. [Abstract] [Google Scholar]
- Jeong GS, Lee SH, Jeong SN, Kim YC, Kim EC. 2009. Anti-inflammatory effects of apigenin on nicotine- and lipopolysaccharide-stimulated human periodontal ligament cells via heme oxygenase-1. Int Immunopharmacol. 9(12):1374–1380. [Abstract] [Google Scholar]
- Jeong GS, Lee DS, Li B, Kim JJ, Kim YC, Kim EC. 2011. Anti-inflammatory effects of lindenenyl acetate via heme oxygenase-1 and AMPK in human periodontal ligament cells. Eur J Pharmacol. 670(1):295–303. [Abstract] [Google Scholar]
- Jeong HG, Pokharel YR, Lim SC, Hwang YP, Han EH, Yoon JH, Ahn SG, Lee KY, Kang KW. 2009. Novel role of Pin1 induction in type II collagen-mediated rheumatoid arthritis. J Immunol. 183(10):6689–6697. [Abstract] [Google Scholar]
- Jimi E, Aoki K, Saito H, D’Acquisto F, May MJ, Nakamura I, Sudo T, Kojima T, Okamoto F, Fukushima H, et al. 2004. Selective inhibition of NF-κB blocks osteoclastogenesis and prevents inflammatory bone destruction in vivo. Nat Med. 10(6):617–624. [Abstract] [Google Scholar]
- Johnson GK, Guthmiller JM. 2007. The impact of cigarette smoking on periodontal disease and treatment. Periodontol 2000. 44:178–194. [Abstract] [Google Scholar]
- Kim YS, Lee YM, Park JS, Lee SK, Kim EC. 2010. SIRT1 modulates high-mobility group box 1-induced osteoclastogenic cytokines in human periodontal ligament cells. J Cell Biochem. 111(5):1310–1320. [Abstract] [Google Scholar]
- Kim YS, Shin SI, Kang KL, Chung JH, Herr Y, Bae WJ, Kim EC. 2012. Nicotine and lipopolysaccharide stimulate the production of MMPs and prostaglandin E2 by hypoxia-inducible factor-1α up-regulation in human periodontal ligament cells. J Periodontal Res. 47(6):719–728. [Abstract] [Google Scholar]
- Kitagawa M, Kudo Y, Iizuka S, Ogawa I, Abiko Y, Miyauchi M, Takata T. 2006. Effect of F-spondin on cementoblastic differentiation of human periodontal ligament cells. Biochem Biophys Res Commun. 349(3):1050–1056. [Abstract] [Google Scholar]
- Kobayashi K, Takahashi N, Jimi E, Udagawa N, Takami M, Kotake S, Nakagawa N, Kinosaki M, Yamaguchi K, Shima N, et al. 2000. Tumor necrosis factor alpha stimulates osteoclast differentiation by a mechanism independent of the ODF/RANKL–RANK interaction. J Exp Med. 191(2):275–286. [Europe PMC free article] [Abstract] [Google Scholar]
- Kuboki S, Sakai N, Clarke C, Schuster R, Blanchard J, Edwards MJ, Lentsch AB. 2009. The peptidyl-prolyl isomerase, Pin1, facilitates NF-kappaB binding in hepatocytes and protects against hepatic ischemia/reperfusion injury. J Hepatol. 51(2):296–306. [Europe PMC free article] [Abstract] [Google Scholar]
- Kurtis B, Tüter G, Serdar M, Pinar S, Demirel I, Toyman U. 2007. Gingival crevicular fluid prostaglandin E(2) and thiobarbituric acid reactive substance levels in smokers and non-smokers with chronic periodontitis following phase I periodontal therapy and adjunctive use of flurbiprofen. J Periodontol. 78(1):104–111. [Abstract] [Google Scholar]
- Leitão RF, Ribeiro RA, Chaves HV, Rocha FA, Lima V, Brito GA. 2005. Nitric oxide synthase inhibition prevents alveolar bone resorption in experimental periodontitis in rats. J Periodontol. 76(6):956–963. [Abstract] [Google Scholar]
- Lee SI, Kim GT, Kim HJ, Park SH, Kim EC. 2014. NOD2 mediates odontoblast differentiation and RANKL Expression. J Dent Res. 93(7):678–684. [Europe PMC free article] [Abstract] [Google Scholar]
- Lee SK, Chung JH, Choi SC, Auh QS, Lee YM, Lee SI, Kim EC. 2013. Sodium hydrogen sulfide inhibits nicotine and lipopolysaccharide-induced osteoclastic differentiation and reversed osteoblastic differentiation in human periodontal ligament cells. J Cell Biochem. 114(5):1183–1193. [Abstract] [Google Scholar]
- Lee TH, Tun-Kyi A, Shi R, Lim J, Soohoo C, Finn G, Balastik M, Pastorino L, Wulf G, Zhou XZ, et al. 2009. Essential role of Pin1 in the regulation of TRF1 stability and telomere maintenance. Nat Cell Biol. 11(1):97–105. [Europe PMC free article] [Abstract] [Google Scholar]
- Lee YM, Shin SY, Jue SS, Kwon IK, Cho EH, Cho ES, Park SH, Kim EC. 2014. The role of PIN1 on odontogenic and adipogenic differentiation in human dental pulp stem cells. Stem Cells Dev. 23(6):618–630. [Europe PMC free article] [Abstract] [Google Scholar]
- Liu T, Schneider RA, Shah V, Huang Y, Likhotvorik RI, Keshvara L, Hoyt DG. 2009. Protein never in mitosis gene A interacting-1 regulates calpain activity and the degradation of cyclooxygenase-2 in endothelial cells. J Inflamm (Lond). 22:6–20. [Europe PMC free article] [Abstract] [Google Scholar]
- Lu KP, Zhou XZ. 2007. The prolyl isomerase PIN1: a pivotal new twist in phosphorylation signalling and disease. Nat Rev Mol Cell Biol. 8(11):904–916. [Abstract] [Google Scholar]
- McGuire JR, McQuade MJ, Rossmann JA, Garnick JJ, Sutherland DE, Scheidt MJ, Van Dyke TE. 1989. Cotinine in saliva and gingival crevicular fluid of smokers with periodontal disease. J Periodontol. 60(4):176–181. [Abstract] [Google Scholar]
- Pi SH, Jeong GS, Oh HW, Kim YS, Pae HO, Chung HT, Lee SK, Kim EC. 2010. Heme oxygenase-1 mediates nicotine- and lipopolysaccharide-induced expression of cyclooxygenase-2 and inducible nitric oxide synthase in human periodontal ligament cells. J Periodontal Res. 45(2):177–183. [Abstract] [Google Scholar]
- Quyen BT, Choi HK, Kang KW. 2013. Pin1 is required for ultraviolet A-stimulated cyclooxygenase-2 induction in mouse epidermal cells. Cancer Lett. 335(1):31–40. [Abstract] [Google Scholar]
- Reese S, Vidyasagar A, Jacobson L, Acun Z, Esnault S, Hullett D, Malter JS, Djamali A. 2010. The Pin 1 inhibitor juglone attenuates kidney fibrogenesis via Pin 1-independent mechanisms in the unilateral ureteral occlusion model. Fibrogenesis Tissue Repair. 3:1. [Europe PMC free article] [Abstract] [Google Scholar]
- Risal P, Park BH, Cho BH, Kim JC, Jeong YJ. 2012. Overexpression of peptidyl-prolyl isomerase Pin1 attenuates hepatocytes apoptosis and secondary necrosis following carbon tetrachloride-induced acute liver injury in mice. Pathol Int. 62(1):8–15. [Abstract] [Google Scholar]
- Ryo A, Liou YC, Wulf G, Nakamura N, Lee SW, Lu KP. 2002. Pin1 is an E2F target gene essential for the Neu/Ras-induced transformation of mammary epithelial cells. Mol Cell Biol. 22(15):5281–5295. [Europe PMC free article] [Abstract] [Google Scholar]
- Ryo A, Suizu F, Yoshida Y, Perrem K, Liou YC, Wulf G, Rottapel R, Yamaoka S, Lu KP. 2003. Regulation of NF-κB signaling by Pin1-dependent prolyl isomerization and ubiquitin-mediated proteolysis of p65/RelA. Mol Cell. 12(6):1413–1426. [Abstract] [Google Scholar]
- Sato N, Takahashi N, Suda K, Nakamura M, Yamaki M, Ninomiya T, Kobayashi Y, Takada H, Shibata K, Yamamoto M, et al. 2004. MyD88 but not TRIF is essential for osteoclastogenesis induced by lipopolysaccharide, diacyl lipopeptide, and IL-1alpha. J Exp Med. 200(5):601–611. [Europe PMC free article] [Abstract] [Google Scholar]
- Shen ZJ, Esnault S, Rosenthal LA, Szakaly RJ, Sorkness RL, Westmark PR, Sandor M, Malter JS. 2008. Pin1 regulates TGF-beta1 production by activated human and murine eosinophils and contributes to allergic lung fibrosis. J Clin Invest. 118(2):479–490. [Abstract] [Google Scholar]
- Shen ZJ, Hu J, Ali A, Pastor J, Shiizaki K, Blank RD, Kuro-o M, Malter JS. 2013. Pin1 null mice exhibit low bone mass and attenuation of BMP signaling. PLoS One. 8(5):e63565. [Europe PMC free article] [Abstract] [Google Scholar]
- Tanaka H, Tanabe N, Shoji M, Suzuki N, Katono T, Sato S, Motohashi M, Maeno M. 2006. Nicotine and lipopolysaccharide stimulate the formation of osteoclast-like cells by increasing macrophage colony-stimulating factor and prostaglandin E2 production by osteoblasts. Life Sci. 78(15):1733–1740. [Abstract] [Google Scholar]
- Wiegand S, Dakic B, Rath AF, Makarova G, Sterz C, Meissner W, Bette M, Adamkiewicz J, Müller-Brüsselbach S, Müller R, et al. 2009. The rotamase Pin1 is up-regulated, hypophosphorylated and required for cell cycle progression in head and neck squamous cell carcinomas. Oral Oncol. 45(10):e140. [Abstract] [Google Scholar]
- Zhou L, Park BH, Park JH, Jang KY, Park HS, Wagle S, Lee KB, Kim JR. 2013. Overexpression of the prolyl isomerase PIN1 promotes cell growth in osteosarcoma cells. Oncol Rep. 29(1):193–198. [Abstract] [Google Scholar]
Articles from Journal of Dental Research are provided here courtesy of International and American Associations for Dental Research
Full text links
Read article at publisher's site: https://doi.org/10.1177/0022034514563335
Read article for free, from open access legal sources, via Unpaywall:
https://europepmc.org/articles/pmc4438738?pdf=render
Citations & impact
Impact metrics
Citations of article over time
Smart citations by scite.ai
Explore citation contexts and check if this article has been
supported or disputed.
https://scite.ai/reports/10.1177/0022034514563335
Article citations
Amentoflavone Induces Ferroptosis to Alleviate Proliferation, Migration, Invasion and Inflammation in Rheumatoid Arthritis Fibroblast-like Synoviocytes by Inhibiting PIN1.
Cell Biochem Biophys, 01 Oct 2024
Cited by: 0 articles | PMID: 39354278
The double-edged nature of nicotine: toxicities and therapeutic potentials.
Front Pharmacol, 15:1427314, 14 Aug 2024
Cited by: 1 article | PMID: 39206262 | PMCID: PMC11350241
Review Free full text in Europe PMC
SARS-CoV-2, periodontal pathogens, and host factors: The trinity of oral post-acute sequelae of COVID-19.
Rev Med Virol, 34(3):e2543, 01 May 2024
Cited by: 1 article | PMID: 38782605
Review
Nicotine in Inflammatory Diseases: Anti-Inflammatory and Pro-Inflammatory Effects.
Front Immunol, 13:826889, 18 Feb 2022
Cited by: 29 articles | PMID: 35251010 | PMCID: PMC8895249
Review Free full text in Europe PMC
Shared Molecular Mechanisms between Atherosclerosis and Periodontitis by Analyzing the Transcriptomic Alterations of Peripheral Blood Monocytes.
Comput Math Methods Med, 2021:1498431, 03 Dec 2021
Cited by: 8 articles | PMID: 34899963 | PMCID: PMC8664523
Go to all (25) article citations
Data
Data behind the article
This data has been text mined from the article, or deposited into data resources.
BioStudies: supplemental material and supporting data
GEO - Gene Expression Omnibus
- (1 citation) GEO - GSE7321
Similar Articles
To arrive at the top five similar articles we use a word-weighted algorithm to compare words from the Title and Abstract of each citation.
HIF-2 Inhibition Supresses Inflammatory Responses and Osteoclastic Differentiation in Human Periodontal Ligament Cells.
J Cell Biochem, 116(7):1241-1255, 01 Jul 2015
Cited by: 26 articles | PMID: 25565665
Anti-inflammatory and anti-osteoclastogenic effects of zinc finger protein A20 overexpression in human periodontal ligament cells.
J Periodontal Res, 51(4):529-539, 09 Nov 2015
Cited by: 21 articles | PMID: 26548452
Thymosin Beta-4 Suppresses Osteoclastic Differentiation and Inflammatory Responses in Human Periodontal Ligament Cells.
PLoS One, 11(1):e0146708, 20 Jan 2016
Cited by: 14 articles | PMID: 26789270 | PMCID: PMC4720371
Pin1 Modulation in Physiological Status and Neurodegeneration. Any Contribution to the Pathogenesis of Type 3 Diabetes?
Int J Mol Sci, 19(8):E2319, 08 Aug 2018
Cited by: 3 articles | PMID: 30096758 | PMCID: PMC6121450
Review Free full text in Europe PMC