Abstract
Free full text

BRAF(V600E) co-opts a conserved MHC class I internalization pathway to diminish antigen presentation and CD8+ T-cell recognition of melanoma
Abstract
Oncogene activation in tumor cells induces broad and complex cellular changes that contribute significantly to disease initiation and progression. In melanoma, oncogenic BRAF(V600E) has been shown to drive the transcription of a specific gene signature that can promote multiple mechanisms of immune suppression within the tumor microenvironment. We show here that BRAF(V600E) also induces rapid internalization of MHC class I (MHC-I) from the melanoma cell surface and its intracellular sequestration within endolysosomal compartments. Importantly, MAP kinase inhibitor treatment quickly restored MHC-I surface expression in tumor cells, thereby enhancing melanoma antigen-specific T-cell recognition and effector function. MAP kinase pathway-driven re-localization of HLA-A*0201 required a highly conserved cytoplasmic serine phosphorylation site previously implicated in rapid MHC-I internalization and recycling by activated immune cells. Collectively, these data suggest that oncogenic activation of BRAF allows tumor cells to co-opt an evolutionarily conserved MHC-I trafficking pathway as a strategy to facilitate immune evasion. This link between MAPK pathway activation and the MHC-I cytoplasmic tail has direct implications for immunologic recognition of tumor cells, and provides further evidence to support testing therapeutic strategies combining MAP kinase pathway inhibition with immunotherapies in the clinical setting.
INTRODUCTION
Two hallmarks of melanoma are the frequent presence of MAP kinase (MAPK) pathway-activating oncogenic mutations and immune suppression within the tumor microenvironment (TME) (1). Several recent studies support that these two hallmarks are intimately linked, with oncogenic signaling regulating the transcription of multiple genes that can collectively suppress the antitumor immune response. These include upregulating immunomodulatory chemokines and cytokines that promote recruitment and activation of suppressive immune cell subsets into the TME, in addition to downregulating the expression of melanoma differentiation antigens that can be recognized by cytotoxic T lymphocytes (2–8). We show here that oncogenic BRAF V600E mutations, which are the most prevalent (~50%) activating kinase mutations in melanoma, may also promote immune escape by directly modulating the surface expression and intracellular distribution of MHC class I (MHC-I) molecules in tumor cells. BRAF(V600E) signaling in melanoma cells leads to specific, constitutive internalization of MHC-I from the tumor cell surface and its intracellular sequestration within endocytic compartments. This reduces melanoma-specific CD8+ T-cell recognition and function. The effect is reversed by clinically relevant MAPK pathway inhibitors. The BRAF(V600E)-induced cellular redistribution of MHC-I is rapid and requires a highly conserved serine phosphorylation site within the MHC-I cytoplasmic domain, showing strong similarities to a specialized MHC-I trafficking pathway utilized by activated immune cells (9–12). These results show that oncogenic BRAF V600E mutations directly reduce CD8+ T-cell recognition of melanomas by co-opting a conserved internalization pathway involving the MHC-I cytoplasmic tail.
MATERIALS AND METHODS
Cell culture and lentiviral transduction
Four human melanoma cell lines were studied; two lines expressed V600E-mutated BRAF (Mel888 and WM793) and two lines expressed wild-type (WT) BRAF (CHL1 and Mewo). All cell lines were obtained from Dr. Michael Davis Lab, at the M.D. Anderson Cancer Research Center. All cells were verified by DNA fingerprinting within 6 months of initiating these studies. All cell lines were cultured in RPMI 1640 medium containing 10% fetal bovine serum (Gibco), 1% penicillin-streptomycin (Pen-Strep, Cellgrow), and maintained at 37°C in 5% CO2. HLA-A2 negative Mel888 and WM793 parental cells were transduced to express HLA-A*0201 variants using lentiviral gene transfer vectors as previously described (13). The human phosphoglycerate kinase (hPGK) promoter was used to drive the expression of WT HLA-A*0201 or one of three cytoplasmic tail variants: ΔTail, S335A, or Y320A. Transduced cells expressing comparable levels of surface HLA-A*0201 were isolated by cell sorting and used in subsequent studies. MART-1(27–35) specific tumor-infiltrating lymphocytes (TIL) were maintained in TIL culture media containing RPMI-1640, 10% human serum AB (Gemini), 0.1% 2-mercaptoethanol (Gibco), 1% sodium pyruvate, 1% Pen-Strep, and 3000 IU/mL of IL2 (Proleukin, Novartis).
MAP kinase pathway inhibitors and flow cytomteric analyses
The BRAF(V600E)-specific inhibitor (BRAFi) dabrafenib GSK2118436 and MEK inhibitor (MEKi) trametinib GSK1120212 (Selleckchem) were used in these studies to inhibit MAPK pathway activation. Melanoma cell lines were seeded at 1.0 × 106 cells in a 12-well plate and cultured in the presence of BRAFi (50nM), MEKi (50nM), or DMSO (solvent control) for different time points. This concentration was chosen because it effectively blocked the MAPK pathway without inducing rapid cell death or toxicity (Suppl. Figs. 1 and 4). Surface MHC-I expression was quantified by staining cells with anti-human HLA A,B,C-APC (W6/32) or anti-human HLA-A2-APC (BB7.2, Biolegend) and measuring fluorescence using a FACScanto II flow cytometer (BD Biosciences). Data were further analyzed using Flowjo analysis software (Treestar).
T-cell assays
TILs obtained from a HLA-A*0201-positive melanoma patient TIL infusion product and showing >98% MART-1(27–35)-specificity by tetramer staining were used for all CD8+ T-cell assays. Transduced Mel888 cells (which endogenously express MART-1) were co-cultured with MART-specific TILs at a 1:1 effector-to-target ratio (10,000 cells each) at 37°C for 4 hours, following a 3-hour pre-incubation with BRAFi (50nM) or DMSO. Transduced WM793 cells (which do not express MART-1) were pulsed with titrated concentrations of MART-1(27–35) peptides and washed prior to co-culture. TILs were incubated with melanoma cell lines for 4 hours in the presence of GolgiStop (BD Biosciences), washed, fixed, permeabilized, and stained with anti-human IFNγ conjugated to APC (BD Biosciences). Intracellular IFNγ production by CD8+ T cells was then determined by flow cytometric analysis. Mel888 cells were also co-incubated with TILs for 8 hours, after which time supernatant was collected and analyzed by ELISA to measure IFNγ concentration (eBiosciences). Plates were read using SpectraMax® M5/M5e Multimode Plate Reader and analysis program.
Statistical analysis
Graph Pad Prism 6 was used for graphing data and statistical analysis. A student’s t-test was used to analyze the statistical significance of all flow cytometry data. A one-way ANOVA test was used to analyze confocal microscopy quantification data. A p-value less than or equal to 0.05 was the threshold used to determine statistical significance.
Confocal Microscopy
Due to space restrictions, confocal microscopy methods are included in Supplemental Data.
RESULTS
MAP kinase pathway inhibition induces rapid upregulation of MHC class I surface expression in BRAF mutant melanoma cell lines
To assess the impact of BRAF(V600E) mutation on expression of immunomodulatory genes in melanoma, primary human melanocytes were lentivirally transduced to express green fluorescent protein (GFP) alone, or BRAF(V600E) plus GFP concurrently (4). Flow cytometric analysis of multiple transduced melanocyte lines revealed that ectopic overexpression of BRAF(V600E) consistently induced substantial reductions (3 to 10-fold) in surface expression of HLA-A,B,C (Figures 1A, B).

(A) Flow cytometric analysis showing HLA-A,B,C surface expression in human dermal melanocytes transduced with lentiviral vectors expressing BRAF(V600E) and GFP or GFP alone. (B) Summary of MHC-I expression in 3 melanocyte lines, each transduced in triplicate. MFI, mean fluorescence intensity. (C) Melanoma cell lines harboring V600E-mutated BRAF (Mel888 and WM793) or WT BRAF (CHL1) were treated for 3h with either DMSO, dabrafenib 50 nM (BRAFi), or trametinib 50 nM (MEKi), then stained for total HLA-A,B,C and analyzed by flow cytometry. (D) Time course experiment showing total MHC-I upregulation in Mel888, WM793, and CHL1 melanoma cells treated with dabrafenib for 30 minutes, 60 minutes, or 3 hours, as measured by flow cytometry. (E) Confocal microscopy images of DMSO- or BRAFi-treated Mel888 and CHL1 cells following staining with fluorescently-labeled HLA-A,B,C-specific antibody. (F) Quantification of confocal imaging, with MHC-I surface expression expressed as mean pixel counts for at least 40 imaged cells within each treatment group. All experiments were performed at least 4 times with similar results. **, p<0.01; ns, not significant.
To determine whether this modulation of MHC-I surface expression was physiologically relevant for melanoma, we initiated a series of MAPK pathway inhibitor studies in melanoma cell lines harboring either V600E-mutated or wild-type (WT) BRAF. Following MAPK pathway inhibition (Supplemental Figure 1), CHL1 melanoma cells bearing WT BRAF showed no detectable alterations in MHC-I expression with either the BRAF(V600E)-specific inhibitor dabrafenib (BRAFi) or the MEK inhibitor trametinib (MEKi) (Figure 1C). By contrast, BRAFi or MEKi treatment of two V600E-positive melanoma cell lines (Mel888 and WM793) significantly increased total cell surface MHC-I (Fig. 1C). This upregulation was specific for MHC-I, as expression of other melanoma cell surface molecules HLA-DR, PD-L1, and MSCP was unaffected by drug treatment (Supplemental Fig. 2). Confocal microscopy analysis of inhibitor-treated cells confirmed these findings (Figures 1D and E).
The extent of MHC-I upregulation observed varied depending on the experiment, cell line, and dose of inhibitor used, but typically ranged from ~70 – 120%. MAPK pathway inhibition was previously shown to increase MHC-I expression after 24 – 48 hours treatment with BRAFi or MEKi, which was attributed to an increase in transcription of HLA genes in addition to a number of antigen-processing components (14–16). However, we detected MHC-I upregulation within 30 minutes of inhibitor treatment, with maximal HLA-A,B,C cell surface levels occurring at 2 to 3 hours (Figure 1F). This rapid response time made transcriptional upregulation an unlikely explanation, since total cellular HLA-A,B,C protein levels remained unaltered for several hours following inhibitor treatment (data not shown). We therefore investigated for possible post-translational mechanisms that would cause the observed changes in MHC-I cell surface expression.
Modulation of MHC-I expression by the MAP kinase pathway is mediated by MHC-I cytoplasmic tail Serine-335
The ~35 amino acid cytoplasmic domain of MHC-I contains two conserved phosphorylation sites, Tyrosine-320 and Serine-335, which have both been previously linked to MHC-I trafficking in immune cells (10,12,17–20). To explore whether MAPK pathway activation modulates MHC-I melanoma cell surface expression through a cytoplasmic tail-dependent mechanism, we generated lentiviral constructs designed to express tail variants of HLA-A*0201 (A2) (13). Mel888 and WM793 melanoma cells were then transduced to express either wild-type (WT) or one of three cytoplasmic tail mutants of HLA-A2: ΔTail, containing a deletion of the entire cytoplasmic domain; Y320A or S335A, containing alanine point substitutions to the tyrosine and serine phosphorylation sites, respectively (Figure 2A).
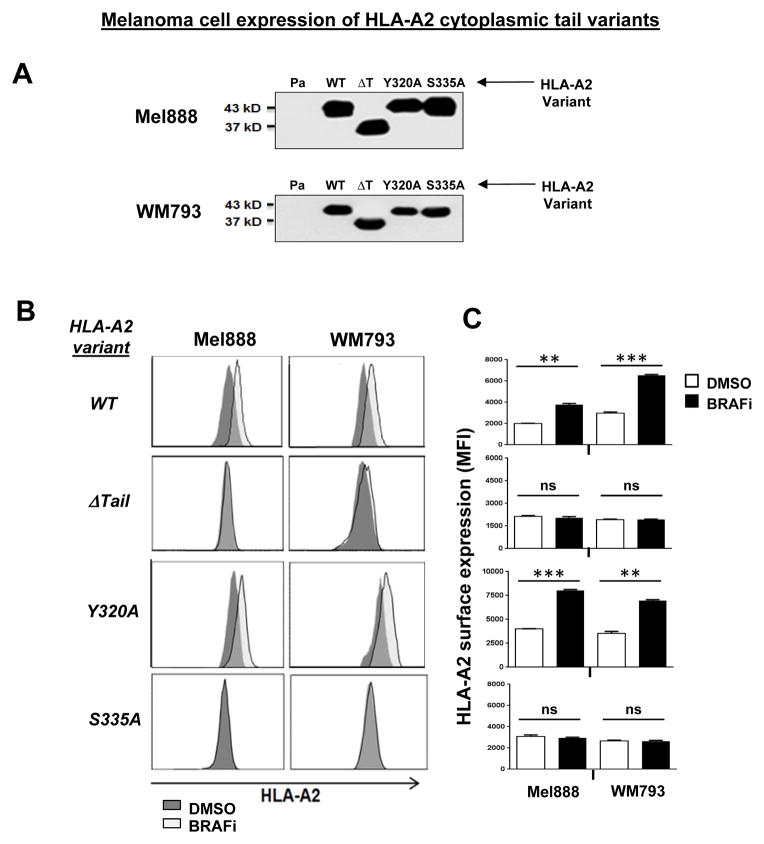
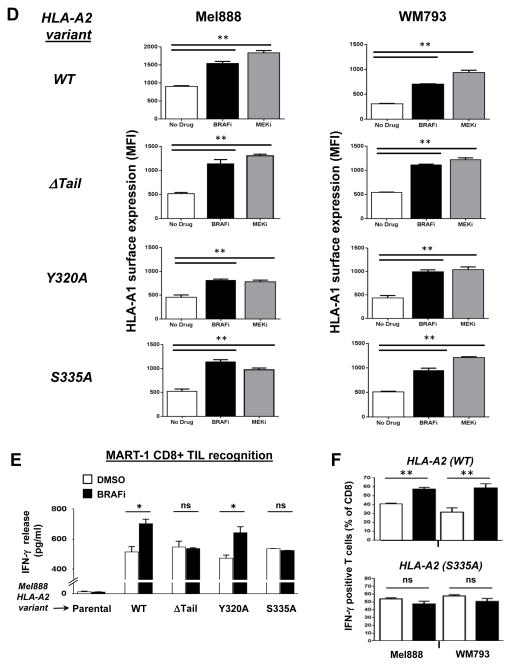
(A) Western blot showing HLA-A*0201 immunoprecipitated from Mel888 and WM793 melanoma cells lentivirally transduced to express WT HLA-A*0201 or one of three cytoplasmic tail mutants of HLA-A*0201: ΔTail (ΔT), Y320A, or S335A. (B) Transduced Mel888 and WM793 melanoma cells were treated for 3 hours with either DMSO or dabrafenib 50 nM (BRAFi), then stained for HLA-A*0201 and analyzed by flow cytometry. (C) Quantification of BRAFi-induced changes in HLA-A*0201 surface expression, as measured by flow cytometry. MFI, mean fluorescence intensity. (D) HLA-A2 transduced Mel888 or WM793 cells were treated with DMSO or MEKi for 3 hours. Following treatment, cells were stained with a fluorescently-labeled HLA-A1-specific antibody and analyzed by flow cytometry. (E) Interferon-gamma (IFNγ) release by MART-1-specific CD8+ TILs during 8 hours of co-culture with HLA-A*0201-transduced Mel888 cells pre-treated for 3 hours with DMSO or dabrafenib 50 nM, as measured by ELISA. (F) Percentage of intracellular IFNγ-positive TILs following 3 hours of co-culture with DMSO- or BRAFi-treated HLA-A*0201-transduced Mel888 cells or MART-1(27–35) peptide-pulsed WM793 cells, as measured by flow cytometry. All data shown are from representative experiments performed at least 3 times with comparable results. *, p<0.05; **, p<0.01; *** p <0.005; ns, not significant.
As expected, WT A2 surface expression rapidly increased in both transduced cell lines following MAPK pathway inhibition with either BRAFi or MEKi (Figs 2B, 2C and Supplemental Figs. 3 and 4). By contrast, cytoplasmic tail-deleted A2 showed no change in surface expression following treatment with either inhibitor. Interestingly, point mutations to the cytoplasmic tyrosine or serine phosphorylation sites of HLA-A2 showed distinct consequences: while cell surface levels of the Y320A mutant increased in response to MAPK pathway inhibition (similarly to WT A2), expression of the S335A mutant did not change. Surface expression of WT HLA-A*0101, endogenously expressed by all transduced cell lines, was monitored as a positive control for MHC-I upregulation (Figure 2D). Thus, modulation of MHC-I cell surface expression by the MAPK pathway requires the MHC-I cytoplasmic tail, and specifically the Serine-335 phosphorylation site.
To examine the consequences of MAPK pathway-induced MHC-I modulation for CD8+ T-cell recognition of tumor cells, we next initiated a series of experiments using the A2-transduced melanoma cells as target cells. HLA-A2-restricted TILs with a high level of specificity for the MART-1(27–35) peptide (21) were co-incubated with A2-transduced Mel888 cells following 3 hr treatment with either DMSO or BRAFi. ELISA results showed that interferon-gamma (IFNγ) release by TILs after 8 hours was significantly higher in the BRAFi-treated cells expressing WT A2 or Y320A, but not in the cells expressing ΔTail or S335A variants of HLA-A2 (Fig. 2E). The augmentation of IFNγ release from TILs following inhibitor treatment was comparable to that observed in parallel when T2 target cells were pulsed with a ~5-fold increase in MART-1 peptide (Supp. Fig. 5). Since MAPK inhibition has previously been shown to increase the transcription of melanoma differentiation antigens including MART-1 (3), we also utilized an intracellular IFNγ staining assay to assess TIL reactivity in response to DMSO- or BRAFi-treated Mel888 cells at earlier time points (3 hours), prior to increased MART-1 protein expression (Fig. 2E and Supp. Figs. 6 and 7). In addition, since WM793 cells do not naturally express MART-1, we also measured TIL reactivity in response to A2-transduced WM793 cells pulsed with MART-1 peptide following DMSO or BRAFi treatment (Fig. 2F and Supp. Fig. 6). These data were consistent with those from earlier experiments, and demonstrated that MHC-I upregulation resulting from MAPK pathway inhibition led to significantly increased melanoma-specific CD8+ T-cell recognition and function.
BRAF(V600E) promotes rapid MHC-I internalization and intracellular sequestration that is reversible with MAP kinase pathway inhibition
We performed confocal microscopy studies to further characterize the regulation of MHC-I trafficking and intracellular localization by the MAPK pathway in melanoma cells. Melanoma cells harboring WT or V600E-mutated BRAF were surface stained with a fluorescently-labeled, HLA-A,B,C-specific monoclonal antibody (mAb). At different time points after being returned to tissue culture cells were stained with a secondary antibody conjugated to a different fluorophore (red). This protocol allowed us to readily visualize and distinguish MHC-I molecules internalized over the course of time in culture (green) from MHC-I remaining at the cell surface (yellow in merged image). BRAF(V600E)-expressing WM793 cells accumulated a significantly higher level of internalized MHC-I over 30 and 60 minutes compared to that accumulated by WT BRAF-expressing CHL1 cells (Figure 3A). Similar differences in MHC-I internalization were observed between Mel888 [BRAF(V600E)] and MeWo (BRAF WT) melanoma cell lines (data not shown).
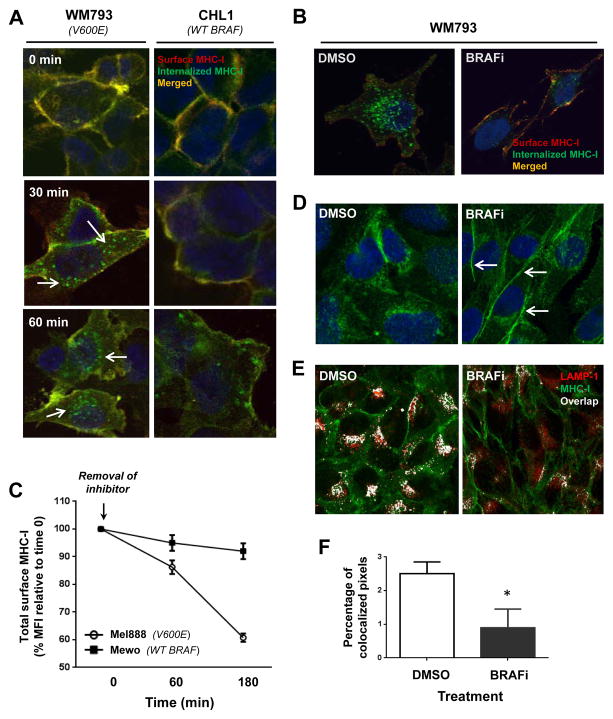
(A) WM793 and CHL1 melanoma cells were surface-labeled with Alexa488-conjugated HLA-A,B,C-specific monoclonal antibody (green, 1° label) and then cultured at 37 C. At the indicated time points, cells were stained with an Alexa568-conjugated secondary antibody (red, 2° label) to double-label the remaining cell surface MHC-I (yellow), and analyzed by confocal microscopy. White arrows indicate MHC-I (green) that was internalized during time in culture. (B) Same experiment as in (A), except WM793 cells were pre-treated with either DMSO or dabrafenib 50 nM for 2 hours prior to HLA-A,B,C surface labeling, and then cultured at 37 C for 90 minutes with continued exposure to DMSO or BRAFi. (C) Time course of MHC-I surface expression in Mel888 [BRAF(V600E)] or MeWo (BRAF WT) melanoma cells following removal of dabrafenib from cell culture medium, as measured by flow cytometry. (D) Confocal images showing cellular distribution of total HLA-A,B,C in permeabilized WM793 cells following a 2-hour treatment with DMSO or dabrafenib. White arrows indicate increased cell surface localization following BRAFi treatment. (E) Double staining of total MHC-I (green) and the endolysosomal marker LAMP-1 (red), with white color indicating overlap of the two markers. (F) Percentage of pixels showing green/red (MHC-I and LAMP-1) co-localization, quantified from at least 40 imaged cells within each treatment group. All data shown are from representative experiments performed at least 3 times with comparable results. *, p<0.05
We repeated the experiment after pre-treating the WM793 cells for 2 hours with either DMSO or BRAFi. BRAFi treatment dramatically reduced the amount of internalized MHC-I while maintaining a relatively high level of cell surface expression (Fig. 3B). Conversely, removal of BRAFi from the cell media resulted in a rapid and progressive disappearance of cell surface MHC-I in V600E-expressing Mel888 cells, but not in WT BRAF-expressing MeWo cells (Fig. 3C). The BRAFi-induced shift towards a higher surface-to-intracellular MHC-I ratio was also confirmed by permeabilizing WM793 cells prior to staining in order to visualize the steady state distribution of total cellular MHC-I (Fig. 3D).
In order to better understand the fate of internalized MHC-I molecules, we co-stained permeabilized melanoma cells for total HLA-ABC along with the endolysosomal marker LAMP-1. While control WM793 cells showed a clear co-localization of MHC-I molecules within LAMP-1-positive endocytic compartments, BRAFi-treated cells showed ~3-fold to 4-fold less co-localization of the two markers (Figs. 3E and F). To determine whether this increased endolysosomal trafficking induced by MAPK pathway activation might be associated with an increased rate of MHC-I degradation, we performed an 35S-methionine pulse-chase experiment designed to assess the molecular half-life of MHC-I molecules. Neither BRAFi nor MEKi treatment of melanoma cells had any measurable effect on MHC-I half-life (Supp. Fig. 8). Taken together, the results support that BRAF(V600E) and MAPK pathway activation do not directly promote MHC-I degradation, but instead regulate the trafficking and cellular localization of MHC-I in melanoma cells. Thus, pathway activation leads to a significant reduction in tumor antigen-specific CD8+ T-cell recognition, thereby potentially further promoting tumor cell escape from immune-mediated cell killing.
DISCUSSION
MHC-I-mediated antigen presentation by cancer cells constitutes a central focus of antitumor CD8+ T-cell responses. Herein, we report a novel mechanism of oncogene-induced immunomodulation in which oncogenic BRAF(V600E) mutant proteins promote cellular redistribution of MHC-I molecules in melanoma cells. Our data support a model in which the BRAF(V600E) mutation drives rapid and constitutive internalization of MHC-I from the cell surface and subsequent sequestration within endocytic compartments, as depicted in Figure 4. Inhibition of BRAF(V600E) or MEK in human melanoma cells with a BRAF(V600E) mutation decreases MHC-I internalization, resulting in less endolysosomal sequestration and increased surface expression, which in turn augments CD8+ T-cell recognition of tumor cells.
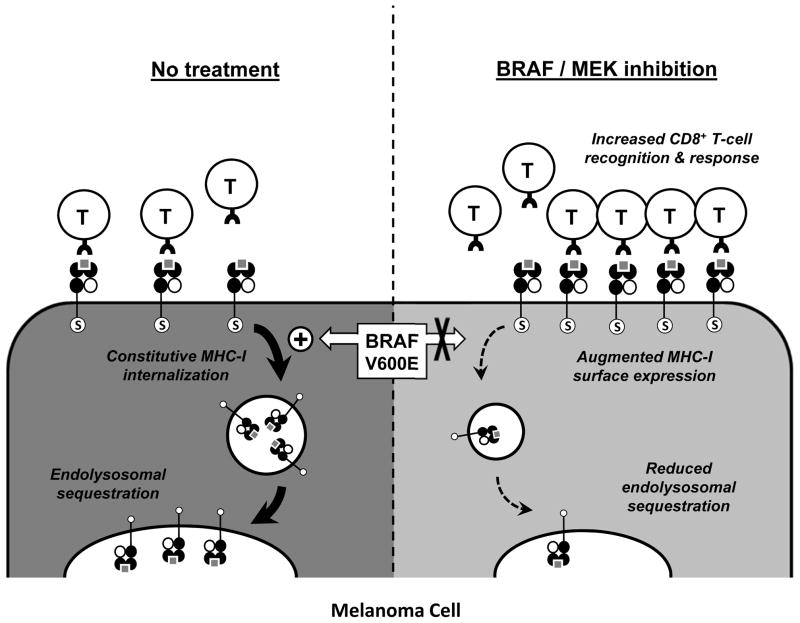
Oncogenic activating BRAF mutations (e.g., V600E) in melanoma cells drive the rapid and constitutive internalization of MHC-I molecules from the cell surface and their subsequent sequestration within LAMP1-positive endolysosomal compartments (left). This redistribution process requires the conserved serine-335 phosphorylation site (S) found within the cytoplasmic tail of MHC-I. Pharmaceutical inhibition of MAPK signaling can inhibit MHC-I internalization, thus altering the equilibrium and resulting in reduced endolysosomal sequestration and enhanced MHC-I surface expression within hours (right). This augmented MHC-I surface expression in turn leads to increased tumor cell recognition and function of melanoma antigen-specific CD8+ T cells.
MAPK pathway inhibition has previously been reported to drive increased expression of MHC-I, in addition to that of melanoma differentiation antigens such as MART-1 and gp100 (3,13–15). However, these changes were shown to take place over days of inhibitor treatment and relied largely on transcriptional upregulation. By contrast, the changes in MHC-I expression we observed occurred within 1 to 2 hours of drug treatment, precluding a transcriptional explanation. This short time of onset for the effects is more suggestive of a post-translational mechanism (22), and our data support that phosphorylation of Serine-335, but not Tyrosine-320, on the MHC-I cytoplasmic domain was required for this MAPK pathway-mediated effect.
Deletion of the MHC-I tail region that includes Serine-335 has previously been shown to abrogate the rapid internalization and recycling of MHC-I that occurs in lymphocytes upon activation (9,10). This region has also been shown to control the rate of surface MHC-I internalization in dendritic cells (DC), in which recycling of MHC-I is known to play a critical role in antigen cross-presentation (12,19,20,23). Moreover, the MHC-I cytoplasmic tail has been shown to mediate surface clustering of MHC-I, which can significantly impact CD8+ T-cell recognition (24). Interestingly, while Tyrosine-320 was required for transit of MHC-I into LAMP-1-positive endocytic compartments of DCs (12,19), these studies suggest that Serine-335 may play a more dominant role in controlling endocytic trafficking in melanoma cells. Although MHC-I phosphorylation has been associated with intracellular trafficking MHC-I in lymphoblastoid cell lines (25), it remains to be determined whether the same mechanism plays a role in tumor cells and which kinases and/or phosphatases might mediate this process. Studies are currently ongoing to address this question, in addition to examining whether MAPK pathway inhibition can induce qualitative shifts in the global immunopeptidome, which could have critical consequences for antitumor T-cell responses.
While many questions remain, the results presented here are in accordance with and add to a number of recent studies demonstrating that the oncogenic BRAF(V600E) mutation, which is a frequent event in melanoma and also detected in several other cancer types, promotes immune suppression in cancer through multiple mechanisms (2–7,26). Intracellular sequestration or degradation of MHC-I through targeting the cytoplasmic tail is a common immune evasion strategy used by viruses (27–29), so it is perhaps not surprising that some tumors have evolved similar mechanisms to avoid T-cell recognition (30). These findings have potentially important clinical implications for cancer patients, and support the emerging notion that oncogene-targeted agents will show therapeutic synergy when used in combination with immune-based therapies.
Acknowledgments
This work was funded by the following grants: National Institute of Allergic and Infectious Diseases (NIAID) grant R01-AI085371, Cancer Prevention and Research Institute of Texas (CPRIT) grant RP110248, and Melanoma SPORE grant CA093459. Thanks also to Drs. Willem Overwijk and Lisa Boulanger for critical reading of the manuscript.
References
Full text links
Read article at publisher's site: https://doi.org/10.1158/2326-6066.cir-15-0030
Read article for free, from open access legal sources, via Unpaywall:
http://cancerimmunolres.aacrjournals.org/content/canimm/3/6/602.full.pdf
Citations & impact
Impact metrics
Citations of article over time
Alternative metrics
Article citations
Machine learning and single-cell RNA sequencing reveal relationship between intratumor CD8<sup>+</sup> T cells and uveal melanoma metastasis.
Cancer Cell Int, 24(1):359, 30 Oct 2024
Cited by: 0 articles | PMID: 39478575 | PMCID: PMC11523669
Immunotherapy for pediatric low-grade gliomas.
Childs Nerv Syst, 40(10):3263-3275, 17 Jun 2024
Cited by: 0 articles | PMID: 38884777
Review
It's high-time to re-evaluate the value of induced-chemotherapy for reinforcing immunotherapy in colorectal cancer.
Front Immunol, 14:1241208, 18 Oct 2023
Cited by: 0 articles | PMID: 37920463 | PMCID: PMC10619163
Review Free full text in Europe PMC
Enhanced Vaccine Immunogenicity Enabled by Targeted Cytosolic Delivery of Tumor Antigens into Dendritic Cells.
ACS Cent Sci, 9(9):1835-1845, 14 Sep 2023
Cited by: 0 articles | PMID: 37780364 | PMCID: PMC10540291
BRAF Mutations in Melanoma: Biological Aspects, Therapeutic Implications, and Circulating Biomarkers.
Cancers (Basel), 15(16):4026, 08 Aug 2023
Cited by: 16 articles | PMID: 37627054 | PMCID: PMC10452867
Review Free full text in Europe PMC
Go to all (98) article citations
Data
Data behind the article
This data has been text mined from the article, or deposited into data resources.
BioStudies: supplemental material and supporting data
Similar Articles
To arrive at the top five similar articles we use a word-weighted algorithm to compare words from the Title and Abstract of each citation.
Selective BRAFV600E inhibition enhances T-cell recognition of melanoma without affecting lymphocyte function.
Cancer Res, 70(13):5213-5219, 15 Jun 2010
Cited by: 471 articles | PMID: 20551059
Coexpression of NRASQ61R and BRAFV600E in human melanoma cells activates senescence and increases susceptibility to cell-mediated cytotoxicity.
Cancer Res, 66(13):6503-6511, 01 Jul 2006
Cited by: 63 articles | PMID: 16818621
KIT Suppresses BRAFV600E-Mutant Melanoma by Attenuating Oncogenic RAS/MAPK Signaling.
Cancer Res, 77(21):5820-5830, 25 Sep 2017
Cited by: 12 articles | PMID: 28947418 | PMCID: PMC5679278
Endocytic Recycling of MHC Class I Molecules in Non-professional Antigen Presenting and Dendritic Cells.
Front Immunol, 9:3098, 07 Jan 2019
Cited by: 39 articles | PMID: 30666258 | PMCID: PMC6330327
Review Free full text in Europe PMC
Funding
Funders who supported this work.
NCATS NIH HHS (1)
Grant ID: UL1 TR000371
NCI NIH HHS (2)
Grant ID: CA093459
Grant ID: P50 CA093459
NIAID NIH HHS (2)
Grant ID: R01 AI085371
Grant ID: R01-AI085371