Abstract
Free full text

Transfer of regulatory T cells into abortion-prone mice promotes the expansion of uterine mast cells and normalizes early pregnancy angiogenesis
Abstract
Implantation of the fertilized egg depends on the coordinated interplay of cells and molecules that prepare the uterus for this important event. In particular, regulatory T cells (Tregs) are key regulators as their ablation hinders implantation by rendering the uterus hostile for the embryo. In addition, the adoptive transfer of Tregs can avoid early abortion in mouse models. However, it is still not defined which mechanisms underlie Treg function during this early period. Cells of the innate immune system have been reported to support implantation, in part by promoting angiogenesis. In particular, uterine mast cells (uMCs) emerge as novel players at the fetal-maternal interface. Here, we studied whether the positive action of Tregs is based on the expansion of uMCs and the promotion of angiogenesis. We observed that abortion-prone mice have insufficient numbers of uMCs that could be corrected by the adoptive transfer of Tregs. This in turn positively influenced the remodeling of spiral arteries and placenta development as well as the levels of soluble fms-like tyrosine kinase 1 (sFlt-1). Our data suggest an interplay between Tregs and uMCs that is relevant for the changes required at the feto-maternal interface for the normal development of pregnancy.
After the egg is fertilized, the uterus prepares for implantation. Genetic factors are relevant for the quality and viability of the blastocyst; however complex interactions between the immune system and the endocrine system are necessary for an adequate environment where the embryo is able to grow. For implantation to be successful, it is necessary to have a healthy uterine milieu that allows the invasion of the blastocyst and the rapid growth of the placenta while supporting the transformation of uterine into decidual cells. This is mediated and facilitated by immune cell populations, the cytokines they secrete and by hormonal changes. Innate immune cells of importance for implantation are present already before pregnancy. They usually present a unique phenotype that greatly differs from the phenotype of their counterparts located in the periphery or in other tissues. Among the innate immune cells, the most intensively studied are the uterine natural killer cells (uNKs)1. They contribute to angiogenesis and to the remodeling of spiral arteries (SAs)1,2. Uterine dendritic cells (uDCs) are present in high numbers at specific sites along the non-pregnant uterus as shown in vivo3 and are important for implantation4,5. Uterine mast cells (uMCs) also differ enormously from MCs found in other tissues and are involved in implantation6,7. Especially MC-associated proteases (Mcpt), including tryptases and chymases, are involved in uMC function8. Uterine macrophages directly communicate with trophoblasts in a bidirectional way9. They are in charge of maintaining the luteal microvascular network that is necessary for the integrity of the corpus luteum and progesterone production10. A recently described population of second-trimester decidual neutrophils shows a unique phenotype and possesses angiogenic properties11. From this information, a picture emerges, where cells of the innate immune system are responsible for the changes necessary to support pregnancy, namely the promotion of angiogenesis and tissue remodeling. An imbalance in cell number or abnormal distribution can cause suboptimal angiogenesis, which is often related to pregnancy complications. Elevated levels of the anti-angiogenic factor placental soluble fms-like tyrosine kinase 1 (sFlt-1) has been linked to endothelial dysfunction that in turn causes hypertension and proteinuria in preeclampsia12. Angiotensin Receptor II (AT2) seems to be of relevance as well as it is involved in cardiovascular functions during pregnancy13.
Already at copulation, the female´s immune system gets in contact with male antigens present in sperm; animal studies as well as studies performed with samples from patients immediately after coitus indicate that seminal fluid rapidly attracts cells from the innate and adaptive immune system14,15. Thus, the maternal immune system is aware very early of the presence of foreign, paternal antigens. Animals lacking T and B cells can get pregnant and have progeny16, so that at the first glance it could be inferred that adaptive immune cells are not absolutely necessary for pregnancy. Studies in the last years, however, have shown that a small subtype of adaptive CD4+ T cells is necessary for implantation. The depletion of CD4+Foxp3+ regulatory T cells (Tregs) before mating drastically influences the uterine environment and hampers the implantation of the fetus17, while depletion at later time points only provokes a mild augmentation in the abortion rate18. Hence, it is of central importance to understand the local mechanisms governing the implantation of the embryo. Here, we used a well-established model in which disturbed pregnancy tolerance can be restored by the addition of Tregs before implantation19. With this model, we aimed to elucidate whether Treg transfer activates uMCs and positively influences angiogenesis. Various studies suggest a direct interaction between MCs and Tregs. It could be demonstrated that MCs are critical in Treg-mediated allograft tolerance and that Tregs activate and recruit MCs via IL-920,21. The suppression of MC degranulation by Tregs can dampen allergic MC responses, and in vivo depletion of Tregs provokes MC-related anaphylactic symptoms22,23. Furthermore, MC-derived IL-2 supports Treg-mediated suppression during allergic dermatitis24 and MC-derived transforming growth factor (TGF-) β is able to induce the conversion of naïve T cells into Tregs in vitro25. We are particulary interested in the interplay between Tregs and MCs as MCs are not only present in the female reproductive tract26,27,28 but also migrate from the periphery to the uterus upon female hormone augmentation29. When pregnancy occurs, uMC numbers further increase and remain high during early gestation6. uMCs have a positive influence on placentation and the remodeling of SAs as well as on placenta size and fetal growth. MCs are multifaceted cells that interact with other immune and non-immune cells. Whether Treg/MCs communication or interplay is of relevance for pregnancy establishment and maintenance was not explored and is the main aim of this study.
Results
uMC numbers peak during sexual receptivity and remain high if pregnancy takes place in normal but not in abortion-prone mice
We first quantified the numbers of MCs in Toluidine blue-stained slides of uteri from CBA/J mice and observed an oscillation in uMC numbers at the different phases of the estrous cycle (Fig. 1a). The number of uMCs peaked at estrus, which represents the period of sexual receptivity. We next studied the mRNA transcripts for molecules that are stored in MC granula and secreted upon activation. mRNA levels of the Mcpt-1, Mcpt-6 and Carboxypeptidase A3 (Cpa3) were significantly elevated at the metestrus phase (Fig. 1b–d). This is interesting as this phase follows the estrus, showing that MC-related proteases augment immediately after the peak of MC numbers, which suggests their local activation followed by the secretion of mediators upon degranulation. This in turn coincides with the time point at which the uterus undergoes degenerative modifications.
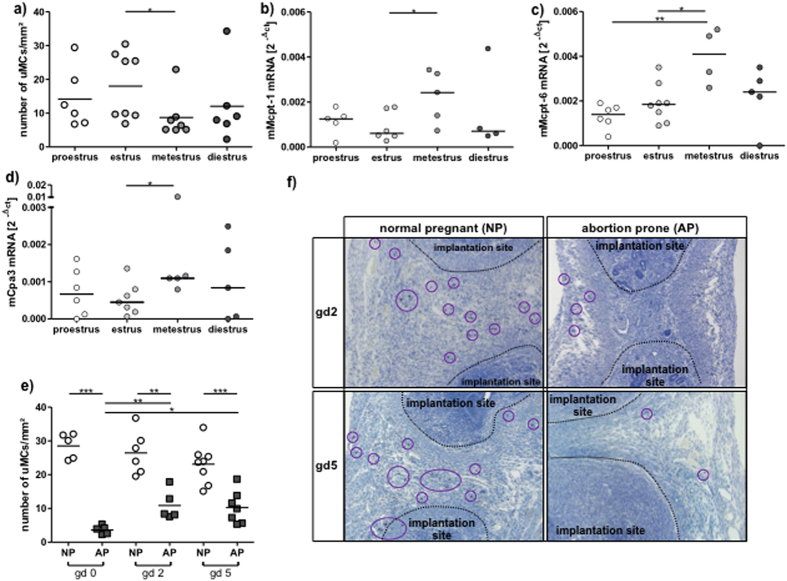
Abortion-prone mice have diminished uMC numbers. (a) Number of uMCs
per 1mm2 was quantified in Tolouidine blue stained
paraffin sections (5
μm) from uteri of virgin CBA/J females
(n
=
6–8) during different phases of the estrous
cycle. The mRNA levels of MC-related proteases Mcpt-1 (b), Mcpt-6
(c) and Cpa3 (d) were measured in uterine tissue of virgin
CBA females at proestrus, estrus, metestrus or diestrus. (e) uMCs
number per 1
mm2 was quantified in uterine paraffin
sections (5 μm) of BALB/c-paired CBA/J females (normal pregnant, NP)
or DBA/2-mated CBA/J females (abortion-prone, AP) at gestational days (gd)
0, 2 and 5 of pregnancy. (f) During pregnancy MCs are localized in
the decidua between the implantation sites. MCs (magnification ×100)
were visualized after Toluidine blue O staining (0.1%) at days 2 and 5 of
pregnancy in NP and AP mice and are indicated by circles. For
(a,e) the results are presented as single values with
means. Statistical differences were obtained using unpaired t- test
(*p
<
0.054, **p
<
0.005,
***p
<
0.001). For (b–d) the
results are presented as individual values plus medians. Statistical
differences were obtained using non-parametric Mann-Whitney-U- test
(*p
<
0.054, **p
<
0.005).
We next studied the localization and numbers of uMCs during early pregnancy. For that, we analyzed samples from BALB/c-mated CBA/J females known to have normal pregnancies (NP) and from DBA/2J-mated CBA/J females that have reportedly augmented abortion rates (abortion-prone group, AP) due to impaired feto-maternal tolerance19. We did so at days 0, 2 and 5 of pregnancy. We observed that in NP females at all three time points, uMC numbers were even higher than at estrus (Fig. 1a,e). Astonishingly, this was not the case for females from the AP group whose uMC levels were comparable to the ones observed during metestrus (Fig. 1a,e). As for their localization, uMCs were located in close proximity to the developing embryo as shown in Fig. 1f.
Adoptive transfer of Tregs diminishes the occurrence of fetal death, which is associated with an augmentation of uMCs
We next employed a well-characterized strategy to rescue fetuses from abortion in
the CBA/J×
DBA/2J combination, which consists in
adoptively transferring antigen-specific Tregs immediately after plug
detection19,30,31. We confirmed that Treg-transferred
females have fewer abortions than the PBS-treated controls and this was
comparable with the abortion rate observed in NP animals (Fig.
2a,b). This was associated with augmented
CD4+Foxp3+ Treg numbers in decidua (Fig. 2c), but also in thymus (Fig. 2d)
and spleen (Fig. 2e). To evaluate whether Treg transfer
has an impact on MC numbers, we studied reproductive tissues as well as the
spleen and lymph nodes. The adoptive transfer of Tregs was associated with an
augmentation in the proportion of
CD117+FcεRIα+ double positive
MCs cells in the decidua, the maternal part of the fetal-maternal interface
(Fig. 2f), in the oviduct (Fig.
2g) and in splenic tissue (Fig. 2h) compared to
AP mice that were sham-treated. Interestingly, the Treg transfer normalized MC
numbers to the levels observed in NP individuals (Fig.
2f–h). In lymph nodes, the MC number remained unchanged (data
not shown). Accordingly to the elevated MC numbers, Mcpt-1
mRNA levels
in the decidua of AP mice increased after Treg transfer (Fig.
2i). IL-9
mRNA was almost indetectable at the feto-maternal
interface but IL-3 and mSCF mRNA were both augmented after Treg transfer (Suppl. Fig. 1). As both factors were
proposed to be growth factors for mast cells, it is possible that Treg directly
or indirectly augment the levels of both, finally resulting in augmented numbers
of uMCs in situ. Blockage of IL-10, identified as the main Treg mediator
in this particular model30, not only abrogated the protective
effect of Tregs (see material and methods) but also hindered Mcpt-1
mRNA
levels augmentation (Suppl. Fig. 2).
As isolation and further culture of uMCs is technically challenging, a final
confirmation of functional changes in uMCs remains open, but the augmentation of
Mcpt-1 after Treg transfer and its lack of augmentation when the main Treg
mediator was blocked strongly suggest that Treg transfer influences not only MC
numbers but also their activity.

(a) Fetal death rates were determined at day 12 of pregnancy in normal
pregnant (NP, n=
9), abortion-prone (AP,
n
=
9) and Treg-treated abortion-prone (AP+Treg,
n
=
9) animals. (b) Representative pictures of uteri
of NP, AP and AP
+
Treg are shown. Arrows indicates
abortions. The percentage of CD4+Foxp3+
positive cells in (c) decidua (d) thymus and (e) spleen
as well as the percentage of
CD117+FcεRα+ positive
cells in (f) decidua, (g) oviduct and (h) spleen was
analyzed by flow cytometry at day 12 of pregnancy. (i)
Mcpt-1
mRNA levels were analyzed by real time PCR and the data
expressed as 2−ΔcT. Data are shown as
single values with medians. Statistically significant values are indicated
as follows: *p
<
0.054, **p
<
0.005,
***p
<
0.001 (Mann Whitney-U test).
Placental development and SA remodeling are impaired in abortion-prone mice and restored by the transfer of Tregs
Next, we studied the placentation and SA remodeling in abortion-prone females. Because MC numbers directly correlate with the remodeling of SAs6, we asked whether Treg transfer into abortion-prone females could improve placentation and pregnancy outcome via the upregulation of MC numbers. Placental surface areas were decreased in AP mice, where both Tregs and MCs were present in lower numbers as in NP mice (Fig. 3a,b). This was accompanied by insufficiently remodeled SAs, characterized by an increased wall thickness (Fig. 3c) that resulted in abnormal wall to lumen ratios (Fig. 3d) when compared with NP mice. AP mice showed high SA wall expression of smooth muscle actin and coherently arranged multilayered smooth muscle cells, signs of impaired SA remodeling (Fig. 3e). Adoptive Treg transfer into AP mice completely normalized the shaping of the SAs and resulted in normal placental surface areas; both were comparable to those observed in NP mice (Fig. 3a–e).

Normal pregnant (NP, n=
5), abortion-prone (AP,
n
=
6) and abortion-prone animals transferred with Tregs
(AP
+
Treg, n
=
7) were sacrificed at day 12
of pregnancy. 5
μm cross sections of paraffin embeeded whole
implantation sites (WIS) were stained with H/E. (a) Placental surface
areas were measured in WIS from NP, AP and AP
+
Treg mice.
The results are presented as single values with medians. 1 placenta was
measured for each female and the median was calculated for the group.
Statistical differences were obtained using non-parametric
Mann-Whitney-U- test (*p
<
0.054). (b)
Representative pictures (magnification ×
10) of
Hematoxylin/Eosin stained sections from NP, AP and AP
+
Treg
mice are shown. Broken lines indicate the placenta areas. Spiral artery (SA)
wall and lumen diameters of 2 to 10
SAs per animal of NP
(n
=
5), AP (n
=
6) and
AP
+
Treg (n
=
7) were measured and
calculated as (c) wall thickness and (d) wall/lumen ratio.
Data are expressed as mean
±
SEM and their
statistical significance was analyzed by unpaired t-test
(*p
<
0.054). Representative images of H/E-stained
sections (magnification ×400) and immunofluorescence for smooth
muscle actin and smooth muscle actin
+
DAPI (magnification
×1000) in SA of NP, AP and AP
+
Treg mice are
depicted in (e) (scale
bar
=
20
μm).
Abortion-prone mice show enhanced expression of placental sFlt-1 and AT2, which is reduced by Treg transfer
We next studied the sFlt-1 and AT2 expression in placental tissue of AP mice that presented diminished numbers of Tregs and MCs (Fig. 2c–h), smaller placental areas and impaired SA modification (Fig. 3a–e). In placentas from AP mice sFlt-1 was significantly higher expressed when compared to NP mice at pregnancy day 12 (Fig. 4a–c). The adoptive transfer of Tregs, which leads to more uMCs, normalized sFlt-1 expression (Fig. 4a–c). The same was observed for AT2 (Fig. 4d,e).
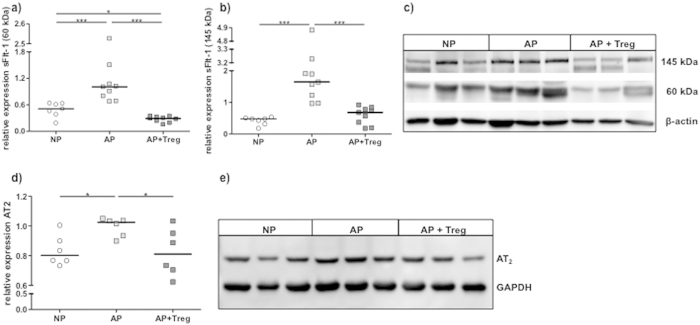
The protein levels of the two pregnancy-relevant isoforms of soluble Flt-1
(a) (60kDa) and (b) (145
kDa) as well as
(d) angiotensin receptor II (AT2) were analyzed in placental
tissue of normal pregnant (NP, n
=
7), abortion-prone (AP,
n
=
9) and abortion-prone animals treated with Tregs
(AP+Treg, n
=
9) by Western blot and expressed as arbitrary
units. The data are expressed as medians and analysed by Mann-
Whitney-U test: p
<
0.054,
**p
<
0.005,
***p
<
0.001(Mann-Whitney-U test). (c)
Representative blots for soluble Flt-1 and (e) AT2 are shown together
with the housekeeping genes beta actin (for sFlt-1) and GAPDH (for AT2).
Discussion
It is widely accepted that major changes at cellular and molecular levels take place in the uterus immediately after fecundation. These dramatic changes allow implantation and support the embryo development and growth. Mouse models are of importance as they can be employed to study the effect of a particular cell type or of cell-cell interactions. Here, we are particularly interested in the possible interaction between Tregs and MCs as both are of pivotal importance for pregnancy albeit their main functions differ greatly. To the best of our knowledge, no studies addressed the possible interaction or interdependence between MCs and Tregs during pregnancy.
Our findings demonstrate that the adoptive transfer of Tregs during early pregnancy influences MCs and this in turn is related to improved SA remodeling and increased placenta size. Interestingly, low levels of Tregs have previously been reported in AP vs NP mice during early pregnancy19,30,31,32,33. Our results confirm and extend these reports as we also found low numbers of CD4+Foxp3+ Tregs in AP mice in different immune-related organs as well as in decidua.
Thus, low Treg numbers correlate with low uMC numbers in animals whose pregnancies do
not progress optimally. In AP animals, not only a higher fetal death rate could be
observed but also a defective placentation. This could explain why Girardi and
colleagues observed intrauterine growth restriction (IUGR) in fetuses from this
combination34. We next transferred Tregs into AP mice. Successful
transfer of Tregs was confirmed by detection of significant upregulation of
CD4+FoxP3+ cells in organs from
AP+
Treg animals in comparison to the AP group. Decidual Treg
levels were also augmented. As expected19, Treg transfer resulted in
a reduction of the fetal death rate. When analyzing surviving fetuses and placentas,
we observed that Treg transfer was able to normalize both, placenta size and the
shaping of SAs. Interestingly, Treg transfer into AP mice was accompanied by a
simultaneous increase of MC numbers in particular of uMCs as well as increased
transcript levels of MC-specific protease 1. This strongly suggests a Treg-induced
upregulation and activation of MCs at the feto-maternal interface. Furthermore, the
blockage of IL-10, the major Treg mediator in this model30, hindered
Mcpt1 augmentation. Evidence of possible Treg-MC interaction can be found in
different models in the literature. For example, Lu and colleagues postulated in
2006 that MCs are essential intermediaries in Treg-dependent tolerance, where IL-9
represents the functional link between the two immune cell populations20. This is in agreement with findings reported by Eller and colleagues
that suggest that IL-9 production by Tregs recruits and activates MCs35. The augmentation in MC numbers after transfer of Tregs in AP mice was not linked
to increased IL-9 at the feto-maternal interface. In our model the transfer of Treg
was rather associated with an increase of IL-3 and mSCF at the feto-maternal
interface. As both are proposed as MC growth factors36,37,38, it is
tempting to speculate that Treg act by augmenting these two mediators. In the
subsequent studies, we will concentrate on dissecting the factors that are involved
in the interaction between these two cell types; this is currently not possible due
to the technical limitations.
A crucial event in fetal development is a correct placentation. Defects in
placentation can cause miscarriage or pregnancy diseases like pre-eclampsia or
IUGR39. Here, we found that placentas from DBA/2J-mated CBA/J
females are decreased in size as compared to NP BALB/c-mated CBA/J females. This is
in agreement with reports claiming that the CBA/J×
DBA/2J
model represents a valid IUGR model as fetuses grow slower in this combination
compared to the CBA/J
×
BALB/c controls34,40. Our data show that Treg transfer was able to normalize placenta size. This in
turn correlated with an augmentation of the numbers of uMCs. Several studies have
linked MCs with placentation6,41,42,43 but as of yet no studies
have shown that Tregs are relevant in this context. In contrast, several previous
reports indicate that Tregs are relevant for implantation17,44,45
and for maintaining immune tolerance30,46. Our results suggest that
Tregs promote the expansion of uMCs, which in turn positively influences
placentation.
To ensure an adequate supply of the fetus with nutrients and oxygen, enough maternal blood has to flow to the fetal side. Here, SAs overtake a central role; as pregnancy advances they change their shape to turn into thin-walled venous-like vessels. This process is called SA remodeling and is induced by the selective reduction of the SM layer11. Insufficient remodeling is linked to pre-eclampsia, fetal growth restriction, miscarriage and preterm birth2,47,48,49. We observed insufficiently remodeled SAs in AP mice; this is indicated by an augmented wall thickness and an increased wall-to-lumen ratio when compared to the NP mice. Immunohistochemically, we found a thicker SM actin layer that indicates a poor apoptosis of this layer. Interestingly, the transfer of Tregs, associated with uMC expansion could normalize both wall thickness and wall to lumen ratio. The SM actin layer was comparable to NP mice. Usually, uMCs are located close to vessels6 as we could confirm here. Functionally, uMCs are able to modulate a proper SA remodeling as we reported for MC-deficient KitW-sh/W-sh animals that were reconstituted with MCs6. It was reported that animals depleted of Tregs during pregnancy showed insufficiently remodeled SAs18. Whether this was a sole effect of Treg absence or depended on other cells was not further explored in the mentioned study. We propose that the transfer of Tregs into AP mice promotes the expansion of uMCs and they in turn promote the remodeling of SAs.
A successful pregnancy depends on a well-balanced angiogenesis/vasculogenesis system. This is mediated by a broad array of angiogenic factors including the interaction between vascular endothelial growth factor (VEGF) and its receptor VEGFR. Elevated levels of the soluble form of VEGFR, namely sFlt-1, are associated with pregnancy complications including pre-eclampsia12. This is due to the fact that sFlt-1 inhibits the mandatory VEGF response by forming a complex with VEGF in the circulation50. In the presence of elevated sFlt-1, the positive angiogenic influence of VEGF at the fetal-maternal interface on placental development and SA formation is impaired. Here, we showed that AP mice, which have impaired placentation and insufficient SA remodeling, exhibit significant elevated sFlt-1 levels. In line with this, a study from 2006 demonstrated significantly decreased free VEGF levels in AP mice in contrast to NP mice34. The same could be shown for sFlt-140. The adoptive transfer of Tregs and the subsequent upregulation of MCs at the feto-maternal interface are associated with downregulated levels of sFlt-1; this may explain why placentation is restored, SAs properly remodeled, and fewer fetuses died.
The renin-angiotensin-aldosterone pathway is one of the most important blood
pressure-regulating systems of the body. Angiotensin (Ang) II is generated from AngI
through the activity of angiotensin-converting enzyme (ACE) and involved in vascular
adaptions and diseases51. Several studies have demonstrated that MC
chymase is able to generate AngII from AngI independently of ACE52,53. Girardi and colleges reported that AP mice show a higher sensitivity to AngII as
AngII treatment in DBA/2J-mated CBA/J females lead to a significant change in blood
pressure. Without application of AngII there were no differences between the groups.
These observations suggest that the local systems of the AP model may serve to
increase the effects of circulating AngII by upregulating the AngII receptor34. We found that AP mice present upregulated levels of AngII receptor.
Transfer of Treg and increase of uMCs was related with downregulation of AngII
receptor to the levels observed in NP mice, suggesting that Treg or uMCs secrete
molecules that control the expression of the receptor. Upregulation of the AngII
receptor at the fetal-maternal interface of pregnant females from the
CBA/J×
DBA/2J combination may maintain the signal strength
of AngII – AngII receptor as compensation for lower AngII concentrations due
to decreased MC levels. The chymase AngII generating system is different from the
ACE-dependent AngII generating system. Tissue originated renin generates
Angiotensinogen I from Angiotensinogen. Generation of AngII from AngI depends on
chymase, which is secreted from the granules of MCs. Chymase-dependent AngII does
influence tissue remodeling; it is however not involved in the regulation of
hypertension54. This would explain the insufficient remodeling of
SAs in AP mice in the absence of elevated systemic blood pressure. In other models,
in which SA remodeling is rather controlled by uNKs, the insufficient remodeling of
SA is directly related with maternal hypertension2.
The data obtained in our study unravel a so far unsuspected cellular interaction that contributes to pregnancy maintenance. We show that the adoptive transfer of Tregs to animals that reject their fetuses is accompanied by an augmentation of the number of uMCs while having positive effects on placentation and the remodeling of SAs, all together leading to healthy fetuses. The mechanisms behind the positive effect of Treg and MC crosstalk at the fetal maternal interface include the maintenance of angiogenesis balance, especially the normalization of sFlt-1 as well as the modulation of receptors for angiotensin. Our study reveals a further aspect of Treg action during pregnancy and highlights the interaction between the innate and adaptive immune system with the final goal of supporting pregnancy and fetal growth.
Materials and Methods
Animals
The present study was approved by the German Ministry (203.h-42502-2-868 Magdeburg, Landesverwaltungsamt, Halle) and carried out according to institutional guidelines and the Guide for the Care and Use of Agricultural Animals in Agricultural Research and Teaching, USA.
The stage of the estrous cycle of sexually mature CBA/J females was determined based on the typical cell content of the fresh vaginal lavage with 0.9% sodium chloride. Females were sacrificed when the stage was clearly defined. To confirm the stage, samples were stained with hematoxylin/eosin (H/E) and analyzed by light microscopy (Axio Observer.A1, Zeiss; magnification ×200).
Female CBA/J (H2k), male DBA/2J (H2d) and male
BALB/c (H2d) mice were purchased from Charles River (Germany) and
Janvier (France). Animals were maintained in the institute’s own barrier
animal facility with a light/dark cycle of 12/12h. Female CBA/J mice
were mated either with BALB/c males (normal pregnant, NP) or DBA/2J males
(abortion-prone, AP). Successful mating was confirmed by the detection of a
vaginal plug. The day of plug detection was considered as day 0 of pregnancy and
females were separated from the males. CBA/J females were sacrificed on days 0,
2, 5 or 12 of pregnancy. The number of animals included in each group and of
samples used for each analysis is depicted in the Figure legends.
Isolation and Transfer of Tregs
Tregs were isolated from the spleens and lymph nodes (inguinal, para-aortic) of
normal pregnant females on day 14 of pregnancy as described elsewhere19,30. 2×
105 cells were
diluted in 200
μl PBS and injected intravenously into
DBA/2J-mated CBA/J females at day 0 of pregnancy. Control animals received PBS.
In a further experiment, we used samples from animals that have received Treg
following the same protocol and were additionally treated with 1
mg of
anti-IL-10
mAb at days 0 and 7 of pregnancy30. Controls
received either Treg alone or Treg
+
1
mg IgG30. The abortion rates in the groups was as follows: median of
abortion: 0.00 for AP
+
Treg; 10.55 for AP+IgG+Treg and 33.31
for AP
+
anti-IL-10
+
Treg.
Sample collection and histology
Uterine horns from animals at different phases of the estrous cycle, or gd 0, 2
or 5 were dissected, fixed in 96% ethanol, embedded in paraffin and cut
longitudinally into 5μm sections. For visualization of uMCs,
sections were stained with Toluidine blue dye (0.1% aqueous solution). The
number of uMCs was calculated per 1
mm2 by using an
Eyepiece-micrometer (Zeiss).
One implantation site per female was collected on day 12 of pregnancy for
paraffin embedding with previous fixation in 4% (w/v) PFA with 0.1M
sucrose (pH 7.4) for 6
h. Placental and decidual tissue were washed with
PBS, pH 7.40, snap-frozen, and kept at −80
°C until use
for RNA and protein isolation.
Quantitative histological measurements
The measurements of placental surface areas were performed according to Croy and
colleagues55. Briefly, 5μm transversal cross
sections of feto-placental units at day 12 of pregnancy were stained with H/E
and the software AxioVision4 (Zeiss) was used to measure the placental surface
areas at a X10 magnification. The quantitative measurements of SAs were
performed as described elsewhere53. Shortly, the SA wall and
lumen diameters were measured and/or expressed as wall to lumen ratios and wall
thickness.
Immunofluorescence
Heat induced antigen retrieval of transversal cross-sections of feto-placental
units was carried out in 10mmol/l Tris/1mmol/l EDTA buffer (pH 6.0) for
10
min in the microwave. Incubation with anti-smooth muscle actin (Dako)
was done overnight at 4
°C followed by an AF555 conjugated
secondary antibody (Invitrogen) for 1
h at room temperature. VECTASHIELD
mounting medium containing DAPI (VECTOR laboratories) was used to counterstain
DNA.
Flow Cytometry
Tissue from spleen, thymus, lymph nodes (inguinal, mesenteric and para-aortic) and oviducts were processed as described elsewhere19. Uterine tissue was enzymatically digested using Liberase TL (Roche). Cells were stained with the following antibodies: FITC-conjugated anti-FcεRIα, PE-conjugated anti-CD117, PerCP-CyTM5.5-conjugated anti-CD4, Alexa Fluor 647-conjugated anti-Foxp3. Besides the FcεRIα antibody (eBioscience) all antibodies were purchased from Becton Dickinson (BD).
Real Time Reverse Transcriptase Polymerase Chain Reaction (RT-PCR)
Total RNA isolation from frozen decidual tissue was performed by using Trizol
(Gibco) and a homogenizer (Ultra Turrax T8). The RNA was extracted with
chloroform, precipitated with isopropanol, washed in 80% ethanol, and finally
diluted in RNase-free water. RNA quantity and quality was determined by
ultraviolet absorbance at 260nm. Total RNA (2
μg) was
initially incubated with oligo dTs (Amersham) for 10
min at
75
°C followed by 5
min incubation on ice. Subtracted
mRNA was than incubated with dNTPs (2.5
mmol/L, Amersham), DNase I
(2
U/ml, Stratagene) and RNase inhibitor (40
U/ml, Promega) in
reaction buffer. The mixture was incubated for 30
min at
37
°C and afterwards heated for 5
min at
75
°C. Reverse transcriptase (200
U/ml, Promega) and
RNase inhibitor in RNase-free water was added to the mixture and incubated at
42
°C for 60
min. Incubation at 94
°C
for 5
min followed.
Real-time polymerase chain reaction (RT-PCR) amplifications were performed in an
iCycler (BioRad). Beta-actin or GAPDH were used as housekeeping genes.
Experiments were run in duplets. Amplification reactions were performed as
follows: initial denaturation at 95°C for 5
min
followed by 40 cycles of denaturation at 95
°C for
45
sec and annealing at either 56
°C or
60
°C for 60 sec.
SDS page, Western blot
During and after sonication placental tissue was incubated in lysis buffer (10%
NP-40, 0.1mg/ml n-Dodecil-β-D-maltoside, 500
mM sodium
fluoride, 10
mM Sodium metavanadate, 100
mM PMSF, 1M Tris,
0.5
M EDTA, 5
M NaCl) for 60
min. Afterwards cells were
centrifuged, supernatants were collected and stored overnight at
−80
°C. Protein content was determined by Bradford assay
(BioRad) as indicated by the manufacturers. Proteins (50
μg)
were resolved on a 8% or 12% SDS-PAGE at 100
V and transferred onto a
0.45
μm nitrocellulose membrane in transfer buffer containing
20%(v/v) methanol, 0.19
M glycine and 0.025
M Tris (pH 8.3) at a
constant voltage on ice. After blocking nonspecific-binding sites with 5% (w/v)
skim milk powder in TBS with 0.05% (v/v) Tween for 1
h, blots were
incubated using the following antibodies: Flt-1 (C-17, 1:250, Santa Cruz),
AT2 (H-143, 1:500, Santa Cruz), β-actin (AC-15, 1:10000,
Sigma-Aldrich), GAPDH (FL-335, 1:10000, Santa Cruz). The expression of sFlt-1
(two relevant isoforms for pregnancy) was analyzed by using the program
GeneTools (SynGene) and was referred to β-actin. The expression of
AT2 (41
kDa) was referred to GAPDH.
Data analysis and statistics
GraphPad Prism 5.0 software was used for statistical analysis. The Shapiro-Wilk
test was applied to determine whether or not the values follow a normal
distribution. Data obtained by flow cytometry and PCR among all groups were
calculated by the non-parametric Kruskal-Wallis test followed by the
Mann-Whitney-U test to calculate the difference between two
independent groups. Quantification of uterine MCs per 1 mm2 was
done by calculating the mean MC number at different areas of the uterus. Groups
were compared by the unpaired t-test. For histological measurements, two
to ten SAs per female were measured by AxioVision4 (Zeiss), the mean was
calculated, and the differences between the animal groups analyzed using
unpaired t-test; differences regarding the placental surface areas
between the groups (1 to 2 placentas/female/group) were analyzed by
Mann-Whitney-U test. For all tests, P
value<
0.054 was considered to be statistically significant.
The number of animals or samples used for a determined experiment as well as the
statistical test used and the P values obtained are indicated in each
Figure legend.
Additional Information
How to cite this article: Woidacki, K. et al. Transfer of regulatory T cells into abortion-prone mice promotes the expansion of uterine mast cells and normalizes early pregnancy angiogenesis. Sci. Rep. 5, 13938; 10.1038/srep13938 (2015).
Acknowledgments
We are very thankful to Kristina Heinze and Markus Scharm for their excellent technical assistance and to Dr. F. Röhl for his excellent assistance with the statistic analysis. This work benefitted from the support of the DFG SPP 1394 “Mast cells-Promoters of Health and Modulators of Disease (ACZ and MM)”. It was funded with grants from the DFG (ZE 526/6-1 and DFG 526/6-2) and Fritz Thyssen (10.08.2.179) to ACZ.
Footnotes
Author Contributions K.W., N.M., A.S. and A.G. performed experiments, K.W. analyzed data. K.W. and N.M. contributed to manuscript preparation. M.M. provided scientific input and contributed to manuscript preparation. A.C.Z. supervised the project, provided the financial support and wrote the paper. All authors reviewed the paper.
References
- Rätsep M. T. et al. Uterine natural killer cells: supervisors of vasculature construction in early decidua basalis. Reproduction (Cambridge, England) 149, R91–102 (2015). [Abstract] [Google Scholar]
- Linzke N., Schumacher A., Woidacki K., Croy B. A. & Zenclussen A. C. Carbon monoxide promotes proliferation of uterine natural killer cells and remodeling of spiral arteries in pregnant hypertensive heme oxygenase-1 mutant mice. Hypertension 63, 580–588 (2014). [Abstract] [Google Scholar]
- Zenclussen A. C., Olivieri D. N., Dustin M. L. & Tadokoro C. E. In vivo multiphoton microscopy technique to reveal the physiology of the mouse uterus. American journal of reproductive immunology (New York, N.Y.: 1989) 69, 281–289 (2013). [Abstract] [Google Scholar]
- Plaks V. et al. Uterine DCs are crucial for decidua formation during embryo implantation in mice. The Journal of clinical investigation 118, 3954–3965 (2008). [Abstract] [Google Scholar]
- Barrientos G. et al. CXCR4(+) dendritic cells promote angiogenesis during embryo implantation in mice. Angiogenesis 16, 417–427 (2013). [Abstract] [Google Scholar]
- Woidacki K. et al. Mast cells rescue implantation defects caused by c-kit deficiency. Cell death & disease 4, e462 (2013). [Europe PMC free article] [Abstract] [Google Scholar]
- Bosquiazzo V. L., Ramos J. G., Varayoud J., Muñoz-de-Toro M. & Luque E. H. Mast cell degranulation in rat uterine cervix during pregnancy correlates with expression of vascular endothelial growth factor mRNA and angiogenesis. Reproduction (Cambridge, England) 133, 1045–1055 (2007). [Abstract] [Google Scholar]
- Welle M. Development, significance, and heterogeneity of mast cells with particular regard to the mast cell-specific proteases chymase and tryptase. Journal of leukocyte biology 61, 233–245 (1997). [Abstract] [Google Scholar]
- Fest S. et al. Trophoblast-macrophage interactions: a regulatory network for the protection of pregnancy. American journal of reproductive immunology (New York, N.Y.: 1989) 57, 55–66 (2007). [Abstract] [Google Scholar]
- Care A. S. et al. Macrophages regulate corpus luteum development during embryo implantation in mice. The Journal of clinical investigation 123, 3472–3487 (2013). [Europe PMC free article] [Abstract] [Google Scholar]
- Amsalem H. et al. Identification of a novel neutrophil population: proangiogenic granulocytes in second-trimester human decidua. Journal of immunology (Baltimore, Md.: 1950) 193, 3070–3079 (2014). [Abstract] [Google Scholar]
- Maynard S. E. et al. Excess placental soluble fms-like tyrosine kinase 1 (sFlt1) may contribute to endothelial dysfunction, hypertension, and proteinuria in preeclampsia. The Journal of clinical investigation 111, 649–658 (2003). [Europe PMC free article] [Abstract] [Google Scholar]
- Pulgar V. M., Yamashiro H., Rose J. C. & Moore L. G. Role of the AT2 receptor in modulating the angiotensin II contractile response of the uterine artery at mid-gestation. Journal of the renin-angiotensin-aldosterone system: JRAAS 12, 176–183 (2011). [Europe PMC free article] [Abstract] [Google Scholar]
- O’Leary S., Jasper M. J., Robertson S. A. & Armstrong D. T. Seminal plasma regulates ovarian progesterone production, leukocyte recruitment and follicular cell responses in the pig. Reproduction (Cambridge, England) 132, 147–158 (2006). [Abstract] [Google Scholar]
- Sharkey D. J., Tremellen K. P., Jasper M. J., Gemzell-Danielsson K. & Robertson S. A. Seminal fluid induces leukocyte recruitment and cytokine and chemokine mRNA expression in the human cervix after coitus. Journal of immunology (Baltimore, Md.: 1950) 188, 2445–2454 (2012). [Abstract] [Google Scholar]
- Bosma M. J. & Carroll A. M. The SCID mouse mutant: definition, characterization, and potential uses. Annual review of immunology 9, 323–350 (1991). [Abstract] [Google Scholar]
- Teles A. et al. Control of uterine microenvironment by foxp3(+) cells facilitates embryo implantation. Frontiers in immunology 4, 158 (2013). [Europe PMC free article] [Abstract] [Google Scholar]
- Samstein R. M., Josefowicz S. Z., Arvey A., Treuting P. M. & Rudensky A. Y. Extrathymic generation of regulatory T cells in placental mammals mitigates maternal-fetal conflict. Cell 150, 29–38 (2012). [Europe PMC free article] [Abstract] [Google Scholar]
- Zenclussen A. C. et al. Abnormal T-cell reactivity against paternal antigens in spontaneous abortion: adoptive transfer of pregnancy-induced CD4+CD25+ T regulatory cells prevents fetal rejection in a murine abortion model. The American journal of pathology 166, 811–822 (2005). [Europe PMC free article] [Abstract] [Google Scholar]
- Lu L.-F. et al. Mast cells are essential intermediaries in regulatory T-cell tolerance. Nature 442, 997–1002 (2006). [Abstract] [Google Scholar]
- Jones T. G., Finkelman F. D., Austen K. F. & Gurish M. F. T regulatory cells control antigen-induced recruitment of mast cell progenitors to the lungs of C57BL/6 mice. Journal of immunology (Baltimore, Md.: 1950) 185, 1804–1811 (2010). [Europe PMC free article] [Abstract] [Google Scholar]
- Gri G. et al. CD4+CD25+ regulatory T cells suppress mast cell degranulation and allergic responses through OX40-OX40L interaction. Immunity 29, 771–781 (2008). [Europe PMC free article] [Abstract] [Google Scholar]
- Frossi B. et al. Single-cell dynamics of mast cell-CD4+ CD25+ regulatory T cell interactions. European journal of immunology 41, 1872–1882 (2011). [Abstract] [Google Scholar]
- Hershko A. Y. et al. Mast cell interleukin-2 production contributes to suppression of chronic allergic dermatitis. Immunity 35, 562–571 (2011). [Europe PMC free article] [Abstract] [Google Scholar]
- Zhang W. et al. Transforming growth factor beta 1 plays an important role in inducing CD4(+)CD25(+)forhead box P3(+) regulatory T cells by mast cells. Clinical and experimental immunology 161, 490–496 (2010). [Abstract] [Google Scholar]
- Shelesnyak M. C. ANTIHISTAMINES AND THE OVUM. British medical journal 2, 1268 (1963). [Europe PMC free article] [Abstract] [Google Scholar]
- Norrby K. Mast cells and angiogenesis. APMIS: acta pathologica, microbiologica, et immunologica Scandinavica 110, 355–371 (2002). [Abstract] [Google Scholar]
- Bytautiene E., Vedernikov Y. P., Saade G. R., Romero R. & Garfield R. E. IgE-independent mast cell activation augments contractility of nonpregnant and pregnant guinea pig myometrium. International archives of allergy and immunology 147, 140–146 (2008). [Abstract] [Google Scholar]
- Jensen F. et al. Estradiol and progesterone regulate the migration of mast cells from the periphery to the uterus and induce their maturation and degranulation. PloS one 5, e14409 (2010). [Europe PMC free article] [Abstract] [Google Scholar]
- Schumacher A. et al. Mechanisms of action of regulatory T cells specific for paternal antigens during pregnancy. Obstetrics and gynecology 110, 1137–1145 (2007). [Abstract] [Google Scholar]
- Yin Y., Han X., Shi Q., Zhao Y. & He Y. Adoptive transfer of CD4+CD25+ regulatory T cells for prevention and treatment of spontaneous abortion. European journal of obstetrics, gynecology, and reproductive biology 161, 177–181 (2012). [Abstract] [Google Scholar]
- Zenclussen A. C. et al. Regulatory T cells induce a privileged tolerant microenvironment at the fetal-maternal interface. European journal of immunology 36, 82–94 (2006). [Abstract] [Google Scholar]
- Thuere C. et al. Kinetics of regulatory T cells during murine pregnancy. American journal of reproductive immunology (New York, N.Y.: 1989) 58, 514–523 (2007). [Abstract] [Google Scholar]
- Girardi G., Yarilin D., Thurman J. M., Holers V. M. & Salmon J. E. Complement activation induces dysregulation of angiogenic factors and causes fetal rejection and growth restriction. The Journal of experimental medicine 203, 2165–2175 (2006). [Europe PMC free article] [Abstract] [Google Scholar]
- Eller K. et al. IL-9 production by regulatory T cells recruits mast cells that are essential for regulatory T cell-induced immune suppression. Journal of immunology (Baltimore, Md.: 1950) 186, 83–91 (2011). [Europe PMC free article] [Abstract] [Google Scholar]
- Gurish M. F. & Boyce J. A. Mast cell growth, differentiation, and death. Clin Rev Allergy Immunol. 22, 107–18 (2002). [Abstract] [Google Scholar]
- Eklund K. K., Ghildyal N., Austen K. F. & Stevens R. L. Induction by IL-9 and suppression by IL-3 and IL-4 of the levels of chromosome 14-derived transcripts that encode late expressed mouse mast cell proteases. J Immunol. 151, 4266–4273 (1993). [Abstract] [Google Scholar]
- Rodewald H. R., Dessing M., Dvorak A. M. & Galli S. J. Identification of a committed precursor for the mast cell lineage. Science. 271, 818–822 (1996). [Abstract] [Google Scholar]
- Khong T. Y., Liddell H. S. & Robertson W. B. Defective haemochorial placentation as a cause of miscarriage: a preliminary study. British journal of obstetrics and gynaecology 94, 649–655 (1987). [Abstract] [Google Scholar]
- El-Mousleh T. et al. Exploring the potential of low doses carbon monoxide as therapy in pregnancy complications. Medical gas research 2, 4 (2012). [Europe PMC free article] [Abstract] [Google Scholar]
- Purcell W. M. & Hanahoe T. H. A novel source of mast cells: the human placenta. Agents and actions 33, 8–12 (1991). [Abstract] [Google Scholar]
- Mitani R. et al. Production of human mast cell chymase in human myometrium and placenta in cases of normal pregnancy and preeclampsia. European journal of obstetrics, gynecology, and reproductive biology 101, 155–160 (2002). [Abstract] [Google Scholar]
- Szewczyk G. et al. Mast cells and histamine: do they influence placental vascular network and development in preeclampsia? Mediators of inflammation 2012, 307189 (2012). [Europe PMC free article] [Abstract] [Google Scholar]
- Shima T. et al. Regulatory T cells are necessary for implantation and maintenance of early pregnancy but not late pregnancy in allogeneic mice. Journal of reproductive immunology 85, 121–129 (2010). [Abstract] [Google Scholar]
- Robertson S. A. et al. Seminal fluid drives expansion of the CD4+CD25+ T regulatory cell pool and induces tolerance to paternal alloantigens in mice. Biology of reproduction 80, 1036–1045 (2009). [Europe PMC free article] [Abstract] [Google Scholar]
- Schumacher A. et al. Human chorionic gonadotropin as a central regulator of pregnancy immune tolerance. Journal of immunology (Baltimore, Md.: 1950) 190, 2650–2658 (2013). [Abstract] [Google Scholar]
- Ball E., Bulmer J. N., Ayis S., Lyall F. & Robson S. C. Late sporadic miscarriage is associated with abnormalities in spiral artery transformation and trophoblast invasion. The Journal of pathology 208, 535–542 (2006). [Abstract] [Google Scholar]
- Lyall F. The human placental bed revisited. Placenta 23, 555–562 (2002). [Abstract] [Google Scholar]
- Pijnenborg R., Vercruysse L. & Hanssens M. The uterine spiral arteries in human pregnancy: facts and controversies. Placenta 27, 939–958 (2006). [Abstract] [Google Scholar]
- Kendall R. L., Wang G. & Thomas K. A. Identification of a natural soluble form of the vascular endothelial growth factor receptor, FLT-1, and its heterodimerization with KDR. Biochemical and biophysical research communications 226, 324–328 (1996). [Abstract] [Google Scholar]
- Peach M. J. Renin-angiotensin system: biochemistry and mechanisms of action. Physiological reviews 57, 313–370 (1977). [Abstract] [Google Scholar]
- Reilly C. F., Tewksbury D. A., Schechter N. M. & Travis J. Rapid conversion of angiotensin I to angiotensin II by neutrophil and mast cell proteinases. The Journal of biological chemistry 257, 8619–8622 (1982). [Abstract] [Google Scholar]
- Urata H. et al. Cellular localization and regional distribution of an angiotensin II-forming chymase in the heart. The Journal of clinical investigation 91, 1269–1281 (1993). [Europe PMC free article] [Abstract] [Google Scholar]
- Miyazaki M., Takai S., Jin D. & Muramatsu M. Pathological roles of angiotensin II produced by mast cell chymase and the effects of chymase inhibition in animal models. Pharmacology & therapeutics 112, 668–676 (2006). [Abstract] [Google Scholar]
- Croy B. A. et al. Identification of the primary outcomes that result from deficient spiral arterial modification in pregnant mice. Pregnancy hypertension 1, 87–94 (2011). [Europe PMC free article] [Abstract] [Google Scholar]
Articles from Scientific Reports are provided here courtesy of Nature Publishing Group
Full text links
Read article at publisher's site: https://doi.org/10.1038/srep13938
Read article for free, from open access legal sources, via Unpaywall:
https://www.nature.com/articles/srep13938.pdf
Citations & impact
Impact metrics
Article citations
Beyond Immune Balance: The Pivotal Role of Decidual Regulatory T Cells in Unexplained Recurrent Spontaneous Abortion.
J Inflamm Res, 17:2697-2710, 01 May 2024
Cited by: 1 article | PMID: 38707955 | PMCID: PMC11070170
Review Free full text in Europe PMC
The Role of Regulatory T Cells and Their Therapeutic Potential in Hypertensive Disease of Pregnancy: A Literature Review.
Int J Mol Sci, 25(9):4884, 30 Apr 2024
Cited by: 0 articles | PMID: 38732104 | PMCID: PMC11084408
Review Free full text in Europe PMC
Regulatory T cell adoptive transfer alters uterine immune populations, increasing a novel MHC-IIlow macrophage associated with healthy pregnancy.
Front Immunol, 14:1256453, 13 Oct 2023
Cited by: 0 articles | PMID: 37901247 | PMCID: PMC10611509
Immune Regulation of Seminal Plasma on the Endometrial Microenvironment: Physiological and Pathological Conditions.
Int J Mol Sci, 24(19):14639, 27 Sep 2023
Cited by: 0 articles | PMID: 37834087 | PMCID: PMC10572377
Review Free full text in Europe PMC
Regulatory T cells are paramount effectors in progesterone regulation of embryo implantation and fetal growth.
JCI Insight, 8(11):e162995, 08 Jun 2023
Cited by: 4 articles | PMID: 37191999 | PMCID: PMC10393240
Go to all (30) article citations
Data
Data behind the article
This data has been text mined from the article, or deposited into data resources.
BioStudies: supplemental material and supporting data
Similar Articles
To arrive at the top five similar articles we use a word-weighted algorithm to compare words from the Title and Abstract of each citation.
Adoptive transfer of pregnancy-induced CD4+CD25+ regulatory T cells reverses the increase in abortion rate caused by interleukin 17 in the CBA/JxBALB/c mouse model.
Hum Reprod, 29(5):946-952, 20 Feb 2014
Cited by: 54 articles | PMID: 24556316
Adoptive cell therapy with induced regulatory T cells normalises the abortion rate in abortion-prone mice.
Reprod Fertil Dev, 33(3):220-228, 01 Feb 2021
Cited by: 4 articles | PMID: 33317684
Maternal T Regulatory Cell Depletion Impairs Embryo Implantation Which Can Be Corrected With Adoptive T Regulatory Cell Transfer.
Reprod Sci, 24(7):1014-1024, 11 Nov 2016
Cited by: 18 articles | PMID: 27834288 | PMCID: PMC5933084
Adoptive transfer of Tregs: A novel strategy for cell-based immunotherapy in spontaneous abortion: Lessons from experimental models.
Int Immunopharmacol, 90:107195, 02 Dec 2020
Cited by: 11 articles | PMID: 33278746
Review