Abstract
Free full text

Gender Specific Mutation Incidence and Survival Associations in Clear Cell Renal Cell Carcinoma (CCRCC)
Abstract
Renal cell carcinoma (RCC) is diagnosed in >200,000 individuals worldwide each year, accounting for ~2% of all cancers, but the spread of this disease amongst genders is distinctly uneven. In the U.S. the male:female incidence ratio is approximately 2:1. A potential hypothesis is mutation spectra may differ between tumors dependent upon the gender of the patient, such as mutations of X chromosome encoded genes being more prevalent in male-derived tumors. Combined analysis of three recent large-scale clear cell renal cell carcinoma (CCRCC) mutation sequencing projects identified a significantly increased mutation frequency of PBRM1 and the X chromosome encoded KDM5C in tumors from male patients and BAP1 in tumors from female patients. Mutation of BAP1 had previously been significantly associated with poorer overall survival; however, when stratified by gender, mutation of BAP1 only significantly affected overall survival in female patients. Mutation of chromatin remodeling genes alters gene regulation, but the overall effect of these alterations may also be modified by the presence of other gender specific factors. Thus, the combination of gender and mutation of a specific gene, such as BAP1, may have implications not only for prognosis but also for understanding the role of chromatin remodeling gene mutations in kidney cancer progression.
Introduction
Renal cell carcinoma (RCC) is diagnosed in >200,000 individuals in the world each year, accounting for ~2% of all cancers, but the incidence of this disease amongst genders is distinctly uneven. In the U.S. between the years of 2001–2010 the age-adjusted incidence rate per 100,000 cancers of the kidney and renal pelvis for males increased from 17.5–20.6 and for females increased from 8.6 to 10.0; however, the ratio of incidence between males and females has remained at approximately 2:1 (ranging from 1.78:1–2.07:1) [1]. This gender bias is not unique to RCC, for instance, a similar gender bias is observed in bladder cancer in the U.S. where between the years of 2001–2010 the male to female ratio has remained at approximately 4:1 (ranging from 3.87:1–4.21:1) [1]. There are currently no known causes for this bias. The recent reports of large scale sequencing and integrated analyses of clear cell RCC (CCRCC), the most common type of RCC (representing ~75% of all cases), provides a large amount of mutation data for CCRCC samples, allowing for the investigation of potential hypotheses [2–4].
One such hypothesis is the potential for gender specific differences in the rate of X chromosome mutation between male and female patients. In males, each cell contains a single X chromosome whilst the complimentary Y chromosome contains similar versions of only a fraction of the genes present on the X chromosome. Thus, a single inactivating mutation of an X chromosome gene in a male patient results in complete loss of gene activity. In females, each cell contains two X chromosomes, one of which is largely inactivated at a very early stage of embryogenesis. Thus, for the majority of genes, a single inactivating mutation would have to occur in the gene on the activated X chromosome to result in loss of gene activity, the only exception being the small number of genes that escape X inactivation. However, this potential mechanism is dependent upon X chromosome gene mutation being observed in, and being relevant to, the carcinogenesis of CCRCC.
Recent studies of CCRCC have highlighted the importance of mutation in chromatin remodeling/modifier genes in CCRCC tumorigenesis, including components of the SWI/SNF complex such as PBRM1, ARID1A and SMARCA4 and histone residue modifiers such as SETD2, KDM5C (JARID1C) and KDM6A (UTX). While several of these most commonly mutated chromatin remodeling/modifier genes are present on chromosome 3p, a chromosomal region that is lost in a high percentage of CCRCC tumors, three of these genes, KDM5C (JARID1C), KDM6A (UTX) and STAG2, are located on the X chromosome [2,5]. In this study the distribution of X chromosome and other genomic alterations was compared between tumors derived from male and female patients. Three recent reports of CCRCC were used to compile a dataset of 628 tumors for use in this analysis.
Methods
Ethics Statement
All patient data was acquired from three previously published studies and each study stated that written informed consents were obtained.
The TCGA KIRC cohort report by the TCGA stated in their methods that “Specimens were obtained from patients, with appropriate consent from institutional review boards”. This data is available in volume 499 of Nature on pages 43–49 [2]. TCGA data concerning genomic and clinical information is organized into two categories: one that is openly accessible to the public and one that has controlled access, available only to qualified researchers obligated to secure the data. The open access data set contains only information that is not individually unique and does not pose a risk of patient re-identification. All the data used within this manuscript was obtained from the open access data set and has passed the criteria for unrestricted publication with the following statement “No restrictions; all data available without limitations” listed at http://cancergenome.nih.gov/abouttcga/policies/publicationguidelines.
The Japanese cohort reported by Sato et al stated in their methods that “Paired tumor-normal DNA was isolated from 240 ccRCC specimens and subjected to comprehensive molecular analyses after written informed consent was obtained. This study was approved by the ethics committee of the Graduate School of Medicine at the University of Tokyo.” This data is available in volume 45 of Nature Genetics on pages 860–867 [3].
The Chinese cohort reported by Guo et al stated in their supplementary methods that “A signed written consent from each patient was obtained before the recruitment in the study according to the regulations of the institutional ethics review boards.” This data is available in volume 44 of Nature Genetics on pages 17–19 [4].
Data Retrieval
All the TCGA KIRC cohort patient clinical and mutation data was accessed from the online open access Nature article entitled “Comprehensive molecular characterization of clear cell renal cell carcinoma” in volume 499, pages 43–49 by selecting the supplementary information [2]. The supplementary information contained in files entitled “Data_File_S2_clinical_dataset.xlsx” and “Data_File_S10_KIRC-BCM-BI-UCSC-gapfill-v1.11.txt” contains all the data used herein.
All the Sato et al cohort patient clinical and mutation data was accessed from the online Nature Genetics article entitled “Integrated molecular analysis of clear-cell renal cell carcinoma” in volume 45, pages 860–867 by selecting the supplementary information [3]. The supplementary information contained in files entitled “Supplementary table 1. Characteristics of the patients” and “SupplementaryTable 3. List of mutations in exome-sequencing” contains all the data used herein.
All the Guo et al cohort patient clinical and mutation data was accessed from the online Nature Genetics article entitled “Frequent mutations of genes encoding ubiquitin-mediated proteolysis pathway components in clear cell renal cell carcinoma” in volume 44, pages 17–19 by selecting the supplementary information [4]. The supplementary information contained in files entitled “Supplementary Text and Figures” and “Supplementary Table 5” contains all the data used herein.
All mutation data retrieved from these three source studies was collated and tabulated into S1 Table.
Data Analysis
Fisher’s exact tests were used to compare the rates of mutation for each gene between the two genders with a p-value of <0.05 considered indicative of a positive trend and a p-value of <0.005 considered statistically significantly due to Bonferroni correction. Kaplan-Meier survival curves were calculated using the cBioPortal software (http://www.cbioportal.org/index.do) [6,7]. The cBioPortal software was used to assess the mutation status of genes within the TCGA bladder cohort, produce oncoprints, and assess the mutual exclusivity of mutations within the cohorts.
Results
To evaluate whether gender bias was present, the mutation rate per gender was assessed for ten genes commonly mutated in CCRCC consisting of the 3 genes present on the X chromosome (KDM5C, KDM6A and STAG2), the 4 genes present within the commonly lost and mutated chromosome 3p region (VHL, PBRM1, SETD2 and BAP1) and the ARID1A (chr.1p), PTEN (chr.10q) and TP53 (chr.17p) genes. These genes were assessed by combining the data from three recent studies of CCRCC tumors consisting of cohorts representing patients from the U.S.A., Japan and China that all demonstrated a higher degree of male patients. The U.S.A. based cancer genome atlas (TCGA) clear cell kidney cancer (KIRC) report consisted of 424 sequenced samples with 277 males and 147 females (male/female ratio 1.88:1) [2]. The Japanese cohort reported by Sato et al consisted of 106 sequenced samples with 78 males and 28 females (male/female ratio 2.79:1) [3]. The Chinese cohort reported by Guo et al consisted of 98 sequenced samples with 59 males and 39 females (male/female ratio 1.51:1) [4]. This produced a total of 628 sequenced samples with 414 males and 214 females (male/female ratio 1.93:1) that were investigated for nine of the ten genes (excluding STAG2) and 530 sequenced samples (male/female ratio 2.03:1) that were investigated for STAG2 due to an absence of data in the Guo et al publication.
The combined cohort demonstrated no difference in mutation frequency between male or female derived tumors for 6 out of ten genes, including two of the X chromosome genes, KDM6A and STAG2 (Fig 1 and Table 1). Two genes, BAP1 and ARID1A, demonstrated an increased mutation rate in female derived tumors, but only BAP1 was considered statistically significant after Bonferroni correction (p = 0.0042) (Fig 1 and Table 1). Notably, this trend was statically significant in the TCGA KIRC cohort alone (p = 0.0001), but was not observed in either of the other two cohorts (Table 1), possibly a reflection of the differing genetic backgrounds present in these ethnically different cohorts. The combined BAP1 mutation rate in CCRCCs was 8.8% in comparison to 6.3% in males and 13.6% females (Fig 1). Two genes, KDM5C and PBRM1, demonstrated a statistically significant increase in mutation rate in male derived tumors with p-values of p<0.0001 and p = 0.0041 respectively. The trend for KDM5C was observed in all three cohorts with no KDM5C mutations observed in any female derived tumors in two of the cohorts and the remaining TCGA KIRC cohort demonstrated only 3 out of 29 KDM5C mutations in female derived tumors and a statistically significant trend of increased mutation rate in male derived tumors (p = 0.0039) (Table 1). The combined KDM5C mutation rate in CCRCCs was 6.7% in comparison to 9.4% in males and 1.4% females (Fig 1). The trend for PBRM1 was present in all three cohorts; however it was only the combination of these cohorts that demonstrated statistical significance (Table 1). The combined PBRM1 mutation rate in CCRCCs was 32.8% in comparison to 36.7% in males and 25.2% females (Fig 1).
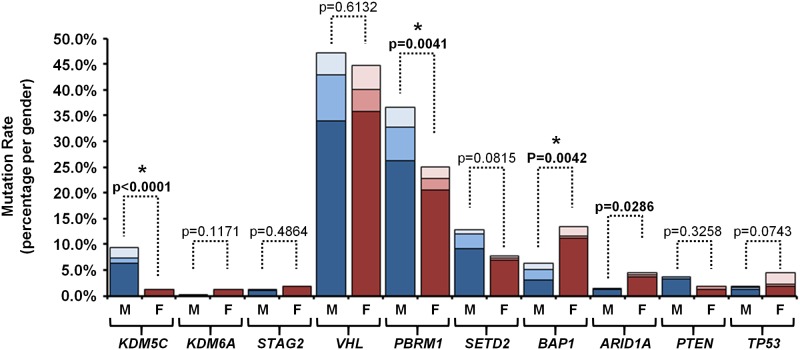
The percentage of mutation for 10 genes commonly mutated in CCRCC genes were collated for 628 tumors from three large sequencing projects (STAG2 data was only available from two projects) [2–4]. The difference in mutation frequency between genders was assessed by Fisher’s Exact tests. Bold text represents statistically significant differences before Bonferroni correction (p-value < 0.05) and * represents statistically significant differences after Bonferroni correction (p-value < 0.005). The expected percentage of mutations in male or female patients was calculated based on the overall mutation rate and the ratio of males to females. The blue bars represent the male patients with dark blue signifying the TCGA cohort, blue signifying the Sato et al cohort and light blue signifying the Guo et al cohort. The red bars represent the female patients with dark red signifying the TCGA cohort, red signifying the Sato et al cohort and light red signifying the Guo et al cohort.
Table 1
Chromosome X | Chromosome 3p | Chr. 1 | Chr. 10 | Chr. 17 | |||||||
---|---|---|---|---|---|---|---|---|---|---|---|
Mutated Gene | KDM5C | KDM6A | STAG2 | VHL | PBRM1 | SETD2 | BAP1 | ARID1A | PTEN | TP53 | |
All Cohorts | Total | 42 (6.7%) | 4 (0.6%) | 9 (1.4%) | 291 (46.3%) | 206 (32.8%) | 70 (11.1%) | 55 (8.8%) | 16 (2.5%) | 19 (3.0%) | 18 (2.9%) |
Male | 39 | 1 | 5 | 195 | 152 | 53 | 26 | 6 | 15 | 8 | |
Female | 3 | 3 | 4 | 96 | 54 | 17 | 29 | 10 | 4 | 10 | |
Fisher's Exact | <0.0001 | 0.1171 | 0.4864 | 0.6132 | 0.0041 | 0.0815 | 0.0042 | 0.0286 | 0.3258 | 0.0743 | |
TCGA KIRC | Total | 29 (6.8%) | 4 (0.9%) | 8 (1.9%) | 218 (51.4%) | 153 (36.1%) | 53 (12.5%) | 37 (8.7%) | 13 (3.1%) | 17 (4.0%) | 9 (2.1%) |
Male | 26 | 1 | 4 | 141 | 109 | 38 | 13 | 5 | 14 | 5 | |
Female | 3 | 3 | 4 | 77 | 44 | 15 | 24 | 8 | 3 | 4 | |
Fisher's Exact | 0.0039 | 0.1223 | 0.4566 | 0.8383 | 0.0567 | 0.3555 | 0.0001 | 0.0706 | 0.1929 | 0.5047 | |
Sato et al | Total | 4 (3.8%) | 0 (0.0%) | 1 (0.9%) | 46 (43.4%) | 32 (30.2%) | 13 (12.3%) | 9 (8.5%) | 2 (1.9%) | 2 (1.9%) | 3 (2.8%) |
Male | 4 | 0 | 1 | 37 | 27 | 12 | 8 | 1 | 1 | 2 | |
Female | 0 | 0 | 0 | 9 | 5 | 1 | 1 | 1 | 1 | 1 | |
Fisher's Exact | 0.5712 | 1.0000 | 1.0000 | 0.1875 | 0.1489 | 0.1767 | 0.4397 | 0.4604 | 0.4604 | 1.0000 | |
Guo et al | Total | 9 (9.2%) | 0 (0.0%) | n/a | 27 (27.6%) | 21 (21.4%) | 4 (4.1%) | 9 (9.2%) | 1 (1.0%) | 0 (0.0%) | 6 (6.1%) |
Male | 9 | 0 | n/a | 17 | 16 | 3 | 5 | 0 | 0 | 1 | |
Female | 0 | 0 | n/a | 10 | 5 | 1 | 4 | 1 | 0 | 5 | |
Fisher's Exact | 0.0104 | 1.0000 | n/a | 0.8194 | 0.1312 | 1.0000 | 1.0000 | 0.3980 | 1.0000 | 0.0354 |
The greatest increase in mutation rate in male derived tumors was observed in the X chromosome encoded KDM5C gene, but the other two X chromosome genes, KDM6A and STAG2, demonstrated no gender bias and the BAP1 and PBRM1 genes that additionally demonstrated gender bias are both on chromosome 3. This suggests that the chromosomal position of the mutated gene may only be one factor in determining any mutation rate differences and could be gene specific. To further assess the potential for both gender bias in mutation rate and a correlation between mutation of X chromosome encoded genes and male derived tumors a similar analysis was performed on the TCGA bladder urothelial carcinoma (BLCA) cohort. The TCGA BLCA cohort consisted of 128 tumors derived from 96 male patients and 32 female patients (male:female ratio = 3:1) and eight genes were evaluated including the same three X chromosome encoded genes (KDM5C, KDM6A and STAG2), four additional chromatin remodeling/modifier genes (ARID1A, SETD2, MLL2 and MLL3) and TP53. This demonstrated no increased mutation rate for X chromosome encoded genes in male derived tumors or statistically significant increases in mutation rate associated with either gender (S2 Table).
The three genes that demonstrated significant gender specific mutation rates in CCRCC; KDM5C, PBRM1 and BAP1, also represent genes that are highly associated with this type of cancer. Comparison of the three cohorts with the large number of cancer sequencing projects (n = ~70) available on the cBio Portal [6,7] website were assessed using cBio Portal software and demonstrated that these three cohorts represent the three highest mutation frequencies of PBRM1 mutation, the 3rd to 5th highest mutation frequencies of BAP1 mutation and the 2nd, 3rd and 7th highest mutation frequencies of KDM5C mutation (Fig 2). An assessment of mutual exclusivity between these ten selected genes within the TCGA KIRC cohort also demonstrated that BAP1 and PBRM1 were the only genes that demonstrated a statistically significant tendency towards mutual exclusivity (S1 Fig). This mutual exclusivity was more pronounced in the male patients where no tumors had mutation of both genes, in contrast, in the female patients 8 out of the 24 tumors with BAP1 mutations also harbored PBRM1 mutations (S1 Fig). This is suggestive of a similar resultant effect of losing either gene, especially in males, and in combination with a potential selection bias between the genders, that this resultant effect is more potent with PBRM1 loss in males and BAP1 loss in females.
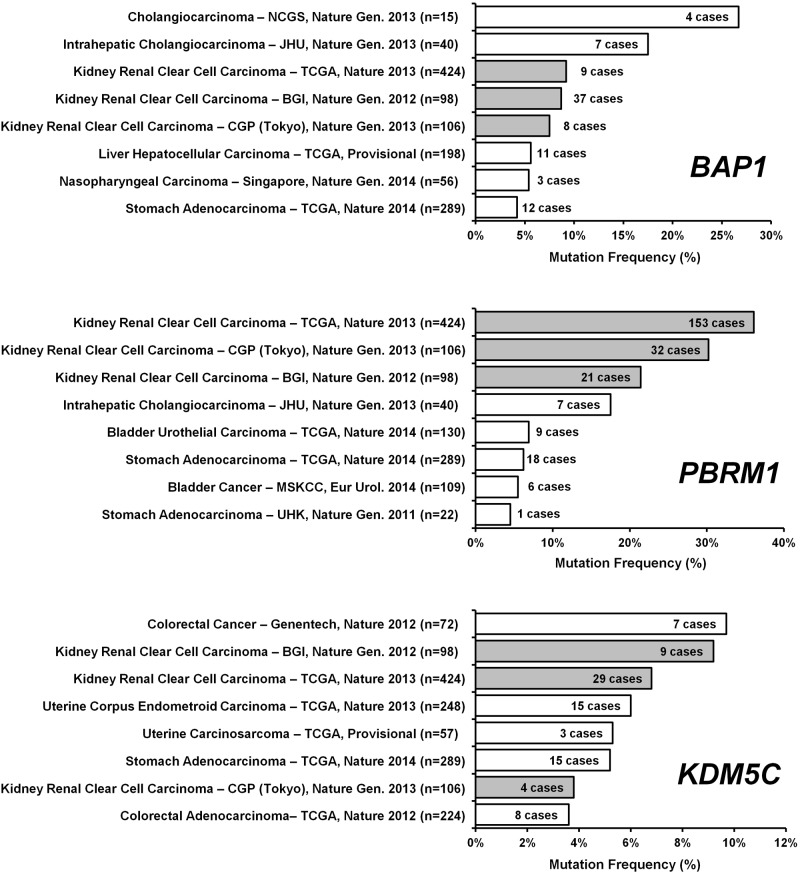
The relative mutation frequencies of PBRM1, BAP1 and KDM5C in the three CCRCC cohorts were compared to the large number of cancer genome studies listed within the cBioPortal software (http://www.cbioportal.org/index.do) [6,7]. The three CCRCC cohorts were highlighted in grey with the TCGA cohort referred to as TCGA, Nature 2013, the Sato et al cohort referred to as CGP (Tokyo), Nature Gen. 2013 and the Guo et al cohort referred to as BGI, Nature Gen. 2013.
In addition to their strong association with CCRCC, mutation of these chromatin remodeling/modifier genes has been associated with poorer patient survival and higher stage disease. The survival data was publically available for the TCGA KIRC cohort and mutation in these three genes was assessed in total and by gender using the cBio Portal software. Comparison of male and female patients demonstrated no difference in survival between the two genders (p = 0.516) (S2 Fig). Mutation of BAP1 was associated with a significantly poorer survival in total (p = 0.0039) and in female patients (p = 0.0021), but not in male patients (p = 0.7659) (Fig 3). Whereas, mutation in either PBRM1 or KDM5C did not associate with a statistically significant effect on patient survival in total or by gender (Fig 3 and S2 Fig).
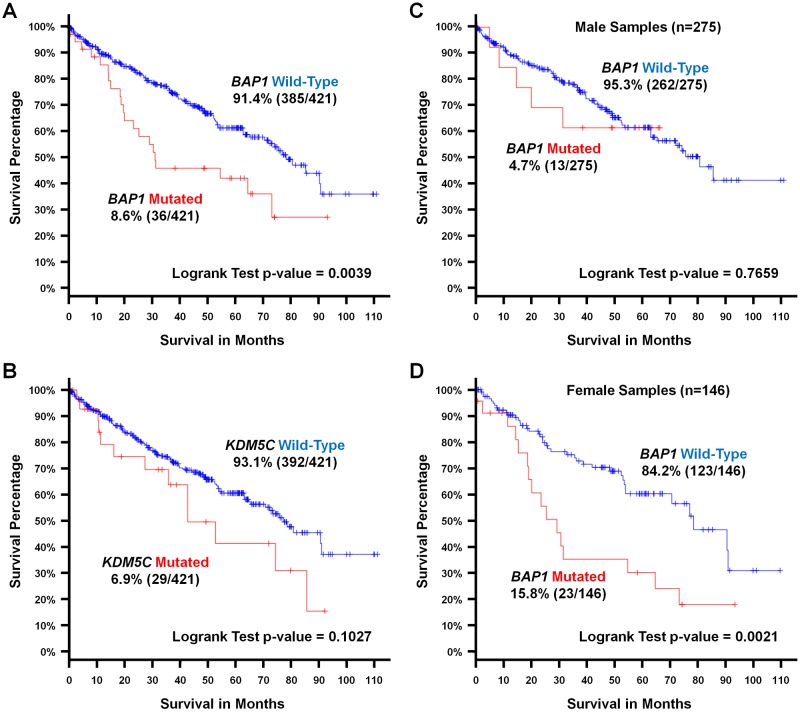
(A and B) Kaplan-Meier survival analysis graphs were produced comparing samples with or within mutation of either BAP1 or KDM5C for CCRCC TCGA cohort and (C and D) comparing the differences in survival with or without mutation of BAP1 for each gender. Analysis was performed using the cBioPortal software (http://www.cbioportal.org/index.do) [6,7] and mutated samples were represented by red lines and wild-type samples by blue lines. Survival data was available for 421 samples within the CCRCC TCGA cohort consisting of 275 males and 146 females. p-values less than 0.01 were considered statistically significant.
Discussion
The observation of gender bias in clear cell renal cell carcinoma is likely to be the result of multiple factors including genetic and environmental, as well as the differing basic physiology between males and females, such as differing levels of hormones. Increased mutation rate within X chromosome encoded genes in male patients presents as one potential genetic factor and this analysis suggests that this may be true for specific X chromosome genes, such as KDM5C, but was not universally observed for the X chromosome genes associated with CCRCC. The observed significance of the KDM5C gene mutation may be due to its relatively high mutation rate in CCRCC compared to the other two X chromosome encoded genes assessed, KDM6A and STAG2, or due to mutation of this gene having a greater effect on CCRCC tumorigenesis than the other two. Interestingly, a recent publication demonstrated tumor heterogeneity in two CCRCC tumors where one tumor from a male patient had the same VHL and PBRM1 mutations in all regions but had gained different KDM5C mutations in different tumor regions demonstrating the repeated importance of losing expression of this gene [8]. Additionally, a recent report suggested that the loss of KDM5C activity has a greater tumorigenic effect in cells that have lost VHL which may explain the higher rate of KDM5C mutation in the frequently VHL mutated CCRCCs [9].
A further factor concerning X chromosome encoded genes is the effect of X inactivation in female patients. Although the majority of genes on the X chromosome are subject to inactivation of one allele, both KDM5C and KDM6A are examples of genes that escape X inactivation, therefore resulting in two active copies in female cells that would need to be biallelically inactivated in a similar manner to any autosomal gene to match a single mutation in a male tumor [10–12]. This is possibly due to the existence of homologues of these two genes on the Y chromosome with KDM5D demonstrating an ~85% identity to KDM5C and UTY demonstrating an ~83% identity to KDM6A (UTX) gene. These genes may vary in their abilities to compensate for loss of the X chromosome versions and the so the increases in KDM5C mutation but not KDM6A mutation in males may be due to the inability of KDM5D to compensate for KDM5C loss. Few studies have assessed this, but in murine neurons it has been shown that KDM5D is expressed at lower levels than KDM5C [13]. The observation of a gender bias in KDM5C mutation was observed in all three cohorts demonstrating no differences due to the differing ethnical background of each cohort, but this observation should be investigated in further cohorts to confirm this correlation.
The observation that mutations of PBRM1 or BAP1 are more common in males and females respectively seems less logical as they are both present on chromosome 3p rather than a sex chromosome. Similarly to KDM5C, both PBRM1 and BAP1 are chromatin remodelers/modifiers and the loss of each gene is thought to have differing effects on mRNA expression profiles, but result in similar overall effects such that a degree of mutual exclusivity is observed between these mutations. Analysis of the CCRCC TCGA cohort demonstrated that loss of these chromatin modifiers produces different mRNA profiles, although loss of multiple genes was observed in some tumors [2]. PBRM1, BAP1 and KDM5C represent chromatin modifying or remodeling genes and thus their loss should affect the mRNA expression profiles within the cells. It has been demonstrated that the normal kidney tissue in male and female rats express differing mRNA profiles and proposed that this might contribute to the molecular mechanism than produces the gender-related differences in renal disease incidence in humans [14]. The loss of chromatin remodeling/modifier genes may therefore have differing effects within this different backgrounds and potentially, the loss of a specific gene may be more advantageous to a tumor that is exposed to the basic physiology of one gender in comparison to the other. Thus, even if mutation of any one of these three genes is equally as likely, the selection pressure for mutation of a specific gene will differ between the genders due to the more beneficial effects of the loss of that gene in a specific gender. It is possible that this is the major driving force for the increased KDM5C mutation frequency in males and its position on the X chromosome is simply an additional advantage to its increased mutation frequency. The convergence of gender specific mRNA expression and effect of loss of a chromatin modifier gene may also explain the observation that BAP1 mutations in female patients predict for significantly poorer survival, but not in male patients. This observation could be very important, but needs to be further confirmed in larger cohorts. This observation may also be dependent upon the ethnic background of the assessed cohort as the female bias for BAP1 mutation was only observed in the TCGA cohort and it was this cohort that provided the survival analysis. Assuming this effect is confirmed in further studies, it may be very important for modifying therapy based on personalized medicine as females with BAP1 mutations may require more immediate and aggressive therapeutic intervention. Additionally, if targeted therapies are identified for BAP1 mutated RCCs the gender of the clinical trial participants should be considered when the trial is planned and analyzed.
In summary, gender specific mutation of genes important for tumorigenesis is evident in CCRCC and the combination of gender and mutation of a specific gene, such as BAP1, may affect the overall survival of the patient. These findings could affect interpretation of genetic alterations in patients with CCRCC and could provide insight into the role of gender factors in initiation or progression of this and other malignancies.
Supporting Information
S1 Fig
Mutual Exclusivity of Common CCRCC-Associated Mutations.(A) Mutual exclusivity of 10 genes (KDM5C, KDM6A, STAG2, VHL, PBRM1, BAP1, SETD2, ARID1A, PTEN and TP53) commonly mutated in the CCRCC TCGA cohort was analyzed using the the cBioPortal software (http://www.cbioportal.org/index.do) [6,7]. (B) This data was separated by gender to produce oncoprints with green boxes designating mutation.
(TIF)
S2 Fig
Gender and Mutation Specific Survival Analysis.(A) Kaplan-Meier survival analysis graphs for the CCRCC TCGA cohort were produced comparing samples based on Gender, (B) with or without mutation of PBRM1 and (C) comparing the differences in survival with or within mutation of PBRM1 or KDM5C for each gender. Analysis was performed using the cBioPortal software (http://www.cbioportal.org/index.do) [6,7] and mutated samples were represented by red lines and wild-type samples by blue lines. p-values less than 0.01 were considered statistically significant.
(TIF)
S1 Table
Mutations identified in the TCGA KIRC, Sato et al, and Guo et al cohorts for 10 Genes Commonly Mutated in Clear cell Renal Cell Carcinoma.(XLSX)
S2 Table
Analysis of Mutation Frequency in 8 Genes Commonly Mutated in Bladder Cancer.(XLSX)
Funding Statement
This research was supported by the Intramural Research Program of the NIH, National Cancer Institute, Center for Cancer Research. Funding was provided by a grant (ZIA BC011028) awarded to Dr. W. Marston Linehan. The funder had no role in study design, data collection and analysis, decision to publish, or preparation of the manuscript.
Data Availability
All relevant data are within the paper and its Supporting Information files.
References
Articles from PLOS ONE are provided here courtesy of PLOS
Full text links
Read article at publisher's site: https://doi.org/10.1371/journal.pone.0140257
Read article for free, from open access legal sources, via Unpaywall:
https://journals.plos.org/plosone/article/file?id=10.1371/journal.pone.0140257&type=printable
Citations & impact
Impact metrics
Citations of article over time
Alternative metrics
Smart citations by scite.ai
Explore citation contexts and check if this article has been
supported or disputed.
https://scite.ai/reports/10.1371/journal.pone.0140257
Article citations
Sex chromosome-encoded protein homologs: current progress and open questions.
Nat Struct Mol Biol, 31(8):1156-1166, 09 Aug 2024
Cited by: 2 articles | PMID: 39123067
Review
Sex and survival outcomes in patients with renal cell carcinoma receiving first-line immune-based combinations.
Cancer Immunol Immunother, 73(8):142, 04 Jun 2024
Cited by: 0 articles | PMID: 38832989 | PMCID: PMC11150359
Sex-specific survival gene mutations are discovered as clinical predictors of clear cell renal cell carcinoma.
Sci Rep, 14(1):15800, 09 Jul 2024
Cited by: 0 articles | PMID: 38982123 | PMCID: PMC11233666
High-frame-rate contrast-enhanced ultrasound to differentiate between clear cell renal cell carcinoma and angiomyolipoma.
BMC Cancer, 24(1):659, 30 May 2024
Cited by: 1 article | PMID: 38816725 | PMCID: PMC11138005
Discovery and Validation of Survival-Specific Genes in Papillary Renal Cell Carcinoma Using a Customized Next-Generation Sequencing Gene Panel.
Cancers (Basel), 16(11):2006, 25 May 2024
Cited by: 0 articles | PMID: 38893126 | PMCID: PMC11171119
Go to all (45) article citations
Data
Data behind the article
This data has been text mined from the article, or deposited into data resources.
BioStudies: supplemental material and supporting data
Similar Articles
To arrive at the top five similar articles we use a word-weighted algorithm to compare words from the Title and Abstract of each citation.
Genomic Biomarkers of a Randomized Trial Comparing First-line Everolimus and Sunitinib in Patients with Metastatic Renal Cell Carcinoma.
Eur Urol, 71(3):405-414, 15 Oct 2016
Cited by: 122 articles | PMID: 27751729 | PMCID: PMC5431298
Aberrant promoter hypermethylation of PBRM1, BAP1, SETD2, KDM6A and other chromatin-modifying genes is absent or rare in clear cell RCC.
Epigenetics, 8(5):486-493, 01 May 2013
Cited by: 35 articles | PMID: 23644518 | PMCID: PMC3741218
Clinical and pathological impact of VHL, PBRM1, BAP1, SETD2, KDM6A, and JARID1c in clear cell renal cell carcinoma.
Genes Chromosomes Cancer, 53(1):38-51, 29 Oct 2013
Cited by: 77 articles | PMID: 24166983
The roles of chromatin-remodelers and epigenetic modifiers in kidney cancer.
Cancer Genet, 208(5):206-214, 20 Feb 2015
Cited by: 38 articles | PMID: 25873528 | PMCID: PMC4494076
Review Free full text in Europe PMC
Funding
Funders who supported this work.
Intramural NIH HHS (1)
Grant ID: ZIA BC011028