Abstract
Free full text

The SMARCA2/4 ATPase domain surpasses the bromodomain as a drug target in SWI/SNF mutant cancers: Insights from cDNA rescue and PFI-3 inhibitor studies
Associated Data
Abstract
The SWI/SNF multi-subunit complex modulates chromatin structure through the activity of two mutually exclusive catalytic subunits, SMARCA2 and SMARCA4, which both contain a bromodomain and an ATPase domain. Using RNAi, cancer-specific vulnerabilities have been identified in SWI/SNF mutant tumors, including SMARCA4-deficient lung cancer, however, the contribution of conserved, druggable protein domains to this anticancer phenotype is unknown. Here, we functionally deconstruct the SMARCA2/4 paralog dependence of cancer cells using bioinformatics, genetic and pharmacological tools. We evaluate a selective SMARCA2/4 bromodomain inhibitor (PFI-3) and characterize its activity in chromatin-binding and cell-functional assays focusing on cells with altered SWI/SNF complex (e.g. Lung, Synovial Sarcoma, Leukemia, and Rhabdoid tumors). We demonstrate that PFI-3 is a potent, cell-permeable probe capable of displacing ectopically expressed, GFP-tagged SMARCA2-bromodomain from chromatin, yet contrary to target knockdown, the inhibitor fails to display an antiproliferative phenotype. Mechanistically, the lack of pharmacological efficacy is reconciled by the failure of bromodomain inhibition to displace endogenous, full-length SMARCA2 from chromatin as determined by in situ cell extraction, chromatin immunoprecipitation and target gene expression studies. Further, using inducible RNAi and cDNA complementation (bromodomain- and ATPase-dead constructs), we unequivocally identify the ATPase domain, and not the bromodomain of SMARCA2, as the relevant therapeutic target with the catalytic activity suppressing defined transcriptional programs. Taken together, our complementary genetic and pharmacological studies exemplify a general strategy for multi-domain protein drug-target validation and in case of SMARCA2/4 highlight the potential for drugging the more challenging helicase/ATPase domain to deliver on the promise of synthetic-lethality therapy.
Introduction
Epigenetic dysregulation plays a fundamental role in the development of cancer (1). Large-scale genome sequencing have uncovered recurrent somatic mutations and copy-number (CN) changes in histone-modifying enzymes and chromatin remodeling complexes supporting a causal role for altered epigenetics states in tumorigenesis (2-4). While the mechanistic consequences of these alterations remain poorly understood, it is appreciated that such events promote acquisition of cell oncogenic capabilities through deregulation of nucleosome-dynamics, gene transcription, DNA replication and repair (5). Indeed, chromatin regulators are emerging as therapeutic targets and inhibitors of histone-modifying enzymes, as well as bromodomains which ‘read’ the histone marks, have recently shown efficacy in preclinical and clinical settings through their ability to reverse oncogenic transcriptional programs (6-8).
The Switch/Sucrose Non Fermentable (SWI/SNF) is a multi-subunit chromatin remodeling complex that consists of one of two mutually exclusive helicase/ATPase catalytic subunits, SMARCA2 and SMARCA4. Together with core and regulatory subunits, SMARCA2/4 couple ATP hydrolysis to the perturbation of histone-DNA contacts. This sculpting of the nucleosomal landscape at promoters provides access to transcription factors and cognate DNA elements facilitating both gene activation and repression (9). Because various SWI/SNF subunits are mutated or lost at high frequency in human tumors (2-4,10), this complex has garnered considerable attention (11). A tumor suppressive role has most strongly been demonstrated in childhood malignant rhabdoid tumors, in which the SMARCB1 (Snf5) subunit is biallelicaly inactivated in nearly all cases (10). Accordingly, knock-out of mouse SMARCB1 results in fully penetrant and lethal cancers with 11 weeks median onset (12). In human synovial carcinoma, recurrent chromosomal translocations, which are diagnostic of the malignancy, result in oncogenic fusions (SS18-SSX) that alter the composition/function of the SWI/SNF complex (13). Pointing to the broader relevance of SWI/SNF in cancers are frequent inactivating mutations in accessory subunits, including ARID1A in ovarian and endometrial carcinomas (14,15), and PBRM1 in renal cell carcinomas (16).
Context-specific molecular vulnerabilities that arise during tumor evolution represent an attractive class of drug targets; however, the frequency and spectrum of somatic lesions often confound efforts to identify such therapeutic targets solely based on genomic information (17). To address this challenge, functional, unbiased chemical and genetic loss-of-function (LOF) platforms, which use either drug-like small-molecules or siRNA/shRNA libraries, hold the promise to systematically identify non-obvious target-genotype interactions that might impact clinical decisions (17-19). Recently, using genetic LOF approaches, three groups have independently identified SMARCA2 as an essential gene in SMARCA4-deficient lung cancer (20-22) proposing a synthetic lethality therapeutic approach. However, it remains unclear whether small-molecule inhibitors of the SMARCA2 bromodomain or ATPase domain can mimic the reported RNAi phenotypes resulting from paralog dependency in SWI/SNF (11,23).
Several subunits in the SWI/SNF complex contain bromodomains, which are evolutionary conserved protein-protein interaction modules that bind acetyl-lysine on proteins and histone tails (6,24). Bromodomains are druggable and following the antitumor activity of JQ1 (6), there is interest in broadly developing small-molecules inhibitors against other family members to dissect their therapeutic potential (1,6,24,25). Here, we speculate that SMARCA2/4 bromodomains could contribute to either assembly or targeting of the SWI/SNF complex to specific genomic loci providing an intervention drug target rationale. However, because bromodomains are frequently found in large protein complexes (and often flanked by additional domains involved in chromatin-binding and protein-protein interactions) RNAi-mediated depletion alone does not reveal the contribution of individual domains to the LOF phenotype, representing a specific challenge for drug-target validation.
In this report we conduct complementary cDNA rescue and pharmacological studies to explore whether the bromodomain of SMARCA2/4 represents a tractable target in SWI/SNF mutant cancers. We characterize the PFI-3 bromodomain inhibitor in biochemical assays and across preclinical models with altered SWI/SNF complex (lung, synovial sarcoma, leukemia, and rhabdoid tumor cells) and discover that bromodomain function of SMARCA2/4 is dispensable for tumor cell proliferation, while the catalytic ATPase activity is essential.
Materials and Methods
Bioinformatics
Genome sequencing data and CN information were downloaded from cBioPortal (Supplementary Table S1). Cell line genomic annotation was from the Sanger (www.cancerrxgene.org) and the Broad Institutes (www.broadinstitute.org/ccle). Outlier sum statistics (26) and standard software packages for sequence analysis were used.
Cell lines
Cells obtained from the American Type Culture Collection (ATCC) were cultured accordingly: RPMI-1640 (A549, H1299, H157, H520, H460, HeLa, and THP-1); Iscove's Modified Dulbecco's Medium (MV-4-11); McCoy's 5a Modified Medium (A-204 and G-401) and supplemented with 10% FBS (Gibco). The Aska and Yamato cells (Osaka Medical Center, Japan) were grown in Dulbecco's Modified Eagle Medium (20% FBS). All Cell lines were mycoplasma negative (LookOut® Mycoplasma Kit PCR, Sigma) and maintained at low passage (<3 month) after thawing from master vials (IACS and Pfizer BioBanks) subjected to short tandem repeat (STR) profiling of polymorphic loci (Promega PowerPlex® 16 system) with a >80% match criteria for cell line authentication.
Immunoblotting
Analysis was performed on whole-cell lysates (Supplementary Information) using primary antibodies: SMARCA4 (Abcam, #108318), SMARCA2 (Abcam, #15597), HA-Tag (Cell Signaling, #2367), α-Tubulin (Cell signaling, 3873) and secondary antibodies (Li-Cor, #926-68020, #926-32111).
Assays
PFI-3 is available from SGC (http://www.thesgc.org/chemical-probes/PFI-3). Bromodomain selectivity was measured using ligand binding, site-directed competitive assays (BROMOscan, DiscoverRx) (27). Cell potency was measured using in situ cell extraction, CellTiter-Glo (Promega) and clonogenic assays (Supplementary Information).
RNAi, plasmids, gene expression and ChIP
SMARCA2/4 shRNA (SIGMA TRC-collection) and siRNA (ON-TARGET PLUS Dharmacon) sequences are listed in Supplementary Table S2. ATPase-dead (K785A) and Bromodomain mutations (Y1497F and N1540W) were made in human SMARCA4 cDNA (GeneCopoeia #GC-Y3533) using site-directed mutagenesis (QuickChange, Agilent Technologies) with equivalent mutations in SMARCA2 (GeneCopoeia #GC-Z4424). All cDNAs, subcloned into lentiviral vectors, were sequence verified and virus generation, infection and generation of stable cell lines were conducted following standard procedures (Supplementary Information). Gene expression (Affymetrix) data and methods have been deposited with NCBI (GSE69088). ChIP and qPCR was conducted as previously described (28).
Results
Genomic alterations in SWI/SNF across human tumors
To build upon recent meta-analysis (2-4), we first examined both SWI/SNF mutation and CN variation drawing on a larger set of patient tumors (n = 10,038) from 45 genome sequencing studies (Supplementary Table S1). Clearly, genomic alterations in the 20 canonical SWI/SNF subunits are highly prevalent (Fig. 1A) occurring in 15% of all cancers (3,4,10). Cancers with the highest frequency of lesions in SWI/SNF subunits are rhabdoid tumors, female cancers including ovarian, uterine, cervical and endometrial, lung and gastric adenocarcinoma, melanoma, esophageal, and renal clear cell carcinoma (Fig. 1A, Supplementary Table S1). A tumor suppressive role of the SWI/SNF complex in these contexts has been recognized based on the high frequency of inactivating mutations, which is further underscored by mouse genetic studies (10,29). In contrast, SWI/SNF mutations do not emerge as significant recurrent alterations in glioblastoma, thyroid cancer, multiple myeloma and acute myeloid leukemia (AML). In AML, SMARCA4 may instead be an oncogene driving cMYC transcription in concert with BRD4 (7,11,30,31). As such, it appears that cellular and tissue context defines the tumor suppressive or oncogenic functions of the SWI/SNF complex (5,11,23).
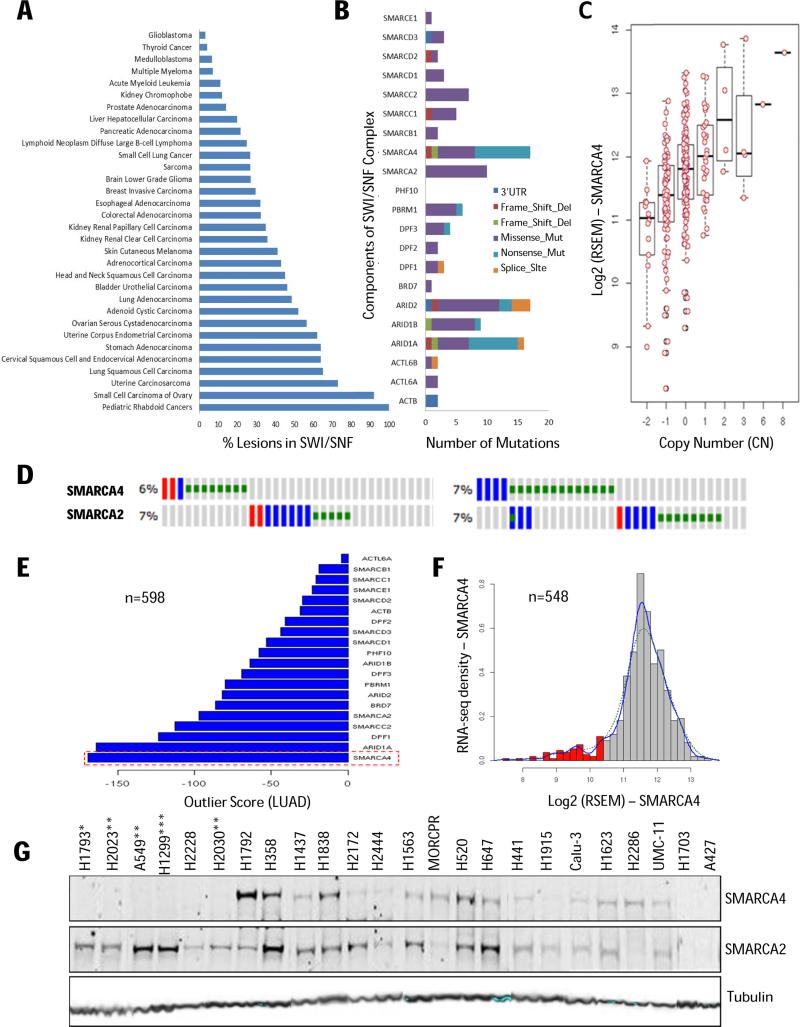
(A) Percentage distribution of lesions (mutations and CN changes) in SWI/SNF components across tumors profiled by TCGA and other laboratories (Supplementary Table S1). (B) SWI/SNF mutation spectrum in LUAD (n=229 tumors; 121 mutations). (C) Correlation of SMARCA4 CN with gene expression (RNA-Seq Expression by Expectation Maximization (RSEM)). (D) CN loss of SMARCA2/4 are mutually exclusive in LUAD (left panel, n = 493) and LUSC (right panel, n = 490). Oncoprint (www.cbioportal.org): blue - high CN loss (GISTIC 2.0 threshold value of −2); red - high CN gain (GISTIC 2.0 threshold value of 2); green - mutations. (E) SMARCA4 has the highest negative outlier sum statistics among SWI/SNF components (LUAD; n = 598). (F) Histogram showing bimodal distribution of SMARCA4 gene expression (LUAD; n = 548) highlighting the predicted patient ‘responder’ population (red). (G) Protein expression and SMARCA4 genomic annotation across lung cancer cell lines: Mutation (*), Copy-Number Loss (**) and Gene Silencing (***).
SMARCA4 deficiency is prevalent and mutually exclusive to CN loss in lung cancer
In primary human lung adenocarcinoma (LUAD) about half of the SMARCA4 mutations are deleterious (nonsense and frameshift mutations) and occur at a 7% frequency in TCGA patient samples (Fig. 1B). Overall, SWI/SNF complex components are mutated in 71/229 patients with an average mutation rate of ~1.7 per sample. In addition, 2 copy loss of SMARCA4 is observed in 14 out of 299 LUAD cases adding to the fraction of SMARCA4-deficient tumors. Because heterozygous SMARCA4 knockout mice are haploinsufficient and tumor prone (29), we also analyzed copy-number driven mRNA expression and conclude that loss of one allele is also sufficient to decrease SMARCA4 expression (Fig. 1C). Focusing on LUAD and lung squamous cell carcinoma (LUSC), mapping of the genomic annotation onto individual patient samples revealed that loss of SMARCA2 and SMARCA4 are largely mutually exclusive (Fig. 1D; Supplementary Fig. S1A). Moreover, with respect to gene expression, outlier statistics identifies SMARCA4, along with ARID1A, as the most significantly altered subunits in the SWI/SNF complex in LUAD (Fig. 1E) with similar profiles observed for LUSC (Supplementary Fig. S1B). At the genome level, SMARCA4 ranks in the top 5% of all genes with negative outlier sum statistics and its bimodal expression profile (Fig. 1F) clearly defines a SMARCA4-deficient patient population.
In good concordance with cell line annotation at the Sanger and the Broad Institutes, SMARCA4 protein expression was non-detectable by western-blot in ~20% (12/50) of lung cancer lines (Fig. 1G; Supplementary Fig. S1C-E). Of eleven SMARCA4-mutant cell lines, only one (NCI-H2286) displayed measurable protein expression (Supplementary Fig. S1F). However, as previously noted, non-detectable SMARCA4 protein levels (as assessed by immunoblotting) occurs more often than predicted from mutation and CN analysis suggesting promoter methylation and epigenetic silencing may be additional oncogenic mechanisms for SMARCA4 loss (32). Paradoxically, a few cell lines appear to have non-detectable expression of both SMARCA2 and SMARCA4 (Fig. 1G). However, such complete dual-loss of SMARCA2/4 is not observed in primary LUAD and LUSC tumors as indicated by both our CN analysis (using both GISTIC and ABSOLUTE algorithms; Supplementary Fig. S1G) and gene expression considerations: Although 3% (19/549) of LUAD patients can be categorized as having low expression of both SMARCA2 and SMARC4 (10th percentile; hypergeometric distribution), the expression profile for SMARCA2 lacks the bimodal distribution characteristic for SMARCA4-null cancers (Fig. 1F). Altogether, the above biomarker assessment (i.e. SMARCA4 loss) outlines a large, well-defined patient population in need of novel molecularly-targeted therapies.
Genotype-specific vulnerability and paralog dependency examined in SMARCA4-reconstituted cells
The recent discovery that SMARCA2 knockdown inhibits the growth of SMARCA4-deficient cancer cells (20-22) has opened a potential therapeutic avenue and received considerable attention (11,23,33). However, it is unknown whether small-molecule inhibitors against the ATPase or bromodomain can mimic the RNAi phenotype. Hence, defining the contribution of each domain to the LOF phenotype is required for prioritizing drug discovery to achieve tangible clinical therapeutic endpoints. To explore this, we first selected a representative panel of SMARCA4-deficient and proficient lung cancer cells (Fig. 2A) and evaluated multiple SMARCA2 shRNAs for knockdown efficiency (Fig. 2B) and phenotype. Using either viability (Fig. 2C) or long-term clonogenic assays (Fig. 2D; Supplementary Fig. S2), we observed robust, genotype-specific growth inhibition when SMARCA2 was depleted in SMARCA4-deficient cell lines (A549, H1299, H157). In contrast, knockdown in SMARCA4-proficient cells (H460, H520, Hela), had no effect on viability confirming the reported synthetic-lethal SMARCA2/4 interaction (20-22).
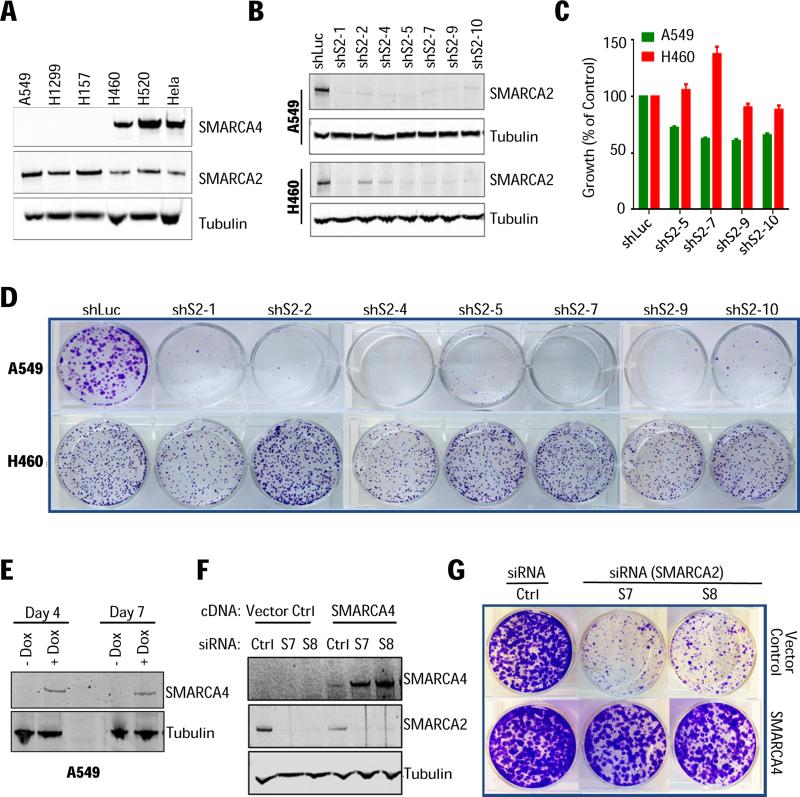
(A) SMARCA2/4 protein levels in cell lines selected for RNAi studies. (B) A549 (SMARCA4-deficient) and NCI-H460 (SMARCA4-proficient) cells transduced with control (shLuc) or SMARCA2-targeting shRNAs (shS2) and assessed for knockdown and (C) cell viability one week post-puromycin selection (SD, n=6). (D) Clonogenic assay and crystal violet staining of colonies after 10-14 days. (E) A549 cells with inducible expression/reconstitution of full-length SMARCA4 cDNA grown in the presence or absence of doxycycline (+/−Dox) and analyzed 4 and 7 days post-doxycycline induction. (F) Following doxycycline treatment (5 days), A549 cells were transfected with either non-silencing control (Ctrl) or SMARCA2 siRNAs (S7 and S8) and evaluated for protein knockdown (5 days post-transfection) and (G) clonogenicity.
Next, we engineered SMARCA4-deficient A549 cells to reexpress a doxycycline-inducible wild-type SMARCA4 cDNA (Fig. 2E). Control cells treated with SMARCA2 siRNAs did not grow, whereas the expression of SMARCA4 (+Dox) completely restored growth (Fig. 2F-2G). Most strikingly, SMARCA4 expression increased 5-fold following SMARCA2 depletion (Fig. 2F) indicative of compensation, a finding that supports the reciprocal assembly and stability of SMARCA2 and SMARCA4 into the SWI/SNF complex (22).
PFI-3 is a selective, potent and cell-permeable SMARCA2/4 bromodomain inhibitor
To explore pharmacological inhibition of the SMARCA2 bromodomain, we next evaluated the small-molecule inhibitor PFI-3 (Fig. 3A) discovered through a collaboration between the Structural Genomic Consortium (SGC) and Pfizer. Biochemically, we determined that PFI-3 binds avidly to both SMARCA2 and SMARCA4 bromodomains (BROMOScan Kd's between 55 and 110 nM) consistent with the binding constant (Kd = 89 nM) measured by isothermal titration calorimetry (www.thesgc.org/chemical-probes/PFI-3). Moreover, using recombinant purified bromodomains, we discovered that PFI-3 binds with similar avidity to both the short and long isoform of SMARCA2 revealing that the alternatively-spliced 18 amino acid insert (34) does not impair PFI-3 binding (Fig. 3A). Moreover, profiling against 32 bromodomains at DiscoveRx (27) confirmed exquisite selectivity vs. other subfamilies (Fig. 3C; Supplementary Table S3) expanding the PFI-3 selectivity information obtained using differential scanning fluorimetry (DSF). In summary, we find there is a good concordance between the ligand competition (BROMOScan) and the direct biophysical binding (DSF) assays and note that in addition to targeting SMARCA2/4, PFI-3 also has activity (~70% inhibition at 2 uM) against the structurally related fifth bromodomain from PBRM1, another SWI/SNF subunit.
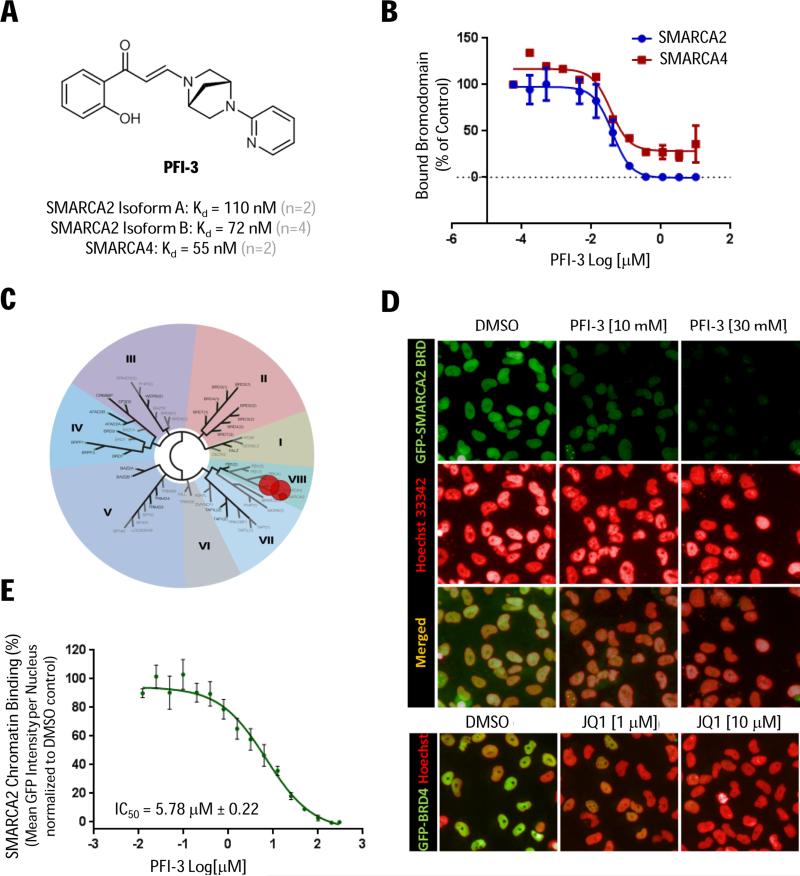
(A) Chemical structure of PFI-3 and biochemical potency (BROMOScan Kd's). (B) BROMOScan dose-response curves using recombinant purified bromodomains. (C) PFI-3 selectivity (2 μM) across 32 bromodomains (DiscoverRx). (D) In situ cell extraction of Hela cells expressing GFP-tagged SMARCA2 bromodomain (green) co-treated with SAHA (5 μM) and PFI-3 (or DMSO control) for 2 hours with Hoescht nuclear counterstain (red). Hela control cells expressing GFP-tagged BRD4 treated (2 hours) with JQ1. (E) Displacement of the SMARCA2 bromodomain from chromatin (IC50) quantified based on mean GFP signal per nucleus (SD, n=6).
In cell-based chromatin binding assays, using in situ cell extraction techniques to remove non-chromatin bound proteins, we observed dose-dependent displacement of GFP-tagged SMARCA2 bromodomain (i.e. 132 amino acid residues) by PFI-3 (Fig. 3D-3E). Notably, the inhibitor showed prolonged cell-target engagement with similar potency (IC50 = 5.78 uM) following 2 and 24 hours treatment (Supplementary Fig. S3). As a negative control, JQ1 did not inhibit the binding of ectopically expressed SMARCA2 bromodomain, but selectively displaced GFP-tagged BRD4 (Fig. 3D and data not shown). Taken together, our cell-biochemical data cooperate the accelerated fluorescence recovery after photobleaching (FRAP) reported for PFI-3 (35) and we conclude that PFI-3 is a selective, cell-permeable probe suitable to study the inhibition of SMARCA2/4 bromodomains in cells.
PFI-3 does not phenocopy the growth inhibitory effects of SMARCA2 knockdown in lung cancer
Armed with PFI-3 and motivated by the context-specific phenotype of SMARCA2 depletion (Fig. 2), we evaluated PFI-3 in the SMARCA4-deficient responder lines (A549, H1299, H157), but observed no anti-proliferative effects in either 3-day cell viability (Fig. 4A) or long-term clonogenic assays (Fig. 4B; Supplementary Fig. S4). Since SWI/SNF is a multi-subunit complex containing numerous chromatin-interacting domains, we speculated that selective SMARCA2 bromodomain inhibition by itself is not sufficient to dislodge the endogenous SWI/SNF complex from chromatin. To elaborate on this, the binding of endogenous (full-length) SMARCA2 to chromatin was monitored by immunofluorescence (IF) in A549 cells using SMARCA2 knockdown as a specificity control (Fig. 4C-4E). Even high concentrations of PFI-3 (30 μM; 1 and 24 hours) were unable to displace the SMARCA2 protein from chromatin (Fig. 4C-4D). Again, we cross-validated the in situ cell extraction assay using the reference JQ1 inhibitor, which potently inhibited chromatin-binding of endogenous BRD4 (Fig. 4C, lower panel) but not SMARCA2 (data not shown). Taken together, these data suggest that the bromodomain of SMARCA2 is dispensable for chromatin binding and SWI/SNF oncogenic activity in lung cancer.
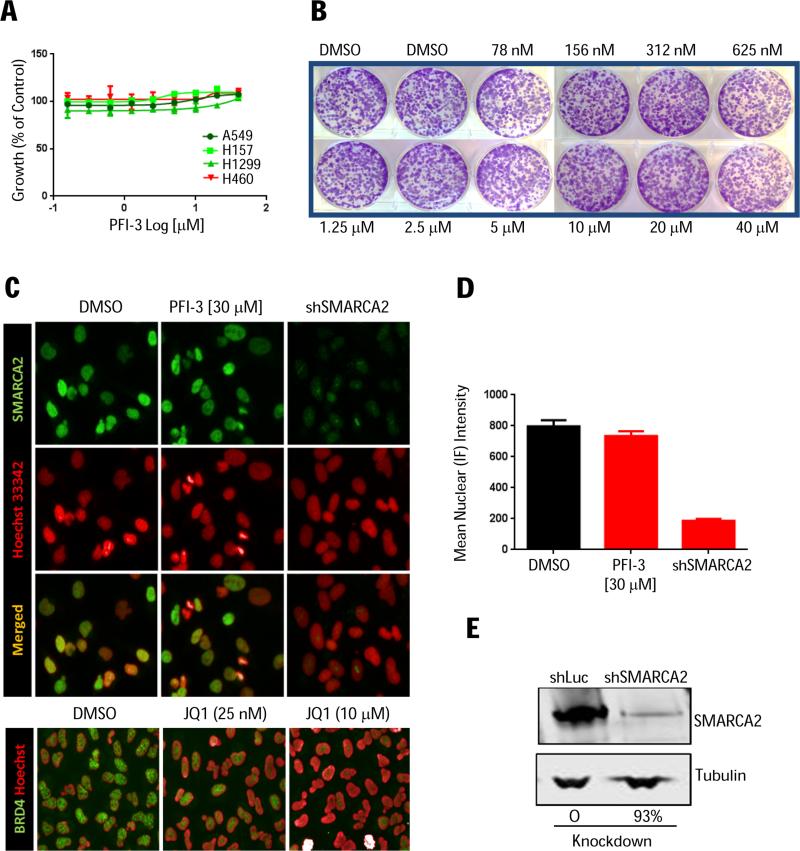
(A) Viability of SMARCA4-deficient (A549, H1299 and H157) or SMARCA4-proficient (H460) cells following PFI-3 treatment (72 hrs). Error bars represent SD, n=3. (B) A549 clonogenic assay (PFI-3 and media replenished every three days for 1.5 weeks). (C) In situ cell extraction (A549 cells) treated with PFI-3 or JQ1 control for 2 hrs followed by IF staining for endogenous, chromatin-bound bromodomain (green) and Hoescht nuclear counterstain (red). (D) IF quantification using SMARCA2 knockdown as specificity control with (E) corresponding immunoblot confirmation.
PFI-3 treatment of synovial sarcoma cells and target gene promoter occupancy studies
As alterations in SWI/SNF have been implicated in disease progression of synovial sarcomas (13), we also evaluated the pharmacological activity of PFI-3 in Yamato and Aska cells. These cells harbor the hallmark recurrent chromosomal translocation t(X;18)(p11.2;q11.2), which fuses the SS18 gene, an integral subunit of SWI/SNF complex, to one of the three SSX genes (SSX1, SSX2, and SSX4), observed in >95% of patients (36). Incorporation of the SS18-SSX fusion protein into the SWI/SNF complex results in eviction and degradation of the tumor-suppressor SMARCB1. The altered SWI/SNF complex binds to the Sox2 locus resulting in aberrant Sox2 expression, which is essential for proliferation of synovial sarcomas (13). Accordingly, Yamato and Aska cells show high levels of Sox2 expression (37). Hence, we hypothesized that PFI-3 may inhibit the altered SWI/SNF complex and impair cell growth, but we did not observe inhibition of cell proliferation in either 4-day viability (Fig. 5A) or long-term proliferation assays (Fig. 5B). We then assessed Sox2 expression and found that PFI-3 treatment (Day 3 and Day 6) failed to reduce Sox2 transcript levels at pharmacologically relevant concentrations (Fig. 5C; Supplementary Fig. S5).
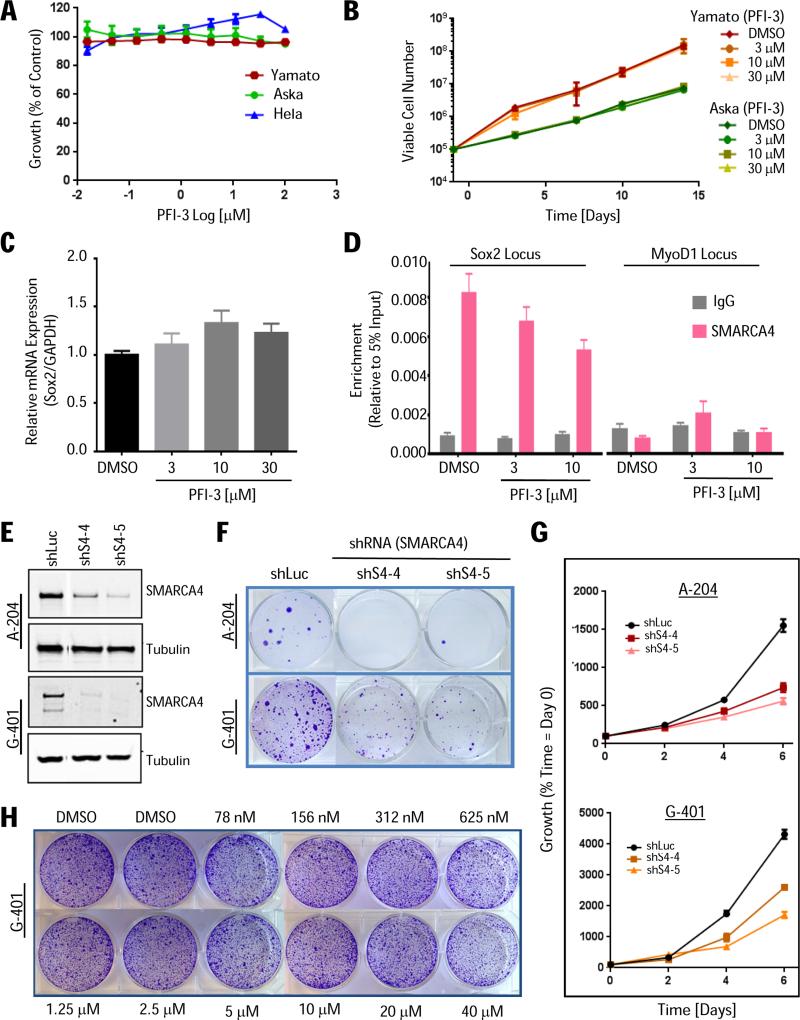
(A) Viability of synovial sarcoma (Aska and Yamato) and HeLa cells treated with PFI-3 (96 hrs) relative to DMSO-treated controls (SEM, n=3). (B) Long-term (2-week) proliferation assay. Cells were split and replenished with fresh media/PFI-3 every 3 or 4 days counting viable cells (SEM, n=3). (C) PFI-3 treatment (3 days) does not repress Sox2 expression in Yamato cells. Sox2 transcript levels (RT-qPCR) normalized to GAPDH (SEM, n=12). (D) Control (DMSO) and PFI-3-treated Yamato cells (day 3) subjected to anti-SMARCA4 ChIP followed by qPCR for Sox2 promoter regions (target gene) or MyoD1 exon1 locus (negative control). The decrease in occupancy at the Sox2 locus (10 uM) is small but significant *P ≤ 0.05 (SEM, n=9). (E) A-204 and G-401 rhabdoid cells transduced with SMARCA4-targeting (shS4-4, shS4-5) or control (shLuc) shRNAs and analyzed for (E) protein knockdown (1 week post-puromycin selection), (F) colony formation (2-3 weeks post-puromycin), and (G) viability (CellTiter-Glo; 6 days post-puromycin). Error bars represent SD, n=6. (H) PFI-3 does not impair growth of G-401 cells (clonogenecity 1.5 weeks; similar data for A-204 not shown). Media/PFI-3 was replenished every three days.
To elaborate on the lack of inhibitor-induced phenotype, we examined SWI/SNF binding at the transcriptionally active Sox2 promoter. SMARCA4 ChIP demonstrated high SWI/SNF complex occupancy at the Sox2 promoter in Yamato cells, as previously reported (13), while no enrichment was observed at the MyoD1 locus, a transcriptionally silent locus as confirmed by RNA Polymerase II ChIP (Fig. 5D; Supplementary Fig. S5D). Importantly, we also examined if PFI-3 treatment could impair binding of the SWI/SNF complex to the Sox2 locus, but observed only a minor change in Sox2 promoter occupancy suggesting inefficient inhibition of SWI/SNF binding (Fig. 5D). These data are consistent with the in situ cell extraction results for PFI-3 (Fig. 4C-4D) showing that SMARCA2/4 bromodomain inhibition cannot displace the multi-subunit SWI/SNF complex from chromatin.
PFI-3 treatment of SMARCA4-dependent rhabdoid cancer or leukemia cells
Rhabdoid tumors are distinctly characterized by biallelic inactivation of SMARCB1, a core subunit of the SWI/SNF complex (10) and genetic studies have demonstrated that oncogenesis mediated by SMARCB1 loss is dependent on the residual activity of SMARCA4-containing SWI/SNF complex (38). To establish a benchmark for PFI-3 treatment of A-204 and G-401 rhabdoid tumor cells, we identified two shRNAs that produced effective (>80%) SMARCA4 protein knockdown (Fig. 5E) and confirmed inhibition of cell viability in both short-term proliferation and long-term clonogenic assays (Fig. 5F-G). In contrast to the RNAi phenotype, pharmacological bromodomain inhibition did not impact the growth of rhabdoid cancer cells (Fig. 5H).
Lastly, we extended our phenotypic evaluation of PFI-3 to leukemia as previous RNAi studies have shown that AML cells depend on SMARCA4 to support oncogenic transcriptional programs (30,31). Similar to our findings across lung, synovial sarcomas and rhabdoid tumor cell lines, PFI-3 treatment did not afford an anti-cancer phenotype in THP-1 and MV4-11 leukemic cells (Supplementary Fig. S6) highlighting the critical importance of pharmacological drug target validation as a follow-up to RNAi-mediated knockdown studies.
Synthetic lethality of SMARCA2 knockdown is linked to the catalytic ATPase activity
To genetically validate the PFI-3 results, we next used a 3’UTR targeting shRNA (shS2) to knockdown endogenous SMARCA2 in H1299 cells engineered to express either wildtype (WT), ATP-binding pocket deficient (K755A) (20) or bromodomain mutant (N1482W) (39) forms of SMARCA2 (Fig. 6A). Ectopic expression of either SMARCA2 WT or the bromodomain binding-deficient mutant (BRD-Mut), but not the ATPase-dead form (ATP-Dead), completely rescued the RNAi-mediated LOF phenotype (Fig. 6B-C). Likewise, A549 cells reconstituted with SMARCA4 WT or BRD-Mut (N1540W, Y1497F), but not ATP-Dead (K785A), were able to grow upon SMARCA2 knockdown (Fig. 6D-F). We also subjected the isogenic matched-pair cell lines to in situ cell extraction and discovered that the SMARCA2 mutants bound chromatin similarly to that of WT (Fig. 7A-B). Hence, failure of the ATP-Dead construct to rescue is due to lack of catalytic activity and not due to gross impairment in chromatin binding. Altogether, our genetic assessment clearly demonstrates that SMARCA4-deficient cancer cells do not require a functional SMARCA2/4 bromodomain for growth. Instead, we unequivocally identify the catalytic activity of the ATPase domain as the appropriate, albeit more challenging, small-molecule drug target.
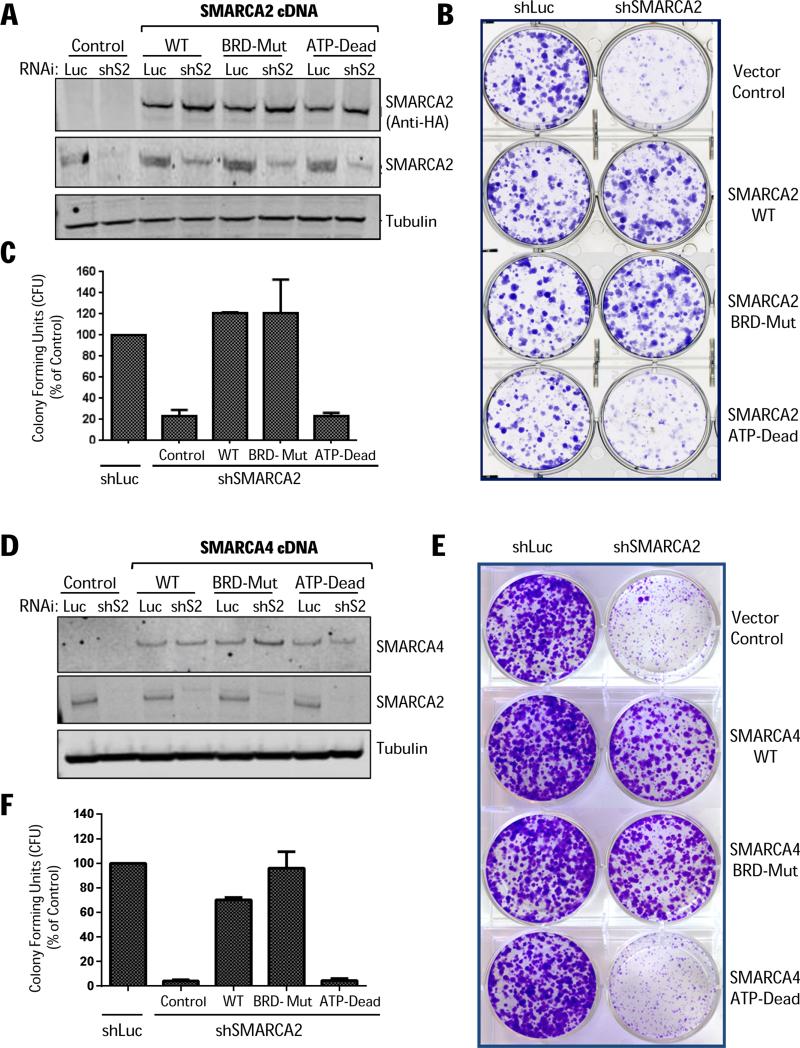
(A) SMARCA2 cDNA rescue experiments in H1299 cells transduced with SMARCA2-targeting (5’UTR) shRNA (shS2) or control shRNA (shLuc) and analyzed by immunoblotting 10 days post-puromycin selection. (B) In parallel, the isogenic cell lines were seeded in 6-well plates (24-hours post-puromycin selection) and after 2 weeks stained by crystal violet and (C) colony forming units (CFU) were quantified. (D) SMARCA4 cDNA rescue/reconstitution experiments in A549 cells transduced with indicated shRNAs and analyzed by immunoblotting 10 days post-puromycin selection. (E) Clonogenic assay (crystal violet staining; 1.5 weeks) and (F) quantification of colony forming units (SD, n=3).
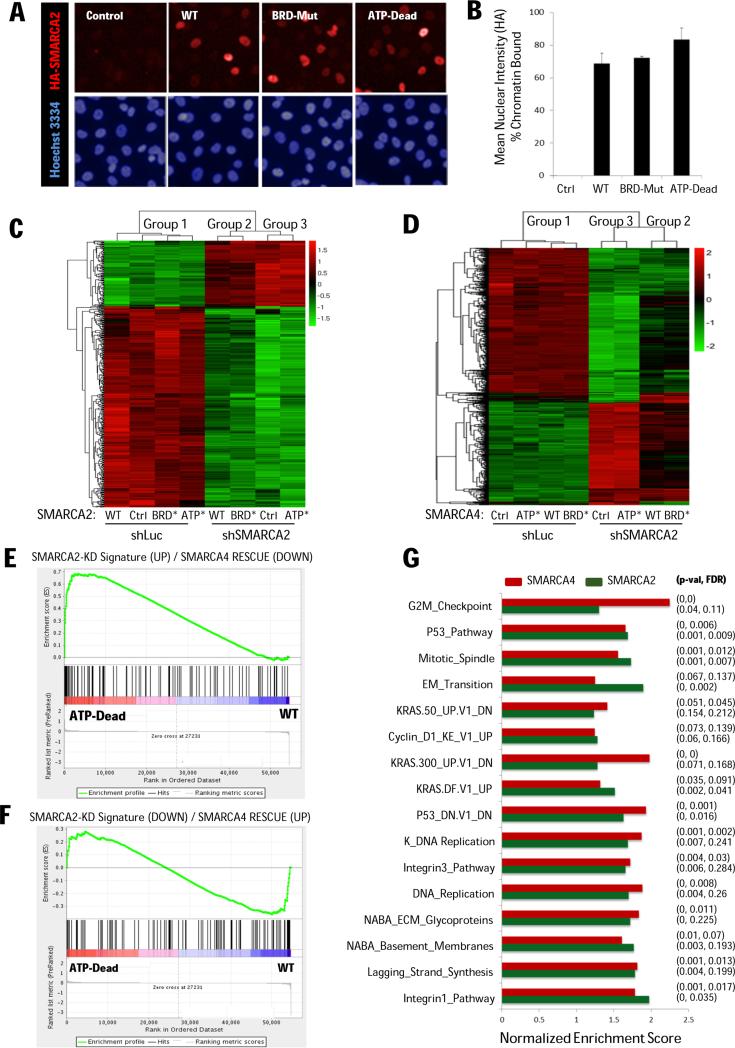
(A) IF images (H1299 cells) expressing either vector control, or HA-tagged SMARCA2 wild-type, bromodomain-mutant, or ATPase-dead constructs (red) and Hoechst counterstain (blue). (B) Quantification of chromatin binding (normalized to non-extracted IF signal). (C) Clustering of 1000 most variable genes for SMARCA2 and (D) SMARCA4 rescue experiments. (E) SMARCA2 knockdown signatures (derived from A549 cells reconstituted with SMARCA4) comprising up-regulated (UP) and (F) down-regulated (DOWN) genes. The ranked gene list (X-axis) was derived from the SMARCA2 rescue experiments comparing ATP-Dead with WT as query. Genes responding differently (interaction contrast – Group 3 vs Group 2) where ranked according to their p-values with direction provided by the fold change. (G) Significantly enriched gene sets (mSigDB) shared between SMARCA2 and SMARCA4 focusing on their ATPase activity (i.e. WT vs ATP-Dead cDNA expression).
Genome-wide microarray analysis of SMARCA2/4 rescue experiments
To examine the dependency on ATPase activity, we generated microarray expression data (GSE69088) for the above cDNA rescue experiments. Unsupervised clustering of the top variable genes, revealed 3 distinct expression profiles that were robust to the gene set size while clustering (Fig. 7C-D). In the absence of SMARCA2 knockdown (shLuc), all H1299 derivative lines clustered together (Group 1) irrespective of the nature of the ectopically expressed SMARCA2 constructs. On the other hand, the SMARCA2 knockdown cells (shSMARCA2), showed strong differential gene expression defining two distinct clusters: cells rescued with either WT or BRD-Mut (Group 2) versus cells expressing either vector control (Ctrl) or ATP-Dead (Group 3). Notably, expression/rescue using either SMARCA2 or SMARCA4 showed identical clustering behavior and differences in mRNA levels within the three groups were non-significant. Hence, the transcriptional profiles reinforce the view that BRD-Mut is able to perform similar functions to the WT gene while ATP-Dead, despite retaining its ability to bind chromatin (Fig. 7A-B), cannot. Consistent with the phenotypic responses (Fig. 6), gene set enrichment analysis (GSEA) revealed up-regulation of apoptosis and death pathways in Group 3 versus the rescued cell lines (Group 2) highlighting the observed context-specific synthetic lethality (Supplementary Fig. S8).
The ATPase activity common between SMARCA2 and SMARC4 share a suppressive function on gene expression programs
Next, focusing on the requirement for ATPase activity, we compared the SMARCA2 and SMARCA4 rescue profiles to see if similar gene expression programs may account for the observed functional complementation. To establish a framework for this analysis, we first derived gene expression signatures for SMARCA4 expression in A549 cells (in the context of SMARCA2 knockdown) comprising the top-100 up-regulated and down-regulated genes, respectively (Supplementary Table S4). Using GSEA, we then looked for enrichment of these signatures with gene lists from SMARCA2 rescue experiments as queries (Figure 7E-F; Supplementary Table S5). When comparing ATP-Dead to WT, the enrichment profiles suggest that the ATPase enzymatic activity preferentially reverses expression of genes that are up-regulated upon RNAi-mediated (synthetic lethal) knock-down of SMARCA2 (Fig 7E). Genes down-regulated upon SMARCA2 knockdown were not fully reversed by the rescue (Fig. 7F; Supplementary Table S5). Therefore, our microarray data clearly shows that the ATPase domain of SMARCA2/4 complements each other at the transcriptional level exerting similar suppressive function on specific gene expression programs.
Next, using GSEA, we identified biological pathways/states shared by both rescue experiments leveraging annotated pathways from the Molecular Signature Database (mSigDB; The Broad Institute). GSEA identified enrichment of cell proliferation (cell cycle, cyclin D1, G2M checkpoint) chromatin remodeling (mitotic spindle, DNA replication and synthesis) and tumorigeneis (EMT, integrin, KRAS and P53 pathway signatures). These gene expression programs, which are enriched in rescued cells compared to cells lacking ATPase activity common to SMARCA2/4, have bonafide tumorigenic functions (Fig. 7G).
In conclusion, the SMARCA4 cDNA complementation (i.e. re-expression) and the SMARCA2 RNAi rescue experiments are consistent with the observed lack of pharmacological activity of PFI-3 in SWI/SNF mutant cancers and we demonstrate for the first time that selective SMARCA2/4 bromodomain inhibition is not a feasible therapeutic strategy for targeting aberrant SWI/SNF activity in SWI/SNF-mutant cancers. Instead, drug discovery efforts should be focused on inhibiting the ATPase catalytic activity to deliver on the promise of robust, cancer-specific synthetic lethal therapy.
Discussion
A common theme has emerged from genetic studies where imbalances between various paralogous subunits within SWI/SNF (e.g. SMARCA2/4 and ARID1A/B) can render cells more tumorigenic and simultaneously hypersensitive to targeting of the residual complex (13,20-23,40,41). However, despite the high prevalence of genomic lesions in SWI/SNF, studies have not addressed how this observation can be translated into effective, drug discovery endpoints.
In this study, we first demonstrated context-specific anti-proliferative phenotype of SMARCA2 depletion in SMARCA4-deficient lung cancer using multiple, non-overlapping hairpins, as well as independent siRNAs, validating the synthetic-lethal relationship between SMARCA2 and SMARCA4 (20-22). We further showed that expression of either SMARCA2 or SMARCA4 completely rescued the effects of SMARCA2 knockdown in SMARCA4-deficient cells indicating paralog dependence and reciprocal role of these two subunits in tumorigenesis. The functional complementation of SMARCA2/4 was also evident at the transcriptional level where the ATPase activity appears to control gene programs related to proliferation, cell cycle and chromatin remodeling. Furthermore, genomic analysis revealed mutual exclusivity of SMARCA2 and SMARCA4 mutations in LUSC and LUAD carcinoma and expression-based biomarker analysis outlined a SMARCA4-deficient patient population predicted to depend exclusively on SMARCA2 activity (Fig. 1). Because SMARCA2-deficient mice are viable showing no overt phenotype (42), while SMARCA4 inactivation is embryonic lethal (43), one might anticipate a significant therapeutic window, if selective small-molecule SMARCA2 inhibitors can be developed that mimic the RNAi knockdown phenotype.
SMARCA2 contains an ATPase and bromodomain suggesting at least two tractable avenues for inhibitor development. Targeting the acetyl-lysine recognition function of SWI/SNF bromodomains (e.g. the SMARCA2/4, PBRM1, BRD7, and BRD9 subunits) represents an unexplored opportunity for perturbation of the SWI/SNF complex. Recently, the anti-cancer activity of BET bromodomains inhibitors has fueled the development of novel chemical scaffolds that selectively target other bromodomains (6,24,25) and the PFI-3 inhibitor exemplifies one such novel chemical probe. However, despite being broadly available from SGC, no phenotypic data has yet been reported. Hence, we subjected PFI-3 to rigorous biochemical and cellular characterization confirming its exquisite selectivity, potency and cell permeability (Fig. 3). Such pharmacodynamics studies are a critical component of drug target validation studies as they provided confidence that a compound-induced phenotype (or lack thereof) correlate with biochemical target engagement in cells.
Surprisingly, in contrast to SMARCA2 knockdown, PFI-3 didn't display any anti-proliferative phenotype in SMARCA4-deficient lung cell lines across a variety of biological assays. Likewise, in models harboring defined SWI/SNF alterations, including synovial sarcoma (SSX-fusion), rhabdoid tumors (SMARCB1-null), and leukemia (SMARCA4-dependent), PFI-3 didn't mimic the anti-cancer phenotype observed upon RNAi-mediated knockdown of SMARCA2/4 (31,38,44). Mechanistically, and consistent with the lack of cellular phenotype, we discovered that PFI-3 cannot displace endogenous SMARCA2 (i.e. lung) or SMARCA4 (i.e. synovial sarcoma) from chromatin potentially due to the activity of other chromatin-interacting SWI/SNF subunits highlighting challenges in targeting large protein complexes. This result is in sharp contrast to efficient chromatin displacement of endogenous BRD4 by JQ1 (Fig. 4C) and we note the contrasting feature of the BET family of tandem bromodomains, which are not flanked by other known regulatory or conserved domains.
Recent studies have highlighted the role of residual SWI/SNF complex along with paralog dependence indicating a potential combinatorial role of chromatin-interacting domains in SWI/SNF recruitment (5). Our data further highlights the need to conduct similar target identification/validation studies of other paralog subunits like ARID1A and ARID1B that form mutually exclusive SWI/SNF complexes and display a synthetic lethal relationship (40). Additional vulnerabilities like antagonism between SMARCB1 and EZH2, which renders rhabdoid tumors dependent on EZH2 for disease maintenance (45), present another promising approach to target SWI/SNF mutant cancers. The anti-proliferative response to SMARCA4 knockdown and EZH2 inhibitor treatment highlights clear dependencies on SWI/SNF activity (Fig. 5E-H; Supplementary Fig. S7A). However, it remains to be investigated whether other SWI/SNF-mutant cancers are sensitive to EZH2 inhibition since we did not see activity of the EZH2 inhibitor in SMARCA4-deficient lung cancer cells (Supplementary Fig. S7B).
For SWI/SNF mutant cancers, our target validation approach has focused on dissecting the functional contribution of an impaired SMARCA2/4 bromodomain or ATPase domain to cellular phenotype through parallel cDNA complementation and rescue experiments. The observation that expression of BRD-Mut, but not ATP-Dead, can rescue the SMARCA2 knockdown phenotype is consistent with the pharmacological PFI-3 inhibitor data. Thus, our genetic and chemical findings converge and we unequivocally conclude that small-molecule inhibition of the bromodomain is dispensable for the ability of the SWI/SNF complex in controlling tumor growth. As such, the present study is the first to deprioritize SMARCA2/4 bromodomain inhibition as a tractable target in genetically defined lung, synovial sarcoma, leukemia and rhabdoid tumors. However, we cannot exclude that compounds with a selectivity profile that simultaneously inhibits additional bromodomains in SWI/SNF (e.g. PBRM1, BRD7, and BRD9 subunits) could be an efficacious strategy (although pleiotropic bromodomain inhibition in normal cells could be a potential concern). While the importance of ATPase activity has previously been shown for chromatin remodeling (20), our studies pinpoint the ATPase activity as the molecular synthetic-lethal target providing a genetically validated strategy for targeting SWI/SNF mutant lung cancer.
ATPases represents a large and diverse family of proteins, many of which perform chaperone-like functions assisting in the assembly, operation and disassembly of protein complexes (46). Not surprisingly, since numerous cellular processes are driven by energy-dependent conformational changes in multi-subunit complexes, ATPases have been implicated in various human diseases with several inhibitors in clinical use. However, most of these don't directly engage/bind the nucleotide-binding site (47). Developing potent inhibitors that must compete with intracellular concentrations of ATP (2–10 mM) have been challenging. Phosphate groups contribute significant nucleotide-binding affinity, but to overcome poor cell-permeability of negatively-charged phosphate groups, the majority of synthetic ATP analogues are devoid of highly charged phospho-mimetic groups (47). Moreover, the high sequence homology between ATP-binding sites (among ATPases and other ATP-binding proteins) represents a profound selectivity challenge. Overall, there is a need for the development of novel, potent and bioavailable ATPase inhibitors, and the successful design of ATP-competitive kinase inhibitors, yet another class of ATP-binding enzymes, supports at least in principal, the feasibility of targeting the ATP-binding site of SMARCA2.
SMARCA2 belongs to the SNF2 family of chromatin remodeling ATPases and contain most of the conserved motifs found in SF2 helicases (48). However, SMARCA2/4 share little overall sequence homology with other helicases and even less homology with other ATPases (46,48). Moreover, the sequence homology of the SMARCA2 ATPase is limited to only two SNF2 clusters in the human genome – related SMARCA proteins and a class of DNA helicases, suggesting possibility for achieving exquisite selectivity over other ATPases. An obvious challenge would be to obtain selectivity over SMARCA4 as these enzymes are highly homogenous in their active site and dual inhibition in normal cells could limit the therapeutic window (29). Structural insights often guide the design of selective inhibitors, however very few X-ray crystal structures for ATPase domains are currently available for SMARCA2/4-related proteins with most being in open inactive conformation, like the yeast Chd1 ATPase domain, highlighting the need for furthering structural biology. Another barrier for pharmaceutical development of selective ATPase inhibitors is the current lack of commercial high-throughput screening assays and selectivity panels against the large family of ATP-binding proteins. Nevertheless, structural diversity in the vicinity of the nucleotide-binding sites, including possible allosteric sites, should enable SMARCA2 ATPase drug discovery supported by prior identification of potent and selective inhibitors of the ATPase activity of KIF11, Hsp90 and VCP (49, 50). The recent development of selective and cell-potent covalent inhibitors that block ATP binding, as well as allosteric inhibitors that impair nucleotide turnover for the VCP ATPase (50) are also an encouraging avenue for the development of inhibitors targeting the SMARCA2 ATPase catalytic activity.
Taken together, our target validation studies identify the SMARCA2 ATPase domain, but not the bromodomain, as a tractable, albeit more challenging therapeutic target for a well-defined SMARCA4-deficient patient population representing more than 20,000 patients a year in US alone (i.e. 10-20% of NSCLC cases). Moreover, the SMARCA4-deficient patient population generally lacks targetable oncogenes (such as mutant EGFR or ALK translocations) (20), which further emphasize the potential medical impact of developing inhibitors of the ATPase domain of SMARCA2/4.
Supplementary Material
Supplementary Figures
Supplementary Text
Supplementary captions
Acknowledgements
The authors wish to thank Dr. Daniel K. Treiber at DiscoveRx for custom assay development; Drs. Norifumi Naka and Kazuyuki Itoh for the Aska and Yamato cells, and Dr. Chang-gong Liu for microarray services.
Footnotes
The authors disclose no potential conflict of interest
Authors’ Contributions.
Conception and design: B. Vangamudi, J.N. Andersen, S. Sharma, D. R. Owen, T. Paul.
Data Acquisition and Analysis: B. Vangamudi, T. Paul, P. Shah, M. Alimova, L. Nottebaum, S. Sharma, D. Verhelle, J.N. Andersen.
Bioinformatics: P. Shah.
Writing, review and/or revision of the manuscript: J.N. Andersen, B.Vangamudi, S. Sharma, W. Palmer, D. Verhelle, P. Shah, T. Paul, M. Alimova, T. Heffernan, J.R. Marszalek, D. Owen, P. Jones.
Study supervision: J.N. Andersen, S. Sharma
Rest of the authors equally contributed to administrative, technical, intellectual, or material support.
References
Full text links
Read article at publisher's site: https://doi.org/10.1158/0008-5472.can-14-3798
Read article for free, from open access legal sources, via Unpaywall:
https://cancerres.aacrjournals.org/content/canres/75/18/3865.full.pdf
Citations & impact
Impact metrics
Citations of article over time
Alternative metrics
Smart citations by scite.ai
Explore citation contexts and check if this article has been
supported or disputed.
https://scite.ai/reports/10.1158/0008-5472.can-14-3798
Article citations
Unlocking cellular plasticity: enhancing human iPSC reprogramming through bromodomain inhibition and extracellular matrix gene expression regulation.
Stem Cells, 42(8):706-719, 01 Aug 2024
Cited by: 0 articles | PMID: 38825983
Chromatin remodellers as therapeutic targets.
Nat Rev Drug Discov, 23(9):661-681, 16 Jul 2024
Cited by: 1 article | PMID: 39014081
Review
New Horizons of Synthetic Lethality in Cancer: Current Development and Future Perspectives.
J Med Chem, 67(14):11488-11521, 02 Jul 2024
Cited by: 0 articles | PMID: 38955347 | PMCID: PMC11284803
Review Free full text in Europe PMC
Targeting SWI/SNF Complexes in Cancer: Pharmacological Approaches and Implications.
Epigenomes, 8(1):7, 04 Feb 2024
Cited by: 2 articles | PMID: 38390898 | PMCID: PMC10885108
Review Free full text in Europe PMC
Degraders in epigenetic therapy: PROTACs and beyond.
Theranostics, 14(4):1464-1499, 27 Jan 2024
Cited by: 1 article | PMID: 38389844 | PMCID: PMC10879860
Review Free full text in Europe PMC
Go to all (126) article citations
Data
Data behind the article
This data has been text mined from the article, or deposited into data resources.
BioStudies: supplemental material and supporting data
Similar Articles
To arrive at the top five similar articles we use a word-weighted algorithm to compare words from the Title and Abstract of each citation.
Dual loss of the SWI/SNF complex ATPases SMARCA4/BRG1 and SMARCA2/BRM is highly sensitive and specific for small cell carcinoma of the ovary, hypercalcaemic type.
J Pathol, 238(3):389-400, 21 Dec 2015
Cited by: 105 articles | PMID: 26356327 | PMCID: PMC4832362
SWI/SNF Complex-deficient Undifferentiated/Rhabdoid Carcinomas of the Gastrointestinal Tract: A Series of 13 Cases Highlighting Mutually Exclusive Loss of SMARCA4 and SMARCA2 and Frequent Co-inactivation of SMARCB1 and SMARCA2.
Am J Surg Pathol, 40(4):544-553, 01 Apr 2016
Cited by: 91 articles | PMID: 26551623
Residual complexes containing SMARCA2 (BRM) underlie the oncogenic drive of SMARCA4 (BRG1) mutation.
Mol Cell Biol, 34(6):1136-1144, 13 Jan 2014
Cited by: 121 articles | PMID: 24421395 | PMCID: PMC3958034
Synthetic lethality: targeting SMARCA2 ATPase in SMARCA4-deficient tumors - a review of patent literature from 2019-30 June 2023.
Expert Opin Ther Pat, 34(3):159-169, 01 Mar 2024
Cited by: 2 articles | PMID: 38578210
Review