Abstract
Free full text

Antibody responses to envelope glycoproteins in HIV-1 infection
Abstract
Antibody responses to the HIV-1 envelope glycoproteins can be classified into three groups. Binding but non-neutralizing responses are directed to epitopes that are expressed on isolated envelope glycoproteins but not on the native envelope trimer found on the surface of virions and responsible for mediating the entry of virus into target cells. Strain-specific responses and broadly neutralizing responses, in contrast, target epitopes that are expressed on the native trimer, as revealed by recently resolved structures. The past few years have seen the isolation of many broadly neutralizing antibodies of remarkable potency that have shown prophylactic and therapeutic activities in animal models. These antibodies are helping to guide rational vaccine design and therapeutic strategies for HIV-1.
The humoral immune response to HIV-1 has been the focus of intense interest since the first identification of the virus in 1983 and the development of antibody (Ab)-based diagnostics of infection. Detailed studies during acute and chronic HIV-1 infection have revealed that Ab responses to HIV-1 infection are complex and evolve over time. The latter characteristic is due to the changing nature of B cell epitopes through mutation during an ongoing viral infection, but it probably also reflects changes in CD4+ T cell help, either because of changes in T cell epitopes through mutation or the elimination of CD4+ T cells through infection. Complexity and change are particularly apparent in Ab responses to the viral surface envelope (Env) glycoproteins, which are by far the most studied and important functional responses and the only ones considered here.
Ab responses to Env proteins fall into three groups. The first group encompasses responses to Env proteins that do not neutralize viruses, even those bearing an Env protein sequence identical to that of the immunizing antigen. Such Abs typically bind to Env epitopes that are not presented on the native Env trimer spike (known as a ‘functional spike’) that mediates entry into target cells (Fig. 1). An inability of an Ab to bind to functional spikes precludes neutralizing activity. ‘Neutralization’ is defined as the loss of infectivity that occurs when an Ab molecule binds a virion and usually does not require the involvement of any other Ab activity. Nevertheless, non-neutralizing Abs to HIV-1 may have antiviral activity because of the relatively unstable nature of the functional spike. Thus, there is evidence that over time, the spike structure decays to reveal epitopes that can be recognized by this class of Abs (Fig. 1). The presence of decayed Env proteins on virions that still possess a sufficient complement of functional spikes to permit infection provides an opportunity for the non-neutralizing Abs to act antivirally—for example, through Fc fragment–mediated phagocytosis of infectious virions or sequestration of virions on Fc receptor (FcR)-bearing cells. A common example of this type of Ab is directed against the immunodominant domain of glycoprotein 41 (gp41) that interacts with the HIV-1 envelope protein gp120 and is hidden from Ab recognition on the Env trimer. If gp120 is shed from the trimer, gp41 is left in the viral membrane as six-helical bundles (known as ‘stumps’), which can be recognized by Abs. Such non-neutralizing Abs can also act antivirally by targeting nonfunctional forms of Env on infected cells (Fig. 1).
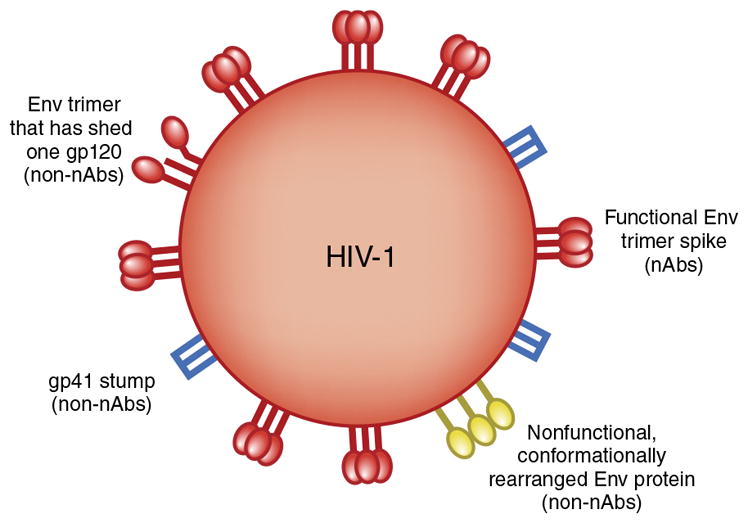
Schematic of some of the forms of Env protein that may be present on infectious HIV-1 and available to elicit Ab responses. Only neutralizing Abs (nAbs) will bind to functional Env trimer spikes, although neutralizing Abs could, in principle, be elicited by other forms of Env protein. A range of non-neutralizing Abs (non-nAbs) and some neutralizing Abs will bind to nonfunctional Env proteins. Non-neutralizing Abs could be elicited by the types of nonfunctional Env protein shown, but also by, for example, monomeric gp120 or Env debris from infected cells. The molecules shown on virions could also be expressed on infected cells. Other forms of Env protein may be expressed on HIV-1 (refs. 1,2,98).
The second group encompasses the responses to Env proteins that neutralize virus in a highly strain-specific manner. These responses typically target regions on the variable (V) loops or other regions of gp120 (Figs. 1 and and2)2) with relatively high sequence variation (such as the C3 region targeted during infection with clade C HIV-1), bind to Env trimers of the immunizing or infecting (‘autologous’) strain of HIV-1 and neutralize that strain but not most other (‘heterologous’) strains. These responses emerge relatively early in infection, tend to be immunodominant and are the mechanism by which HIV-1 continually avoids Ab control, as changes in these variable epitopes readily lead to neutralization escape.
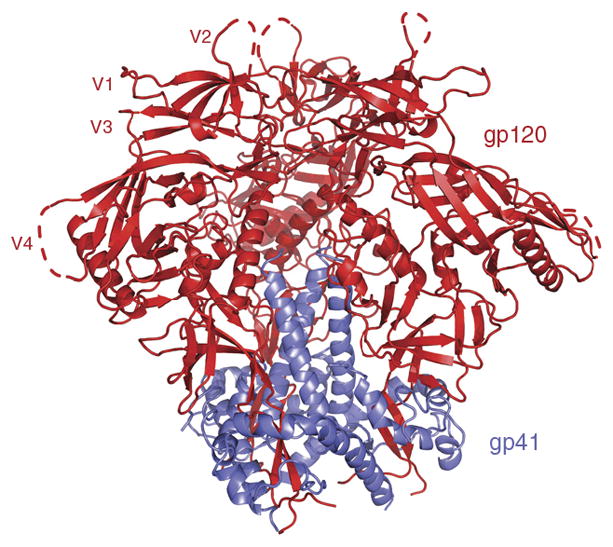
Structure of the HIV-1 Env trimer. Ribbon representation of the viral spike trimer structure (without bound Abs) with three identical gp120 (red) and gp41 (blue) molecules and variable loop regions (V1–V4). Dotted lines indicate loop regions not fully resolved within the crystal structures. The structure of the native cleaved trimeric Env, BG505 SOSIP.664, bound to several neutralizing Abs, has been solved by both cryo-electron microscopy19 and X-ray crystallography18,20. Image adapted from ref. 20, Nature Publishing Group.
The third group is represented by responses to Env proteins that neutralize a broad spectrum of global HIV-1 strains. Here we are referring to Abs that can neutralize the majority of diverse HIV-1 strains (i.e., that have breadth of coverage) with in vitro neutralization potencies often well below 1 μg per milliliter (i.e., an IC50 (the Ab concentration needed to achieve 50% neutralization in standard assays) of less than 1 μg/ml). These responses target regions of the Env spike that show relative conservation either in sequence or amino acid character. Such Abs usually have evolved mechanisms to deal with the variability that surrounds the small oases of accessible conservation on the Env trimer. The Abs typically take years to evolve a high level of neutralization breadth and potency and have an array of features not found in most Ab responses. Induction of these types of Ab response—those that attain both neutralization potency and breadth—would be the goal of vaccination to protect against the diversity of global strains. Here we discuss these three types of Ab response in detail.
HIV-1 Env antigens
Env proteins are believed to be presented to the human humoral immune system in many forms during natural HIV-1 infection, including as functional Env trimers on the surface of virions and infected cells, disassembled or conformationally altered Env trimers, shed monomeric gp120, gp41 stumps left in membranes after gp120 is shed, and aggregated gp160 from the cytoplasm of infected cells1,2. In addition, gp120 can bind to the monomeric coreceptor CD4 on the cell surface, which leads to the enhanced exposure of additional epitopes, such as the CD4-induced epitope, a phenomenon that has been observed in HIV-1 infection3 and in gp120-immunization experiments4. Given these different forms of Env protein and the instability of the native trimer, it may be difficult to be dogmatic about the conformation of the immunogen that has triggered or contributed to a given anti-Env response. That said, there is a subset of Abs that are Env-trimer specific (i.e., bind to conformationally intact trimers but not to gp120) (refs. 5–11), and elicitation of these Abs would probably require immunization with trimers at some point. Furthermore, Abs to epitopes hidden within the trimer would not be induced by immunization with a stable trimer. However, many epitopes are presented on multiple forms of Env protein (for example, both gp120 and trimer), which complicates understanding of the genesis of such responses.
Much structural work has been done on various forms of gp120 and gp41, often in complex with Abs, and these studies, together with Ab mapping by both binding and neutralization, have defined many Env epitopes12–14. More recently, structures of a recombinant stabilized form of the Env trimer (Fig. 2) thought to closely mimic the functional trimer15–17 have been determined18–20, and these have revealed much about the nature of Env epitopes and the corresponding Ab responses. In brief, the hidden or obstructed nature of many epitopes on the trimer that are available on monomeric gp120 is apparent. This observation is undoubtedly associated with the prevalence of many non-neutralizing Abs in Env responses. On the fully functional viral spike, neighboring protomers (for example, gp120s) and glycans obstruct access to many gp120 epitopes. gp41 is largely buried in the interior of the trimer. The relative accessibility of the variable loops targeted by strain-specific neutralizing Ab responses is clear on the structures and contrasts, for example, with the limited access of the CD4-binding site, which is targeted by a subset of broadly neutralizing Ab responses.
Env protein–binding but non-neutralizing Ab responses
Serological assessment of Ab responses to HIV-1 has focused on Abs that target the glycoproteins gp120 and gp41, which noncovalently associate and trimerize to form the Env viral spike14. Early binding assays most often used monomeric gp120 or soluble forms of uncleaved (non-native) trimeric gp140, typically in an enzyme-linked immunosorbent assay format. Use of these antigens enables the detection of anti-Env immunocomplexes as early as 1 week after HIV-1 infection21. Subsequently, Abs to gp41 are detected first, followed by Abs to variable regions of gp120, such as the V3 loop. These binding Abs do not seem to produce selection pressure on the virus or affect plasma viral load22,23. Because functional trimeric Env proteins are prone to disassembly, as described above, it is likely that a large fraction of the gp120 and gp41 response is directed to dissociated gp120 and gp41 proteins and does not react with native Env trimers24. This might partially explain the apparent lack of in vivo activity of these early Ab responses.
However, it is likely that some non-neutralizing Abs can act antivirally through Fc-mediated effector activities, so these are of functional interest. Immunoglobulin G (IgG) can interact with transmembrane adaptors (FcγRs), the neonatal Fc receptor (FcRn) and the initiator of the classical complement cascade C1q. The interaction of Abs bound to Env proteins on cells with FcγRs can trigger Ab-dependent cellular cytoxicity (ADCC) and Ab-mediated cellular phagocytosis (ADCP). In early assay formats, ADCC was often assessed by coating CD4+ target cells with monomeric gp120, which measures only a subset of Abs reactive with gp120. Subsequent ADCC assays have used HIV-1-infected target cells that express native Env proteins on their surface and thus provide a more physiological assessment of possible antiviral effects in vivo25–28. A further assay used in vitro is Ab-dependent cell-mediated virus inhibition, which provides a measure of the combined antiviral effect of ADCC, neutralization and other possible inhibitory mechanisms of Ab in vitro29. ADCC and ADCP are initiated by interactions between Ab Fc regions with FcγRs on cells of the innate immune system. ADCC occurs via natural killer effector cells, whereas ADCP is mediated by monocytes, macrophages or dendritic cells that can internalize Ab-coated virus or cells. FcγR-mediated effector functions are dependent on IgG subclass and Fc glycosylation. As an example, IgGs lacking Fc glycan fucose residues mediate ADCC more effectively than do those containing fucose. Additionally, FcγRs are subclassified as activating (FcγRI, FcRγIIa, FcγRIIc, FcγRIIIa and FcγRIIIb) or inhibitory FcγR (FcγRIIb), and these are activated differentially by different Ab glycoforms30. For example, the addition of terminal galactose or sialic acid residues to the IgG glycans can promote anti-inflammatory activity by enhancing the interaction with the inhibitory FcγRIIb. Thus, in addition to the conventional measurements of Ab binding, biochemical and binding analyses now include glycan analysis by mass spectrometry, measurements of the affinity of the binding of Ab Fc to FcγRs and Fc-mediated effector functions such as ADCC. An analysis of the Ab-glycosylation profile during HIV-1 infection has suggested that HIV-1-infected subjects who control viremia and show strong Fc-mediated antiviral activity have an HIV-1-specific Ab-glycosylation profile shifted toward agalactosylated glycoforms that are better able to recruit antiviral activity of the innate immune system31.
It is worth emphasizing that Fc-mediated effector functions and virus neutralization are not mutually exclusive; thus, neutralizing Abs may similarly trigger Fc-mediated effector functions32,33. Interest in non-neutralizing Abs has been driven in part by the results of the RV144 vaccine study, which showed a 31% reduction in infection rate despite the absence of detectable HIV-1-neutralizing Abs elicited by the vaccine34. An immune-correlates analysis has associated non-neutralizing Abs to the V1V2 region of gp120 with a lower risk of infection35, and these Abs mediate ADCC under certain experimental conditions36. Additionally, Abs of the IgG3 isotype, which are potent mediators of ADCC, are associated with lower infection risk37. In addition to their potential role in clearance of virally infected cells, Abs generated by immunization can be present at mucosal surfaces, as IgG or IgA, and could interact with a virus to impair its ability to breach the mucosal barrier. Such Abs could act via aggregation of virions and, potentially, by interacting with mucosal secretions (e.g., mucins) to trap and clear virions away from the mucosal surface38–40. IgA can also prevent transcytosis across epithelial cells and Abs at subepithelial or submucosal layers could recruit cells of the innate immune system to mediate phagocytosis, viral inhibition or ADCC41.
Although some data have associated strong Ab-dependent cell-mediated virus inhibition responses with slower disease progression in long-term nonprogressors42,43, one of the major concerns with Env protein–binding but non-neutralizing Abs from a physiological standpoint is the lack of convincing evidence of protection44 or therapeutic activity in animal models under conditions for which neutralizing Abs provide solid antiviral activity, including complete protection against infection and complete suppression of virus in infected animals45–52. It has been suggested that non-neutralizing Abs may show effects only in an appropriate polyclonal composition, but no such composition has yet been identified44.
Strain-specific neutralizing Ab responses
For analysis of neutralizing Ab responses to functional native Env proteins, cell-entry-competent Env-pseudoviruses are often used. Here an Env protein–deficient HIV-1 molecular clone is pseudo-typed with a full gp160 Env protein to express a single round of replication Env-pseudovirus that can enter host cells expressing CD4 and the coreceptor CCR5. This allows accurate measurement of the neutralization of viruses incorporating autologous Env proteins from the same donor as the serum sample, or diverse heterologous HIV-1 strains53,54. With these assays, autologous virus-neutralizing Abs are generally detected weeks or months after Abs that bind gp120 or gp41 and are generally not detected until 12 or more weeks of HIV-1 infection14,21,55,56. The reasons for the slow development of autologous or strain-specific neutralizing Ab responses are unclear. It is possible that impaired CD4+ T cell help may have a role or that Abs to non-neutralizing epitopes are dominant and thus delay the response to neutralizing epitopes found on the native trimer. As their name implies, such responses neutralize very narrowly—typically only the autologous virus of those tested.
The escape of HIV-1 from neutralization by strain-specific neutralizing Ab responses was first documented more than 15 years ago57,58 and has been described in detail with Env proteins cloned from plasma to express autologous Env-pseudoviruses55,56. Hence, at any given time point during the course of HIV-1 infection, most circulating viruses are resistant to neutralizing Abs in serum from the same time point. Subsequently, neutralizing Abs in serum that can neutralize the escaped virus arise, and an ongoing process of viral escape and Ab evolution is believed to drive, in some individuals, the development of cross-reactive neutralizing Abs59–62. Strain specificity was initially thought to be explained mainly by neutralizing Abs that target highly variable regions of Env proteins, such as V1V2 or V3, and this is the case in some donors (Fig. 2). In clade C infection, the moderately variable α2 helix region that is C-terminal to V3 is a target of the early autologous neutralizing Ab response60,61. Likewise, several studies have shown that the V1V2 region is also targeted early60–62. Common themes among these descriptions are that the early Ab response is highly restricted (to the autologous virus and perhaps a few others) but is able to drive viral escape mediated by mutation of one amino acid or a few amino acids. Thus, one prevailing hypothesis for the development of serological neutralization breadth is that the accumulation of a large number of strain-specific neutralizing Abs results in a polyclonal response that can neutralize many diverse HIV-1 strains. Although the polyclonality of sera certainly has some role in neutralization breadth, many published studies have suggested that effective broad neutralization by sera from infected donors results from the activity of potent broadly neutralizing Abs of a small number of neutralizing Ab specificities, rather than a combination of many strain-specific Abs5,6,63–67.
Broadly neutralizing Ab responses
Over the past 10 years, major efforts have been made to delineate the polyclonal serum response to HIV-1 to understand particularly the epitopes on Env proteins targeted by broadly neutralizing Abs that develop during natural infection68. Initially, this was done by adsorbing sera with specific antigens such as gp120 and gp140, or mutant variants of these proteins, or by using Env-pseudoviruses that contain mutations or chimeric sequence exchanges in specific regions of Env proteins63–65,69. These approaches have provided key insights into the neutralization epitopes recognized by HIV-1-positive sera, but more definitive information would come from the isolation of neutralizing monoclonal Abs (mAbs) from infected donors whose sera has demonstrated potent cross-reactive HIV-1 neutralization. Two principal factors have facilitated the isolation of large numbers of potent broadly neutralizing Abs. First, large cohorts have been examined for the breadth of serum neutralization to give the best chance of recovery of broadly neutralizing Abs10,70,71. Second, effective methods for the reliable isolation of broadly neutralizing Abs from HIV-1-infected donors have been adopted, including the ability to culture and activate individual memory B cells and directly screen supernatants for neutralization5–7,9,10,72 and to isolate single B cells directly by antigen-specific cell sorting73–75. The ability to then recover genes encoding Ab heavy or light chains from individual B cells allows the reconstruction of the functional Ab. Before 2009, only a handful of broadly neutralizing Abs had been isolated, and these defined three major Env antigenic sites: the membrane-proximal external region (MPER) of gp41, the CD4-binding site of gp120 and an exclusively glycan epitope on the outer domain of gp120. mAb-isolation methods have enabled the isolation of hundreds of broadly neutralizing Abs over the past 5 years, many of which have demonstrated remarkable potency and breadth of reactivity against diverse HIV-1 strains. Newly identified antigenic sites include a gp120 V2-glycan site at the apex of the Env trimer, a gp120 V3-glycan site centered on the glycan at Asn332 and an extended region including residues from both gp120 and gp41 between the MPER and gp120 protomers (Fig. 3).
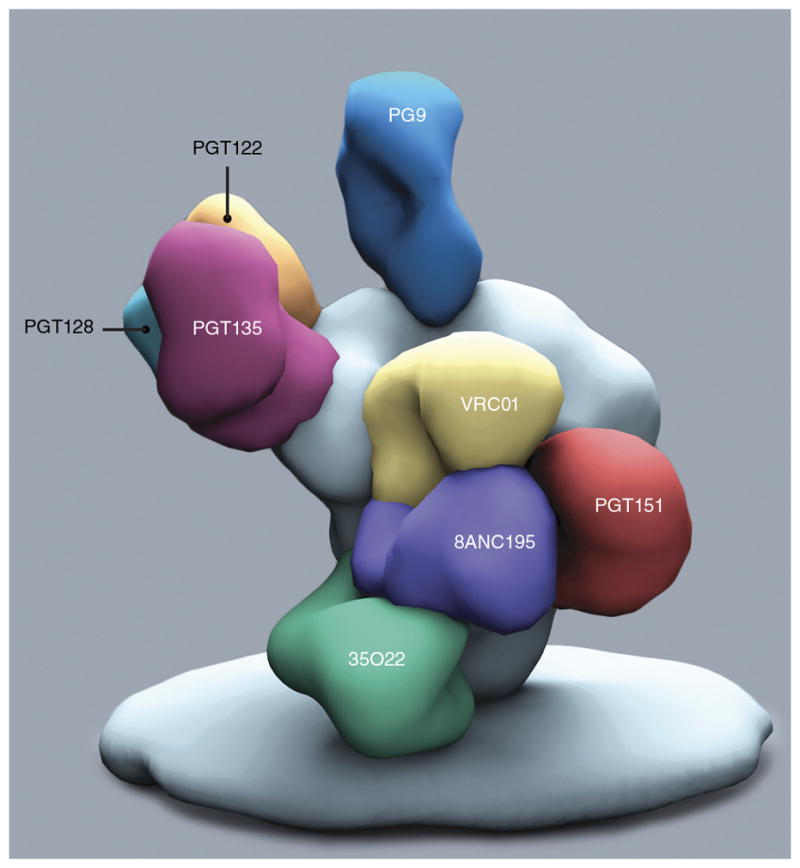
Broadly neutralizing Abs (antigen-binding (Fab) fragments) bound to the HIV-1 Env trimer. Shown are binding locations for prototype Abs to gp41 and gp120 (35O22 (ref. 10), 8ANC195 (ref. 99), PGT151 (refs. 7,8)); to the CD4-binding site (VRC01 (ref. 74)); to high-mannose-patch (PGT122, PGT128, PGT135 (refs. 6,18,100–102)); and to V2 apex (PG9 (refs. 5,103)). The Abs to MPER72,104,105 bind very close to the viral membrane; the MPER is not included in the recombinant trimer structure18. Other much-studied broadly neutralizing Abs include b12 (ref. 106) and 3BNC117 (Abs to the CD4-binding site)107; 10-1074 (Ab to the high-mannose patch)108; CAP256 (ref. 9), PGT145 (ref. 6) and PGDM1400 (ref. 73) (Abs to the V2 apex). Image courtesy of A. Ward and C. Corbaci.
A great deal of effort in the past few years has gone into studying the broadly neutralizing Abs23,59,76–83. Characteristics of note include higher levels of somatic hypermutation than those typically observed for Abs to many other pathogens (particularly the VRC01 class of broadly neutralizing Abs directed to the CD4-binding site), the frequent use of insertions and deletions, long heavy-chain complementarity-determining region 3 regions (particularly the broadly neutralizing Abs that recognize protein-glycan epitopes requiring penetration of the glycan shield, such as the V2 apex and high-mannose patch epitopes) and restricted germline use (particularly the VRC01 class of broadly neutralizing Abs directed to the CD4-binding site)84–86. One important question about broadly neutralizing mAbs is whether a high degree of somatic hypermutation and frequent insertions and deletions are necessary for broad neutralization or whether they simply reflect long-term infection and an extended period of Ab mutation and selection. A number of studies suggest that considerably lower levels of somatic hypermutation than typically observed in broadly neutralizing Abs can indeed yield relatively broad and potent neutralization9,87,88. Some insertions and deletions seem to be required for broad neutralization; others do not85,86. Another important question is the origin of long heavy-chain complementarity-determining region 3 regions. Data suggest that these features are generated mainly during variable-diversity-joining recombination before antigen contact rather than via insertions after antigen contact9,89.
A range of broadly neutralizing mAbs has been shown to provide sterilizing immunity against challenge with chimeric simian-human immunodeficiency viruses (SHIVs) in macaque monkeys and against HIV-1 in humanized mice45–48,83,90. Early studies showed a strong correlation between in vitro neutralization and in vivo protection45, and titration studies have indicated that sterilizing immunity is achieved at serum Ab concentrations in the approximate range of tenfold to a few hundred-fold in vitro serum neutralizing titers48,90–93. Evidence in macaques32,94 and in humanized mice33,90 has accumulated showing that Fc receptor–mediated activities are important in the protective activity of broadly neutralizing Abs against HIV-1 challenge. Notably, potent broadly neutralizing mAbs have dramatic effects on the control of virus in established infection in humanized mice and macaques50,52,95. Escape from neutralization has been described in a number of instances, typically less frequently in combination therapies than in monotherapy47,95. Remarkably, the single mAb PGT121 has produced sustained suppression in some macaques and led to an absence of escape from neutralization50. The presence of pre- existing Ab responses in infected animals may help to block escape pathways96. The sustained nature of control in some animals may reflect enhanced T cell responses after Ab therapy50.
Several groups of investigators have studied the lineages of broadly neutralizing Abs by first isolating a broadly neutralizing mAb from memory B cells after several years of HIV-1 infection. Through the use of knowledge of the Ab sequence and next-generation sequencing to identify related gene transcripts, the maturation of the Ab lineage can be followed over time in longitudinal B cell samples starting early after infection. In one example, the mAb CH103 targeted the CD4-binding site of gp120 and neutralized 55% of diverse HIV-1 strains88. However, the unmutated ancestor of this Ab lineage bound only to the gp120 of the autologous founder virus, and early intermediates showed strain-specific autologous virus neutralization. During the process of coevolution of virus and Ab, the maturing CH103 Ab lineage gained neutralization breadth, presumably through focusing on conserved features of the CD4-binding-site epitope that are common to most viruses97. A similar phenomenon was observed with the lineage of an Ab to the quaternary V1V2 region of the Env trimer9. The mature Ab CAP256 that was isolated 2 years after HIV-1 infection was both highly potent and relatively broadly neutralizing. However, its unmutated ancestor showed only weak neutralization of the founder virus, with both potency and breadth of neutralization increasing over time.
Conclusions
We began with the statement that Ab responses to HIV-1 are complex. Study of that complexity has yielded a wealth of information on the viral Env protein and on Abs that can recognize it. Researchers now appreciate the full range of defensive features that the HIV-1 Env trimer incorporates to minimize recognition by Abs, as seen in the elegant published structures. Investigators also appreciate the power of evolutionary forces to yield Abs capable of penetrating the defenses of Env protein and are beginning to understand how these Abs arise in natural infection. Some of the broadly neutralizing mAbs that have been generated are so potent, and their activity against diverse global isolates is so extensive, that they are now being considered as prophylactic and therapeutic agents, including being part of strategies to attempt to target the latent reservoir of HIV-1. Finally, the broadly neutralizing Abs suggest that a vaccine against HIV-1 should be possible, if researchers can meet the challenge of designing immunogens to elicit such Abs.
Acknowledgments
Supported by the National Institute of Allergy and Infectious Diseases (D.R.B.), the Center for HIV/AIDS Vaccine Immunology and Immunogen Discovery (D.R.B.), the International AIDS Vaccine Initiative (D.R.B.), the Bill and Melinda Gates Foundation (D.R.B.), the Ragon Institute of MGH, MIT and Harvard (D.R.B.) and the intramural research program of the Vaccine Research Center, National Institute of Allergy and Infectious Diseases (J.R.M.).
References
Full text links
Read article at publisher's site: https://doi.org/10.1038/ni.3158
Read article for free, from open access legal sources, via Unpaywall:
https://europepmc.org/articles/pmc4834917?pdf=render
Citations & impact
Impact metrics
Citations of article over time
Alternative metrics
Smart citations by scite.ai
Explore citation contexts and check if this article has been
supported or disputed.
https://scite.ai/reports/10.1038/ni.3158
Article citations
Beyond glycan barriers: non-cognate ligands and protein mimicry approaches to elicit broadly neutralizing antibodies for HIV-1.
J Biomed Sci, 31(1):83, 21 Aug 2024
Cited by: 0 articles | PMID: 39169357 | PMCID: PMC11337606
Review Free full text in Europe PMC
Comparison of the immunogenicity of mRNA-encoded and protein HIV-1 Env-ferritin nanoparticle designs.
J Virol, 98(9):e0013724, 13 Aug 2024
Cited by: 0 articles | PMID: 39136461
mRNA-based HIV-1 vaccines.
Clin Microbiol Rev, 37(3):e0004124, 17 Jul 2024
Cited by: 0 articles | PMID: 39016564
Review
High frequency of HIV precursor-target-specific B cells in sub-Saharan populations.
Cell Rep, 42(12):113450, 28 Nov 2023
Cited by: 0 articles | PMID: 38019653 | PMCID: PMC10886445
HIV-1 envelope diversity and sensitivity to broadly neutralizing antibodies across stages of acute HIV-1 infection.
AIDS, 38(4):607-610, 16 Nov 2023
Cited by: 1 article | PMID: 38416554 | PMCID: PMC10906214
Go to all (280) article citations
Similar Articles
To arrive at the top five similar articles we use a word-weighted algorithm to compare words from the Title and Abstract of each citation.
Conformational Epitope-Specific Broadly Neutralizing Plasma Antibodies Obtained from an HIV-1 Clade C-Infected Elite Neutralizer Mediate Autologous Virus Escape through Mutations in the V1 Loop.
J Virol, 90(7):3446-3457, 13 Jan 2016
Cited by: 23 articles | PMID: 26763999 | PMCID: PMC4794693
Consistent elicitation of cross-clade HIV-neutralizing responses achieved in guinea pigs after fusion peptide priming by repetitive envelope trimer boosting.
PLoS One, 14(4):e0215163, 17 Apr 2019
Cited by: 32 articles | PMID: 30995238 | PMCID: PMC6469787
Closing and Opening Holes in the Glycan Shield of HIV-1 Envelope Glycoprotein SOSIP Trimers Can Redirect the Neutralizing Antibody Response to the Newly Unmasked Epitopes.
J Virol, 93(4):e01656-18, 05 Feb 2019
Cited by: 47 articles | PMID: 30487280 | PMCID: PMC6363999
Challenges for structure-based HIV vaccine design.
Curr Opin HIV AIDS, 4(5):431-440, 01 Sep 2009
Cited by: 76 articles | PMID: 20048708
Review
Funding
Funders who supported this work.
Intramural NIH HHS
NIAID NIH HHS (1)
Grant ID: UM1 AI100663