Abstract
Free full text

Growth hormone actions during development influence adult phenotype and longevity
Abstract
There is considerable evidence that exposure to undernutrition, overnutrition, stress or endocrine disruptors during fetal development can increase the probability of obesity, hypertension, cardiovascular disease and other problems in adult life. In contrast to these findings, reducing early postnatal growth by altering maternal diet or number of pups in a litter can increase longevity. In hypopituitary Ames dwarf mice, which are remarkably long lived, a brief period of growth hormone therapy starting at 1 or 2 weeks of age reduces longevity and normalizes (“rescues”) multiple aging-related traits. Collectively, these findings indicate that nutritional and hormonal signals during development can have profound impact on the trajectory of aging. We suspect that altered “programming” of aging during development may represent one of the mechanisms of the Developmental Origins of Health and Disease (DOHaD) and the detrimental effects of “catch-up” growth.
Interest in the role of early life events in shaping developmental trajectories and adult phenotypes dates far back into the history of experimental biology. It includes studies of environmentally induced phenocopies [1–3] and concepts of the channels of development and the epigenic landscape proposed by Conrad Waddington in the 1940s [4]. More recent work focused attention on the role of maternal undernutrition, overnutrition and stress on the incidence of obesity, metabolic syndrome and chronic diseases in the offspring. Studies in this area started with the demonstration that starvation during pregnancy and reduced birth weight of human infants were associated with increased risk of hypertension, cardiovascular disease and diabetes [5–7]. Experimental studies of the effects of reducing food availability during pregnancy in sheep and rats on the adult phenotypic characteristics of the offspring confirmed these observations and implicated epigenetic mechanism including altered DNA methylation in mediating the effects of maternal nutritional status on disease risk factors in their adult progeny [7–11]. Much of the recent work in this area was undertaken in an effort to identify the mechanisms behind the alarming increase in the incidence of obesity in both technologically advanced and developing countries during the past few decades. Although polymorphism of numerous genes has been related to human obesity, this vastly important public health issue is emerging too rapidly to be explained by purely genetic mechanisms.
Results available to date indicate that multiple environmental factors including maternal undernutrition and, curiously, overnutrition as well as maternal stress and exposure to a variety of chemicals, including endocrine disruptors, during pregnancy and lactation modulate obesity risk in the offspring [12–15]. In the context of this article, it is important to emphasize that nutritional interventions, as well as stress, can produce multiple alterations in endocrine signaling and that obesity, metabolic syndrome and chronic disease are intricately linked to the mechanisms of aging and to the control of longevity. Ongoing studies in our laboratory are aimed at elucidating the role of growth hormone (GH) during development in the control of aging and our key findings will be presented and discussed in this article.
I. Growth hormone and aging
Studies in mice in which spontaneous mutations or experimental gene disruption interfere with biosynthesis or action of GH established a major role of this hormone in the control of aging and longevity. Animals lacking GH due to abnormal development of the anterior pituitary or to the absence of hypothalamic GH releasing hormone and animals with GH resistance produced by disruption of GH receptors exhibit remarkable and reproducible extension of longevity in both females and males [16–19]. Importantly, increase of the lifespan in these mutants is associated with multiple indices of delayed and/or slower aging including maintenance of youthful levels of cognitive function into advanced age [20,21] and delayed onset of fatal neoplastic disease [22–24].
Studies in several laboratories identified multiple candidate mechanisms for the extensions of healthspan and lifespan of GH-related mutants. These include improved anti-oxidant defense mechanisms, stress resistance, reduced chronic low-grade inflammation in the adipose tissue and in the central nervous system, reduced mTOR signaling, reduced cell senescence and improved maintenance of stem cells and multiple metabolic adjustments [25,26]. Among the metabolic alterations in these mutants, enhanced insulin sensitivity (combined with reduced insulin levels) [25–27] increased thermogenesis and oxygen consumption [19,28] and increased reliance on fatty acids as metabolic fuel [28] attracted our particular attention as potential anti-aging mechanisms.
Interestingly, the inverse relationship of longevity to GH/IGF-1 signaling and somatic growth discovered in mutant, gene “knockout” and transgenic mice was also found in comparisons of different strains of genetically normal mice [29] and in studies of differences in adult body weight and longevity in individual mice from a normal non-inbred population [30,31]. Moreover, activity of the somatotropic axis and body size are inversely related to longevity in other mammalian species with different breeds of domestic dogs providing the most striking examples [32,33]. In humans, the impact of GH on aging is somewhat less clear and controversial, but lower circulating IGF-1 levels, mutations interfering with the function of IGF-1 receptors and shorter stature have all been related to improved old age survival and increased longevity [34–37]. Moreover, individuals with mutations interfering with GH biosynthesis or action were found to be significantly less likely to develop cancer, diabetes or atherosclerosis [38,39].
2. Is aging related to GH actions at particular stages of the life history?
The very striking survival benefits of mutations blocking GH synthesis or actions brought up the question of why these or similar mutations have not been naturally selected for thousands of years ago. One of the possible explanations that has been suggested by us and others posits that the promotion of somatic growth, sexual maturation and fecundity by GH would serve to enhance evolutionary fitness (i.e. probability of producing and raising offspring), while the life shortening effects of the somatotropic axis (such as acceleration of aging, inducing insulin resistance and promoting cancer) would have little impact on the reproductive success under natural conditions. If correct, this explanation would imply that GH has predominantly beneficial effects during development and early adulthood and detrimental (“pro-aging”) effects later on. Such relationship between early life and late life effects of GH would fit the concept of “antagonistic pleiotropy” which features prominently in the current understanding of the relationship of aging to evolutionary processes. Effects of GH therapy in genetically dwarf rats support this line of reasoning by demonstrating a delicate balance between the presumably beneficial effects of GH on development and the impact of GH and GH-dependent IGF-1 during adulthood on the burden of age-related disease including incidence of tumors [40].
Another question stemming from the evidence that hypopituitary Prop1df and Pit1dw mice, as well as GH-deficient Ghrh−/− and GH-resistant Ghr−/− mice, live much longer than their normal siblings [16–19] concerns the possible importance of reduced postnatal growth and dwarf phenotype in the determination of the rate of aging and the lifespan. Under this scenario, GH stimulation of early life growth including the peri-pubertal growth spurt would be exerting pro-aging effects and absence of this stimulatory input in genetically dwarf mice would explain, or at least contribute to their delayed aging and extended longevity. To test this hypothesis, two laboratories tested the effects of early GH therapy on longevity of hypopituitary mice. Vegara et al. reported that treating Snell dwarf mice with daily GH or GH plus thyroxine injections starting at 4 weeks of age and continuing for 11 weeks had the expected stimulatory effect on growth and sexual maturation but did not alter longevity [24]. Our initial studies of the effects of treating juvenile Ames dwarf mice with GH also failed to affect longevity. However, when we changed the protocol for one group of male Ames dwarfs, starting GH therapy at two rather than 3 or 4 weeks of age and injecting GH twice rather than once per day, we obtained a striking reduction of longevity of GH treated vs. control dwarf mice [41]. More recently, we were able to reproduce this result and to show that early GH therapy shortens longevity in both sexes of Ames dwarf mice (Sun, et al., unpublished). In these studies, the 6 weeks course of GH treatment starting at 1 rather than 2 weeks of age was similarly effective in reducing longevity of the dwarfs, and injecting juvenile normal mice with an identical dose of GH (on the body weight basis) had no or very little impact on their longevity (Sun, et al., unpublished).
Unexpectedly, concomitant treatment of juvenile Ames dwarf mice with thyroxine severely reduced (in females) or eliminated (in males) the effect of early GH treatment on longevity [42]. Treatment with thyroxine alone during the same period (between 2 and 8 weeks or age) had no significant impact on longevity [42] and 11 weeks of treatment of Snell dwarfs starting at 4 weeks of age similarly failed to affect their longevity [24]. These findings were counterintuitive. Both Ames and Snell dwarf mice are hypothyroid due to congenital TSH deficiency [43–45] and hypothyroidism is suspected to contribute to their delayed and/or slower aging by reducing body temperature [46] and likely through other mechanisms. Earlier studies have shown that in rats longevity can be extended by experimentally induced hypothyroidism and reduced by chronic thyroxine treatment [47,48]. Snell dwarf mice injected with thyroxine or a combination of thyroxine and GH for 11 weeks starting at 4 weeks of age and given food supplemented with thyroid powder for the rest of their lives lived shorter than control dwarf animals [24].
The mechanisms responsible for the differential impact of early treatment with GH alone or GH plus thyroxine on longevity of Ames dwarf mice remain to be elucidated. One could speculate that the treatment with thyroxine accelerated maturational changes in the brain and possibly other tissues and that this reduced the sensitivity to GH actions or prematurely closed the “time window” of the developmental programming of aging. Analysis of tissues collected one day after completing the treatment of juvenile mice with GH or GH plus thyroxine indicated that administration of thyroxine significantly attenuated the stimulatory impact of GH on the expression of IGF-1, Akt2, Glut4 and STAT-1 in the skeletal muscle, and IGF-1 in periovarian white adipose tissue (pWAT). Moreover, thyroxine treatment enhanced the suppressive effect of GH on the pWAT expression of IR, PI3K, PPARy, STAT-5b and FOXO3 and reduced the expression of adiponectin [42]. We do not know whether these effects persisted when animals were no longer treated with GH or GH + thyroxine.
3. Can treatment of juvenile dwarf mice with GH normalize (“rescue”) their adult phenotype?
Results obtained in Ames dwarf mice treated with GH during their development indicate that similarly to what is known about early origins of obesity, metabolic syndrome and other risk factors for chronic disease, the biological process of aging and the determination of longevity are profoundly influenced by early life “programming” and that GH is among the early signals involved in this process. In an attempt to identify mechanisms mediating the effects of early GH therapy on aging, we are currently analyzing adult phenotypic characteristics of animals that had been treated with GH during their development (Fig. 1). We are particularly interested in the characteristics that are known to be involved in the control of aging or have been identified as putative mechanisms of extended longevity of Ames dwarf mice.
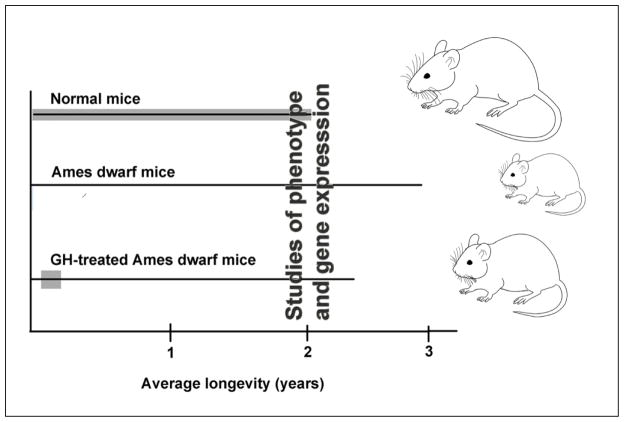
Outline of studies aimed at identifying mechanisms linking GH signaling during development with longevity.
Reduced levels of IL-6 and increased levels of adiponectin in blood plasma of Ames dwarf mice [49] indicate that these animals are somehow protected from a chronic low-grade inflammation that is believed to be among the key mechanisms of aging. Moreover, expression of pro-inflammatory cytokines is reduced in the adipose tissue and in the brain of these animals [49,50 and unpublished data]. Collaborative studies with Dr. Sadagurski in the Richard Miller laboratory, recently provided evidence that astrogliosis and microglial expression of tumor necrosis factor α (TNF-α) are profoundly suppressed in the hypothalamus of Ames dwarf as compared to normal mice and that these changes are completely prevented in animals that had been treated with GH during their development [51]. Importantly, the animals used in this study were examined at 18 months of age; that is 16 months after the GH treatment was completed. The examined markers of hypothalamic inflammation did not differ between dwarfs injected with saline vehicle alone and those left untreated, indicating that the effects of early GH therapy were not due to the stress of twice-daily injections. We suspect that the normalization (“rescue”) of the adult levels of hypothalamic inflammation by early GH therapy may have contributed to reduced longevity of animals given identical treatment because inflammatory tone in this part of the brain has been convincingly linked to the control of aging [52,53].
Other adult phenotypic characteristics that are suspected of involvement in the control of aging that are drastically altered in Ames dwarfs and completely or partially normalized by the early therapy include increased oxygen consumption per unit of body mass (Fig. 2), reduced respiratory quotient signifying a shift in mitochondrial energy substrates preference from carbohydrates to fatty acids, and alterations in the hepatic expression of genes related to stress responses and xenobiotic metabolism, as well as resistance to the detrimental effects of high-fat diet on glucose homeostasis (Sun, et al, unpublished data and Hill et. al, unpublished).
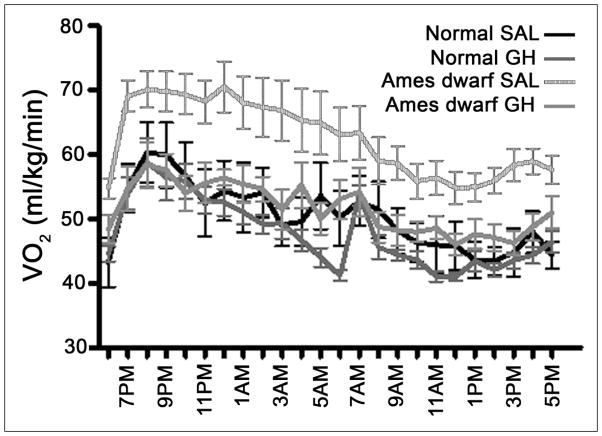
Oxygen consumption (VO2) measured at 9 months of age in normal and Ames dwarf mice that had been treated with growth hormone (GH) or saline vehicle (SAL) between 2 and 8 weeks age, Six weeks of treatment with GH during development normalizes adult oxygen consumption.
These findings identify candidate mechanisms responsible for reduced longevity of Ames dwarfs treated with GH during their pre-and peri-pubertal development. Further work will be necessary to identify molecular and cellular (presumably epigenetic) changes that are induced by GH during development, persist into adult life, perhaps permanently, and interfere with the remarkable extension of longevity that is characteristic of these mutants.
4. Nutritional interventions during development can influence aging–possibly via endocrine mechanisms
It is now well established that altered availability of nutrients during development can alter the risk of adult disease. As was mentioned earlier in this article, nutritional factors with the best documented negative impact on adult health include maternal undernutrition during pregnancy leading to reduced birth weight, disproportion between the availability of nutrients during postnatal vs. fetal development (as embodied in the “thrifty phenotype hypothesis”) and overnutrition, including maternal obesity, gestational diabetes and macrosomia [5–11].
Longevity can also be affected by nutritional interventions. Offspring of female mice fed a low-protein diet were shown to be long-lived [54]. We have previously reported that a modest reduction in nutrient availability during nursing produced by experimentally increasing the number of pups in a litter (“litter crowding”) can cause a significant increase in longevity [55]. Involvement of endocrine pathways in mediating the effects of nutritional interventions on the trajectory of aging can be suspected from the major impact of nutritional status on IGF-1 and insulin levels, on hepatic sensitivity to GH and on whole animal insulin resistance. To address the suspected interaction of reduced early postnatal nutrition and suppressed somatotropic signaling, we decided to examine the effects of litter crowding on the longevity of GH-resistant Ghr−/− mice and their normal siblings. Results available to date indicate that litter crowding extends longevity in normal animals genetically distinct from those used in the study of Sun et al. [55], although this effect appears to be sex-related and may be specific to females. The results also suggest that litter crowding reduces early mortality of Ghr−/− females. We must await data on average and maximal longevity to draw definitive conclusions from this study, but survival benefit of litter crowding in animals that lack GH receptors and are known to derive little or no survival benefit from calorie restriction initiated in adulthood [56,57] would be intriguing by implying differential mechanisms of the effects of early and transient as compared to adult and chronic calorie restriction.
Current understanding of the interactions between nutrient availability and somatotropic signaling during different stages of development is fragmentary and much remains to be learned about the impact of these interactions on the development of adult phenotypes and aging. In contrast to the apparent benefits of GH deficiency during development in hereditary dwarf mice, metabolic defects in rats born to severely underfed dams were corrected by injections of GH during postnatal development [58,59]. Moreover, longevity of genetically dwarf rats was extended by GH therapy early in life and these benefits were ascribed to correcting their developmental deficits [40]. Much additional work will be needed before results obtained in different species and in studies using vastly different protocols can be meaningfully compared and hopefully reconciled.
5. Discussion and conclusions
Findings described in this article have several novel, and we believe important, implications. First, it appears that the remarkable increase in longevity of mice lacking GH or GH receptors [16–19] may be due, in large measure, to the absence of GH signals during a particular, apparently relatively short segment of their life history. Second, alterations in the availability of nutrients and in endocrine function during the first few weeks of postnatal life have a major role in determining adult phenotypic characteristics known or suspected to be related to the mechanisms of aging. Importantly, these phenotypic changes are associated with changes in longevity. Third, the well-documented changes in the risk of diabetes, cardiovascular disease and other health problems in response to early nutritional (and presumably also hormonal) environment are accompanied and likely driven by changes in the trajectory of aging. This last implication may be particularly important because the probability of developing most of the chronic, non-infectious diseases increases exponentially with chronological age. In other words, the findings discussed in this article may have some potentially very important clinical and public health implications. High-energy food, particularly when consumed in amounts that are out of proportion with energy expenditure of children growing in the 21st century environment may lead to excessive activation of GH, insulin/IGF-1 and mTOR signaling and thus may set the stage for accelerated aging and early onset of age-associated chronic diseases. Interested readers may wish to consult publications of T. Samaras [34] for further thoughts on this subject.
When viewed from a broader biological perspective, the impact of early hormonal and nutritional interventions on longevity adds to the ongoing debate about programming of aging [60–62]. The force of natural selection obviously declines during the post-reproductive life. Senescence (defined as increasing frailty) and long post-reproductive survival are generally believed to be exceedingly uncommon in the natural environment. Therefore, many biologists conclude that the rate of aging and the species-specific lifespan do not reflect genetic/evolutionary programming. However, it has also been proposed that post-reproductive survival may benefit offspring and their progeny (the “grandmother effect” [63]) and may also have a positive impact on the reproductive success of genetically related individuals by helping in “niche maintenance” [64]. Consequently, genetic predispositions to longevity could be subject to positive selection.
The impact of GH actions during development on aging could also be related to the complex relationships between growth, sexual maturation, fecundity and longevity, with many examples of trade-offs between reproduction and aging. In Ghr−/−, Snell and Ames dwarf mice, sexual maturation is delayed and in dwarf mice it can be accelerated by treatment with GH (in dwarfs) or IGF-1 (Ghr−/−) [24–27,65]. Correlation between the age of female sexual maturation and circulating IGF-1 levels (a GH-dependent trait) was detected on analysis of multiple inbred mouse strains in which IGF-1 levels are negatively related to longevity [31]. Interestingly, delayed sexual maturation and reduced fecundity in long-lived GH-related mouse mutants [24–27] is associated with various indices of a delay in reproductive aging [66,67, and unpublished observations]. It could be argued that correcting the delay in sexual maturation in Ames dwarf mice by early GH treatment may account for or contribute to their reduced longevity.
These issues notwithstanding, findings discussed in this article indicate that genetic differences in the function of the endocrine system during development as well as early nutritional and other environmental factors can “program” many features of adult phenotype, including the suspected mechanisms and the trajectory of aging. Available evidence suggests that he role of the endocrine system in the control of longevity may involve interplay of developmental “programming” and effects of hormones in adult life.
Acknowledgments
Work described in this article was supported by NIH P01AG031736 and R01AG019899. We thank Dr. O. Arum, A. Spong and other members of our laboratory who were importantly involved in various stages of this research and we apologize to those whose work pertinent to the discussed issues we have failed to mention or cite.
Footnotes
Publisher's Disclaimer: This is a PDF file of an unedited manuscript that has been accepted for publication. As a service to our customers we are providing this early version of the manuscript. The manuscript will undergo copyediting, typesetting, and review of the resulting proof before it is published in its final citable form. Please note that during the production process errors may be discovered which could affect the content, and all legal disclaimers that apply to the journal pertain.
References
Full text links
Read article at publisher's site: https://doi.org/10.1016/j.exger.2015.12.011
Read article for free, from open access legal sources, via Unpaywall:
https://europepmc.org/articles/pmc4930735?pdf=render
Citations & impact
Impact metrics
Citations of article over time
Smart citations by scite.ai
Explore citation contexts and check if this article has been
supported or disputed.
https://scite.ai/reports/10.1016/j.exger.2015.12.011
Article citations
Klotho in pregnancy and intrauterine development-potential clinical implications: a review from the European Renal Association CKD-MBD Working Group.
Nephrol Dial Transplant, 39(10):1574-1582, 01 Sep 2024
Cited by: 1 article | PMID: 38486352 | PMCID: PMC11427066
Review Free full text in Europe PMC
Impact of visceral adipose tissue on longevity and metabolic health: a comparative study of gene expression in perirenal and epididymal fat of Ames dwarf mice.
Geroscience, 46(6):5925-5938, 22 Mar 2024
Cited by: 0 articles | PMID: 38517641 | PMCID: PMC11493907
Growth hormone - past, present and future.
Nat Rev Endocrinol, 14(5):285-300, 16 Mar 2018
Cited by: 112 articles | PMID: 29546874
Review
Differential effects of early-life nutrient restriction in long-lived GHR-KO and normal mice.
Geroscience, 39(3):347-356, 18 May 2017
Cited by: 21 articles | PMID: 28523599 | PMCID: PMC5505892
Similar Articles
To arrive at the top five similar articles we use a word-weighted algorithm to compare words from the Title and Abstract of each citation.
Early Life Interventions Can Shape Aging.
Front Endocrinol (Lausanne), 13:797581, 25 Feb 2022
Cited by: 4 articles | PMID: 35282433 | PMCID: PMC8916564
Review Free full text in Europe PMC
Longevity is impacted by growth hormone action during early postnatal period.
Elife, 6:e24059, 04 Jul 2017
Cited by: 41 articles | PMID: 28675141 | PMCID: PMC5515575
Growth hormone modulates hypothalamic inflammation in long-lived pituitary dwarf mice.
Aging Cell, 14(6):1045-1054, 12 Aug 2015
Cited by: 49 articles | PMID: 26268661 | PMCID: PMC4693470
Long-lived hypopituitary Ames dwarf mice are resistant to the detrimental effects of high-fat diet on metabolic function and energy expenditure.
Aging Cell, 15(3):509-521, 17 Mar 2016
Cited by: 31 articles | PMID: 26990883 | PMCID: PMC4854906
Funding
Funders who supported this work.
NIA NIH HHS (3)
Grant ID: K01 AG048264
Grant ID: P01 AG031736
Grant ID: R01 AG019899
NIH (2)
Grant ID: R01AG019899
Grant ID: P01AG031736