Abstract
Free full text

Tumor-expressed IDO recruits and activates MDSCs in a Treg-dependent manner
Associated Data
Abstract
Indoleamine 2,3-dioxygenase (IDO) has been described as a major mechanism of immunosuppression in tumors, though the mechanisms of this are poorly understood. Here, we find that expression of IDO by tumor cells results in aggressive tumor growth and resistance to T-cell targeting immunotherapies. We demonstrate that IDO orchestrates local and systemic immunosuppressive effects through recruitment and activation of myeloid-derived suppressor cells (MDSCs), through a mechanism dependent on regulatory T cells (Tregs). Supporting these findings, we find that IDO expression in human melanoma tumors is strongly associated with MDSC infiltration. Treatment with a selective IDO inhibitor in vivo reversed tumor-associated immunosuppression by decreasing numbers of tumor-infiltrating MDSCs and Tregs, and abolishing their suppressive function. These findings establish an important link between IDO and multiple immunosuppressive mechanisms active in the tumor microenvironment, providing a strong rationale for therapeutic targeting IDO as one of the central regulators of immune suppression.
eTOC BLURB
IDO mediates immune inhibition in tumors, though the mechanisms of this are poorly understood. Holmgaard et al. demonstrate that tumor IDO is a central regulator of both local and systemic immunosuppression and resistance to immunotherapy, which is orchestrated through expansion, recruitment, and activation of MDSCs in a Treg-dependent manner.
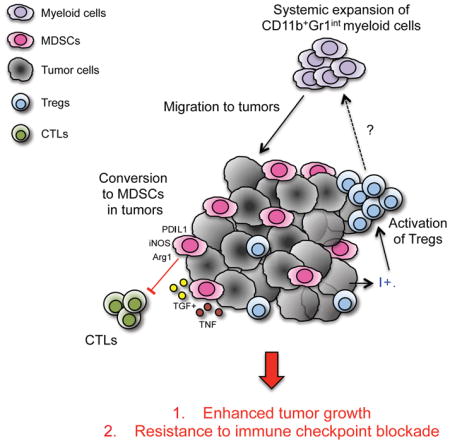
INTRODUCTION
Indoleamine 2,3-dioxygenase (IDO) is expressed in many human cancers, and high IDO expression is associated with advanced disease stage and tumor metastasis in a variety of cancer types (Munn, 2012). In cancer, IDO can either be expressed directly by the tumor cells themselves, or induced indirectly in host antigen presenting cells by the presence of tumor. By driving IDO overexpression, tumors are able to create an immunosuppressive microenvironment that blocks the antitumor immune response (Prendergast et al., 2014). Several mechanisms of IDO-mediated immune suppression have been described. IDO inhibits the activation of effector T cells (Teff) through depletion of the essential amino acid tryptophan and promotes differentiation and activation of Foxp3+ regulatory T cells (Tregs) through production of kynurenine (Munn and Mellor, 2007).
We have previously demonstrated in animal models that IDO expression mediates resistance to cancer immunotherapy with immunomodulatory antibodies targeting CTLA-4 and PD-1 (Holmgaard et al., 2013). Here, in order to delineate the mechanisms of tumor cell IDO-induced immunosuppression, we developed a B16 melanoma model overexpressing IDO (B16-IDO). When implanted into mice, B16-IDO tumors exhibited aggressive tumor growth, characterized by rapid tumor progression and resistance to T-cell-targeting immunotherapies. This effect was associated with systemic expansion of myeloid cells and marked recruitment of myeloid-derived suppressor cells (MDSCs) into the tumor microenvironment. Importantly, similar correlation between IDO expression and MDSC infiltration was seen in human melanoma samples and other animal tumor models naturally expressing high levels of IDO. Inhibition of IDO or depletion of Tregs resulted in reduction of MDSCs and reversal of immune suppression. Our findings establish tumor IDO expression as a key regulator of immunosuppression in the tumor microenvironment and on systemic level and provide a strong rationale for therapeutic targeting of this pathway.
RESULTS
Suppressive MDSCs are highly expressed in melanoma patients with IDO positive tumors
Munn et al. has previously shown that the presence of an abnormally high level of IDO-expressing cells in the sentinel LN of patients with malignant melanoma correlate with poor long-term prognosis (Munn et al., 2004). Similarly, in our studies, we find that the majority of patients (33 out of 36 cases) with resected melanoma displayed high levels of IDO in their tumors (Fig. 1A). These findings suggested that IDO might play a mechanistic role in tumor progression and prompted us to examine the influence of IDO on the melanoma tumor microenvironment in more details.
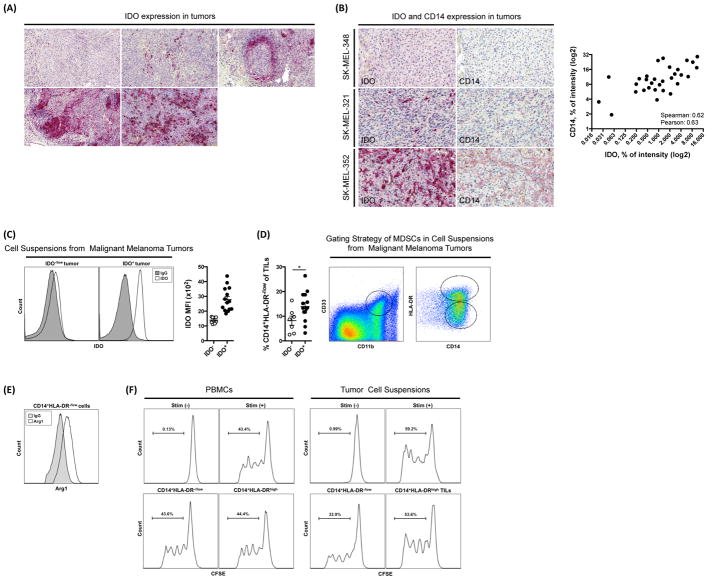
(A) Expression of IDO in tumor tissue from tumors of 5 patients with malignant melanoma determined by IHC. (B) Representative IHC staining of CD14 and IDO in tissue from metastatic human melanoma tumors and correlation between IDO and CD14 intensity. (C) Intracellular staining and MFI for IDO within the CD45− gated population of cell suspensions obtained from metastatic melanoma tumors. (D) Frequency of CD11b+CD33+CD14+HLA-DR−/low cells in cell suspensions of IDO+ and IDO− tumors and gating strategy. (E) Expression of Arg1 in CD11b+CD33+CD14+HLA-DR−/low gated population. (F) T cell suppression assay with CD14+HLA-DR−/low cells enriched from PBMCs or cell suspensions from tumors of patients with IDO+ tumors. Data are representative of 36 patients (A), 33 patients (B), 22 patients (C–E), and 5 patients (F).
Critical components of the tumor microenvironment include immunosuppressive MDSCs. MDSCs in cancer patients have been characterized by the expression of CD14, CD11b, CD33, Arg1 and low/absent expression of HLA-DR (Gabrilovich et al., 2012). While analyzing the tumor microenvironment of IDO-expressing tumors from patients with malignant melanoma, we noted an increase in CD14+ cells. Using IHC, we show that the intensity of CD14 expression is positively correlated with the intensity of IDO expression in these tumors (Fig. 1B). This was supported by analysis of data in the TCGA database showing a positive correlation between IDO and CD14 mRNA in human melanoma (Fig. S1). To formally quantify this correlation, cell suspensions prepared from tumor samples from patients with malignant melanoma were stained for intracellular expression of IDO and divided into IDO− and IDO+ groups accordingly (Fig. 1C). Supporting our findings, by flow cytometry we found that the number of CD11b+CD33+CD14+HLA-DR−/low cells was significantly increased in patients with IDO+ tumors (Fig. 1D). When examining the functional capacity of the myeloid cells, we found that the tumor-infiltrating CD11b+CD33+CD14+HLA-DR−/low cells were characterized by high expression of Arg1 (Fig. 1E), indicating that these cells can be defined as MDSCs. Furthermore, we demonstrate that CD14+HLA-DR−/low cells isolated from IDO+ tumors were able to suppress proliferation of autologous CD8+ T cells in vitro (Fig. 1F). In contrast, a similar population enriched from peripheral blood mononuclear cells (PBMCs) was not suppressive (Fig. 1F), suggesting that the MDSCs are activated at the tumor site. These data suggested that there may be a link between IDO expression and immunosuppressive MDSCs in cancer and prompted us to examine the influence of IDO on tumor-infiltrating MDSCs in more details using experimental tumor models. For this purpose, we used two different model systems: the murine B16 tumor cell line transduced to overexpress IDO as well as the 4T1 tumor cell line with known high endogenous IDO expression.
Expression of IDO in melanoma is associated with aggressive tumors
In contrast to the high levels of IDO expression observed in human melanoma tissue (Fig. 1A), we did not detect IDO expression in the well-described murine B16 melanoma cell line (Fig. S2A). This observation may reflect the heterogeneity of IDO expression in human tumors. Thus, in order to model melanoma cells expressing IDO, we generated a B16 melanoma cell line overexpressing IDO-GFP fusion protein (B16-IDO) and a control cell line overexpressing GFP alone (B16-WT). Expression of IDO was confirmed by flow cytometry and Western blot analysis (Fig. S2A and S2B). When injected into naive mice, the B16-IDO tumors grew significantly faster and produced larger tumors (Fig. 2A). IDO expression did not influence the growth of B16 cells in vitro (Fig. S2C), or in irradiated naive mice (Fig. 2A), suggesting that the enhanced growth of the B16-IDO tumors was not related to a higher intrinsic growth rate of these cells. The levels of tryptophan and its IDO-generated degraded product, L-kynurenine, were evaluated by ELISA in blood and tumor supernatants, demonstrating decreased level of tryptophan and increased level of L-kynurenine in the B16-IDO tumors, but not in peripheral blood (Fig. 2B). To determine whether constitutive expression of IDO by B16 cells carried any implications for efficacy of cancer immunotherapy, animals bearing B16-IDO or B16-WT tumors were treated with antibodies to CTLA-4, PD-1, or combination of both. While combination therapy led to 60% long-term animal survival in the B16-WT tumor-bearing mice, B16-IDO tumors were completely resistant to therapy (Fig. 2C). Altogether, these results suggest that IDO expression by B16 tumor cells promotes tumor growth and resistance to immune checkpoint blockade immunotherapy.
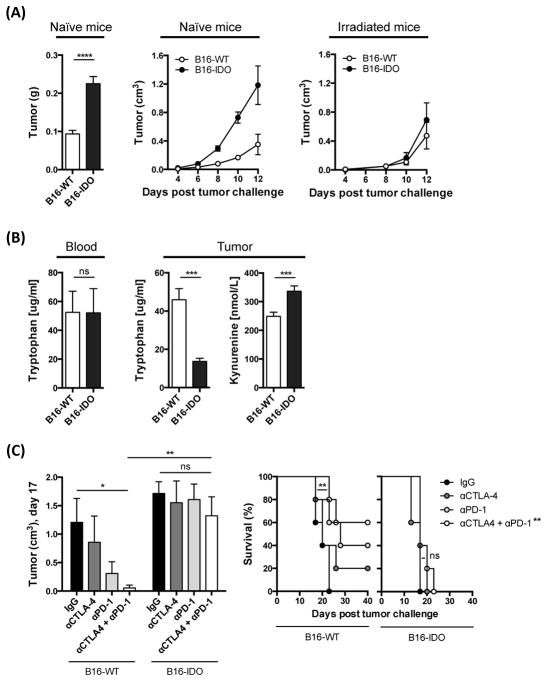
(A) Mean weight and average growth rate of B16-WT and B16-IDO tumors in naive and irradiated mice. (B) Levels of tryptophan and L-kynurenine in tumor and blood evaluated by ELISA. (C) Mean size of αCTLA-4 + αPD-1 treated B16-IDO and B16-WT tumors and long-term survival. Data are represented as mean ± SEM.
IDO inhibits proliferation and accumulation of tumor-specific T cells at the tumor site
To assess tumor-specific immune responses in the B16-IDO and B16-WT tumors, mice received a labeled cohort of activated transgenic CD8+ T cells, recognizing a peptide from the melanosomal antigen gp100 (pmel). In mice with B16-WT tumors, we observed a robust proliferation and increase in number of pmels in TDLNs as well as in tumors (Fig. S2D). In contrast, pmels had significantly lower accumulation in the B16-IDO tumors and TDLNs (Fig. S2D). These data indicate that tumor-expressed IDO leads to inhibition of proliferation of tumor-specific T cells, and prevents their accumulation at the tumor site, which may be responsible for the observed enhanced tumor progression.
Suppressive myeloid cells are expanded in IDO-expressing tumors
To establish the mechanism responsible for the inhibition of tumor-specific T cells in the IDO-expressing tumors, we analyzed the tumor immune composition. While the absolute numbers of CD45+ cells in the B16-IDO and B16-WT tumors were comparable (Fig. S3A), the relative frequency of CD3+ cells was significantly lower in the B16-IDO tumors (Fig. 3A). This decrease in CD3+ T cells corresponded to a diminished infiltration of both CD8+ and CD4+ Teff cells (Fig. 3B), but a notable increase in Foxp3+ Tregs (Fig. 3B). Activation of Tregs in tumor models in vivo has previously been linked to IDO so this finding was not surprising (Sharma et al., 2007). There were also decreased percentages of CD19+ B cells, NK cells, and natural killer T cells (NKT) noted at the tumor site, but these differences did not reach statistical significance (Fig. 3A and B). Importantly, there was a significant increase in myeloid CD11b+ cells in the B16-IDO tumors compared to B16-WT tumors (Fig. 3A), resulting in decreased intratumoral ratio of CD3+ T cells to CD11b+ cells (Fig. 3C). Of note, the CD11b+ cells isolated from the B16-IDO tumors, but not from the B16-WT were capable of suppressing CD8+ T-cell proliferation in vitro (Fig. 3D), suggesting that these cells may play a role in the observed aggressiveness of the B16-IDO tumors.
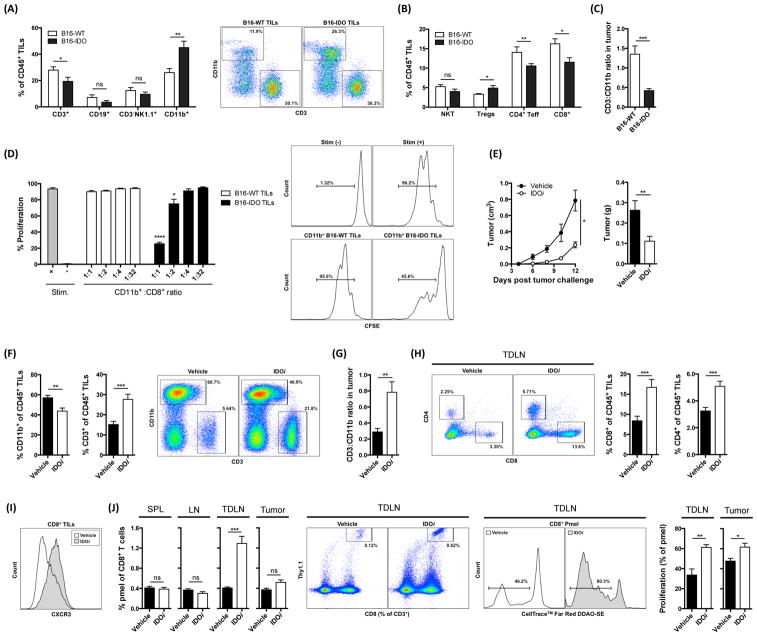
(A) B16-IDO and B16-WT tumors were analyzed for infiltrating immune cells. Results for CD3+ T cells, CD19+ B cells, CD3−NK1.1+ NK cells, and CD11b+ myeloid cells as frequencies of CD45+ cells and representative dot plots. (B) Results for T cell subsets: NK1.1+ T cells (NKT), CD4+Foxp3+ Tregs, CD4+Foxp3− Teff cells, and CD8+ T cells as frequencies of CD45+ cells. (C) CD3+/CD11b+ ratios. (D) In vitro suppressive activity of CD11b+ cells from B16-IDO or B16-WT tumors. Representative histograms of CD8+ T-cell proliferation are shown in CD11b+/CD8+ ratio of 1:1. (E) Average growth and mean weight of IDOi and vehicle treated B16-IDO tumors. (F) Relative percentages of CD11b+ and CD3+ cells of CD45+ cells in IDOi and vehicle treated B16-IDO tumors and representative flow plots. (G) Calculated CD3+/CD11b+ ratios. (H) Percent of tumor-infiltrating CD4+ and CD8+ T cells gated on CD45+ population and representative plots. (I) Example of CXCR3 staining within the CD8+ gated population. (J) Representative plots and graphs showing percentage pmels of total CD8+ T cells in LN, TDLN, spleen, and tumor, and proliferation of pmels in tumor and TDLNs, of IDOi and vehicle treated B16-IDO tumor-bearing mice. Data are shown as mean ± SEM.
Inhibition of IDO blocks expansion of myeloid CD11b+ cells in tumors
To determine whether the expansion of CD11b+ cells in B16-IDO tumors was indeed caused by IDO, mice with B16-IDO tumors were treated with the IDO inhibitor indoximod (IDOi). Therapy with IDOi reduced the growth rate and size of B16-IDO tumors significantly (Fig. 3E), which was associated with decrease in the number of CD11b+ cells and increase in CD3+ T cells in tumors (Fig. 3F), resulting in increased CD3+/CD11b+ ratios (Fig. 3G). Furthermore, treatment with IDOi led to an increase in CD4+ and CD8+ T cells in the tumor (Fig. 3H) with upregulation of the Teff cell marker CXCR3 on the tumor-infiltrating CD8+ T cells (Fig. 3I). To assess for tumor-specific responses, we adoptively-transferred activated pmels. IDOi treatment resulted in increase and significant proliferation of pmels within the TDLNs and tumors of B16-IDO tumor-bearing mice (Fig. 3J), and led to significantly reduced tumor growth (Fig. S3B). These results indicate that tumor IDO expression not only favors expansion of CD11b+ but also blocks trafficking and proliferation of tumor-specific T cells at the tumor site.
CD11b+ cells infiltrating IDO-expressing tumors exhibit MDSC properties
In order to evaluate the distinct suppressive abilities of the CD11b+ cells found in the B16-IDO and B16-WT tumors, we initially analyzed expression of the myeloid differentiation antigen Gr1 (Ly6G). It has previously been demonstrated that the intensity of Gr1 staining identifies CD11b+ cells with functional heterogeneity (Gabrilovich and Nagaraj, 2009), and consisted of two major subsets with different Gr1 expression levels, termed Gr1high and Gr1int cells, but not Gr1high cells being suppressive (Dolcetti et al., 2010). The majority of the tumor-infiltrating CD11b+ cells of both B16-IDO and B16-WT tumors expressed Gr1 (Fig. 4A). Interestingly, the increase in myeloid CD11b+ cells observed in the B16-IDO tumors corresponded to a predominant increase in the Gr1int population (Fig. 4A). We found that CD11b+Gr1int but not CD11b+Gr1high cells purified from B16-IDO tumors were capable of suppressing T-cell proliferation (Fig. 4B). Interestingly, CD11b+Gr1int cells isolated from B16-WT tumors were incapable of suppressing CD8+ T-cell proliferation (Fig. 4B). The CD11b+Gr1int subsets from B16-IDO and B16-WT tumors were then tested for differential expression of various surface markers, which have previously been described in tumor-derived CD11b+ cells with immunosuppressive properties (Dolcetti et al., 2010). Phenotypically, CD11b+Gr1int cells from both B16-IDO and B16-WT tumors showed low levels of MHC class II and CD11c and intermediate expression of F4/80 (Fig. 4C), confirming their status as immature myeloid cells and distinguishing them from mature myeloid cells such as dendritic cells and macrophages. Furthermore, this population showed expression of the typical monocyte marker Ly6C (Fig. 4C). Thus, while we were not able to distinguish the two CD11b+Gr1int populations based on the majority of phenotypic markers, the cells demonstrated clear functional differences with regards to immunosuppression. To this end, we observed that expression of IL-4Rα, which has been suggested as a functional marker of immunoregulatory CD11b+ cells (Gallina et al., 2006), was higher in the CD11b+Gr1int population from B16-IDO tumors (Fig. 4D), and thus, IL-4Ra was the only predictive marker of the suppressive activity. Taken together, these data indicate that tumor IDO expression results in expansion of CD11b+Gr1int cells, which possess immunosuppressive properties, consistent with their functional definition as MDSCs. These results support our initial findings in the patient tumor samples and thus highlight an important link between IDO expression and MDSCs in cancer.
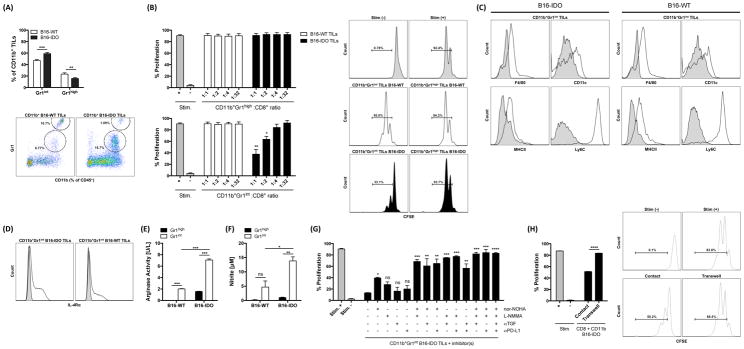
(A) CD11b+ subsets obtained from B16-IDO and B16-WT tumors were evaluated for CD11b versus Gr1 expression and graphed as percent Gr1int and Gr1high cells. (B) Suppressive properties of CD11b+Gr1high and CD11b+Gr1int cells from B16-IDO or B16-WT tumors. Representative histograms of CD8+ T-cell proliferation are shown in CD11b+ to CD8+ T cell ratio of 1:1. (C) CD11b+Gr1int subsets from B16-IDO and B16-WT tumors were evaluated for expression of F4/80, CD11c, MHC class II and Ly6C markers (open histograms) against their matched isotype controls (filled histograms). (D) IL-4Rα expression (open histograms) within the CD11b+Gr1int gated populations against matched isotype control (filled histograms). (E) Arg1 activity in CD11b+Gr1high and CD11b+Gr1int subsets from B16-IDO and B16-WT tumors. (F) Nitrite/NO concentration in supernatants of LPS stimulated CD11b+Gr1high and CD11b+Gr1int cells. (G) Suppressive activity of CD11b+Gr1int cells from B16-IDO tumors in the presence of inhibitors of Arg1 (nor-NOHA), iNOS (LNMMA), TGFβ (anti-TGFβ) and/or PD-L1 (anti-PD-L1). Data are shown for CD8+ to CD11b+Gr1int ratios of 1:1. (H) Suppressive activity CD11b+ cells from B16-IDO tumors measured with CD11b+ cells in contact with CD8+ T cells or placed in 0.4 μm cell culture inserts. Data are shown for CD8+ to CD11b+ ratios of 1:1. Data are represented as mean ± SEM.
B16-IDO tumor-infiltrating MDSCs upregulate arginase, iNOS and production of immunosuppressive cytokines
To gain further insight into the different suppressive character of CD11b+Gr1+ cells found in B16-IDO and B16-WT tumors, we measured the production and expression of factors that have been linked to the immunosuppressive activity of MDSCs (Gabrilovich et al., 2012). We observed that CD11b+Gr1int cells sorted from B16-IDO tumors displayed significantly higher arginase-1 (Arg1) and NO production as compared to CD11b+Gr1int cells from B16-WT tumors or to their granulocytic CD11b+Gr1high counterparts (Fig. 4E and F). In contrast, CD11b+Gr1high subsets produced highly elevated levels of ROS compared with CD11b+Gr1int cells, as evidenced by increased DCFDA incorporation (Fig. S4A). To verify that these molecules were indeed important for suppression, we measured T-cell proliferation with CD11b+Gr1int cells obtained from B16-IDO tumors in presence of inhibitors of Arg1 and inducible NO synthase (iNOS). Indeed, combination of Arg1 inhibitor with iNOS inhibitor completely blocked the suppressive activity (Fig. 4G). It has also been suggested that activated MDSCs produce high levels of transforming growth factor-β (TGFβ) and mediate their suppressive activity through PD-L1 expression (Gabrilovich et al., 2012). To this end, the addition of either αTGFβ or αPD-L1 antibody to Arg1 and iNOS inhibitors further augmented T-cell proliferation (Fig. 4G). Using a transwell system, we further found that inhibition of CD8+ T-cell proliferation was contact-dependent (Fig. 3H). Importantly, increased expression of Arg1, NO, TGFβ, PD-L1, and IL-4Rα was confirmed on mRNA level by real time PCR (RT-PCR) (Fig. S4B). The upregulation of these molecules in CD11b+Gr1int cells from B16-IDO tumors compared to CD11b+Gr1int cells from B16-WT tumors could explain their distinct suppressive capacity.
Activation of MDSCs is dependent on tumor-expressed IDO
We next proceeded to evaluate whether IDO was also required for tumor-infiltrating CD11b+Gr1int cells to be activated and become functional MDSCs. Treatment of B16-IDO tumor-bearing animals with IDOi revealed a significant reduction in intratumoral CD11b+Gr1int cells (Fig. 5A), with a concomitant decrease of IL-4Rα expression (Fig. 5B) and loss of suppressive activity in this subset (Fig. 5C). Thus, inhibition of IDO did not only reduce the frequency of tumor-infiltrating MDSCs, but also inhibited their capability to suppress T-cell proliferation, indicating that activation of MDSCs in tumors requires IDO. Addition of IDOi in vitro on the other hand, did not restore T-cell proliferation (Fig. 5C), indicating that MDSCs do not exert their suppressive capacity through expression of IDO. In support of the latter, B16-IDO tumors grown in IDO−/− mice exhibited a similar aggressive behavior and inhibition of IDO in these animals also reduced tumor growth (Fig. 5D) and increased ratios of intratumoral CD3+ to CD11b+Gr1+ cells (Fig. 5E), indicating that the contribution of host-derived IDO is absent or minor in this model.
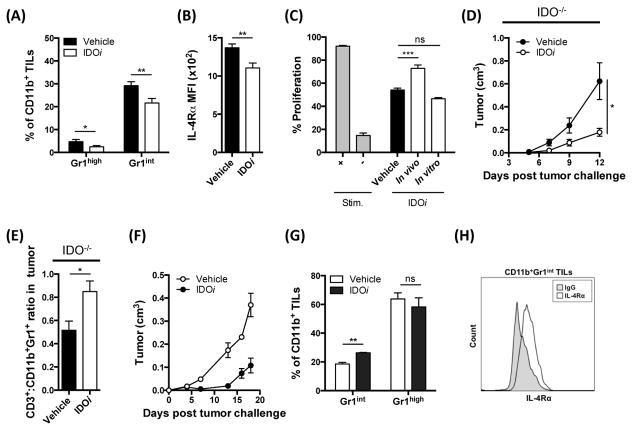
(A) Frequencies of Gr1high and Gr1int subsets of total CD11b+ cells from B16-IDO tumors of IDOi or vehicle treated mice. (B) MFI for IL-4Rα expression within the CD11b+Gr1int population. (C) T cell suppression assay with CD11b+Gr1int cells purified from B16-IDO tumors in the presence of an IDOi added in vitro or administered in vivo. (D) Growth curve for B16-IDO tumors in IDOi treated IDO−/− mice. (E) Ratio of CD3+ to CD11b+Gr1+ cells in B16-IDO tumors in IDOi treated IDO−/− mice. (F) Average growth rate and mean weight of IDOi and vehicle treated 4T1 tumors. (G) Relative percentages of Gr1+ of total CD11b+ cells in 4T1 tumors after IDOi therapy. (H) IL-4Rα expression within the CD11b+Gr1int gated population of TILs from untreated 4T1 tumor-bearing mice. Data are represented as mean ± SEM.
MDSCs are expanded in a murine model expressing endogenous IDO
We next sought to determine whether IDO also drives the expansion of MDSCs in a tumor model naturally expressing high levels of IDO. Analysis of several murine cancer cell lines revealed high levels of IDO expression in the 4T1 breast cancer cell line when implanted in vivo (Fig. S5). Interestingly, this model has previously been shown to expand immunosuppressive CD11b+Gr1int cells (Dolcetti et al., 2010). Treatment of 4T1 tumor-bearing mice with IDOi reduced the tumor growth rate (Fig. 5F). This was accompanied by a significant decrease in the intratumoral CD11b+Gr1int cells, but not CD11b+Gr1high cells (Fig. 5G). Notably, CD11b+Gr1int cells purified from the 4T1 tumors were characterized by expression of IL-4Rα (Fig. 5H), suggesting that this cell population is indeed immunosuppressive.
Tumor IDO-induced expansion of MDSCs is systemic
Expansion of MDSCs has been previously described in the spleens of EL-4, CT26 and 4T1 mouse tumor models (Dolcetti et al., 2010; Youn et al., 2008), suggesting that tumors drive systemic MDSC expansion. Similar to these findings, analysis of spleens from B16-IDO tumor-bearing mice revealed expansion of CD11b+ cells (Fig. 6A), with predominance of Gr1+ cells (Fig. 6B). Surprisingly, while the CD11b+Gr1int splenocytes from the 4T1 and EL-4 tumor-bearing mice were shown to possess immunosuppressive activity (Dolcetti et al., 2010; Youn et al., 2008), the CD11b+Gr1int cells from spleens of B16-IDO tumor-bearing mice did not suppress CD8+ T-cell proliferation (Fig. 6C). While these splenic CD11b+Gr1int cells were phenotypically similar to the corresponding CD11b+Gr1int cells isolated from B16-IDO tumors (Fig. S6A), they produced significantly lower levels of Arg1 (Fig. S6B) and NO (Fig. S6C) and showed lower expression of IL-4Rα (Fig. S6D). The splenic expansion of CD11b+Gr1int cells could be significantly reduced when mice were treated with IDOi (Fig. S6E), further confirming the role of IDO in controlling this process.
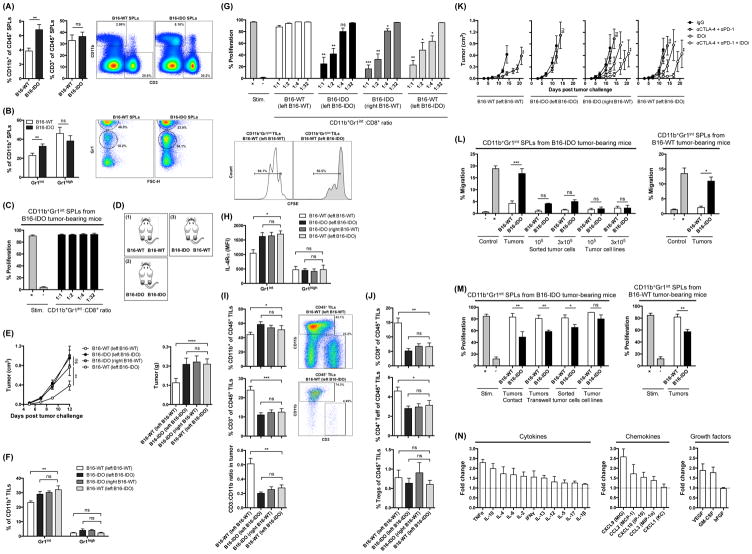
(A) Frequencies of CD11b+ myeloid cells and CD3+ T cells of total CD45+ cells in splenocytes from B16-IDO and B16-WT tumor-bearing mice and representative dot plots. (B) Expression of Gr1 within the CD11b+ gated population and representative plots. (C) Suppressive properties of CD11b+Gr1int splenocytes from B16-IDO tumor-bearing mice. (D) Bilateral flank tumor models: (1) B16-WT tumors on both flanks, (2) B16-IDO tumors on both flanks, and (3) B16-IDO tumor on one flank and B16-WT on the contralateral flank. (E) Mean growth and weight of bilateral flank tumors. (F) Infiltration of CD11b+Gr1int and CD11b+Gr1high myeloid cells in bilateral flank B16-IDO and B16-WT tumors. (G) Suppressive activity of CD11b+Gr1int cells from B16-IDO or B16-WT tumors of bilateral flank tumor-bearing mice and representative histograms. (H) MFI for IL-4Rα expression within CD11b+Gr1int and CD11b+Gr1high gated populations. (I) Frequencies of CD11b+ and CD3+ of CD45+ total cells and CD3+/CD11b+ ratios in bilateral flank B16-IDO and B16-WT tumors, and representative plots. (J) Frequencies of CD8+, CD4+Foxp3−, and CD4+Foxp3+ cells of CD45+ total cells in bilateral flank B16-IDO and B16-WT tumors. (K) Mean tumor growth of αCTLA-4 + αPD-1 and/or IDOi treated B16-IDO and B16-WT tumors in bilateral flank tumor models. (L) Migration of CD11b+Gr1int splenocytes purified from B16-IDO and B16-WT tumor-bearing mice towards homogenized tumors, sorted tumor cells or tumor cell lines as indicated. (M) Suppressive activity of CD11b+Gr1int splenocytes of B16-IDO and B16-WT tumor-bearing mice pre-cultured with homogenized tumors, sorted tumor cells or tumor cell lines. CD11b+Gr1int cells were pre-cultured in contact with tumor cells or placed in 0.4 μm cell culture inserts as specified. (N) Relative levels of soluble factors in supernatants of B16-IDO tumors compared to B16-WT tumors, as determined by Luminex. Data are represented as mean ± SEM.
Recruitment of CD11b+Gr1int cells and activation of immunosuppressive properties are dependent on the tumor microenvironment
The findings above suggested that while B16-IDO tumors induce systemic expansion of the CD11b+Gr1int cells, the tumor microenvironment might be necessary to render them suppressive. To explore this possibility, we established bilateral flank tumor models bearing B16-IDO tumors in one flank and B16-WT tumors in the contralateral flank (Fig. 6D). Remarkably, B16-IDO tumors resulted in enhanced growth of B16-WT tumors on the contralateral flank (Fig. 6E), which was associated with increase in CD11b+Gr1int cells in the B16-WT tumors (Fig. 6F), and acquisition of immunosuppressive properties by these cells (Fig. 6G). Consistent with their suppressive activity, these cells were characterized by increased expression of IL-4Rα (Fig. 6H). In addition, we observed lower percentages of CD3+ T cells in these tumors and as a result, a decrease in the intratumoral ratio of CD3+ T cells to CD11b+ cells (Fig. 6I). The observed decrease in CD3+ T cells was due to reduction of both CD4+Foxp3− and CD8+ T cells, but not CD4+Foxp3+ Tregs (Fig. 6J). Notably, the antitumor effect of αCTLA-4 + αPD-1 combination therapy in the B16-WT tumor model was also completely lost in the B16-IDO/B16-WT bilateral flank tumor model, and this could be overcome by therapeutic inhibition of IDO with IDOi (Fig. 6K). Altogether, these results demonstrate that the IDO-driven expansion of CD11b+Gr1int cells is indeed systemic, but require the effect of the tumor microenvironment for activation of their suppressive activity.
To understand the mechanisms of expansion of CD11b+Gr1int cells in tumors, we analyzed Bromodeoxyuridine (BrdU) incorporation in CD11b+Gr1int cells in bone marrow (BM), blood, spleen, and tumor tissue of B16-IDO tumor-bearing mice. High percentages of BrdU+ CD11b+Gr1int cells were mainly detected in BM and in the spleens but not in tumors (Fig. S6F), suggesting that their accumulation in tumors is likely due to the recruitment from the periphery and less likely due to proliferation at the tumor site. In support of this hypothesis, splenic CD11b+Gr1int cells exhibited an increased ability to migrate through collagen-coated culture inserts toward B16-IDO tumors in vitro, which was not observed with B16-WT tumors (Fig. 6L). This effect required all components of the tumor microenvironment to be present, as migration was significantly diminished toward tumor cells sorted from B16-IDO tumors and completely lost when B16-IDO tumor cell lines cultured in vitro were used as attractants (Fig. 6L).
These findings thus suggest that the CD11b+Gr1int cells likely traffic into the tumor microenvironment from the periphery. To determine whether CD11b+Gr1int cells are functionally activated by the B16-IDO tumors, CD11b+Gr1int splenocytes purified from B16-IDO or B16-WT tumor-bearing mice were cultured with B16-IDO or B16-WT tumors and were tested for the capacity to suppress CD8+ T-cell proliferation. We found that CD11b+Gr1int cells cultured in the presence of B16-IDO tumors but not B16-WT tumors were able to suppress CD8+ T-cell proliferation (Fig. 6M). The establishment of suppressive capabilities did not require direct contact between CD11b+Gr1int MDSCs and tumors as suppression was also observed when CD11b+Gr1int MDSCs were separated from the tumors by a porous membrane (Fig. 6M). CD11b+Gr1int cells co-cultured with B16-IDO tumor cells sorted from tumors also acquired suppressive capability, albeit lower, while co-culture with the in vitro-cultured B16-IDO cell line had no effect (Fig 6M). These results indicate that CD11b+Gr1int cells gain their suppressive capacity at the tumor site, and suggest that this is mediated by soluble factors, likely produced by different components of the tumor microenvironment.
The B16-IDO tumor microenvironment contains high levels of pro-inflammatory cytokines and chemokines
To identify the potential mediators of the preferential accumulation and activation of CD11b+Gr1int MDSCs in the B16-IDO tumors, the expression of chemokines, inflammatory cytokines, and growth factors in tumors was analyzed, revealing increased expression of multiple factors in the B16-IDO tumors (Fig. 6N). The most differentially expressed factors were TNFα, IL-10, IL-4, IL-6, IL-2, IFNγ, IL-13, MIG, MCP-1, MIP-1α, IP-10 and 2 growth factors: VEGF and GM-CSF (p values < 0.01)(Fig. 6M). Several of these factors have been described to be implicated in recruitment of MDSCs and monocytes as well as expansion, activation and function of MDSCs, and are known to be produced by tumor cells, T cells or by activated MDSC themselves (Gabrilovich and Nagaraj, 2009). Interestingly, despite being immunosuppressive, the B16-IDO tumor microenvironment appeared to exhibit markedly higher levels of pro-inflammatory cytokines, suggesting that the recruitment and/or activation of MDSCs in the B16-IDO tumor could be a part of an adaptive immune response.
Accumulation of MDSCs in tumors is dependent on adaptive immunity
These results prompted us to examine the accumulation and functional capacity of CD11b+Gr1+ cells in B16-IDO tumors grown in Rag−/− mice, deficient in T and B cells (Mombaerts et al., 1992). We observed that the absolute number of CD11b+Gr1+ cells in tumors was significantly reduced when B16-IDO tumors were implanted in Rag−/− mice (Fig. 7A), while this reduction was not apparent in B16-WT tumors implanted in Rag−/− mice (Fig. 7A). Furthermore, CD11b+Gr1+ cells purified from B16-IDO tumors harvested from Rag−/− mice were no longer able to suppress proliferation of CD8+ T cells (Fig. 7B). Finally, the migration of splenic CD11b+Gr1+ cells towards B16-IDO tumors grown in Rag−/− mice was completely lost (Fig. 7C). Together, these data show that the adaptive immune response, i.e. T and/or B cells, are likely required for recruitment and functional activation of MDSCs in B16-IDO tumors.
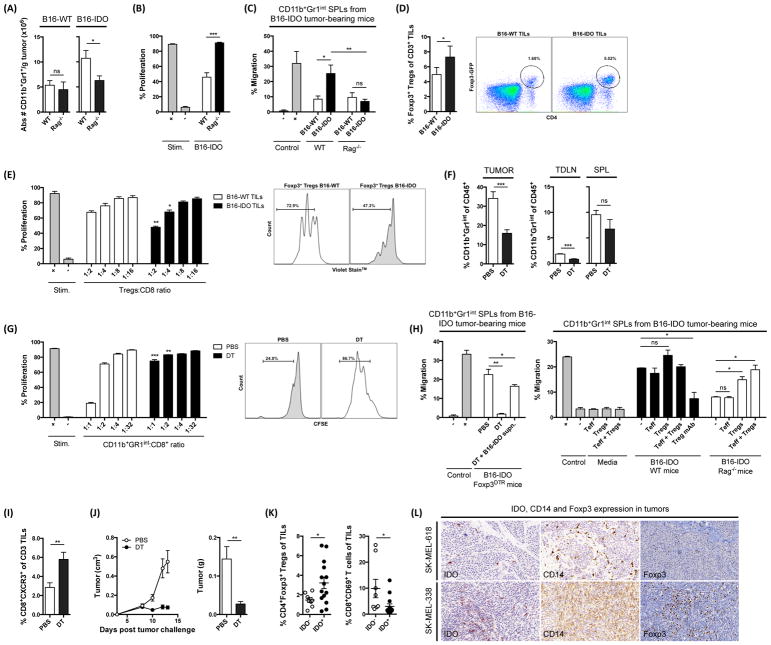
(A) Absolute number of CD11b+Gr1+ cells per gram of tumor in B16-WT and B16-IDO tumors from WT and Rag−/− mice. (B) In vitro suppressive activity of CD11b+Gr1int cells purified from B16-IDO tumors of WT or Rag−/− mice measured in CD11b+Gr1int to CD8+ T cell ratios of 1:1. (C) Migration of CD11b+Gr1int splenocytes of B16-IDO tumor-bearing mice towards B16-IDO tumors harvested from WT or Rag−/− mice. (D) Frequency of Foxp3+ Tregs of total CD3+ T cells in B16-IDO and B16-WT tumors of Foxp3GFP mice. Representative dot plots showing GFP-Foxp3+ expression within CD45+ gated populations. (E) Suppressive properties of Foxp3+ Tregs sorted from B16-IDO and B16-WT tumors of Foxp3GFP mice. Representative histograms of CD8+ T-cell proliferation are shown in Foxp3+ Tregs to CD8+ T cell ratio of 1:1. (F) Frequency of CD11b+Gr1int cells of total CD45+ cells in tumor, TDLNs and spleens of B16-IDO tumor-bearing Foxp3DTR mice injected with DT or PBS. (G) Suppression assay with CD11b+Gr1int cells purified from B16-IDO tumors of Foxp3DTR mice injected with DT and PBS. Representative histograms showing CD8+ T-cell proliferation in CD8+ to CD11b+Gr1int ratios of 1:1. (H) Migration of CD11b+Gr1int splenocytes towards homogenized B16-IDO tumors from Foxp3DTR, WT or Rag−/− mice. Tumors were pre-incubated with Treg-blocking antibody or tumor-infiltrating Treg and/or Teff cells in vitro, as indicated. (I) Frequency of CD8+CXCR3+ T cells of total CD3+ T cells B16-IDO tumors of Foxp3DTR mice injected with DT or PBS. (J) Mean growth rate and weight of B16-IDO tumors in Foxp3DTR mice injected with DT or PBS. (K) Percentage of CD4+Foxp3+ Tregs and CD69+CD8+ T cells in cell suspensions of IDO+ and IDO− human metastatic melanoma tumors. (L) Representative IHC staining of IDO, CD14 and Foxp3 in tissue from metastatic human melanoma tumors. Data represents mean +/− SEM (A–E) or one representative experiment (F–J). Data in K–L are representative of 22 patients.
In previous studies, IDO expression has been demonstrated to induce and activate Foxp3+ Tregs (Munn and Mellor, 2007). As the only adaptive population that increased in the B16-IDO tumors was Foxp3+ Tregs (Fig. 3B), we hypothesized that the IDO-mediated activation of Tregs may be responsible for the observed MDSC expansion. To examine the role of Tregs in IDO-mediated MDSC recruitment, we first tested the frequency and suppressive capacity of the Tregs in the B16-IDO tumors. For this purpose, we used transgenic mice expressing Foxp3-GFP fusion protein (Foxp3GFP knock-in mice), which allowed us to specifically detect and sort live Foxp3+ Tregs (Fontenot et al., 2003). Similar to what we observed in WT mice (Fig. 3B), the frequency and absolute number of Foxp3+ Tregs was significantly higher in B16-IDO tumors than B16-WT tumors when tumors were harvested from Foxp3GFP mice (Fig. 7D). Moreover, Tregs purified from B16-IDO tumors were significantly more potent in suppressing CD8+ T-cell proliferation than Tregs from B16-WT tumors (Fig. 7E). These data show that tumor IDO expression changes both the quantity and the suppressive function of tumor-infiltrating Tregs.
Systemic depletion of Foxp3+ Tregs inhibits recruitment and functional activation of MDSC in IDO-expressing tumors
To evaluate the influence of Tregs on accumulation and activation of MDSCs in vivo, we utilized a transgenic mouse strain engineered with a transgene that expresses the diphtheria toxin receptor (DTR) under control of the Foxp3 promoter (Foxp3DTR mice). In Foxp3DTR mice, diphtheria toxin (DT) administration results in 90–95% depletion of Foxp3+ Tregs within 3 days of daily injection (Li et al., 2010). DT treatment resulted in near complete elimination of Foxp3+ Tregs in TDLNs, spleens and tumors in B16-IDO tumor-bearing Foxp3DTR mice (Fig. S7). Remarkably, DT treatment led to a significant reduction in CD11b+Gr1int MDSCs in the tumors and spleens (Fig. 7F). Furthermore, CD11b+Gr1int cells purified from B16-IDO tumors treated with DT were significantly less suppressive in vitro than CD11b+Gr1int cells from untreated B16-IDO tumors (Fig 7G). To investigate the role of Tregs on the recruitment of MDSCs to the tumor site, we performed migration experiments using B16-IDO tumors grown in Foxp3DTR mice treated with DT. Consistent with the findings described above, there was almost a complete loss of migration of CD11b+Gr1int splenocytes towards Treg-depleted B16-IDO tumors (Fig. 7H). Similar, findings were observed when B16-IDO tumors were pre-incubated with Tregblocking antibody in vitro prior to using them as attractants in the migration assay (Fig. 7H). Importantly, migration was partially restored by addition of supernatant from non-Treg depleted B16-IDO tumors of Foxp3DTR mice (Fig. 7H). Similarly, the migration of CD11b+Gr1int splenocytes to B16-IDO tumors harvested from Rag−/− mice could be restored by addition of B16-IDO tumor-infiltrating Tregs in vitro (Fig. 7H), but not by addition of tumor-infiltrating CD3+Foxp3− Teff (Fig. 7H). The migration was increased further by addition of both tumor-infiltrating Tregs and Teff cells (Fig. 7H). Altogether, these data suggest that tumor-infiltrating Tregs and to some extent effector T cells play a role in the recruitment and activation of suppressive MDSCs. Finally, depletion of Foxp3+ Tregs resulted in significantly greater accumulation of effector CD8+CXCR3+ T cells at the tumor site (Fig. 7I) and led to significant delay in tumor growth (Fig. 7J). Supporting these findings, we observed a significant increase in Foxp3+ Tregs in the IDO+ human melanoma tumor samples along with decrease in CD8+CD69+ T cells (Fig. 7K and L). In summary, our findings establish a link between IDO, Tregs, and MDSCs, which may help to explain the highly immunosuppressive nature of the IDO-expressing tumors. At present, the mechanisms of Treg-mediated MDSC recruitment and activation are not fully understood and further studies will be needed to investigate these pathways.
DISCUSSION
Tumors use a variety of suppressive strategies to escape from antitumor immune responses. In particular, upregulation of IDO in tumors has been associated with poor prognosis (Munn, 2012). IDO catabolizes the initial rate-limiting reaction of the kynurenine pathway. Several mechanisms of IDO-mediated immune suppression have been described. Both, tryptophan depletion and kynurenine accumulation exert immune regulatory effects leading amongst others to T-cell suppression and Treg induction (Munn and Mellor, 2007).
To better understand the mechanisms of tumor IDO-mediated immune suppression, here we developed a tumor model overexpressing IDO (B16-IDO), which allowed us to isolate the effects of IDO expression by comparing it to the parental B16-WT cell line. IDO-expressing B16 tumors exhibited aggressive tumor growth, and in addition to lack of tumor-specific T cells at the tumor site, unexpectedly were characterized by a high infiltration of CD11b+Gr1int cells, which were functionally suppressive in T-cell proliferation assays and exhibited phenotypic characteristics of MDSCs.
MDSCs are a heterogeneous population comprising monocytic and granulocytic cells that employ a broad variety of suppressive mechanism (Gabrilovich and Nagaraj, 2009). It is well established that MDSCs accumulate in the BM, blood, spleen and tumor of tumor-bearing mice and cancer patients and suppress T cell function (Gabrilovich and Nagaraj, 2009; Serafini et al., 2006). MDSCs are comprised of two subsets: cells with granulocytic phenotype that are Gr1high and cells with monocytic phenotype that are Gr1int (Gabrilovich and Nagaraj, 2009; Peranzoni et al., 2010; Serafini et al., 2006), with CD11b+Gr1int cells being most suppressive (Dolcetti et al., 2010). Our data demonstrated expansion of only CD11b+Gr1int monocytic MDSCs in the B16-IDO tumors and showed that only the CD11b+Gr1int population, but not CD11b+Gr1high cells suppressed T cell function in vitro. Interestingly, CD11b+Gr1int cells derived from B16-WT tumors were not suppressive, which was in concert with previous findings from our group (Lesokhin et al., 2012). These findings indicate that IDO expression by the tumor did not only lead to expansion of the CD11b+Gr1int population, but conferred suppressive properties upon these cells.
Previous studies have revealed a variety of mechanisms underlying suppression of T cells by MDSCs, including Arg1-mediated local depletion of nutrients (i.e. arginine and cysteine) required for adequate T cell activation, and expression of iNOS, which leads to NO production (Gabrilovich and Nagaraj, 2009). We found that CD11b+Gr1int cells within the B16-IDO tumors displayed higher levels of the suppressive enzymes Arg1 and NO, compared to CD11b+Gr1high cells, which could explain their distinct suppressive capacity. Accordingly, immune suppressive activity of CD11b+Gr1int MDSCs from B16-IDO tumors was partially blocked by inhibition of Arg1 and iNOS. While phenotypically CD11b+Gr1int MDSCs isolated from the B16-IDO tumors were very similar to the CD11b+Gr1int cells from the B16-WT tumors, only the B16-IDO MDSCs were suppressive. Additional phenotypic markers have been previously linked to MDSC function, including IL-4Rα, PD-L1, and TGFβ (Gabrilovich et al., 2012). To this end, CD11b+Gr1int MDSCs from B16-IDO tumors showed upregulation of all of these factors (i.e., Arg1, NO, IL-4Rα, PD-L1, and TGFβ) compared to CD11b+Gr1int cells from B16-WT tumors.
The findings above were not only restricted to the B16-IDO model, but were also noted in established 4T1 breast tumors naturally expressing high levels of IDO. Importantly, we saw a similar association in melanoma patients, where tumor expression of IDO was strongly correlated with increased tumor-infiltrating MDSCs. Finally, the recruitment and activation of MDSCs to the B16-IDO tumors could be partially reversed by pharmacological inhibition of IDO. These studies thus establish an important link between IDO expression and MDSC recruitment to tumors, and suggest a new pathway of IDO-mediated immunosuppression. These findings are in concert with previous studies demonstrating that genetic IDO deficiency results in impaired activity of MDSCs (Smith et al., 2012).
Our results further revealed that the CD11b+G1int subpopulation in mice with IDO+ tumors increased in numbers not just within the tumor, but in the spleen as well. Interestingly, the CD11b+G1int enriched from spleens of B16-IDO tumor-bearing mice did not show suppressive activity in vitro and displayed low levels of both Arg1 and iNOS. Thus, in our model, the full spectrum of MDSCs’ suppressive functions might not be acquired in the spleen but upon recruitment to the tumor. In support of this, we found that functional CD11b+G1int MDSCs accumulated in distant non-IDO expressing B16 tumors in a bilateral flank tumor model bearing B16-IDO on one flank and B16-WT tumors on the contralateral flank, and this was associated with increased tumor growth of the B16-WT tumors. These findings are in line with prior studies demonstrating that MDSCs actively migrate to the site of the tumor, where they upregulate the expression of Arg1 and iNOS, and downregulate the production of ROS (Kusmartsev et al., 2004).
The mechanisms by which MDSC functions are activated within the tumor microenvironment remain unclear. We observed upregulation of inflammatory cytokines and growth factors in the B16-IDO tumors, suggesting that the observed recruitment and activation of MDSCs may be a part of an adaptive immune response. Indeed, we found that CD11b+Gr1+ cells were not recruited, expanded or activated by the B16-IDO tumors grown in Rag−/− mice. T cells have previously been demonstrated to play an important role in activation of MDSCs in cancer (Gabrilovich and Nagaraj, 2009). Notably, we observed an increase in the frequency of tumor-infiltrating highly suppressive CD4+Foxp3+ Tregs in the B16-IDO tumors. Prior studies have shown that IDO can induce and activate CD4+Foxp3+ Tregs (Munn, 2012). These findings are suggestive of a link between IDO, Tregs, and MDSCs, and indeed, systemic depletion of Tregs in B16-IDO tumor-bearing mice significantly reduced the number of tumor-infiltrating CD11b+Gr1int cells and prevented migration of CD11b+Gr1int cells to IDO-expressing tumors in vitro. Furthermore, we found that the migration of CD11b+Gr1int splenocytes to B16-IDO tumors harvested from Rag−/− mice was completely restored by addition of Tregs. Finally, CD11b+Gr1int cells isolated form Treg-depleted mice were functionally impaired in their ability to suppress T cells. Thus, in addition to their direct T cell suppressive effects, IDO-induced Tregs may also play an essential role in the recruitment and activation of suppressive MDSCs. In support of these findings, several studies have demonstrated a correlation between MDCSs and Tregs in a variety of cancers including metastatic prostate cancer, glioblastoma and renal cell carcinoma (Gabrilovich et al., 2012), thus linking the two immune suppressive cells subsets in cancer patients.
In summary, our findings indicate that IDO expression by the tumors plays a key role in establishment of both local and systemic immunosuppression, which is mediated by expansion, recruitment, and activation of MDSCs in a Treg-dependent manner. The aberrant expression of IDO has been identified as a critical tipping point in progression of many different cancers (Munn, 2012), and a logical focus for targeted therapy (Liu et al., 2010). MDSCs have been shown to promote tumor growth, survival and treatment resistance in melanoma and other cancers (Gabrilovich and Nagaraj, 2009). Understanding the regulation of MDSC recruitment and function by IDO provides important insights into the link between IDO signaling and development of the immunosuppressive tumor microenvironment, and opens new opportunities for therapeutic intervention.
EXPERIMENTAL PROCEDURES
Tumor challenge and treatment experiments
All mouse procedures and experiments for this study were approved by the MSKCC Institutional Animal Care and Use Committee. Details on mice and cell lines are provided in Supplemental Experimental Procedures (SEP). On day 0 of the experiments, tumor cells were injected intradermally (i.d.) in the flank. Treatments were given as single agents or in combinations as described in SEP.
Isolation of tumor-infiltrating cells and lymphoid tissue cells
Single cell suspensions from mouse tissue were generated and stained with indicated antibodies for immediate analysis and/or flow sorting as described in SEP.
T cell suppression assay
CD8+ cells were purified from single cell suspensions from spleens of naive mice using anti-CD8 (Ly-2) microbeads (Miltenyi Biotech) according to manufacturer’s protocol and labeled with appropriate CellTrace™ Reagent. The labeled CD8+ T cells were then plated onto round bottom 96-well plates coated with 1 μg/ml anti-CD3 (clone 1454-2C11) and 5 μg/ml anti-CD28 (clone 37N). Purified suppressor cells (i.e. MDSCs or Foxp3+ Tregs) were added in indicated ratios and plates were incubated at 37°C. Percent CD8+ T-cell proliferation was measured by assessing dilution of the CellTrace Dye by flow cytometry after 48–72 hours of culture (LSRII flow cytometer, BD Biosciences). Controls were wells without suppressor cells (Stim+) and wells without suppressor cells and anti-CD3/CD28 antibody (Stim−). Details and specific conditions are provided in SEP.
Cell migration
Dissociated tumor cells were placed in the bottom chamber of a 24-well cell culture plate. Purified MDSC splenocytes were labeled with 1 mM CellTrace™ Far Red DDAO-SE (Thermo Fisher Scientific) and place in the upper chamber (3 μm cell culture inserts with PET membranes, BD Falcon). MDSCs were allowed to migrate to the bottom well for 24 hours. Migrated and labeled cells were then analyzed by flow cytometry on a LSRII Flow Cytometer (BD Bioscience) and quantified using Count Bright Absolute Counting Beads (Invitrogen). Percentage migration was calculated as: (number of migrated cells) × 100/(total cells added per well). IL-6, IL-10, MCP-1, IFNγ, TNF, and IL-12p70 recombinant proteins (500 pg/ml, BD Bioscience) (+) or media alone (−) were used for control chambers. Details and specific conditions are provided in SEP.
In vitro differentiation of MDSCs
Purified MDSC splenocytes were labeled with CellTrace™ Far Red DDAO-SE (Thermo Fisher Scientific) and cultured in complete RPMI media with or without dissociated tumor cells at indicated numbers. Two days later, cells were collected and DDAO-SE-labeled MDSCs were isolated by flow (FACSAria™ II Cell Sorter, BD Biosciences). Dead cells were excluded using DAPI (Invitrogen). The flow-sorted MDSCs were subsequently tested in T cell suppression assays as described above.
Cytokine measurements
Cytokine levels in homogenized tumors were measured using Luminex Mouse Cytokine Magnetic 20-Plex Panel (Invitrogen) according to the manufacturer’s instructions and as described in SEP.
In vivo BrdU staining
Tumor-bearing mice were injected with 2 mg BrdU i.p. After 1 hour, mice were sacrificed and BM, blood, spleen and tumor suspensions were prepared. BrdU incorporation in MDSCs was analyzed using the BD FITC BrdU Flow Kit (BD Bioscience) according to the manufacter’s instructions.
Arg1, NO and ROS activity
FACS-sorted MDSCs were washed and analyzed for Arg1, NO and ROS production as described in SEP.
Taqman gene expression analysis
Total RNA extraction and real-time analysis were performed as described in SEP.
Isolation of Pmel lymphocytes and adoptive transfer
Adoptive transfer of in vitro activated pmel cells was performed in mice 5 days after tumor challenge as decribed in SEP.
Treg depletion in mice
Foxp3DTR mice were injected i.p. on 5 consecutive days with DT (250ng/mouse, Sigma-Aldrich). DT treatment was initiated 8–9 days after tumor inoculation when tumors exhibited a volume of approximately 100mm3.
Patient material and analysis
Patient samples were collected on a tissue-collection protocol approved by the Institutional Review Board. Details on processing and analysis are provided in SEP.
Statistics
Statistics are described in SEP.
Acknowledgments
We would like to thank Hong Zhong, Beatrice Yin, and Yuri Igarash for technical assistance. In addition, we thank the Molecular Cytology Core Facility and the Flow Cytometry Facility at Memorial Sloan Kettering Cancer Center.
FUNDING
This work was supported by the Swim across America, Ludwig Institute for Cancer Research, and Breast Cancer Research Foundation. RBH is the recipient of postdoctoral fellowships through The Danish Cancer Society and The Carlsberg Foundation, Denmark. DZ is a Bart A. Kamen Fellow of the Damon Runyon Cancer Research Foundation and has received funding from the Bladder Cancer Awareness Network.
Footnotes
AUTHOR CONTRIBUTIONS
RBH developed the concept, designed, performed and analyzed experiments, and wrote the manuscript. DZ helped with TIL experiments, discussed experiments, and edited and communicated regarding the manuscript. YL and DM did IHC staining in patient tumor samples. BG processed patient samples. JPA, TM, and JDW supervised progress and edited the manuscript.COMPETING INTERESTS
The authors declare that they have no competing interests.
Publisher's Disclaimer: This is a PDF file of an unedited manuscript that has been accepted for publication. As a service to our customers we are providing this early version of the manuscript. The manuscript will undergo copyediting, typesetting, and review of the resulting proof before it is published in its final citable form. Please note that during the production process errors may be discovered which could affect the content, and all legal disclaimers that apply to the journal pertain.
References
- Dolcetti L, Peranzoni E, Ugel S, Marigo I, Fernandez Gomez A, Mesa C, Geilich M, Winkels G, Traggiai E, Casati A, Grassi F, Bronte V. Hierarchy of immunosuppressive strength among myeloid-derived suppressor cell subsets is determined by GM-CSF. Eur J Immunol. 2010;40:22–35. [Abstract] [Google Scholar]
- Fontenot JD, Gavin MA, Rudensky AY. Foxp3 programs the development and function of CD4+CD25+ regulatory T cells. Nat Immunol. 2003;4:330–336. [Abstract] [Google Scholar]
- Gabrilovich DI, Nagaraj S. Myeloid-derived suppressor cells as regulators of the immune system. Nat Rev Immunol. 2009;9:162–174. [Europe PMC free article] [Abstract] [Google Scholar]
- Gabrilovich DI, Ostrand-Rosenberg S, Bronte V. Coordinated regulation of myeloid cells by tumours. Nat Rev Immunol. 2012;12:253–268. [Europe PMC free article] [Abstract] [Google Scholar]
- Gallina G, Dolcetti L, Serafini P, De Santo C, Marigo I, Colombo MP, Basso G, Brombacher F, Borrello I, Zanovello P, Bicciato S, Bronte V. Tumors induce a subset of inflammatory monocytes with immunosuppressive activity on CD8+ T cells. J Clin Invest. 2006;116:2777–2790. [Europe PMC free article] [Abstract] [Google Scholar]
- Holmgaard RB, Zamarin D, Munn DH, Wolchok JD, Allison JP. Indoleamine 2,3-dioxygenase is a critical resistance mechanism in antitumor T cell immunotherapy targeting CTLA-4. J Exp Med. 2013;210:1389–1402. [Europe PMC free article] [Abstract] [Google Scholar]
- Kusmartsev S, Nefedova Y, Yoder D, Gabrilovich DI. Antigen-specific inhibition of CD8+ T cell response by immature myeloid cells in cancer is mediated by reactive oxygen species. J Immunol. 2004;172:989–999. [Abstract] [Google Scholar]
- Lesokhin AM, Hohl TM, Kitano S, Cortez C, Hirschhorn-Cymerman D, Avogadri F, Rizzuto GA, Lazarus JJ, Pamer EG, Houghton AN, Merghoub T, Wolchok JD. Monocytic CCR2(+) myeloid-derived suppressor cells promote immune escape by limiting activated CD8 T-cell infiltration into the tumor microenvironment. Cancer Res. 2012;72:876–886. [Europe PMC free article] [Abstract] [Google Scholar]
- Li X, Kostareli E, Suffner J, Garbi N, Hammerling GJ. Efficient Treg depletion induces T-cell infiltration and rejection of large tumors. Eur J Immunol. 2010;40:3325–3335. [Abstract] [Google Scholar]
- Liu X, Shin N, Koblish HK, Yang G, Wang Q, Wang K, Leffet L, Hansbury MJ, Thomas B, Rupar M, Waeltz P, Bowman KJ, Polam P, Sparks RB, Yue EW, Li Y, Wynn R, Fridman JS, Burn TC, Combs AP, Newton RC, Scherle PA. Selective inhibition of IDO1 effectively regulates mediators of antitumor immunity. Blood. 2010;115:3520–3530. [Abstract] [Google Scholar]
- Mombaerts P, Iacomini J, Johnson RS, Herrup K, Tonegawa S, Papaioannou VE. RAG-1-deficient mice have no mature B and T lymphocytes. Cell. 1992;68:869–877. [Abstract] [Google Scholar]
- Munn DH. Blocking IDO activity to enhance anti-tumor immunity. Frontiers in bioscience. 2012;4:734–745. [Abstract] [Google Scholar]
- Munn DH, Mellor AL. Indoleamine 2,3-dioxygenase and tumor-induced tolerance. J Clin Invest. 2007;117:1147–1154. [Europe PMC free article] [Abstract] [Google Scholar]
- Munn DH, Sharma MD, Hou D, Baban B, Lee JR, Antonia SJ, Messina JL, Chandler P, Koni PA, Mellor AL. Expression of indoleamine 2,3-dioxygenase by plasmacytoid dendritic cells in tumor-draining lymph nodes. J Clin Invest. 2004;114:280–290. [Europe PMC free article] [Abstract] [Google Scholar]
- Peranzoni E, Zilio S, Marigo I, Dolcetti L, Zanovello P, Mandruzzato S, Bronte V. Myeloid-derived suppressor cell heterogeneity and subset definition. Curr Opin Immunol. 2010;22:238–244. [Abstract] [Google Scholar]
- Prendergast GC, Smith C, Thomas S, Mandik-Nayak L, Laury-Kleintop L, Metz R, Muller AJ. Indoleamine 2,3-dioxygenase pathways of pathogenic inflammation and immune escape in cancer. Cancer Immunol Immunother. 2014;63:721–735. [Europe PMC free article] [Abstract] [Google Scholar]
- Serafini P, Borrello I, Bronte V. Myeloid suppressor cells in cancer: recruitment, phenotype, properties, and mechanisms of immune suppression. Semin Cancer Biol. 2006;16:53–65. [Abstract] [Google Scholar]
- Sharma MD, Baban B, Chandler P, Hou DY, Singh N, Yagita H, Azuma M, Blazar BR, Mellor AL, Munn DH. Plasmacytoid dendritic cells from mouse tumor-draining lymph nodes directly activate mature Tregs via indoleamine 2,3-dioxygenase. J Clin Invest. 2007;117:2570–2582. [Europe PMC free article] [Abstract] [Google Scholar]
- Smith C, Chang MY, Parker KH, Beury DW, DuHadaway JB, Flick HE, Boulden J, Sutanto-Ward E, Soler AP, Laury-Kleintop LD, Mandik-Nayak L, Metz R, Ostrand-Rosenberg S, Prendergast GC, Muller AJ. IDO is a nodal pathogenic driver of lung cancer and metastasis development. Cancer Discov. 2012;2:722–735. [Europe PMC free article] [Abstract] [Google Scholar]
- Youn JI, Nagaraj S, Collazo M, Gabrilovich DI. Subsets of myeloid-derived suppressor cells in tumor-bearing mice. J Immunol. 2008;181:5791–5802. [Europe PMC free article] [Abstract] [Google Scholar]
Citations & impact
Impact metrics
Citations of article over time
Alternative metrics
Smart citations by scite.ai
Explore citation contexts and check if this article has been
supported or disputed.
https://scite.ai/reports/10.1016/j.celrep.2015.08.077
Article citations
Influencing immunity: role of extracellular vesicles in tumor immune checkpoint dynamics.
Exp Mol Med, 11 Nov 2024
Cited by: 0 articles | PMID: 39528800
Review
Convergent inducers and effectors of T cell paralysis in the tumour microenvironment.
Nat Rev Cancer, 24 Oct 2024
Cited by: 0 articles | PMID: 39448877
Review
Insights into CSF-1R Expression in the Tumor Microenvironment.
Biomedicines, 12(10):2381, 18 Oct 2024
Cited by: 0 articles | PMID: 39457693 | PMCID: PMC11504891
Review Free full text in Europe PMC
Future investigative directions for novel therapeutic targets in head and neck cancer.
Expert Rev Anticancer Ther, 24(11):1067-1084, 16 Oct 2024
Cited by: 0 articles | PMID: 39412140
Review
Tumour-intrinsic endomembrane trafficking by ARF6 shapes an immunosuppressive microenvironment that drives melanomagenesis and response to checkpoint blockade therapy.
Nat Commun, 15(1):6613, 04 Aug 2024
Cited by: 0 articles | PMID: 39098861 | PMCID: PMC11298541
Go to all (265) article citations
Data
Data behind the article
This data has been text mined from the article, or deposited into data resources.
BioStudies: supplemental material and supporting data
Similar Articles
To arrive at the top five similar articles we use a word-weighted algorithm to compare words from the Title and Abstract of each citation.
Myeloid-derived suppressor cells suppress antitumor immune responses through IDO expression and correlate with lymph node metastasis in patients with breast cancer.
J Immunol, 190(7):3783-3797, 25 Feb 2013
Cited by: 372 articles | PMID: 23440412
Tumor-infiltrating Treg, MDSC, and IDO expression associated with outcomes of neoadjuvant chemotherapy of breast cancer.
Cancer Biol Ther, 19(8):695-705, 08 May 2018
Cited by: 85 articles | PMID: 29621426 | PMCID: PMC6067871
Noncanonical NF-κB activation mediates STAT3-stimulated IDO upregulation in myeloid-derived suppressor cells in breast cancer.
J Immunol, 193(5):2574-2586, 25 Jul 2014
Cited by: 116 articles | PMID: 25063873 | PMCID: PMC4719564
IDO, PTEN-expressing Tregs and control of antigen-presentation in the murine tumor microenvironment.
Cancer Immunol Immunother, 66(8):1049-1058, 09 May 2017
Cited by: 23 articles | PMID: 28488123 | PMCID: PMC5627605
Review Free full text in Europe PMC
Funding
Funders who supported this work.
Breast Cancer Research Foundation
Carlsbergfondet
Damon Runyon Cancer Research Foundation
Kræftens Bekæmpelse
Ludwig Institute for Cancer Research
NCI NIH HHS (3)
Grant ID: R25 CA020449
Grant ID: P30 CA008748
Grant ID: R01 CA056821