Abstract
Purpose
Early and accurate assessment of radiation injury by radiation-responsive biomarkers is critical for triage and early intervention. Biofluids such as urine and serum are convenient for such analysis. Recent research has also suggested that exosomes are a reliable source of biomarkers in disease progression. In the present study, we analyzed total urine proteome and exosomes isolated from urine or serum for potential biomarkers of acute and persistent radiation injury in mice exposed to lethal whole body irradiation (WBI).Methods and materials
For feasibility studies, the mice were irradiated at 10.4 Gy WBI, and urine and serum samples were collected 24 and 72 hours after irradiation. Exosomes were isolated and analyzed using liquid chromatography mass spectrometry/mass spectrometry-based workflow for radiation exposure signatures. A data dependent acquisition and SWATH-MS combined workflow approach was used to identify significantly exosome biomarkers indicative of acute or persistent radiation-induced responses. For the validation studies, mice were exposed to 3, 6, 8, or 10 Gy WBI, and samples were analyzed for comparison.Results
A comparison between total urine proteomics and urine exosome proteomics demonstrated that exosome proteomic analysis was superior in identifying radiation signatures. Feasibility studies identified 23 biomarkers from urine and 24 biomarkers from serum exosomes after WBI. Urinary exosome signatures identified different physiological parameters than the ones obtained in serum exosomes. Exosome signatures from urine indicated injury to the liver, gastrointestinal, and genitourinary tracts. In contrast, serum showed vascular injuries and acute inflammation in response to radiation. Selected urinary exosomal biomarkers also showed changes at lower radiation doses in validation studies.Conclusions
Exosome proteomics revealed radiation- and time-dependent protein signatures after WBI. A total of 47 differentially secreted proteins were identified in urinary and serum exosomes. Together, these data showed the feasibility of defining biomarkers that could elucidate tissue-associated and systemic response caused by high-dose ionizing radiation. This is the first report using an exosome proteomics approach to identify radiation signatures.Free full text

Identifying Urinary and Serum Exosome Biomarkers For Radiation Exposure Using A DDA and SWATH-MS Combined Workflow
Abstract
Background
Early and accurate assessment of radiation injury by radiation-responsive biomarkers is critical for triage and early intervention. Biofluids such as urine and serum are convenient for such analysis, recent research also suggests that exosomes are a reliable source of biomarkers in disease progression. In this study, we analyzed total urine proteome, and exosomes isolated form urine or serum for potential biomarkers of acute and persistent radiation injury in mice exposed to lethal whole body irradiation (WBI).
Methods
For feasibility studies, mice were irradiated at 10.4 Gy WBI, urine and serum samples were collected 24 and 72 hr post-irradiation. Exosomes were isolated and analyzed by LC-MS/MS- based workflow for radiation exposure signatures. A DDA and SWATH-MS combined workflow approach was used to identify significantly exosome biomarkers indicative of acute or persistent radiation-induced responses. For validation studies, mice were exposed to 3, 6, 8 or 10 Gy WBI and samples were analyzed for comparison.
Results
Comparison between total urine proteomics and urine exosome proteomics demonstrated that exosome proteomic analysis was superior in identifying radiation signatures. Feasibility studies identified 23 biomarkers from urine and 24 biomarkers from serum exosomes post-WBI. Urinary exosome signatures identified different physiological parameters than the ones obtained in serum exosomes. Exosome signatures from urine indicated injury of the liver, gastrointestinal, and genitourinary track, whereas serum showed vascular injuries and acute inflammation in response to radiation. Selected urinary exosomal biomarkers also showed changes at lower radiation doses in validation studies.
Conclusion
Exosome proteomics revealed radiation- and time-dependent protein signatures after WBI. 47 differentially secreted proteins were identified in urinary and serum exosomes, together these data showed the feasibility of defining biomarkers that could elucidate tissue-associated and systemic response caused by high dose ionizing radiation. This is a first report employing exosome proteomics approach to identify radiation signatures.
INTRODUCTION
Exposure to IR results in potentially life incapacitating injuries if not diagnosed and treated early [1,2]. While severe radiation injuries lead to acute response and eventually death, non-lethal (small or local) exposure may give rise to delayed injuries such as organ fibrosis, myeloid dysplastic syndrome and cancers. Fast, accurate, and sensitive methodologies are needed to diagnose exposure incidence (dose and time) for providing appropriate medical support. Diagnostic biomarkers that can be reliably detected in biofluids are ideal for early assessment of radiation exposure or injury. Additionally, in the event of mass causality scenario when screening large numbers of individuals is not possible in the first 24 hours, biomarker analysis days after exposure is also equally critical for triage. Urine, in particular being non-invasive and easy to collect, has been a primary focus for identifying radiation injury signatures. Some of the earlier exploratory studies of urine proteome have indicated that biological fluids such as serum and urine could be a promising source for identifying diagnostic markers of radiation exposure [3–5]. Metabolomic analysis of urine samples by Tyburski et al [6] showed some markers varying with radiation dose but no dose- or time- correlation was achieved for more than one time point. In addition, the markers observed showed saturation at a non-lethal dose of 3 Gy, thus making them highly impractical in determination of radiation doses that are sublethal to lethal, precisely the kind of injuries that need medical intervention to improve the survival outcome.
This is a first report on proteomic analysis of urine and serum to identify time dependent radiation signatures using DDA and DIA approaches. We also focused on characterizing urinary and serum exosomes for identifying biomarkers indicative of radiation exposure and/or radiation-induced tissue injuries. Exosomes are powerful messengers released by all cells under normal as well as stressed conditions into biofluids [7,8] and provide valuable information on disease progression for various disease models, including cancer [9]. Urine exosomes were reported to provide information about the physiological or pathological state of renal cells or origin involving glomerular podocytes [10] Therefore urine and serum exosomes were used to identify early and delayed radiation signatures in lethally irradiated mice. Our analysis identified several biomarkers in urine and serum exosomes with high precision, and potential radiation-responsive pathways in various tissues.
METHODS
Details of the experimental protocols are described in supplementary information (SI).
Animals and Irradiation Procedure
Six -eight weeks old mice were purchased from Charles River Laboratories and subcontracted from NCI, (Fort Dietrick, MD) and housed at the institutional animal facility. For feasibility studies, anesthetized mice were exposed to 10.4 Gy WBI (236cGy/min) using Cesium irradiator as described elsewhere [11]. 10.4 Gy dose was chosen based on our previous studies that established bone marrow and gastrointestinal injury in mice leading to mortality within 2 weeks post-WBI. Mice were returned to their cages with food and water ad libitum. For validation studies, anesthetized mice were exposed to 3, 6, 8, and 10 Gy WBI using the same irradiation protocol. All procedures were conducted by strictly adhering to the institutional IACUC-approved guidelines and protocols.
Sample Collection, Exosome isolation and LC-MS analysis
12hrs prior to sacrifice all mice were food restrained. Animals were lightly anesthetized and blood was collected by retro-orbital sinus bleeding and processed for serum isolation. Serum and Urine exosomes were isolated and analyzed by LC-MS/MS (SI). For the irradiated group, urine was collected at 24 and 72hr, representing the early and delayed responses. For total urine proteome analysis, 20ul from each urine sample (n = 5) were run on SDS-PAGE, subdivided into 6 gel pieces, and proteolytically cleaved to extract peptides for mass spectrometry-based proteomic analysis. For urine exosome proteome analysis, 50μl of urine were used to isolate total exosome (Life Technology, Inc.). 15μg of exosome lysates (n = 5) were run on SDS-PAGE, bands were isolated, digested and run on LC-MS/MS. Similar protocol was followed for serum samples. The sample preparation and MS workflow is illustrated in Figure 1A.
Targeted SRM analysis
A list of precursor and product ion masses of 2 or 3 peptides from EGF, PLAU, VDAC, and HEXB was generated by the Skyline and used to perform targeted MS analysis. The following peptides were used to show quantitative MS results. EGF – INLHPSFVTPGK. PLAU – ESESDYLYP. VDAC – LTFDSSFSPNTGK. HEXB – VEPLNFEGSEK.
Exosome Analysis
Spectral counts of identified proteins (computed as described in SI) were loaded into the Qlucore Omics Explorer (Qlucore AB, Sweden) software, and filtered for uninformative features. Statistical analysis of quantifiable proteins was conducted using the linear model for microarray (LIMMA) framework and FDR-based correction for multiple comparisons.
RESULTS
Global comparison of urine and urinary exosome proteome in response to radiation
Total spectra counts were used to perform global normalization among samples using the Scaffold software. 580 and 235 proteins were identified from the total urine proteome and urine exosome respectively. LIMMA was applied to both datasets to identify proteins that showed radiation dose- and time-dependent changes in urine and serum exosomes. PCA was used to visualize the separation of three experimental groups. We were able to identify 23 proteins from the urine exosome proteome with less than 5% FDR (q < 0.05, adjusted p-value < 0.0214, Figure 1B); however, no protein was found in the whole urine proteome when the same FDR was applied. These results suggest exosome analysis may prove useful in molecular diagnostics of radiation injury.
DDA and DIA combined approach for radiation signatures in serum and urine exosomes
One of the challenges of utilizing the label-free LC-MS workflow is the sensitivity of protein detection and quantification. Traditional LC-MS workflow based on DDA increases overall identification of proteins but suffers from reduced numbers of identifiable peptides across multiple runs [12,13]; DIA on the hand, presents an attractive solution to improve label-free quantification [14]. Additionally, fractionation of total urine and urine exosome also reduced repeated sampling of high abundance proteins and increased the depth of protein identification.
For urine exosome characterization, 274 unique proteins were identified from DDA and 178 unique proteins were identified from DIA respectively (Fig. 2). 384 proteins remained after filtering for low variance, corresponding to 263 unique urine exosome proteins used for analysis. For serum exosome characterization, 434 unique proteins were identified from DDA and 220 unique proteins identified from DIA respectively (Fig. 2). 478 proteins remained after filtering, corresponding to 317 unique serum exosome proteins used for analysis.
GeneOntology analysis
To determine subcellular location and function of the proteins identified in our samples, we used GeneOntology (GO) analysis. Majority of urine and serum exosome proteins were found to be from three subcellular locations: plasma membrane, cytoplasm, and extracellular space (Fig. 3A, top). Urinary exosomes showed significantly increased levels of plasma membrane protein (52.4%) compared to the serum exosomes (13.3%) while, only serum exosomes showed significant increase in nuclear proteins (7.4%).
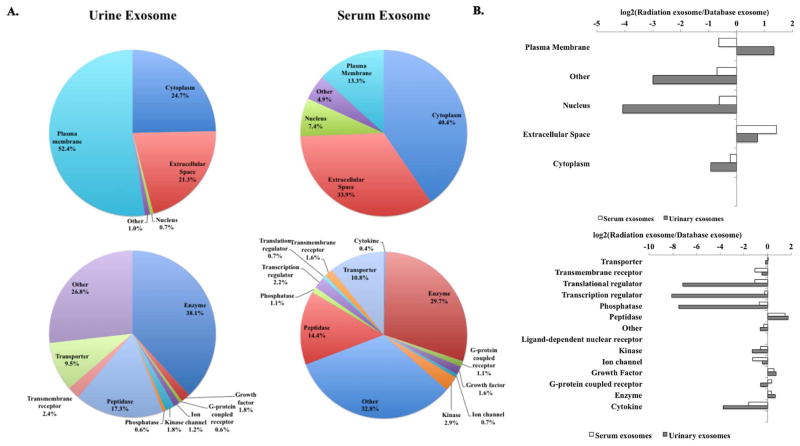
(A) Total urine and serum exosome unique proteins categorized based on subcellular localization and molecular functions re shown. (B) Urinary and serum exosome proteome obtained using GO classification compared to the proteomic dataset from the Vesiclepedia exosome database.
Both urinary and serum exosome hits showed diverse molecular functions, serum exosome analysis identified transporters, transcriptional regulators, phosphatases, G-protein coupled receptors, cytokines and growth factors. Although many were classified as other (function not known), three major categories emerged in urine and serum exosomes identified in this study: enzyme, peptidase, and transporter (Fig. 3A, bottom). We expanded our comparison to examine the subcellular and functional categories of proteins identified in our dataset to the proteomic dataset from the Vesiclepedia exosome database [15] and found that the urinary exosome showed over two-fold increase in plasma membrane proteins compared to the serum exosomes (Fig. 3B, top). Furthermore, both urinary and serum exosome proteins in our dataset showed two-fold or more increase in extracellular proteins than in the Vesicleopedia database (Fig. 3B, top). While serum exosome proteins had a similar distribution of molecular functions except peptidases (> 2 fold increase), striking reduction in transcriptional regulators, translational regulators, and phosphatases from urinary exosomes was observed (Fig. 3B, bottom).
Identification of radiation biomarkers from urinary exosomes
From the urinary exosome dataset, 23 proteins were altered post-WBI with 5% FDR and adjusted p-value < 0.004 (Fig. 4A). Most of radiation sensitive biomarkers in urinary exosomes showed elevated levels in irradiated mice compared to the controls. Among these, 14 showed at least 2 fold higher expression (Fig. 4A); these biomarkers also indicated alteration in radiation-responsive signaling pathways such as redox, apoptosis and inflammatory response. These included PRDX6, a non-selenium thioredoxin with phospholipase A2 activity [16]. PRDX6 is highly expressed in lungs, liver, kidney, testis, and brain, especially in the epithelium [17,18]. VDAC1, an outer mitochondrial membrane protein known to be crucial for regulation of mitochondrial function [19], was an early response protein observed to be elevated at 24 h and remained elevated at 72h (6A). VDAC1 is a key pro-apoptotic molecule in cytochrome C mediated apoptosis, and therefore is a surrogate marker for increased apoptosis in kidney at 24 and 72 hr post-WBI. [20]. PLAU, a serine kinase was significantly lower in irradiated cohorts, again indicative of increased apoptosis [21]. Others proteins included PLD3 and UROM, which were also reported as biomarkers of renal injury and graft rejection in renal transplantation patients [22]. These markers therefore represent acute and persistent renal epithelial tissue damage occurring in response to WBI. Other biomarkers of early response (only at 24 hr) included A2M [23,24], ALB, GLB, HEXB, AGT, and GALNS, routinely found in urine and lysosomes.
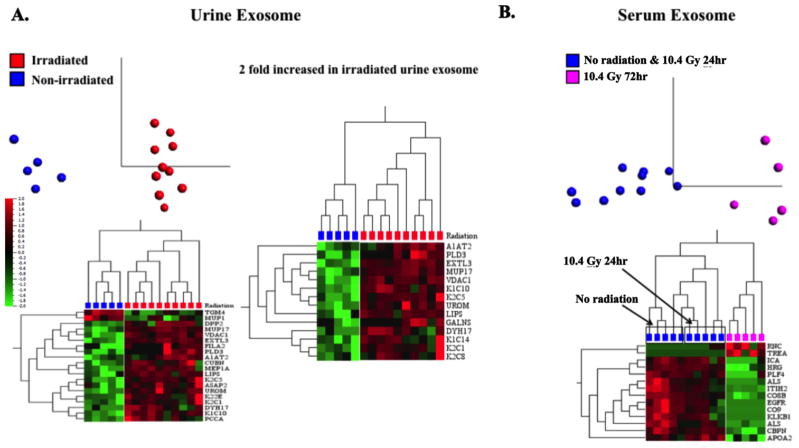
(A) PCA showed a distinct separation of these two groups of mice (p<0.05). A heat map showed the differential expression of 20 urine exosome proteins in response to radiation exposure. A second heat map displaying proteins had at least a 2-fold increase in irradiated mice in response to radiation compared to the non-irradiated mice. (B) A distinct protein signature was found in serum exosomes between the first two groups of mice (non-irradiated and 10.4 Gy 24hr) and the third group of mice (10.4 Gy 72hr) (p<0.05).
Relative quantification of peptides from urinary exosome biomarkers using Skyline peak area quantification was also conducted on urine exosome proteome. The left panel in Figure 5 shows spectral counts for various proteins identified in groups I(top), II(middle), and III(bottom). As indicated in the right panel, VVFIFGPDK, derived from PRDX6 from the first group (top) and QFYSVFDR, derived from CATE from the second group (middle) confirms that these proteins indeed showed radiation-induced reduction in the spectral counts. WTEYGLTFTEK, derived from VDAC1 shown in the third group shows radiation-induced increased counts in urine exosomes.
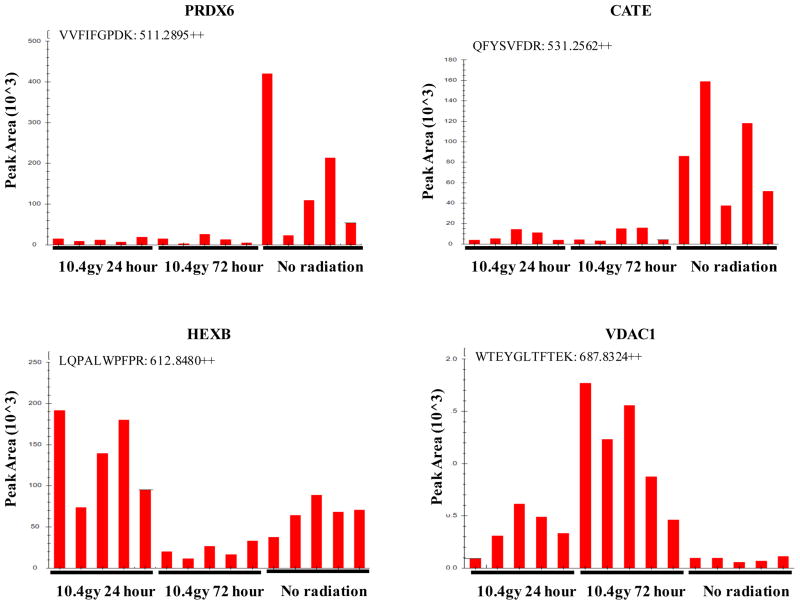
Top Row - Down-regulated with radiation: VVFIFGPDK, derived from PRDX6 and extracted by precursor ion at m/z 511.2895 (2+) and QFYSVFDR, derived from CATE and extracted by precursor ion at m/z 531.2562 (2+). Bottom Left - Acute-phase response: LQPALWPFPR, derived from HEXB and extracted by precursor ion at m/z 612.8480 (2+). Bottom Right - Late-phase response: WTEYGLTFTEK, derived from VDAC1 and extracted by precursor ion at m/z 687.8324 (2+).
Identification of radiation biomarkers from serum exosomes
Exosome protein profiles of serum samples with 5%FDR and adjusted p-value < 0.009 are shown in Fig. 4B. Figure 6B shows key proteins that were identified in serum exosome analysis. A2M, which was down-regulated in urine exosomes at 72 hour post-WBI, is also an APP known to have beneficiary effects on sepsis in terms of recruitment of neutrophil at the site of infection or on the surface of endothelial cells [23]. A2M levels were correlated with surviving sepsis patients [25]. It is interesting that A2M was the only APP marker that was identified in urine exosomes; whereas, several APP markers such as SAA1 and 2, SPA3N, APOA2 were identified in serum exosomes [23,24]. Also, the kinetics of APP changes detected in serum versus urine was different. Most APP in serum exosomes were early responsive and were highly elevated at 24 hr post-WBI, and returned to normalcy by 72 hr post-WBI, the only APP found in urine (A2M) was slow responsive and was down-regulated at 72 h, interestingly it was not identified in serum exosomes. Additionally, progressive reduction of fibrinolysis (KLKB1 and PROS1), cell motility/adhesion factors (EGFR, HRG, IGFALS, and TF), and alternative complement pathway components (C8A, C8B, C8G, and C9) was observed in serum exosome biomarkers after radiation exposure (Fig. 6B).
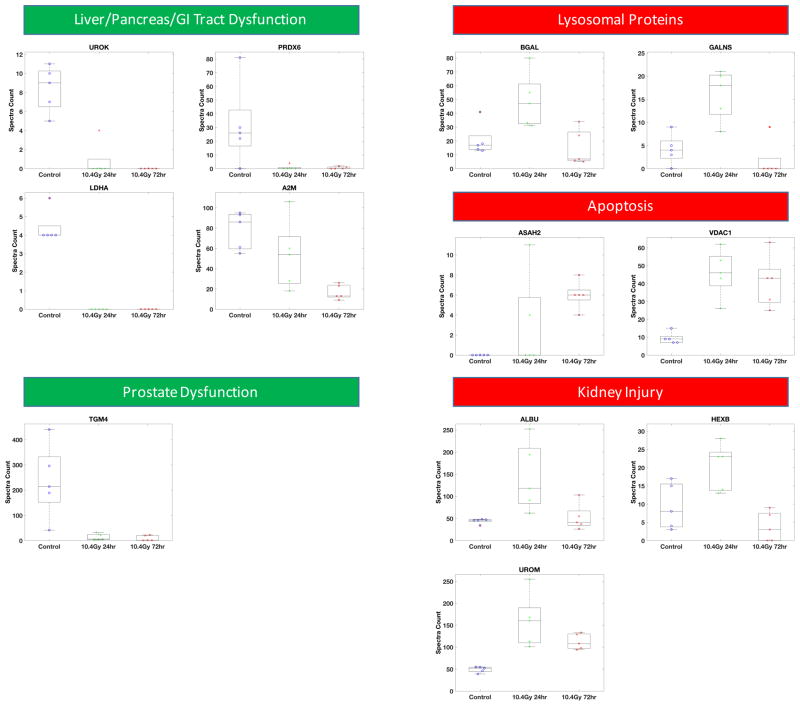

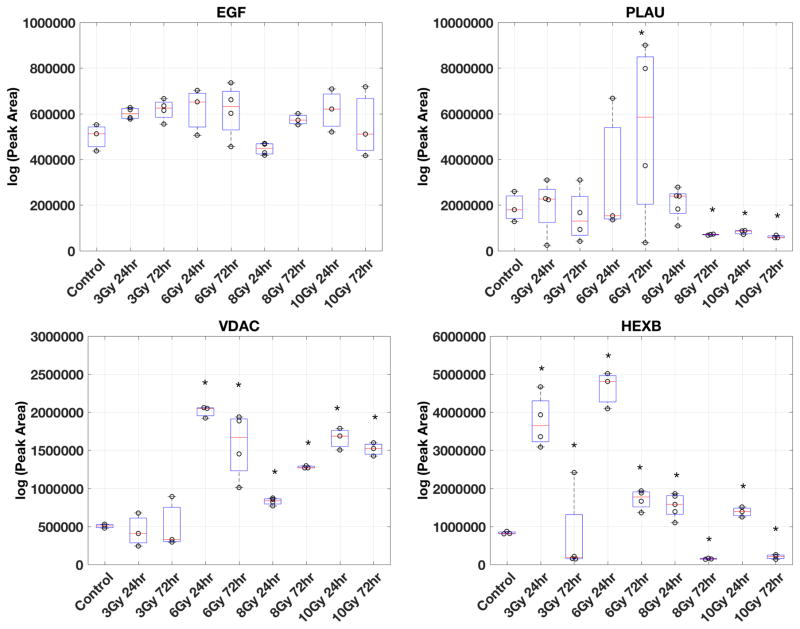
Five functional categories of urinary exosome biomarkers (A) and four functional categories of serum exosome biomarkers (B) are shown. Examples of proteins in each functional category from the biomarker panel are shown. Semi-quantitative measurements of protein abundance (spectra counts) and variations among biological replicates are plotted for each protein in each group (control, 10.4 Gy 24hr, and 10.4 Gy 72hr). Nice groups of samples were collected for targeted MS analysis (C). Group 1: urine from non-irradiated mice (Control). Group 2 & 3: urine from 3 Gy WBI mice collected at 24 hours (3 Gy 24hr) or collected at 72 hours (3 Gy 72hr). Group 4 & 5: urine from 6 Gy WBI mice collected at 24 hours (6 Gy 24hr) or collected at 72 hours (6 Gy 72hr). Group 6 & 7: urine from 8 Gy WBI mice collected at 24 hours (8 Gy 24hr) or collected at 72 hours (8 Gy 72hr), Group 8 & 9: urine from 10 Gy WBI mice collected at 24 hours (10 Gy 24hr) or collected at 72 hours (10 Gy 72hr). Urine exosome was isolated from urine collected from each group of mice (method described in the Methods). Peptides from four proteins (EGF, PLAU, VDAC, HEXB) were used to acquire targeted measurements from urine exosome. The Skyline software generated the peak area of each peptide and the log value of peak area was used in the boxplot to show the variability and expression differences among these 9 groups of mice. Student t-test was used to determine if the expression level of each protein from irradiated urine exosome comparing to urine exosome from the non-irradiated controls. * above the boxplot indicates significantly altered peak area (p<0.05) in the irradiated group comparing to the control group.
Validation of radiation biomarkers from urinary exosomes
Global profiling of proteome provides a catalogue of proteins with semi-quantitative measurements for relative quantitative comparisons among samples. To verify the precision of these semi-quantitative measurements, we employed MS-based targeted protein analysis, (SRM) to obtain quantitative measurements of several potential biomarkers of radiation-induced injuries identified from urine exosomes. In addition, we also studied changes of these biomarker candidates at lower doses of radiation and early (24hr) as well as late (72hr) time points by the targeted MS analysis.
Using the semi-quantitative data analyzed by the Skyline as described in figure 5, we selected 2–3 peptides from EGF, PLAU, VDAC, and HEXB. An inclusion list containing the precursor ion and fragment ion masses of these peptides was uploaded to the Orbitrap Fusion Tribrid mass spectrometer to perform targeted MS analysis. EGF was found in our study to have consistent expression in all urine exosome lysates regardless of radiation doses or time of collection and was used as the loading control. Acquired SRM data were uploaded to the Skyline software and the peak area (ion intensity x retention time) for each peptide was generated and exported as a list for quantitative analysis. Urine exosome lysates from at least two mice were analyzed per group (n=2–5 mice per group). MS-based targeted analysis of a representative peptide from each protein at different radiation doses and time is shown in figure 6C. As shown in figure 6C, there was no significant difference in EGF levels at 3, 6, 8 and 10 Gy at 24 and 72 h post-WBI. PLAU, VDAC, and HEXB showed similar trends in urine exosomes from 10 Gy WBI mice collected at 24 and 72 hours as predicted by the shotgun proteomics data (Fig. 6C). Interestingly, these three proteins showed different changes at the lower doses of WBI. PLAU levels were significantly lower in 8 Gy WBI mice at 72 hours compared to 24 h and the trend persisted in 10 Gy WBI samples at both time points (Fig. 6C, upper right panel). VDAC was consistently elevated starting at 6 Gy at 24 hours and remained elevated at both time points at 8 and 10 Gy (Fig. 6C lower left panel). Similarly, changes in HEXB levels could be detected as early as 3 Gy WBI at 24 hours (Fig. 6C, lower right panel). Together, our findings suggested that these biomarker candidates, found at the lethal dose WBI (10.4 Gy) in the feasibility studies, could also be useful biomarkers for radiation exposure monitoring and support our on-going research effort of identifying biomarkers for radiation dosimetry from urine exosome.
DISCUSSION
We tested whether exosomes from biofluids can be used for radiation biomarker analysis. Our findings demonstrate that exosomes were not only a viable choice of sample for radiation biomarker analysis, but they also proved to be superior in identifying protein signatures related to radiation exposure. Additionally, exosomes provided valuable information about cellular physiology and pathology, thus identifying pathways that are altered due to the injury or event in question.
As shown in Table 1 (SI), proteins identified in urine exosome are expressed in genitourinary track and almost all of them (except PBSN and ASAH2) have been detected in extracellular vesicles in biofluids and various cell types indicating that these proteins are secreted from cells ubiquitously. In contrast to the biomarkers identified in urinary exosomes, serum exosome proteins are moderately expressed in a wider range of tissues, including other major circulation systems such as cardiovascular and respiratory systems (Table 2a, SI). All of these proteins have been detected in extracellular vesicles, in biofluids and in various cell types (Table 2b, SI). Urine exosomes showed much greater enrichment of plasma membrane proteins while serum exosomes showed enrichment of cytosolic and nuclear fraction. It is known that membrane proteins are shed into urine by kidney in response to irradiation [3,4]. Ahram et al., reported increased shedding of membrane proteins attributable to radiation [26], our finding of increased plasma membrane proteins in urine exosomes and increased peptidases in urine and serum exosomes provide additional in vivo evidence that proteins from plasma membrane could be actively transferred to exosomes and could potentially play a role in eliciting a systemic response in circulation. Furthermore, striking differences of transcriptional and translational regulators secreted in urinary and serum exosomes in the WBI mice were observed, which suggests that serum and urine could reflect different physiological changes in response to radiation exposure. These findings are consistent with our hypothesis that serum and urine harbor different physiological changes in response to radiation exposure in a time-dependent manner. These differential responses were also time-sensitive, urine samples showed changes early on (+24h) while serum samples showed delayed (+72h) response. Urinary biomarkers were indicative of tissue dysfunction, acute kidney injury, increased apoptosis, and dysregulation of lysosomal proteins. Serum exosome biomarkers mirrored systemic symptoms developed in individuals diagnosed with acute radiation syndrome (ARS). Elevation of several acute phase proteins in WBI mice indicated rapid systemic inflammatory response possibly due to damage of vascular endothelial cells. Interestingly, some cytokines (IL6) and acute response proteins (CRP) reported to show radiation-responsive profile [27] were not identified in our serum exosomes analysis suggesting that exosome analysis can be restrictive and may miss important cues otherwise detectable in whole serum analysis.
Table 1
Spectra counts from unique peptides were used as quantitative measurements of protein expression. Red indicates at least 1.5 fold higher expression in the irradiated mice compared to the non-irradiated mice. Green indicates at least 2 fold lower in the irradiated mice compared to the non-irradiated mice. Black indicates no change in protein expression. Expression level of each protein in normal tissue was denoted based on The Human Protein Atlas.
Normal Tissue Expression | Exosomes | ||||||||||||||||
---|---|---|---|---|---|---|---|---|---|---|---|---|---|---|---|---|---|
Accession # | Symbol | 24 h | 72 h | Liver | Pancreas | Kidney/Bladder | Prostate/Testis | GI Tract | Kidney Failure | Glomerular Injury | Plasma | Urine | Cancer | Immune Cells | MSC | Addipocytes | Additional |
Group 1 (11) | |||||||||||||||||
Q8BZH1 | TGM4 | ++ | x | x | |||||||||||||
O08709 | PRDX6 | ++ | ++ | ++ | ++ | ++ | x | x | x | x | Thymocytes | ||||||
P06869 | PLAU | + | + | x | x | ||||||||||||
Q8BFR4 | GNS | + | + | + | x | Retinal epithelial Cells | |||||||||||
P03953 | CFD | ++ | + | x | x | ||||||||||||
Q6QRO59 | Fam115E | ||||||||||||||||
O08976 | PBSN | + | |||||||||||||||
P06151 | LDHA | + | + | + | + | x | Thymocytes | ||||||||||
P70269 | CTSE | + | + | ++ | x | ||||||||||||
P45700 | MAN1A1 | ++ | + | ++ | + | ++ | x | x | |||||||||
PO8226 | APOE | ++ | + | ++ | x | ||||||||||||
Group 2 (1) | |||||||||||||||||
P11591 | MUP5 | x | |||||||||||||||
Group 3 (2) | |||||||||||||||||
Q61838 | A2M | + | x | x | x | x | x | Synovial fluid | |||||||||
P13366 | GZMG | ||||||||||||||||
Group 4 (3) | |||||||||||||||||
P07724 | ALB | + | + | ++ | + | x | x | x | x | Thymocytes | |||||||
P23780 | GLB1 | ++ | + | + | + | x | x | ||||||||||
P11859 | AGT | + | + | + | + | x | x | x | x | ||||||||
Group 5 (2) | |||||||||||||||||
P20060 | HEXB | + | + | + | x | x | Retinal epithelial cells | ||||||||||
Q571E4 | GALNS | ++ | ++ | ++ | ++ | ++ | x | ||||||||||
Group 6 (4) | |||||||||||||||||
Q91X17 | UMOD | ++ | x | x | x | ||||||||||||
Q60932 | VDAC1 | + | ++ | ++ | ++ | ++ | x | x | Thymocytes | ||||||||
Q9JHE3 | LGALS3BP | ++ | ++ | + | x | x | x | x | x | Saliva | |||||||
Q9JHE3 | ASAH2 |
++ indicates high expression and + indicates medium expression in the specified tissue. X marks the detection of the protein in the Vesicleopedia database.
Table 2a
Red indicates at least 1.5 fold higher expression in the irradiated mice compared to the non-irradiated mice. Green indicates at least 2 fold lower in the irradiated mice compared to the non-irradiated mice. Black indicates no change in protein expression. Expression level of each protein in normal tissue was denoted based on The Human Protein Atlas.
Normal tissue Expression | ||||||||||||||
---|---|---|---|---|---|---|---|---|---|---|---|---|---|---|
Accession # | Symbol | 24 h | 72 h | Liver | Pancreas | GI Tract | Salivary Gland | Endocrine gland | Kidney | Testis | Blood and Immune system | CNS | Respiratory System | Cardiovascular system |
Group1 (2) | ||||||||||||||
Q91WP6 | SPA3N | ++ | ||||||||||||
P41317 | MBL2 | |||||||||||||
Group 2 (2) | ||||||||||||||
P05366 | SAA1 | |||||||||||||
P12246 | SAMP | ++ | + | |||||||||||
Group 3 (3) | ||||||||||||||
P05367 | SAA2 | |||||||||||||
P09813 | APOA2 | |||||||||||||
O88947 | FA10 | |||||||||||||
Group 4 (4) | ||||||||||||||
Q9DBD0 | ICA | |||||||||||||
Q9ESB3 | HRG | + | ||||||||||||
P13020 | GSN | + | + | + | + | + | + | |||||||
Q9JN5 | CPN1 | + | + | ++ | + | ++ | ++ | |||||||
Group 5 (13) | ||||||||||||||
Q9Z126 | PF4 | + | ||||||||||||
Q8bh35 | C8B | + | + | + | + | + | ||||||||
Q61703 | ITIH2 | |||||||||||||
Q61702 | ITIH1 | + | + | + | + | |||||||||
P70389 | IGFALS | |||||||||||||
Q8K182 | C8A | |||||||||||||
P26262 | KLKB1 | ++ | + | + | ++ | ++ | ++ | ++ | ++ | + | ||||
Q01279 | EGFR | + | + | + | + | ++ | ||||||||
Q08761 | PROS1 | + | + | + | + | + | + | |||||||
P06683 | C9 | + | + | |||||||||||
Q9Z2U1 | PSMA5 | + | + | + | ++ | + | ++ | + | + | |||||
Q8VCG4 | C8G | + | + | ++ | + | ++ | ++ | + | ||||||
Q92111 | TF |
++ indicates high expression and + indicates medium expression in the specified tissue.
Table 2b
X marks the detection of the protein in the Vesicleopedia database.
Exosomes | |||||||||
---|---|---|---|---|---|---|---|---|---|
Accession # | Symbol | 24 h | 72 h | Plasma | Urine | Cancer | Immune Cells | MSC | Additional Information |
Group1 (2) | |||||||||
Q91WP6 | SPA3N | x | |||||||
P41317 | MBL2 | x | x | Saliva | |||||
Group 2 (2) | |||||||||
P05366 | SAA1 | x | x | x | x | ||||
P12246 | SAMP | x | Saliva | ||||||
Group 3 (3) | |||||||||
P05367 | SAA2 | x | x | x | Saliva | ||||
P09813 | APOA2 | ||||||||
O88947 | FA10 | ||||||||
Group 4 (4) | |||||||||
Q9DBD0 | ICA | x | |||||||
Q9ESB3 | HRG | X | x | x | |||||
P13020 | GSN | x | Oligodendrocytes | ||||||
Q9JN5 | CPN1 | Reticulocytes | |||||||
Group 5 (13) | |||||||||
Q9Z126 | PF4 | x | x | ||||||
Q8bh35 | C8B | x | |||||||
Q61703 | ITIH2 | x | x | x | x | x | Endothelial Cells | ||
Q61702 | ITIH1 | x | x | x | x | ||||
P70389 | IGFALS | x | x | x | x | ||||
Q8K182 | C8A | x | x | ||||||
P26262 | KLKB1 | x | x | x | |||||
Q01279 | EGFR | x | x | x | x | Saliva | |||
Q08761 | PROS1 | x | x | x | |||||
P06683 | C9 | x | x | x | x | Endothelial Cells | |||
Q9Z2U1 | PSMA5 | x | x | x | x | x | Endothelial Cells | ||
Q8VCG4 | C8G | x | x | x | |||||
Q92111 | TF | x | x | x | x | x |
In summary, our study demonstrated that urine exosome proteomic analysis could provide more useful information than the total urine proteome analysis in identifying biomarkers in a time-dependent manner. Our findings also demonstrated that serum and urine samples showed very different radiation signatures indicative of non-overlapping physiological response. Urinary exosome signatures showed dysfunctions of liver, and genitourinary track; whereas, serum exosome signatures showed vascular injuries and acute inflammation. These results showed that non-invasive exosome proteomics can be used in detection of radiation signature and understanding differential tissue responses to determine acute and persistent damage caused by the radiation exposure. Interestingly, many proteins that were identified as radiation biomarkers in the 10.4 Gy experiment, showed no or low changes at lower radiation doses (Fig. 6C). This is anticipated since lower radiation doses are not expected to elicit severe radiation-induced injury observed at high radiation doses. For example, we found increased VDAC secretion in urine exosome from mice exposed to > 6 Gy radiation as early as 24 hours after WBI and its secretion in urine exosome persisted 72 hours after WBI. Hence, VDAC could be a useful biomarker for early detection of medium or high dose radiation exposure. On the other hand, we also found that HEXB could be a useful biomarker for low dose radiation since the secretion of HEXB in urine exosome increased significantly in mice exposed to doses as low as 3 Gy, which is a non-lethal dose in mice. Further studies are needed to confirm which of these proteins show radiation dose sensitivity especially in the dose ranges that are relevant for assessing acute toxicity and triage requirements.
Overall, MS-based targeted analysis we presented in this study showed the promising utility of mass spectrometry-based approach for quantitative validation of detected biomarker candidates as an alternative strategy or complementary to antibody-based validation and new studies will be designed to perform biomarker discovery at escalating radiation doses (radiation biodosimetry) in a time-dependent manner to facilitate patient diagnosis and triage at the time of large-scale radiation exposure.
Supplementary Material
1
2
3
LIST of ABBREVIATIONS
IR | ionizing radiation |
MS | Mass spectrometry |
LC-MS | Liquid chromatography Mass spectrometry |
DDA | data dependent acquisition |
DIA | data independent acquisition |
SDS-PAGE | Sodium dodecyl sulfate polyacrylamide gel electrophoresis |
PCA | Principle component analysis |
FDR | False discovery rate |
SI | Supplementary information |
VDAC1 | Voltage dependent anionic channel 1 |
PLD3 | Phospholipase D3 |
UROM | Uromodulin |
PLAU | Urokinase-type plasminogen activator |
PRDX6 | Peroxiredoxin 6 |
A2M | Alpha 2macroglobulin |
ALB | Albumin |
GLB | Galactosidase beta 1 |
HEXB | Hexosaminidase B |
AGT | Angiotensinogen |
GALNS | Galactosamine (N-acetyl)-6-sulfatase |
APP | Acute phase proteins |
IL6 | Interleukin 6 |
CRP | C-reactive protein |
SAA1 and 2 | Serum amyloid proteins 1 and 2 |
SPA3N | Serpin protease inhibitor |
APOA2 | Apolipoprotein A-II |
KLKB1 | Kallikrein B, plasma |
PROS1 | Vitamin K-dependent protein S |
EGFR | Epidermal growth factor receptor |
HRG | Histidine-rich glycoprotein |
IGFALS | Insulin-like growth factor-binding protein complex acid labile subunit |
TF | Tissue factor |
C8A, C8B, C8G, C9 | Complement pathway components |
SRM | Selected reaction monitoring |
Footnotes
Publisher's Disclaimer: This is a PDF file of an unedited manuscript that has been accepted for publication. As a service to our customers we are providing this early version of the manuscript. The manuscript will undergo copyediting, typesetting, and review of the resulting proof before it is published in its final citable form. Please note that during the production process errors may be discovered which could affect the content, and all legal disclaimers that apply to the journal pertain.
References
Full text links
Read article at publisher's site: https://doi.org/10.1016/j.ijrobp.2016.06.008
Read article for free, from open access legal sources, via Unpaywall:
https://europepmc.org/articles/pmc5042854?pdf=render
Citations & impact
Impact metrics
Article citations
Effect of radiotherapy on the DNA cargo and cellular uptake mechanisms of extracellular vesicles.
Strahlenther Onkol, 199(12):1191-1213, 22 Jun 2023
Cited by: 5 articles | PMID: 37347291
Review
Circulating Exosome Cargoes Contain Functionally Diverse Cancer Biomarkers: From Biogenesis and Function to Purification and Potential Translational Utility.
Cancers (Basel), 14(14):3350, 10 Jul 2022
Cited by: 12 articles | PMID: 35884411 | PMCID: PMC9318395
Review Free full text in Europe PMC
In-plane Extended Nano-coulter Counter (XnCC) for the Label-free Electrical Detection of Biological Particles.
Electroanalysis, 34(12):1961-1975, 14 Jun 2022
Cited by: 1 article | PMID: 37539083 | PMCID: PMC10399599
Therapeutic implications of exosomes in the treatment of radiation injury.
Burns Trauma, 10:tkab043, 21 Jan 2022
Cited by: 9 articles | PMID: 35071650 | PMCID: PMC8778593
Review Free full text in Europe PMC
Exosome-mediated cellular crosstalk within the tumor microenvironment upon irradiation.
Cancer Biol Med, 18(1):21-33, 01 Feb 2021
Cited by: 19 articles | PMID: 33628582 | PMCID: PMC7877182
Review Free full text in Europe PMC
Go to all (17) article citations
Data
Data behind the article
This data has been text mined from the article, or deposited into data resources.
BioStudies: supplemental material and supporting data
Genes & Proteins (Showing 43 of 43)
- (2 citations) UniProt - Q8bh35
- (2 citations) UniProt - Q91WP6
- (2 citations) UniProt - P26262
- (2 citations) UniProt - Q9DBD0
- (2 citations) UniProt - P70389
- (2 citations) UniProt - Q08761
- (2 citations) UniProt - Q92111
- (2 citations) UniProt - Q9Z2U1
- (2 citations) UniProt - P06683
- (2 citations) UniProt - P41317
- (2 citations) UniProt - Q9ESB3
- (2 citations) UniProt - P09813
- (2 citations) UniProt - Q61702
- (2 citations) UniProt - Q9Z126
- (2 citations) UniProt - Q61703
- (2 citations) UniProt - Q9JHE3
- (2 citations) UniProt - P12246
- (2 citations) UniProt - Q01279
- (2 citations) UniProt - P13020
- (2 citations) UniProt - O88947
- (2 citations) UniProt - Q8VCG4
- (2 citations) UniProt - Q8K182
- (2 citations) UniProt - P05366
- (2 citations) UniProt - P05367
- (1 citation) UniProt - Q8BFR4
- (1 citation) UniProt - P07724
- (1 citation) UniProt - O08709
- (1 citation) UniProt - P20060
- (1 citation) UniProt - Q91X17
- (1 citation) UniProt - P70269
- (1 citation) UniProt - P13366
- (1 citation) UniProt - P11591
- (1 citation) UniProt - Q571E4
- (1 citation) UniProt - Q61838
- (1 citation) UniProt - P11859
- (1 citation) UniProt - P23780
- (1 citation) UniProt - P06869
- (1 citation) UniProt - P03953
- (1 citation) UniProt - Q8BZH1
- (1 citation) UniProt - Q60932
- (1 citation) UniProt - O08976
- (1 citation) UniProt - P06151
- (1 citation) UniProt - P45700
Show less
Similar Articles
To arrive at the top five similar articles we use a word-weighted algorithm to compare words from the Title and Abstract of each citation.
Liquid Chromatography-Mass Spectrometry-Based Metabolomics of Nonhuman Primates after 4 Gy Total Body Radiation Exposure: Global Effects and Targeted Panels.
J Proteome Res, 18(5):2260-2269, 18 Mar 2019
Cited by: 19 articles | PMID: 30843397 | PMCID: PMC6559376
Urine Interleukin-18 (IL-18) as a Biomarker of Total-Body Irradiation: A Preliminary Study in Nonhuman Primates.
Radiat Res, 188(3):325-334, 26 Jun 2017
Cited by: 7 articles | PMID: 28650775
Serum microRNAs as Early Indicators for Estimation of Exposure Degree in Response to Ionizing Irradiation.
Radiat Res, 188(3):342-354, 20 Jun 2017
Cited by: 12 articles | PMID: 28632432
Urinary extracellular microvesicles: isolation methods and prospects for urinary proteome.
Proteomics, 14(16):1922-1932, 28 Jul 2014
Cited by: 42 articles | PMID: 24962155
Review
Funding
Funders who supported this work.
NIAID NIH HHS (1)
Grant ID: U19 AI091175
NIGMS NIH HHS (1)
Grant ID: P41 GM103484
National Institutes of Health (1)
Grant ID: U19 AI091175