Abstract
Free full text

Oxidative Stress and the Central Nervous System
Abstract
Biochemical integrity of the brain is vital for normal functioning of the central nervous system (CNS). One of the factors contributing to cerebral biochemical impairment is a chemical process called oxidative stress. Oxidative stress occurs upon excessive free radical production resulting from an insufficiency of the counteracting antioxidant response system. The brain, with its high oxygen consumption and lipid-rich content, is highly susceptible to oxidative stress. Therefore, oxidative stress–induced damage to the brain has a strong potential to negatively impact normal CNS functions. Although oxidative stress has historically been considered to be involved mainly in neurodegenerative disorders such as Alzheimer disease, Huntington disease, and Parkinson disease, its involvement in neuropsychiatric disorders, including anxiety disorders and depression, is beginning to be recognized. This review is a discussion of the relevance of cerebral oxidative stress to impairment of emotional and mental well-being.
Introduction
Oxidative phosphorylation occurring in the mitochondria is a major source of ATP. As a by-product, this process produces free radicals or reactive oxygen species (ROS), reactive nitrogen species (RNS), and carbon- and sulfur-centered radicals (Pero et al., 1990). In moderate or low amounts ROS are considered essential for neuronal development and function, whereas excessive levels are hazardous. ROS-generated nitrous oxide and carbon monoxide promote important physiologic functions, such as long-term potentiation (LTP) via glutamate-dependent mechanisms (O’Dell et al., 1991; Stevens and Wang, 1993; Verma et al., 1993; Zhuo et al., 1993; Knapp and Klann, 2002). Under normal conditions, deleterious effects of ROS production during aerobic metabolism are neutralized by the antioxidant system and in this manner the brain effectively regulates its oxygen consumption and redox generation capacity. When ROS production exceeds scavenging capacity of antioxidant response system, extensive protein oxidation and lipid peroxidation occurs, causing oxidative damage, cellular degeneration, and even functional decline. For example, high ROS concentrations reportedly diminish LTP and synaptic signaling and brain plasticity mechanisms (O’Dell et al., 1991; Stevens and Wang, 1993; Verma et al., 1993; Zhuo et al., 1993; Knapp and Klann, 2002). This is regarded as a state of oxidative stress and becomes particularly hazardous for normal functioning of the brain.
Oxidative stress is often described as a self-propagating phenomenon on the basis of observations that when oxidative stress–induced excessive ROS release triggers cellular damage, damaged macromolecules themselves may behave as and/or become ROS. Consequently, the brain, with its rich lipid content, high energy demand, and weak antioxidant capacity becomes an easy target of excessive oxidative insult (Hulbert et al., 2007). Phospholipids in the brain are particularly vulnerable entities for ROS-mediated peroxidation, but proteins and DNA also are targeted by ROS, which becomes particularly problematic during aging, as aged brains have been reported to exhibit high levels of oxidative stress–induced mutations in the mitochondrial DNA (Gross et al., 1969; Chomyn and Attardi, 2003; Kraytsberg et al., 2003; Trifunovic et al., 2004). Therefore, ROS accumulation is a cellular threat that, if it exceeds or bypasses counteracting mechanisms, can cause significant neuronal damage.
Two kinds of protective mechanisms operate in the brain to tackle the threat posed by ROS, the antioxidant enzyme system and the low-molecular-weight antioxidants (Kohen et al., 1999, 2000). The antioxidant enzyme system includes superoxide dismutase (SOD), glyoxalase, glutathione reductase, glutathione peroxidase, and catalase (CAT) (Griendling et al., 2000). SOD enzymes, including Cu-Zn SOD and Mn-SOD, facilitate spontaneous dismutation of superoxide radicals to generate H2O2, which is further removed by CAT and glutathione peroxidase enzymes (Saso and Firuzi, 2014). The low-molecular-weight antioxidants include glutathione, uric acid, ascorbic acid, and melatonin, which offer neutralizing functions by causing chelation of transition metals (Chance et al., 1979). Glutathione, which occurs in reduced (GSH) and also in oxidized form (glutathione disulfide) is the most important nonenzymatic endogenous antioxidant and can be regenerated by glutathione reductase with the consumption of NADPH (Gul et al., 2000). In this manner optimum levels of reduced GSH are maintained (Kohen and Nyska, 2002; Halliwell, 2006). The endogenous ratio of GSH to glutathione disulfide is considered an indicator of redox homeostasis within a cell. Higher levels of GSH also serve as a cofactor for other enzymes including glyoxalase and peroxidase (Kohen and Nyska, 2002).
In response to oxidative and nitrosative stress, cells increase their antioxidant defenses through activation of nuclear factor erythroid 2–related factor (Nrf2), an important transcription factor (Maes et al., 2011). Nrf2 is a key component of this control system and recognizes the antioxidant response element (ARE) found in the promoter regions of many genes that encode antioxidants and detoxification enzymes such as heme oxygenase 1 (HO-1), NAD(P)H dehydrogenase quinone 1, SOD1, glutathione peroxidase 1 (GPx1), and CAT (Itoh et al., 1997). Thus, Nrf2 pathway activation occurs to combat the accumulation of ROS and RNS species. Owing to its protective properties, Nrf2 has been proposed as a pharmacological target in pathologies with neuroinflammatory and oxidative features, including neurodegenerative and neuropsychiatric diseases. When activated, Nrf2 increases the expression of several endogenous antioxidants. And, upon persistent inflammation and increased ROS levels, as observed during several psychiatric episodes, tissue antioxidant defense mechanisms are saturated to the point they become ineffective (Anderson and Maes, 2014). Cytosolic enzymes such as glyoxalase I by detoxifying methylglyoxal offer protection from oxidative damage (Distler and Palmer, 2012). methylglyoxal generates highly oxidative advanced glycation end products and can further induce oxidative stress and cause cell death (Uribarri et al., 2010).
It is clear that ROS play a crucial pathophysiological role (Campese et al., 2004) and that ROS accumulation increases the susceptibility of brain tissue to damage. Mechanisms by which ROS cause cerebral tissue damage are not well understood but ROS are reported to trigger a variety of molecular cascades that increase blood-brain barrier permeability and alter brain morphology, thus causing neuroinflammation, and neuronal death (Gu et al., 2011). Involvement of hypothalamic-pituitary-adrenal axis–mediated glucocorticoid receptor signaling, glutamate toxicity, and N-methyl-d-aspartate receptor signaling systems also has been suggested (Makino et al., 1996; Okamoto et al., 1999; Tanaka et al., 1999; Albrecht et al., 2010; Nguyen et al., 2011). Thus, evidence of increased brain oxidative damage in the development of central nervous system pathologies has been reported for neurodegenerative diseases, including Alzheimer disease, Parkinson disease, and amyotrophic lateral sclerosis, cerebrovascular disorders, demyelinating diseases, and psychiatric disorders (Sorce and Krause, 2009).
Oxidative Stress and Neurodegenerative Disorders
Neurodegenerative disorders commonly associated with muscular, dementic, and cognitive deficits exhibit brain atrophy, neurofibrillary tangles, plaques, and aggregates as pathologic hallmarks of the disease (Kipps et al., 2005; Obeso et al., 2008; Gandhi and Abramov, 2012). Alzheimer disease, Parkinson disease (PD), and Huntington disease are commonly occurring neurodegenerative disorders that involve neurotoxic aggregation of specific proteins in the brain. Accumulation of misfolded tau and amyloid β proteins occurs in Alzheimer disease, and α-synuclein and mutant Huntington protein (mHtt) accumulate in PD and Huntington disease, respectively. Cause and effect relationship between oxidative stress and these protein aggregates has been theorized. Some studies have reported age-associated increase in oxidative stress–led ROS as a contributor to formation of neuronal plaque, α-synuclein, and mHtt (Li et al., 2013), and other studies have suggested a role for amyloid β protein formation in ROS production (Behl et al., 1997; Abramov and Duchen, 2005; Shelat et al., 2008). Likewise with regard to PD pathology, it is reported that oxidative stress promotes α-synuclein aggregation in dopaminergic neurons, and that α-synuclein further generates intracellular ROS (Xiang et al., 2013). Furthermore, neuronal cell culture studies have implicated free radicals in misfolding and accumulation of mHtt-induced neurotoxicity in PC12 cells. Whereas accumulation of mHtt led to decrease in antioxidant protein peroxiredoxin Prx1, the overexpressed wild-type Prx1 significantly reduced mHtt-induced toxicity (Pitts et al., 2012). Amyloid β-mediated ROS production was reported to induce lipid peroxidation, causing impaired membrane permeability and activating excitotoxicity mechanisms because of increased calcium (Ca2+) influx. This is believed to significantly alter neurotransmission and cognitive functions. In fact, several studies have implicated ROS in amyloid β-induced impairment in LTP, a cellular correlate of learning and memory (Dumont et al., 2009; Ma et al., 2011; Ma and Klann, 2012; Parajuli et al., 2013), also a consequence of aberrant neuronal transmission.
Oxidative Stress and Neuropsychiatric Disorders
Neuropsychiatric disorders are complex and heterogeneous disorders that not only negatively impact quality of life but also significantly affect behavior and cognitive functions (Post, 1992; Kessler, 1997). Several pathophysiological mechanisms have been implicated in these orders, including genetic predisposition, monoamine deficiency, circadian disruptions, hypercortisolemia, and inflammation (Belmaker and Agam, 2008). The involvement of oxidative stress mechanisms have also been suggested in some psychiatric illnesses, including depression, anxiety disorders, schizophrenia, and autism spectrum disorders (Valko et al., 2007; Ng et al., 2008; Bouayed et al., 2009). Increased levels of ROS and RNS (Suzuki and Colasanti, 2001; Dhir and Kulkarni, 2011; Maes et al., 2011) and altered levels of antioxidant glutathione (GSH) were reported in postmortem brain samples of depressed individuals (Gawryluk et al., 2011). Actually, oxidative stress mechanisms have been suggested as targets for novel antidepressants (Lee et al., 2013). This seems reasonable considering reported occurrence of inflammation, oxidative and nitrosative stress, as well as declining levels of plasma concentrations and activity of several key antioxidants in samples from depressed subjects (Maes et al., 2011).
An association between depression and polymorphisms in SOD and CAT genes is also known (Maes et al., 2011). The hypothesis is that the antidepressants exert their therapeutic effect by suppressing proinflammatory cytokines and ROS/RNS production or by enhancing antioxidant defense (Behr et al., 2012). There seems to be strong data to support that depression is accompanied with oxidative stress and that, perhaps, augmentation of antioxidant defenses is one of the mechanisms underlying the neuroprotective effects of antidepressants (Wu et al., 2013). Oxidative stress mechanisms also have been tied to schizophrenia and bipolar disorder. Increased levels of plasma SOD activities were reported in chronic schizophrenic patients who were put on antipsychotic medication, and SOD activities were negatively correlated with positive symptoms of schizophrenics (Ranjekar et al., 2003). Levels of other antioxidants, including glutathione peroxidase (GSH-Px), also have been implicated (Abdalla et al., 1986; Stoklasová et al., 1986; Buckman et al., 1987; Altuntas et al., 2000). It has been suggested that low GSH-Px is a contributing factor to structural brain abnormalities (Buckman et al., 1990; Yao and Reddy, 2011). Several studies have reported that patients with bipolar disorder have significant alterations in antioxidant enzymes, lipid peroxidation, and nitric oxide levels (Andreazza et al., 2008), suggesting the role of free radicals and antioxidants in the pathophysiology of bipolar disorder (Berk et al., 2011; Magalhães et al., 2011; Sarris et al., 2011). Accumulating evidence implicates free radical–mediated pathology, altered antioxidant capacity, neurotoxicity, and inflammation in neuropsychiatric and neurodegenerative disorders.
Oxidative Stress and the Brain
The precise chain of events occurring within the central nervous system that potentially causes or leads to oxidative stress–induced behavioral and cognitive decline is an interesting topic and can be examined at multiple levels. Biochemically, it is evident that different neurons have different levels of vulnerability to oxidative stress. For example, hippocampus, amygdala, and cerebellar granule cells have been reported as the most susceptible to oxidative stress in some studies (Wang and Michaelis, 2010), and consequently are purported to be the first to undergo functional decline. Our own preclinical work has suggested that behavioral and cognitive deficits are attributed to three brain regions: hippocampus, amygdala, and prefrontal cortex (PFC) (Masood et al., 2008; Salim et al., 2010a,b, 2011a,b; Patki et al., 2013a; 2013b; Solanki et al., 2015). Hippocampus seems to be at the hot seat, and it appears that this brain region undergoes major biochemical changes that ultimately determine neuronal connections and function. Within the hippocampus, it is well known that the dentate gyrus–cornu ammonis (CA)3 system exhibits structural plasticity with regenerative/remodeling capacity (Popov and Bocharova, 1992; Sousa et al., 2000; McEwen, 2008). Furthermore, several studies have suggested that pyramidal cells of CA3 and granule cells of the dentate gyrus (DG) are oxidative stress–prone areas, whereas others have suggested that pyramidal cells of CA1 are more susceptible to oxidative damage (Bearden et al., 2009; Cruz-Sánchez et al., 2010; Chang et al., 2012; Huang et al., 2012, 2013; Uysal et al., 2012). Regardless, region-specific elevation of oxidative stress within cornu ammonis areas CA1 and CA3, and DG is important and can have significant functional consequences. This is particularly significant as the DG has a preferential role in learning and memory function, and ventral hippocampus is implicated in anxiety and depression.
Furthermore, amygdala and PFC might undergo dendritic alterations, as evidenced in situations of chronic stress. Dendritic shrinking in medial PFC and dendritic growth in amygdalar neurons in response to stress also has been reported (Wellman, 2001; Vyas et al., 2002; Kreibich and Blendy 2004; Brown et al., 2005; Radley et al., 2006). Stressful stimuli are known to alter prefrontal dendritic architecture and neuronal connectivity within the PFC (Liston et al., 2009; Luethi et al., 2009). Interestingly, higher vulnerability of the hippocampus and amygdala to oxidative stress and breakdown of antioxidant defense system is evident. Therefore, it seems highly plausible that oxidative stress in the brain compromises biochemical integrity of the hippocampus and the amygdala. It is well known that the hippocampal DG-CA3 system regulates structural plasticity, regenerative/remodeling capacity, as well as neurogenesis factors like brain-derived neurotrophic factor (Wang and Michaelis, 2010). It has also been suggested that the pyramidal cells of CA1 and CA3 and granule cells of DG are highly susceptible to oxidative damage. Thus, oxidative damage of DG-CA function may diminish cell proliferation, impair remodeling capacity, alter structural plasticity, and disrupt neurogenesis, collectively disturbing normal synaptic neurotransmission. And, oxidative stress–initiated neuroendocrine alterations within the amygdala, including amygdalar hyperactivity and dendritic shrinking (Wellman, 2001; Vyas et al., 2002; Kreibich and Blendy 2004; Brown et al., 2005; Radley et al., 2006; Wood et al., 2010), can further potentiate synaptic disturbances by disrupting the hippocampus-amygdala projections. Furthermore, free radicals are known to oxidize the extracellular sites of glutamatergic N-methyl-d-aspartate receptors, leading to attenuation of LTP and synaptic neurotransmission (Haxaire et al., 2012; Lee et al., 2012; Rai et al., 2013). Collectively, these events offer an attractive explanation for oxidative stress–induced behavioral and cognitive impairment.
Perhaps, psychologic stress disrupts oxidant-antioxidant balance within the brain, causing impairment of antioxidant enzyme function. This leads to glutathione depletion and increases oxidative stress. Simultaneously occurring glutamate toxicity, calcium imbalance, and mitochondrial impairment collectively intensify oxidative stress, causing biochemical distress in the brain. This disrupts neurocircuitry and weakens hippocampal, amygdalar, and cortical connections, ultimately causing behavioral and cognitive deficits (Fig. 1). It seems reasonable to suggest that, perhaps, tight regulation of oxidative stress, either by enhancing the activity of enzymes of antioxidant defense or by directly quenching pro-oxidants, offers the potential to limit psychiatric symptoms. Thus, data discussed in this review provides a basis for a biologically plausible oxidative stress hypothesis that would explain how oxidative damage might cause psychiatric symptoms.
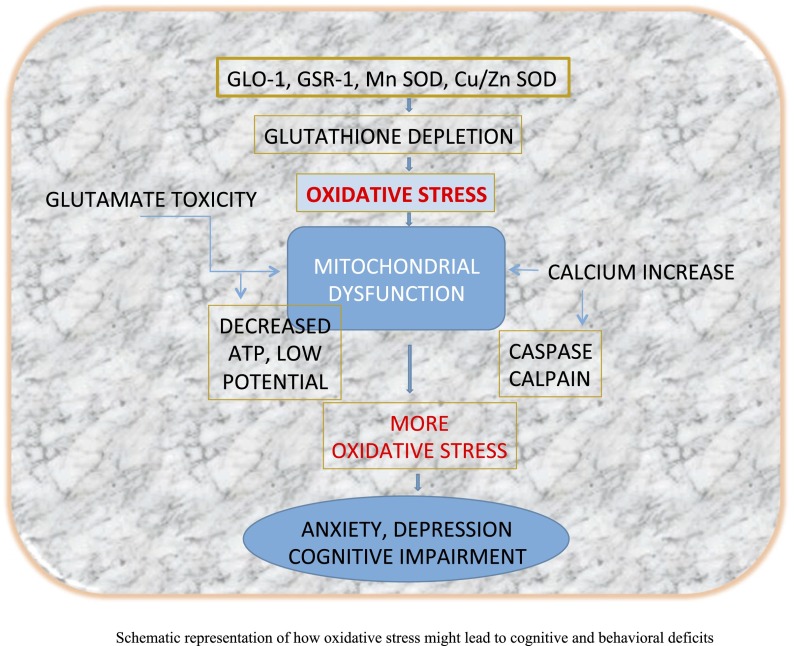
Schematic representation of how oxidative stress might lead to cognitive and behavioral deficits. Persistent psychologic stress disrupts oxidant-antioxidant balance within the brain, causing reduction in antioxidant enzyme function of glyoxalase (GLO)-1, glutathione reductase (GSR)-1, manganese superoxide dismutase (Mn SOD), and Cu/Zn SOD. This leads to glutathione depletion, causing oxidative stress. Simultaneously occurring glutamate toxicity, calcium imbalance, and mitochondrial impairment collectively intensify oxidative stress, causing biochemical distress in the brain. This disrupts neurocircuitry, weakening hippocampal, amygdalar, and cortical connections and ultimately causing behavioral and cognitive deficits.
Acknowledgments
The author’s former and present graduate students, Naimesh Solanki, Ankita Salvi, Hesong Lui, and Fatin Atrooz, are gratefully acknowledged for their hard work in this area of research. Undergraduate students Nada Sarraj, Farida Allam, Amber Ansari, Faizan Jafri, Eisha Khan, Phoebe Dantoin, and Safiyya Zaidi were very helpful in conducting animal behavior work.
Abbreviations
CA | cornu ammonis |
CAT | catalase |
DG | dentate gyrus |
GSH | glutathione |
LTP | long-term potentiation |
mHtt | mutant Huntington protein |
Nrf2 | nuclear factor erythroid 2–related factor |
PD | Parkinson disease |
PFC | prefrontal cortex |
RNS | reactive nitrogen species |
ROS | reactive oxygen species |
SOD | superoxide dismutase |
Footnotes
Funding for this research was provided by a grant from the National Institutes of Health (2R15 MH093918-02) awarded to S.S.
References
- Abdalla DS, Monteiro HP, Oliveira JA, Bechara EJ. (1986) Activities of superoxide dismutase and glutathione peroxidase in schizophrenic and manic-depressive patients. Clin Chem 32:805–807. [Abstract] [Google Scholar]
- Abramov AY, Duchen MR. (2005) The role of an astrocytic NADPH oxidase in the neurotoxicity of amyloid beta peptides. Philos Trans R Soc Lond B Biol Sci 360:2309–2314. [Europe PMC free article] [Abstract] [Google Scholar]
- Albrecht P, Lewerenz J, Dittmer S, Noack R, Maher P, Methner A. (2010) Mechanisms of oxidative glutamate toxicity: the glutamate/cystine antiporter system xc- as a neuroprotective drug target. CNS Neurol Disord Drug Targets 9:373–382. [Abstract] [Google Scholar]
- Altuntas I, Aksoy H, Coskun I, Cayköylü A, Akçay F. (2000) Erythrocyte superoxide dismutase and glutathione peroxidase activities, and malondialdehyde and reduced glutathione levels in schizophrenic patients. Clin Chem Lab Med 38:1277–1281. [Abstract] [Google Scholar]
- Anderson G, Maes M. (2014) Oxidative/nitrosative stress and immuno-inflammatory pathways in depression: treatment implications. Curr Pharm Des 20:3812–3847. [Abstract] [Google Scholar]
- Andreazza AC, Kauer-Sant’anna M, Frey BN, Bond DJ, Kapczinski F, Young LT, Yatham LN. (2008) Oxidative stress markers in bipolar disorder: a meta-analysis. J Affect Disord 111:135–144. [Abstract] [Google Scholar]
- Bearden CE, Thompson PM, Avedissian C, Klunder AD, Nicoletti M, Dierschke N, Brambilla P, Soares JC. (2009) Altered hippocampal morphology in unmedicated patients with major depressive illness. ASN Neuro 1:p. ii e00020, DOI: 10.1042/AN20090026. [Europe PMC free article] [Abstract] [Google Scholar]
- Behl C, Trapp T, Skutella T, Holsboer F. (1997) Protection against oxidative stress-induced neuronal cell death--a novel role for RU486. Eur J Neurosci 9:912–920. [Abstract] [Google Scholar]
- Behr GA, Moreira JC, Frey BN. (2012) Preclinical and clinical evidence of antioxidant effects of antidepressant agents: implications for the pathophysiology of major depressive disorder. Oxid Med Cell Longev 2012:609421. [Europe PMC free article] [Abstract] [Google Scholar]
- Belmaker RH, Agam G. (2008) Major depressive disorder. N Engl J Med 358:55–68. [Abstract] [Google Scholar]
- Berk M, Dean O, Cotton SM, Gama CS, Kapczinski F, Fernandes BS, Kohlmann K, Jeavons S, Hewitt K, Allwang C, et al. (2011) The efficacy of N-acetylcysteine as an adjunctive treatment in bipolar depression: an open label trial. J Affect Disord 135:389–394. [Abstract] [Google Scholar]
- Bouayed J, Rammal H, Soulimani R. (2009) Oxidative stress and anxiety: relationship and cellular pathways. Oxid Med Cell Longev 2:63–67. [Europe PMC free article] [Abstract] [Google Scholar]
- Brown SM, Henning S, Wellman CL. (2005) Mild, short-term stress alters dendritic morphology in rat medial prefrontal cortex. Cereb Cortex 15:1714–1722. [Abstract] [Google Scholar]
- Buckman TD, Kling AS, Eiduson S, Sutphin MS, Steinberg A. (1987) Glutathione peroxidase and CT scan abnormalities in schizophrenia. Biol Psychiatry 22:1349–1356. [Abstract] [Google Scholar]
- Buckman TD, Kling A, Sutphin MS, Steinberg A, Eiduson S. (1990) Platelet glutathione peroxidase and monoamine oxidase activity in schizophrenics with CT scan abnormalities: relation to psychosocial variables. Psychiatry Res 31:1–14. [Abstract] [Google Scholar]
- Campese VM, Ye S, Zhong H, Yanamadala V, Ye Z, Chiu J. (2004) Reactive oxygen species stimulate central and peripheral sympathetic nervous system activity. Am J Physiol Heart Circ Physiol 287:H695–H703. [Abstract] [Google Scholar]
- Chance B, Schoener B, Oshino R, Itshak F, Nakase Y. (1979) Oxidation-reduction ratio studies of mitochondria in freeze-trapped samples. NADH and flavoprotein fluorescence signals. J Biol Chem 254:4764–4771. [Abstract] [Google Scholar]
- Chang BJ, Jang BJ, Son TG, Cho IH, Quan FS, Choe NH, Nahm SS, Lee JH. (2012) Ascorbic acid ameliorates oxidative damage induced by maternal low-level lead exposure in the hippocampus of rat pups during gestation and lactation. Food Chem Toxicol 50:104–108. [Abstract] [Google Scholar]
- Chomyn A, Attardi G. (2003) MtDNA mutations in aging and apoptosis. Biochem Biophys Res Commun 304:519–529. [Abstract] [Google Scholar]
- Cruz-Sánchez FF, Gironès X, Ortega A, Alameda F, Lafuente JV. (2010) Oxidative stress in Alzheimer's disease hippocampus: a topographical study. J Neurol Sci 299:163–167. [Abstract] [Google Scholar]
- Dhir A, Kulkarni SK. (2011) Nitric oxide and major depression. Nitric Oxide 24:125–131. [Abstract] [Google Scholar]
- Distler MG, Palmer AA. (2012) Role of glyoxalase 1 (Glo1) and methylglyoxal (MG) in behavior: recent advances and mechanistic insights. Front Genet 3:250. [Europe PMC free article] [Abstract] [Google Scholar]
- Dumont M, Wille E, Stack C, Calingasan NY, Beal MF, Lin MT. (2009) Reduction of oxidative stress, amyloid deposition, and memory deficit by manganese superoxide dismutase overexpression in a transgenic mouse model of Alzheimer’s disease. FASEB J 23:2459–2466. [Europe PMC free article] [Abstract] [Google Scholar]
- Gandhi S, Abramov AY. (2012) Mechanism of oxidative stress in neurodegeneration. Oxid Med Cell Longev 2012:428010. [Europe PMC free article] [Abstract] [Google Scholar]
- Gawryluk JW, Wang JF, Andreazza AC, Shao L, Young LT. (2011) Decreased levels of glutathione, the major brain antioxidant, in post-mortem prefrontal cortex from patients with psychiatric disorders. Int J Neuropsychopharmacol 14:123–130. [Abstract] [Google Scholar]
- Griendling KK, Sorescu D, Lassègue B, Ushio-Fukai M. (2000) Modulation of protein kinase activity and gene expression by reactive oxygen species and their role in vascular physiology and pathophysiology. Arterioscler Thromb Vasc Biol 20:2175–2183. [Abstract] [Google Scholar]
- Gross NJ, Getz GS, Rabinowitz M. (1969) Apparent turnover of mitochondrial deoxyribonucleic acid and mitochondrial phospholipids in the tissues of the rat. J Biol Chem 244:1552–1562. [Abstract] [Google Scholar]
- Gu Y, Dee CM, Shen J. (2011) Interaction of free radicals, matrix metalloproteinases and caveolin-1 impacts blood-brain barrier permeability. Front Biosci (Schol Ed) 3:1216–1231 Schol Ed. [Abstract] [Google Scholar]
- Gul M, Kutay FZ, Temocin S, Hanninen O. (2000) Cellular and clinical implications of glutathione. Indian J Exp Biol 38:625–634. [Abstract] [Google Scholar]
- Halliwell B. (2006) Reactive species and antioxidants. Redox biology is a fundamental theme of aerobic life. Plant Physiol 141:312–322. [Abstract] [Google Scholar]
- Haxaire C, Turpin FR, Potier B, Kervern M, Sinet PM, Barbanel G, Mothet JP, Dutar P, Billard JM. (2012) Reversal of age-related oxidative stress prevents hippocampal synaptic plasticity deficits by protecting D-serine-dependent NMDA receptor activation. Aging Cell 11:336–344. [Abstract] [Google Scholar]
- Huang TT, Zou Y, Corniola R. (2012) Oxidative stress and adult neurogenesis—effects of radiation and superoxide dismutase deficiency. Semin Cell Dev Biol 23:738–744. [Europe PMC free article] [Abstract] [Google Scholar]
- Huang Y, Coupland NJ, Lebel RM, Carter R, Seres P, Wilman AH, Malykhin NV. (2013) Structural changes in hippocampal subfields in major depressive disorder: a high-field magnetic resonance imaging study. Biol Psychiatry 74:62–68. [Abstract] [Google Scholar]
- Hulbert AJ, Pamplona R, Buffenstein R, Buttemer WA. (2007) Life and death: metabolic rate, membrane composition, and life span of animals. Physiol Rev 87:1175–1213. [Abstract] [Google Scholar]
- Itoh K, Chiba T, Takahashi S, Ishii T, Igarashi K, Katoh Y, Oyake T, Hayashi N, Satoh K, Hatayama I, Yamamoto M, Nabeshima Y. (1997) An Nrf2/small maf heterodimer mediates the induction of phase II detoxifying enzyme genes through antioxidant response elements. Biochem Biophys Res Commun 236:313–322. [Abstract] [Google Scholar]
- Kessler RC. (1997) The effects of stressful life events on depression. Annu Rev Psychol 48:191–214. [Abstract] [Google Scholar]
- Kipps CM, Duggins AJ, Mahant N, Gomes L, Ashburner J, McCusker EA. (2005) Progression of structural neuropathology in preclinical Huntington’s disease: a tensor based morphometry study. J Neurol Neurosurg Psychiatry 76:650–655. [Europe PMC free article] [Abstract] [Google Scholar]
- Knapp LT, Klann E. (2002) Role of reactive oxygen species in hippocampal long-term potentiation: contributory or inhibitory? J Neurosci Res 70:1–7. [Abstract] [Google Scholar]
- Kohen R, Beit-Yannai E, Berry EM, Tirosh O. (1999) Overall low molecular weight antioxidant activity of biological fluids and tissues by cyclic voltammetry. Methods Enzymol 300:285–296. [Abstract] [Google Scholar]
- Kohen R, Nyska A. (2002) Oxidation of biological systems: oxidative stress phenomena, antioxidants, redox reactions, and methods for their quantification. Toxicol Pathol 30:620–650. [Abstract] [Google Scholar]
- Kohen R, Vellaichamy E, Hrbac J, Gati I, Tirosh O. (2000) Quantification of the overall reactive oxygen species scavenging capacity of biological fluids and tissues. Free Radic Biol Med 28:871–879. [Abstract] [Google Scholar]
- Kraytsberg Y, Nekhaeva E, Bodyak NB, Khrapko K. (2003) Mutation and intracellular clonal expansion of mitochondrial genomes: two synergistic components of the aging process? Mech Ageing Dev 124:49–53. [Abstract] [Google Scholar]
- Kreibich AS, Blendy JA. (2004) cAMP response element-binding protein is required for stress but not cocaine-induced reinstatement. J Neurosci 24:6686–6692. [Abstract] [Google Scholar]
- Lee DZ, Chung JM, Chung K, Kang MG. (2012) Reactive oxygen species (ROS) modulate AMPA receptor phosphorylation and cell-surface localization in concert with pain-related behavior. Pain 153:1905–1915. [Europe PMC free article] [Abstract] [Google Scholar]
- Lee SY, Lee SJ, Han C, Patkar AA, Masand PS, Pae CU. (2013) Oxidative/nitrosative stress and antidepressants: targets for novel antidepressants. Prog Neuropsychopharmacol Biol Psychiatry 46:224–235. [Abstract] [Google Scholar]
- Li J, O W, Li W, Jiang ZG, Ghanbari HA. (2013) Oxidative stress and neurodegenerative disorders. Int J Mol Sci 14:24438–24475. [Europe PMC free article] [Abstract] [Google Scholar]
- Liston C, McEwen BS, Casey BJ. (2009) Psychosocial stress reversibly disrupts prefrontal processing and attentional control. Proc Natl Acad Sci USA 106:912–917. [Abstract] [Google Scholar]
- Luethi M, Meier B, Sandi C. (2009) Stress effects on working memory, explicit memory, and implicit memory for neutral and emotional stimuli in healthy men. Front Behav Neurosci 2:5. [Europe PMC free article] [Abstract] [Google Scholar]
- Ma T, Hoeffer CA, Wong H, Massaad CA, Zhou P, Iadecola C, Murphy MP, Pautler RG, Klann E. (2011) Amyloid β-induced impairments in hippocampal synaptic plasticity are rescued by decreasing mitochondrial superoxide. J Neurosci 31:5589–5595. [Europe PMC free article] [Abstract] [Google Scholar]
- Ma T, Klann E. (2012) Amyloid β: linking synaptic plasticity failure to memory disruption in Alzheimer’s disease. J Neurochem 120 (Suppl 1):140–148. [Europe PMC free article] [Abstract] [Google Scholar]
- Maes M, Galecki P, Chang YS, Berk M. (2011) A review on the oxidative and nitrosative stress (O&NS) pathways in major depression and their possible contribution to the (neuro)degenerative processes in that illness. Prog Neuropsychopharmacol Biol Psychiatry 35:676–692. [Abstract] [Google Scholar]
- Magalhães PV, Dean OM, Bush AI, Copolov DL, Malhi GS, Kohlmann K, Jeavons S, Schapkaitz I, Anderson-Hunt M, Berk M. (2011) N-acetylcysteine for major depressive episodes in bipolar disorder. Rev Bras Psiquiatr 33:374–378. [Abstract] [Google Scholar]
- Makino Y, Tanaka H, Dahlman-Wright K, Makino I. (1996) Modulation of glucocorticoid-inducible gene expression by metal ions. Mol Pharmacol 49:612–620. [Abstract] [Google Scholar]
- Masood A, Nadeem A, Mustafa SJ, O’Donnell JM. (2008) Reversal of oxidative stress-induced anxiety by inhibition of phosphodiesterase-2 in mice. J Pharmacol Exp Ther 326:369–379. [Europe PMC free article] [Abstract] [Google Scholar]
- McEwen BS. (2008) Understanding the potency of stressful early life experiences on brain and body function. Metabolism 57 (Suppl 2):S11–S15. [Europe PMC free article] [Abstract] [Google Scholar]
- Ng F, Berk M, Dean O, Bush AI. (2008) Oxidative stress in psychiatric disorders: evidence base and therapeutic implications. Int J Neuropsychopharmacol 11:851–876. [Abstract] [Google Scholar]
- Nguyen D, Alavi MV, Kim KY, Kang T, Scott RT, Noh YH, Lindsey JD, Wissinger B, Ellisman MH, Weinreb RN, et al. (2011) A new vicious cycle involving glutamate excitotoxicity, oxidative stress and mitochondrial dynamics. Cell Death Dis 2:e240. [Europe PMC free article] [Abstract] [Google Scholar]
- Obeso JA, Rodríguez-Oroz MC, Benitez-Temino B, Blesa FJ, Guridi J, Marin C, Rodriguez M. (2008) Functional organization of the basal ganglia: therapeutic implications for Parkinson’s disease. Mov Disord 23 (Suppl 3):S548–S559. [Abstract] [Google Scholar]
- O’Dell TJ, Hawkins RD, Kandel ER, Arancio O. (1991) Tests of the roles of two diffusible substances in long-term potentiation: evidence for nitric oxide as a possible early retrograde messenger. Proc Natl Acad Sci USA 88:11285–11289. [Europe PMC free article] [Abstract] [Google Scholar]
- Okamoto K, Tanaka H, Ogawa H, Makino Y, Eguchi H, Hayashi S, Yoshikawa N, Poellinger L, Umesono K, Makino I. (1999) Redox-dependent regulation of nuclear import of the glucocorticoid receptor. J Biol Chem 274:10363–10371. [Abstract] [Google Scholar]
- Parajuli B, Sonobe Y, Horiuchi H, Takeuchi H, Mizuno T, Suzumura A. (2013) Oligomeric amyloid β induces IL-1β processing via production of ROS: implication in Alzheimer’s disease. Cell Death Dis 4:e975. [Europe PMC free article] [Abstract] [Google Scholar]
- Patki G, Allam FH, Atrooz F, Dao AT, Solanki N, Chugh G, Asghar M, Jafri F, Bohat R, Alkadhi KA, et al. (2013a) Grape powder intake prevents ovariectomy-induced anxiety-like behavior, memory impairment and high blood pressure in female Wistar rats. PLoS One 8:e74522. [Europe PMC free article] [Abstract] [Google Scholar]
- Patki G, Solanki N, Atrooz F, Allam F, Salim S. (2013b) Depression, anxiety-like behavior and memory impairment are associated with increased oxidative stress and inflammation in a rat model of social stress. Brain Res 1539:73–86. [Europe PMC free article] [Abstract] [Google Scholar]
- Pero RW, Roush GC, Markowitz MM, Miller DG. (1990) Oxidative stress, DNA repair, and cancer susceptibility. Cancer Detect Prev 14:555–561. [Abstract] [Google Scholar]
- Pitts A, Dailey K, Newington JT, Chien A, Arseneault R, Cann T, Thompson LM, Cumming RC. (2012) Dithiol-based compounds maintain expression of antioxidant protein peroxiredoxin 1 that counteracts toxicity of mutant huntingtin. J Biol Chem 287:22717–22729. [Europe PMC free article] [Abstract] [Google Scholar]
- Popov VI, Bocharova LS. (1992) Hibernation-induced structural changes in synaptic contacts between mossy fibres and hippocampal pyramidal neurons. Neuroscience 48:53–62. [Abstract] [Google Scholar]
- Post RM. (1992) Transduction of psychosocial stress into the neurobiology of recurrent affective disorder. Am J Psychiatry 149:999–1010. [Abstract] [Google Scholar]
- Radley JJ, Rocher AB, Miller M, Janssen WG, Liston C, Hof PR, McEwen BS, Morrison JH. (2006) Repeated stress induces dendritic spine loss in the rat medial prefrontal cortex. Cereb Cortex 16:313–320. [Abstract] [Google Scholar]
- Rai S, Kamat PK, Nath C, Shukla R. (2013) A study on neuroinflammation and NMDA receptor function in STZ (ICV) induced memory impaired rats. J Neuroimmunol 254:1–9. [Abstract] [Google Scholar]
- Ranjekar PK, Hinge A, Hegde MV, Ghate M, Kale A, Sitasawad S, Wagh UV, Debsikdar VB, Mahadik SP. (2003) Decreased antioxidant enzymes and membrane essential polyunsaturated fatty acids in schizophrenic and bipolar mood disorder patients. Psychiatry Res 121:109–122. [Abstract] [Google Scholar]
- Salim S, Asghar M, Chugh G, Taneja M, Xia Z, Saha K. (2010a) Oxidative stress: a potential recipe for anxiety, hypertension and insulin resistance. Brain Res 1359:178–185. [Europe PMC free article] [Abstract] [Google Scholar]
- Salim S, Asghar M, Taneja M, Hovatta I, Chugh G, Vollert C, Vu A. (2011a) Potential contribution of oxidative stress and inflammation to anxiety and hypertension. Brain Res 1404:63–71. [Europe PMC free article] [Abstract] [Google Scholar]
- Salim S, Asghar M, Taneja M, Hovatta I, Wu YL, Saha K, Sarraj N, Hite B. (2011b) Novel role of RGS2 in regulation of antioxidant homeostasis in neuronal cells. FEBS Lett 585:1375–1381. [Europe PMC free article] [Abstract] [Google Scholar]
- Salim S, Sarraj N, Taneja M, Saha K, Tejada-Simon MV, Chugh G. (2010b) Moderate treadmill exercise prevents oxidative stress-induced anxiety-like behavior in rats. Behav Brain Res 208:545–552. [Abstract] [Google Scholar]
- Sarris J, Mischoulon D, Schweitzer I. (2011) Adjunctive nutraceuticals with standard pharmacotherapies in bipolar disorder: a systematic review of clinical trials. Bipolar Disord 13:454–465. [Abstract] [Google Scholar]
- Saso L, Firuzi O. (2014) Pharmacological applications of antioxidants: lights and shadows. Curr Drug Targets 15:1177–1199. [Abstract] [Google Scholar]
- Shelat PB, Chalimoniuk M, Wang JH, Strosznajder JB, Lee JC, Sun AY, Simonyi A, Sun GY. (2008) Amyloid beta peptide and NMDA induce ROS from NADPH oxidase and AA release from cytosolic phospholipase A2 in cortical neurons. J Neurochem 106:45–55. [Abstract] [Google Scholar]
- Solanki N, Alkadhi I, Atrooz F, Patki G, Salim S. (2015) Grape powder prevents cognitive, behavioral, and biochemical impairments in a rat model of posttraumatic stress disorder. Nutr Res 35:65–75. [Europe PMC free article] [Abstract] [Google Scholar]
- Sorce S, Krause KH. (2009) NOX enzymes in the central nervous system: from signaling to disease. Antioxid Redox Signal 11:2481–2504. [Abstract] [Google Scholar]
- Sousa N, Lukoyanov NV, Madeira MD, Almeida OF, Paula-Barbosa MM. (2000) Reorganization of the morphology of hippocampal neurites and synapses after stress-induced damage correlates with behavioral improvement. Neuroscience 97:253–266. [Abstract] [Google Scholar]
- Stevens CF, Wang Y. (1993) Reversal of long-term potentiation by inhibitors of haem oxygenase. Nature 364:147–149. [Abstract] [Google Scholar]
- Stoklasová A, Zapletálek M, Kudrnová K, Randová Z. (1986) [Glutathione peroxidase activity in the blood in chronic schizophrenia]. Sb Ved Pr Lek Fak Karlovy Univerzity Hradci Kralove Suppl 29:103–108. [Abstract] [Google Scholar]
- Suzuki H, Colasanti M. (2001) NO: a molecule with two masks of ‘NO’ theatre. Biofactors 15:123–125. [Abstract] [Google Scholar]
- Tanaka H, Makino Y, Okamoto K, Iida T, Yan K, Yoshikawa N. (1999) Redox regulation of the glucocorticoid receptor. Antioxid Redox Signal 1:403–423. [Abstract] [Google Scholar]
- Trifunovic A, Wredenberg A, Falkenberg M, Spelbrink JN, Rovio AT, Bruder CE, Bohlooly-Y M, Gidlöf S, Oldfors A, Wibom R, et al. (2004) Premature ageing in mice expressing defective mitochondrial DNA polymerase. Nature 429:417–423. [Abstract] [Google Scholar]
- Uribarri J, Woodruff S, Goodman S, Cai W, Chen X, Pyzik R, Yong A, Striker GE, Vlassara H. (2010) Advanced glycation end products in foods and a practical guide to their reduction in the diet. J Am Diet Assoc 110:911–916 e12. [Europe PMC free article] [Abstract] [Google Scholar]
- Uysal N, Tugyan K, Aksu I, Ozbal S, Ozdemir D, Dayi A, Gönenç S, Açikgöz O. (2012) Age-related changes in apoptosis in rat hippocampus induced by oxidative stress. Biotech Histochem 87:98–104. [Abstract] [Google Scholar]
- Valko M, Leibfritz D, Moncol J, Cronin MT, Mazur M, Telser J. (2007) Free radicals and antioxidants in normal physiological functions and human disease. Int J Biochem Cell Biol 39:44–84. [Abstract] [Google Scholar]
- Verma A, Hirsch DJ, Glatt CE, Ronnett GV, Snyder SH. (1993) Carbon monoxide: a putative neural messenger. Science 259:381–384. [Abstract] [Google Scholar]
- Vyas A, Mitra R, Shankaranarayana Rao BS, Chattarji S. (2002) Chronic stress induces contrasting patterns of dendritic remodeling in hippocampal and amygdaloid neurons. J Neurosci 22:6810–6818. [Abstract] [Google Scholar]
- Wang X, Michaelis EK. (2010) Selective neuronal vulnerability to oxidative stress in the brain. Front Aging Neurosci 2:12. [Europe PMC free article] [Abstract] [Google Scholar]
- Wellman CL. (2001) Dendritic reorganization in pyramidal neurons in medial prefrontal cortex after chronic corticosterone administration. J Neurobiol 49:245–253. [Abstract] [Google Scholar]
- Wood SK, Walker HE, Valentino RJ, Bhatnagar S. (2010) Individual differences in reactivity to social stress predict susceptibility and resilience to a depressive phenotype: role of corticotropin-releasing factor. Endocrinology 151:1795–1805. [Europe PMC free article] [Abstract] [Google Scholar]
- Wu JQ, Kosten TR, Zhang XY. (2013) Free radicals, antioxidant defense systems, and schizophrenia. Prog Neuropsychopharmacol Biol Psychiatry 46:200–206. [Abstract] [Google Scholar]
- Xiang W, Schlachetzki JC, Helling S, Bussmann JC, Berlinghof M, Schäffer TE, Marcus K, Winkler J, Klucken J, Becker CM. (2013) Oxidative stress-induced posttranslational modifications of alpha-synuclein: specific modification of alpha-synuclein by 4-hydroxy-2-nonenal increases dopaminergic toxicity. Mol Cell Neurosci 54:71–83. [Abstract] [Google Scholar]
- Yao JK, Reddy R. (2011) Oxidative stress in schizophrenia: pathogenetic and therapeutic implications. Antioxid Redox Signal 15:1999–2002. [Europe PMC free article] [Abstract] [Google Scholar]
- Zhuo M, Small SA, Kandel ER, Hawkins RD. (1993) Nitric oxide and carbon monoxide produce activity-dependent long-term synaptic enhancement in hippocampus. Science 260:1946–1950. [Abstract] [Google Scholar]
Articles from The Journal of Pharmacology and Experimental Therapeutics are provided here courtesy of American Society for Pharmacology and Experimental Therapeutics
Full text links
Read article at publisher's site: https://doi.org/10.1124/jpet.116.237503
Read article for free, from open access legal sources, via Unpaywall:
https://jpet.aspetjournals.org/content/jpet/360/1/201.full.pdf
Citations & impact
Impact metrics
Article citations
Unraveling the role and mechanism of mitochondria in postoperative cognitive dysfunction: a narrative review.
J Neuroinflammation, 21(1):293, 12 Nov 2024
Cited by: 0 articles | PMID: 39533332 | PMCID: PMC11559051
Review Free full text in Europe PMC
Association Between the Gut Microbiota and Alzheimer's Disease: An Update on Signaling Pathways and Translational Therapeutics.
Mol Neurobiol, 26 Oct 2024
Cited by: 0 articles | PMID: 39460901
Review
Mechanisms of ozone-induced neurotoxicity in the development and progression of dementia: a brief review.
Front Aging Neurosci, 16:1494356, 28 Oct 2024
Cited by: 0 articles | PMID: 39529750
Review
The potential mechanism of mitochondrial homeostasis in postoperative neurocognitive disorders: an in-depth review.
Ann Med, 56(1):2411012, 25 Oct 2024
Cited by: 0 articles | PMID: 39450938 | PMCID: PMC11514427
Review Free full text in Europe PMC
Acyclic sesquiterpenes nerolidol and farnesol: mechanistic insights into their neuroprotective potential.
Pharmacol Rep, 22 Oct 2024
Cited by: 0 articles | PMID: 39436564
Review
Go to all (463) article citations
Similar Articles
To arrive at the top five similar articles we use a word-weighted algorithm to compare words from the Title and Abstract of each citation.
Oxidative Stress is a New Avenue for Treatment of Neuropsychiatric Disorders: Hype of Hope?
Curr Mol Med, 24(12):1494-1505, 01 Jan 2024
Cited by: 2 articles | PMID: 37670697
Review
Vitamin E and other endogenous antioxidants in the central nervous system.
Geriatrics, 53 Suppl 1:S25-7, 01 Sep 1998
Cited by: 31 articles | PMID: 9745632
Review
Mitochondria, metabolic disturbances, oxidative stress and the kynurenine system, with focus on neurodegenerative disorders.
J Neurol Sci, 257(1-2):221-239, 25 Apr 2007
Cited by: 235 articles | PMID: 17462670
Review
[Role of oxidative stress in the pathophysiology of neuropsychiatric disorders].
Seishin Shinkeigaku Zasshi, 116(10):842-858, 01 Jan 2014
Cited by: 5 articles | PMID: 25672211