Abstract
Free full text

Genome editing in the mushroom-forming basidiomycete Coprinopsis cinerea, optimized by a high-throughput transformation system
Abstract
Mushroom-forming basidiomycetes produce a wide range of metabolites and have great value not only as food but also as an important global natural resource. Here, we demonstrate CRISPR/Cas9-based genome editing in the model species Coprinopsis cinerea. Using a high-throughput reporter assay with cryopreserved protoplasts, we identified a novel promoter, CcDED1 pro, with seven times stronger activity in this assay than the conventional promoter GPD2. To develop highly efficient genome editing using CRISPR/Cas9 in C. cinerea, we used the CcDED1 pro to express Cas9 and a U6-snRNA promoter from C. cinerea to express gRNA. Finally, CRISPR/Cas9-mediated GFP mutagenesis was performed in a stable GFP expression line. Individual genome-edited lines were isolated, and loss of GFP function was detected in hyphae and fruiting body primordia. This novel method of high-throughput CRISPR/Cas9-based genome editing using cryopreserved protoplasts should be a powerful tool in the study of edible mushrooms.
Introduction
Mushroom-forming basidiomycetes are unique in that they develop a three-dimensional organized fruiting body useful for the production of a wide range of secondary metabolites as well as supplying food globally. Coprinopsis cinerea is a model basidiomycete species commonly used to study fruiting body development1, 2 and has been screened for functional genes regulating fruiting body development3. Since meiosis in C. cinerea progresses synchronously during spore production1, C. cinerea has also been used as a model in molecular and genetic studies of meiosis. Recently, transcriptome studies of fruiting body development have been aided by deep sequencing methods4, 5. Dissection of the genetic pathways involved in these biological processes has been revealed by these approaches, as well as the identification of some novel promoters. To characterize these genes, it is important to establish and validate their functions in vivo. Accordingly, high-throughput transformation and scalable systems for gene perturbation are worth developing in these species to further research into the genetics of the mushroom-forming basidiomycetes.
Genome engineering tools, including high throughput transformation systems, are relatively less well developed in mushroom-forming basidiomycetes than in other model filamentous fungi. In C. cinerea, gene targeting methods for application to genetic studies have been developed using Δku70 or Δlig4 mutants6; however, high-throughput reverse genetics and appropriate reporter assays remain to be established7. Although a PEG-based transformation system using oidia of C. cinerea has been available for many years8, preparation of protoplasts from C. cinerea oidia is relatively laborious. In some plant pathogenic fungi, protoplasts can be cryopreserved9, which makes it possible to perform a number of transformations with one batch of protoplasts10. To our knowledge, there is a report which described cryopreservation of protoplast of basidiomycete11, however, assessments of cryopreservation buffers and preservation periods remain matters of research.
Genome editing methods are now commonly used to modify genomes in vivo 12. Among the more recently emerging technologies are artificially designed nucleases targeting genes of interest that are introduced into cells to induce error-prone double strand breaks (DSBs) in target sequences12. Currently, the most widespread genome editing technology is the clustered regularly interspaced short palindromic repeat (CRISPR)/CRISPR-associated protein-9 nuclease (Cas9) system, which consists of two components: Cas9 nuclease, and a guide RNA (gRNA) that targets the genome sequence of interest13. A number of customizations of the CRISPR/Cas9 system have been introduced for model organisms. The CRISPR/Cas9 system has been developed in various fungi, such as Neurospora crassa 14, Candida albicans 15, Pyricularia oryzae 16, Trichoderma reesei 17, Aspergillus fumigatus 18 and Ustilago maydis 19. However, there are no published reports of the establishment of CRISPR/Cas9 in mushroom-forming basidiomycetes.
The present study demonstrated CRISPR/Cas9-based genome editing and high-throughput transformation methods in the mushroom-forming basidiomycete C. cinerea. CRISPR/Cas9 customization to C. cinerea was achieved via identification of a novel, constitutively active, promoter screened from a high-throughput transformation method using cryopreserved protoplasts. We developed a novel luciferase assay that takes only 1day to measure promoter activities in C. cinerea protoplasts. This high-throughput transformation and the CRISPR/Cas9 system customized for mushroom-forming basidiomycetes could contribute to the acceleration of genetic studies and molecular breeding in these fungi.
Results
Cryopreservation of protoplasts with sorbitol and DMSO buffer
To establish a concise procedure for conventional PEG-based transformation in C. cinerea, we first developed a new method for protoplast preservation. C. cinerea protoplasts were preserved at −80°C after preparation in various buffers (Fig. 1a,b). We modified the MM buffer [0.5
M mannitol and malate buffer (pH 5.5)8] commonly used for protoplast preparation in C. cinerea, by using sorbitol as an osmotic stabilizer instead of mannitol. To inhibit the growth of ice crystals, DMSO, glycerol, and PEG were tested (Fig. 1b). Protoplasts in five types of buffer (Fig. 1b) were preserved at −80
°C for 3 weeks, and were then analyzed by measuring both survival and transformation rates (Fig. 1c,d). Protoplasts preserved with buffer containing 0.5
M sorbitol and 10% DMSO showed survival and transformation rates comparable to those of freshly prepared protoplasts. Notably, transformants could be obtained even using protoplasts preserved in the buffer for one year (Supplementary Fig. 1). We also tested the effect of the rate of cooling on the transformation rate of preserved protoplasts. When the protoplasts were cooled at a rate of −1
°C/min, their transformation competency was higher than when they were cooled immediately to −80
°C (Supplementary Fig. 2). The transformation rates in independent trials showed different values (Fig. 1d, Supplementary Fig. 2), however, the transformation rates were affected by the slight difference of condition of the fungal cells when oidia and protoplasts were prepared (data not shown).

Cryopreservation of protoplasts of C. cinerea. Evaluation of protoplasts stored in different buffers at −80°C for 3 weeks. (a) Schematic image of the protoplast preservation experiments. (b) The buffers used for cryopreservation of protoplasts. (c) Survival rates defined by the number of surviving cells per 103 preserved cells. Survival rate of fresh protoplasts was defined as 100%. (d) Transformation rates defined by the number of hygromycin-B resistant colonies in 108 preserved cells. Error bars in (b) and (c) show S.D. n
=
3; bar plots with the same lower case characters were statistically significant groups as tested by Turkey’s HSD test (P
<
0.05). (e) Schematic image of the transient expression assay using preserved protoplasts which were stored at −80
°C with buffer 3 for three weeks. (f) Fluorescent images of protoplasts 24
hrs after transformation. Fresh and cryopreserved protoplasts were compared. Scale bar: 50
µm.
Using protoplasts cooled slowly in cryopreservation buffer [0.5M sorbitol and 10% DMSO (pH 5.5)], we next checked the feasibility of transient transformation. Cryopreserved protoplast cells (107) were transformed by the PEG method with a plasmid harboring the GFP gene driven by the glycerol-3-phosphate dehydrogenase 2 promoter from Agaricus bisporus (AbGPD2
pro)7. The transformed protoplasts were transferred directly to a 96-well glass-bottom dish and inspected under a fluorescence microscope after 24
hours (Fig. 1e). The cryopreserved protoplasts displayed clear GFP fluorescence, with transformation rates comparable to those of fresh protoplasts (Fig. 1f), indicating that protoplasts prepared with buffer including 0.5
M sorbitol and 10% DMSO could be stored at −80
°C without any apparent reduction in transformation competency. We thus used cryopreserved protoplasts for all subsequent experiments.
NanoLuc luciferase assay in C. cinerea
Fewer strong promoters are available for use in mushroom-forming basidiomycetes compared to yeast or other model filamentous fungi7. Data from transcriptomic studies using RNA-seq and qRT-PCR had suggested potential candidates for constitutively active promoters in mushroom-forming basidiomycetes20. Because of the limited evaluation methods able to detect promoter activity in vivo, only a few constitutively active promoters from mushroom-forming basidiomycetes have been reported21. Accordingly, we established a luciferase-based promoter-reporter assay in C. cinerea to search for constitutively active promoters with high expression. In a transient assay using 107 cryopreserved protoplasts, we first tested several types of luciferases from firefly, renilla and luminous shrimp (NanoLuc22) (Fig. 2a). Firefly luciferase had no activity in the transient assay, consistent with a previous report7 (Fig. 2b). Unexpectedly, the activity of renilla luciferase was also indistinguishable from that of the negative control. Only NanoLuc exhibited luminescence 24hours after transformation (Fig. 2b). These data indicated that quantitative evaluation of promoter activity in oidia protoplasts could be achieved in C. cinerea using NanoLuc. Since the two other luciferases were not functional, dual-luciferase-assay-based normalization cannot be used in C. cinerea; thus, we normalized the luciferase activity by transformation rate, as determined by co-transformation with a GFP expression plasmid (see Methods).
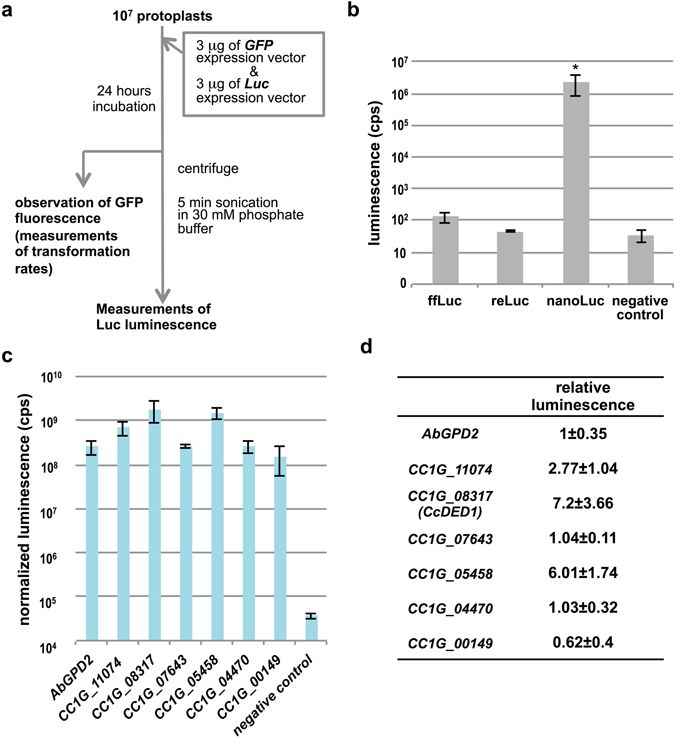
NanoLuc-based luciferase assay using cryopreserved protoplasts identified novel constitutive active promoters in C. cinerea. (a) The flow of luciferase assay in C. cinerea, corresponding data are given in Supplementary Fig. 3. (b) Comparison of three different luciferases driven by AbGPD2pro. Asterisk shows statistical difference calculated with Turkey’s HSD test (P<
0.05). (c) Comparison of activities of various promoters fused with NanoLuc luciferease. Luminescences of NanoLuc were normalized by transformation rate. Bars: S.D., n
=
3. (d) Relative luminescence results of the experiments shown in (c), with the luminescence of AbGPD2pro defined as 1. Number
±
S.E.
Luciferase assay identified novel promoters with strong expression in C. cinerea
Based on our new luciferase assay system, we next analyzed the activities of novel promoters from C. cinerea. We selected six candidate genes which include the presumably constitutive active gene, histone H4, and subjected them to promoter-reporter analysis (Supplementary Table 1). A 2-kbp region from upstream of the start codon of the six candidate genes was fused to the 1st intron of GPD2 from A. bisporus
23 and the NanoLuc coding sequence. Each reporter vector was co-transfected to protoplasts together with the GFP expression plasmid for normalization. All the promoter-reporter experiments were done using protoplast expressing reporters transiently (See Methods). After 24hours of transfection, the luciferase activity in the transformed protoplasts was measured. Three of the six promoters tested showed higher activity compared with the AbGPD2
pro—a conventional constitutively active promoter in C. cinerea (Fig. 2c Supplementary Fig. 3). In particular, the promoter of CC1G_08317 displayed an activity about seven times higher than that of AbGPD2
pro (Fig. 2d). The CC1G_08317 promoter fused with the fluorescent protein Venus also showed strong fluorescence compared with AbGPD2
pro (Supplementary Fig. 4). Vegetative mycelium of CC1G_08317
pro-Venus transformants also showed constitutive Venus fluorescence in vivo (Supplementary Fig. 5). However, according to the expression pattern in the previous study5, CC1G_08317 was not so strongly expressed in 13 developmental stages compared with GPD2 or β-tubulin (Supplementary Fig. 6). Taken together, the NanoLuc-based promoter-reporter assay can thus be used for evaluation of promoter activity in protoplasts of C. cinerea. CC1G_08317 is also annotated as CcDED1—a gene encoding the C. cinerea ortholog of ATP-dependent RNA helicase DED1 in Saccharomyces cerevisiae—. Our data show that CcDED1
pro is a novel strongly expressing promoter in protoplasts of C. cinerea.
Construction of CRISPR/Cas9 system using the novel CcDED1 promoter
No CRISPR/Cas9 system has yet been reported in mushroom-forming basidiomycetes. Therefore, we constructed a CRISPR/Cas9 system optimized for C. cinerea using our new strong promoter, and characterized CRISPR/Cas9 constructs harboring the new promoter “CcDED1 pro” (Fig. 3a). A U6-snRNA promoter from the C. cinerea genome was used for expression of the gRNA. Codon optimization is also an important issue in the optimization of CRISPR/Cas9 systems15, thus we tested four different codon-optimized Cas9: human codon- (hco_Cas9), Arabidopsis codon- (Atco_Cas9), Candida codon- (caco_Cas9), and basidiomycete codon-optimized Cas9 (bco_Cas9, see Methods). As a target for CRISPR/Cas9, we constructed a CRISPR/Cas9 vector with the gRNA targeted to the sequence of the GFP gene24. We transformed cryopreserved C. cinerea protoplasts of a stable GFP expression line with the CRISPR/Cas9 vectors. The transformants were selected on medium containing hygromycin-B (Supplementary Fig. 7). Next, we analyzed the PCR-amplified genomic fragment of target sites from transformants (Fig. 3b). There were no obvious mobility changes in the PCR products from transformants expressing Atco_Cas9, hco_Cas9 or caco_Cas9 (data not shown). On the other hand, direct sequencing with PCR products from transformants expressing bco_Cas9 showed mosaic peaks in some lines (Supplementary Fig. 8). Based on the sequencing analysis, mutation isolation efficiency was 21% (four mosaic lines per 19 transformants). In four putative genome-edited lines, two samples displaying apparently different mobility shift patterns compared to wild-type PCR products (Fig. 3b) were subjected to re-culture and direct sequencing again. The analyses revealed clear mutations: a 75-bp insertion and 1-bp deletion, respectively (Fig. 3c). The positions of these mutations were 3-bp upstream of the protospacer adjacent motif (PAM), where DSB by Cas9 was expected to have occurred13. These mutations clearly indicated that genome editing events by CRISPR/Cas9 had indeed occurred in these transformants.
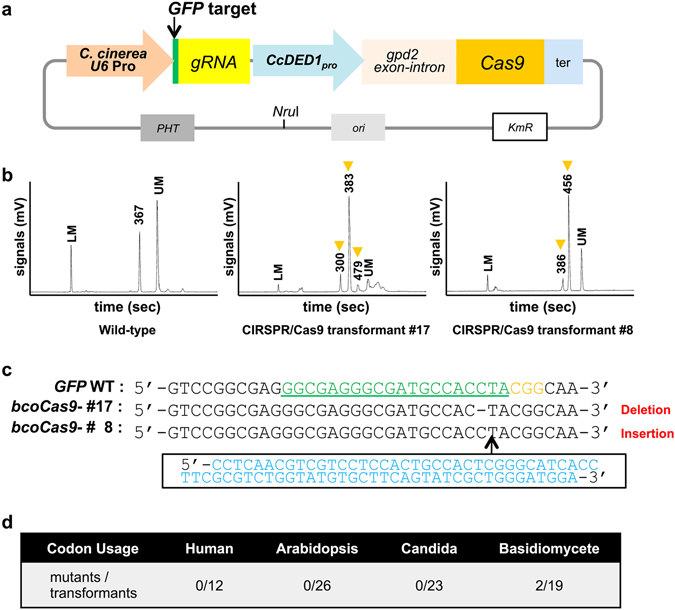
Construction of a CRISPR/Cas9 system in C. cinerea. (a) The CRISPR/Cas9 vector for C. cinerea genome editing. Four types of codon-optimized Cas9 were tested. An NruI site was used for linearization. (b) Capillary gel electrophoresis of the PCR products of CRISPR/Cas9 transformants #17 and #8. Arrowheads show peaks not detected in wild-type. LM; lower marker, UM; upper marker. (c) Sequences obtained by direct sequencing of PCR products from the two CRISPR/Cas9 transformants. The target sequence of gRNA (green) and PAM sequence (yellow) are indicated. The 75-bp insertion in the line #8 is in blue. (d) Comparison of the mutation rates of four types of codon optimization in the transformants as detected by capillary gel electrophoresis analyses.
Isolation of genome editing lines of C. cinerea
To analyze mosaicism of the two mutants, we conducted sequencing by Miseq with low depth. The deep sequencing analysis showed that 74.7% and 91.4%, respectively, of the reads in the two transformants had mutations (Fig. 4a), and the variety of mutations was very low in each mutant. Line #8, which was shown to have the 75bp insertion in direct sequencing analysis (Fig. 3c), did not harbor any other mutations. The other line #17, which was shown to have a 1-bp deletion mutation, had only three types of mutations in the target site (Fig. 4a). Since the mosaicism of transformants could be derived from single transformed cell, genome editing events seem to have been occurred in the various timing of the development of C. cinerea. Since all the detected variations of mutations would cause a frame-shift in, or amino acid insertions to, GFP, these data suggested that both mutant lines would lead to disruption of GFP function. As expected, the two lines showed loss of GFP signal in hyphae and even in fruiting body primordia (Fig. 4b,c). We confirmed that the GFP expression cassette was existed on the genome from both original GFP and mutant lines (Supplementary Fig. 9). These data suggested that the two lines were genome-edited individuals. Taken together, the results showed that the efficiency of our newly constructed bcoCas9-based CRISPR/Cas9 system is sufficient to isolate mutants of genes of interest.
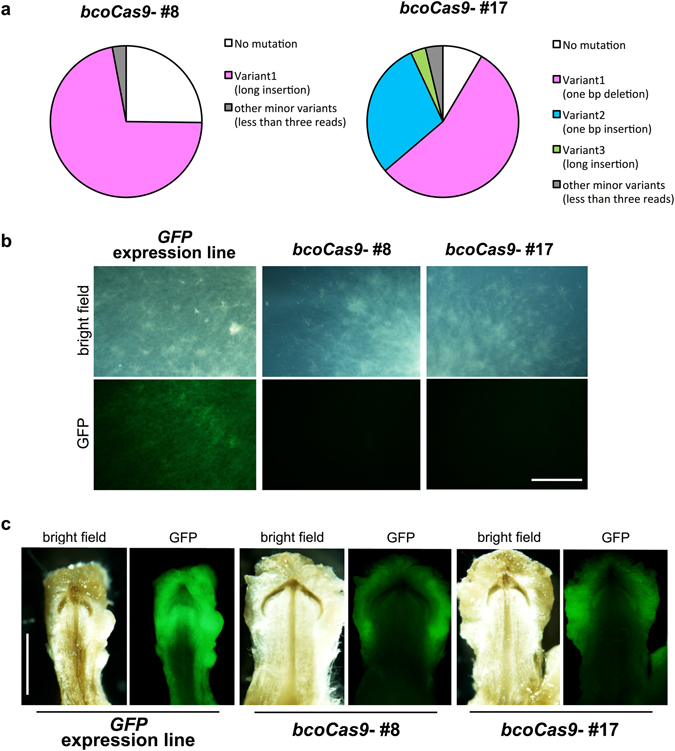
Isolation of genome-edited mushrooms. (a) Analyses of mosaic rates in the C. cinerea CRISPR/Cas9 mutant lines. Ratios of reads with mutation to total reads are described for each variant. Total read counts were 373 reads for #8 and 621 reads for #17, respectively. (b,c) GFP loss-of-function in the C. cinerea CRISPR/Cas9 mutants. GFP fluorescence was diminished in vegetative mycelium (b), and fruiting body primordia (c) in the transformants. Bars=
1
mm.
Discussion
The throughput of reverse genetics is greatly affected by the efficiency of basic transformation techniques. In our study, a buffer consisting of 0.5M sorbitol and 10% DMSO enabled us to preserve protoplasts prepared from C. cinerea oidia that can be stored at −80
°C for up to 1 year with transformation competency, although it is possible that the efficiency could be decreased after 1-year-strotage (Supplementary Fig. 1 and Fig. 1d). Mannitol has commonly been used as an osmotic stabilizer in C. cinerea; however, it is known that mannitol crystallizes at low temperature more easily than sorbitol25. It is consistent with the report that sorbitol could be used as an osmotic stabilizer for cryopreservation of protoplasts of Schizophyllum commune
10. The cryopreservation of protoplasts strengthens efforts to develop high-throughput screening in mushroom-forming basidiomycetes. In practice, we developed the NanoLuc-based reporter assay by throughput screening of more than 50 assay conditions. The repeated comparison of the activities of a number of promoters would be difficult in mushroom-forming basidiomycetes if only freshly prepared protoplasts were used. Our reporter assay used only protoplasts from oidia of C. cinerea; however, it might be possible that protoplasts from other tissues and species were cryopreserved by similar methods. Luciferase is one of the most suitable systems for reporter assays because of its broad dynamic range. This study is the first to combine the use of NanoLuc and cryopreserved protoplasts, establishing the luciferase assay in mushroom-forming basidiomycetes. Unexpectedly, two of three luciferases tested had no activity in C. cinerea (Fig. 2b). NanoLuc has 51% GC content sequence, on the other hand, firefly Luc and renilla Luc have GC contents of 47 and 37%, respectively. Since the GC content of the C. cinerea genome has been reported as about 51%, these data imply that codon usage could affect activity in C. cinerea. This is consistent with the report that AT-Rich region of foregin hph gene might lead truncation of its transcripts in the basidiomycete, S. commune
26. Another possibility might be the mislocalization of firefly Luc and renilla Luc in C. cinerea. The NanoLuc system, in contrast, performed well in our system. NanoLuc will be useful in analyzing cis-elements in the many genes that have been cloned in the long history of studying the genetics of mushroom-forming basidiomycetes27, 28. For instance, cis- analyses of PriA
29, a marker gene for early development of the fruiting body, whose UTR elements has been analyzed30, will be informative in helping to unravel some aspects of the mechanism of the onset of fruiting body development.
In this study, we optimized the CRISPR/Cas9 for mushroom-forming basidiomycetes using the constitutively active promoter CcDED1 pro for Cas9 expression. CcDED1 pro was identified from C. cinerea using a NanoLuc-based reporter assay as a valuable tool for over-expression of transgenes in protoplasts. Interestingly, from in silico analyses, CcDED1 presumably was not so strongly expressed compared with GPD2 or β-tubulin (Supplementary Fig. 6). These data did not correspond to the results of the luciferase assay (Fig. 2). We cloned the 2-kbp upstream region of CcDED1, and it is possible that the activity of the 2-kb promoter might not reflect to its native transcription activity. In addition, there is the possibility that the promoter activity on the ectopically integrated transgene locus differs from its native transcription activity and expression pattern. In the CRISPR/Cas9 system, GC content might also affect the activity of Cas9 in C. cinerea. Only bcoCas9, which most resembled the C. cinerea genome in GC content among the codon variants of Cas9 tested, showed genome editing activity in vivo. These data suggest that codon optimization for other organisms strongly affected the activity of Cas9. The unidentified subcellular localization mechanisms in C. cinerea also might affect the activity of Cas9.
This study is the first to report CRISPR/Cas9-based genome editing in C. cinerea. In the two types of mutation detected by direct sequencing analyses (Fig. 3c), the inserted 75-bp DNA fragment in the target GFP loci of the line #8 perfectly matched the sequence of the C. cinerea genome. The matched sequence corresponded to the gene CC1G_06233, encoding the protein phosphatase methylesterase 1. The fragment of 75bp likely had microhomology to the targeting site (Supplementary Fig. 10). This suggests that a homology-dependent repair pathway could function in C. cinerea when DSBs occur. Mutant isolation efficiency, which is defined by the ratio of isolated genome-edited transformants to all transformants, was 10.5% (two out of 19 transformants). The mutant isolation efficiency can be applied for reverse genetics; however, compared with CRISPR/Cas9 efficiency in other filamentous fungi, the rate was relatively low14–19. To improve the efficiency, screening of other candidate U6 promoters for gRNA expressions, and oidia-specific promoters for Cas9 expression would be future targets.
In conclusion, a high-throughput transformation system, and a CRISPR/Cas9 system customized for C. cinerea were developed in this study. Using the CRISPR/Cas9 system, high-throughput screening to identify key genes regulating processes such as fruiting body development and the generation of useful metabolites will be possible. Our study provides tools to accelerate not only functional genetics in the model mushroom-forming basidiomycetes C. cinerea but also molecular breeding of various edible mushrooms.
Methods
Materials and culture conditions
Coprinopsis cinerea strain #326 (A43mut B43mut pab1-1) was cultured on Malt extract/Yeast extract/Glucose (MYG) medium solidified with 1.5% agar31. Vegetative mycelia of C. cinerea was grown at 28°C (12
hrs/12
hrs light/dark) in a chamber KCLP-1400II CT (Nippon Medical & Chemical Instruments).
Vector construction
Cloning DNA fragments of key components into the vector backbone plasmid was done by using either the standard ligation with T4 DNA ligase (NEB) or an In-Fusion HD-Cloning kit (Clontech). The GFP expression cassette composed of the GPD2 promoter from Agaricus bisporus, EGFP (Clontech) and the heat shock protein 26kDa gene terminator from A. bisporus (T26), was synthesized (GenScript) and cloned into pUC57 to yield pCop007. The wild-type PAB1 from C. cinerea
32 was cloned into Bluescript KS(+) (Stratagene) to yield KS(+)PAB1. Both pCop007 and KS(+)PAB1 were used for establishment of the GFP-expressing C. cinerea line.
NanoLuc™ luciferase (Promega) vectors were constructed as follows: the NanoLuc ORF was amplified by PCR with primers described in Supplementary Table 2.
The NanoLuc fragment was cloned into the Xba I-Sac I site of pCop003 to yield pCop067. Several promoter fragments from C. cinerea were amplified using C. cinerea #326 genomic DNA as a template. The PCR products were cloned into the Asc I- Nco I site of pCop067.
The Cas9 ORF codon-optimized for basidiomycetes was synthesized (GeneScript). The Cas9, gRNA and hygromycin resistance gene cassettes were introduced into plasmid pE33 modified from pDONR221(P3-P2) (Thermo Scientific) to yield the CRISPR/Cas9 vector pCop108 (see Supplemental Methods for details). The annealed oligo DNA fragment corresponding to the GFP gRNA target was inserted into the Bsa I site between the U6-1pr o and the gRNA scaffold sequence to yield pCop108_GFP. Restriction enzymes used in the construction were purchased from NEB. The primers and oligos for the vector construction are listed in Supplementary Table 2.
Cryopreservation
Protoplasts were prepared as described previously8. For cryopreservation, 10µl of protoplast solution suspended in MM buffer (0.5
M mannitol, 0.05
M maleate, pH 5.5) was mixed with 90
μl of preservation buffers. Protoplast solution in preservation buffer was transferred to a deep freezer (−80
°C) with/without a freezing container (Mr. Frosty; Thermo Scientific) for gradual cooling. Components of preservation buffers were as follows; Buffer 1: 0.5
M sorbitol, 0.04
M maleate, pH 5.5, 8% glycerol, Buffer 2: MM buffer (pH 5.5), 8% glycerol, Buffer 3: 0.5
M sorbitol, 0.04
M maleate, pH 5.5, Buffer 4: MM buffer (pH 5.5), 8% glycerol, Buffer 5: 0.5
M sorbitol, 0.02
M maleate, pH 5.5, 10% DMSO, 25% w/v PEG, 0.6% w/v CaCl2.
PEG-based transformation
Transformation was performed as described previously11. Briefly, 108 cells in 100µL of protoplast preservation buffer were mixed with 10
µl of DNA solution containing 1–3
µg plasmids. The mixed solution was added to PEG-Ca buffer (25% w/v PEG and 0.6% w/v CaCl2) and put on ice for 20
min. A further 1
mL of PEG-Ca was added to the sample, which was stood for 5
min at room temperature. 2
mL of STC buffer was then added and the samples stored for 24
hrs. The hygromycin selection of transformed cells was performed as described by Cummings et al.33. Transformed protoplasts were spread on regeneration agar containing 0.1
mM p-aminobenzoic acid. After 17-18
hrs pre-incubation without hygromycin, 5
ml regeneration top-agar containing 600
mg/L hygromycin-B and transformants were sub-cultured onto minimal medium agar plates containing 100
mg/L hygromycin-B. Transformation rates were calculated by the number of hygromycin-B resistant colonies in 108 preserved cells which were transformed with the PHT1 plasmid7.
Transient assay of GFP fluorescence
The PEG-based transformation assay described above was conducted with 107 protoplasts in a final volume of 300µl. 107 cells were sufficient to detect GFP signals in transient assay and also useful to test multiple conditions. The transformed cells were transferred to a 96-well glass bottom dish (Thermo Scientific) and cultured at 28
°C overnight. The cells were inspected using a desktop fluorescent microscope JULI (Ruskinn). The transformation rates were calculated by relating the number of GFP positive protoplasts to all the protoplasts in a field of view. Counting of GFP positive cells and counting all the cells was achieved using the macro of ImageJ34 (http://imagej.nih.gov/ij/).
Luciferase assay
107 protoplasts were co-transformed with 3µg of the NanoLuc vector and 3
µg of the GFP vector pCop007. Transformed protoplasts were incubated at 28
°C for 16–24
hrs. After incubation, protoplasts were centrifuged for 5
min at 600
g. Pellets were re-suspended in 100
µl of phosphate buffer (pH 5.5), mixed by pipetting, and disrupted by 10 time cycles of the 30 sec-sonication with the Bioruptor sonicator (CosmoBio) with HIGH setting/the 30 sec-cooling down on ice. The cell lysates were transferred to OptiPlate-96 (PerkinElmer), and the Luc activity quantified with a Nano-Glo Luciferase assay kit (Promega) following the manufacturer’s protocol. Luminescence was measured with a luminometer GloMax®-Multi Detection System (Promega). Normalized luminescence of each sample was calculated by dividing the luminescence by the transformation rate as following.
Isolation of genome edited lines of C. cinerea
Protoplasts from GFP-expressing C. cinerea were transformed with pCop108_GFP. After 2–3 days incubation, a part of each individual transformed mycelium was picked up and subjected to DNA extraction. The samples were directly dipped in 100µl TE and heated at 95
°C for 10
min. One µl of the extracts was used as the template for PCR using ExTaq polymerase (Takara) following the manufacturer’s protocol. The PCR products were analyzed by capillary based electrophoresis using MultiNA (Shimazu). The samples were also subjected to direct sequencing using an ABI 3130 Genetic Analyzer (Applied Biosystems), followed by deep sequencing. GFP activity was monitored using a fluorescence stereo microscope Leica M205 FA (Leica Microsystems). The primers used for genotyping are listed in Supplementary Table 1.
Deep sequencing analysis
The PCR products of the target site in each transformant were subjected to additional amplification with adaptor-tagged primers (GFP seqF+
adapter: 5′-ACACTCTTTCCCTACACGACGCTCTTCCGATCTCGTAAACGGCCACAAGTTCAG-3′ and GFP seqR
+
adapter: 5′-GTGACTGGAGTTCAGACGTGTGCTCTTCCGATCTCACGAGGGTGGGCCAG-3′). The library was denatured with 1
N NaOH and mixed with a PhiX control (Illumina) prior to analysis with Miseq (Illumina).The reads with mutations were categorized using the 20
bp sequence including the putative DSB site. The mosaic rate of the transgenic lines described in Fig. 4 was calculated with Excel (Microsoft).
Statistical analysis
Statistical analyses were carried out with R (http://www.r-project.org/). The statistical significance (P value) was used to compare samples. The P values of the comparison of multiple samples were computed using Bartlett test and Kruskal-Wallis test following Turkey’s HSD test.
Acknowledgements
We thank M. Fukuhara for her technical assistance. We also thank all the members of Setsuro Fujii Memorial Institute of Medical Science for facilities in this research. We appreciate S. Sawatsubashi for his helpful suggestions for luciferase assays, and H. Muraguchi, Y. Sakamoto, and T. Nakazawa for their critical comments on this manuscript. This work was supported by a donation from Otsuka Pharmaceutical Factory, Inc., a funding for innovative distinctive projects from Tokushima University.
Author Contributions
K.O. and S.S.S. designed the whole project. K.O. and Y.O. designed the CRISPR/Cas9 vectors. H.S., S.S.S., H.C., and E.S. performed most of C. cinerea experiments. S.N. contributed to obtaining the C. cinerea strain. S.S.S., Y.O. and K.O. wrote the manuscript.
Footnotes
Shigeo S. Sugano and Hiroko Suzuki contributed equally to this work.
Electronic supplementary material
Supplementary information accompanies this paper at 10.1038/s41598-017-00883-5
Publisher's note: Springer Nature remains neutral with regard to jurisdictional claims in published maps and institutional affiliations.
References
Articles from Scientific Reports are provided here courtesy of Nature Publishing Group
Full text links
Read article at publisher's site: https://doi.org/10.1038/s41598-017-00883-5
Read article for free, from open access legal sources, via Unpaywall:
https://www.nature.com/articles/s41598-017-00883-5.pdf
Citations & impact
Impact metrics
Citations of article over time
Alternative metrics
Smart citations by scite.ai
Explore citation contexts and check if this article has been
supported or disputed.
https://scite.ai/reports/10.1038/s41598-017-00883-5
Article citations
Current Advances in the Functional Genes of Edible and Medicinal Fungi: Research Techniques, Functional Analysis, and Prospects.
J Fungi (Basel), 10(5):311, 25 Apr 2024
Cited by: 0 articles | PMID: 38786666 | PMCID: PMC11121823
Review Free full text in Europe PMC
Utilization of CRISPR-Cas genome editing technology in filamentous fungi: function and advancement potentiality.
Front Microbiol, 15:1375120, 28 Mar 2024
Cited by: 0 articles | PMID: 38605715 | PMCID: PMC11007153
Review Free full text in Europe PMC
Advancements in genetic studies of mushrooms: a comprehensive review.
World J Microbiol Biotechnol, 40(9):275, 22 Jul 2024
Cited by: 1 article | PMID: 39034336
Review
Pleurotus ostreatus as a model mushroom in genetics, cell biology, and material sciences.
Appl Microbiol Biotechnol, 108(1):217, 19 Feb 2024
Cited by: 0 articles | PMID: 38372792 | PMCID: PMC10876731
Review Free full text in Europe PMC
The Cas9-gRNA ribonucleoprotein complex-mediated editing of pyrG in Ganoderma lucidum and unexpected insertion of contaminated DNA fragments.
Sci Rep, 13(1):11133, 10 Jul 2023
Cited by: 5 articles | PMID: 37429890 | PMCID: PMC10333205
Go to all (34) article citations
Data
Data behind the article
This data has been text mined from the article, or deposited into data resources.
BioStudies: supplemental material and supporting data
Similar Articles
To arrive at the top five similar articles we use a word-weighted algorithm to compare words from the Title and Abstract of each citation.
CRISPR/Cas9-mediated efficient genome editing via protoplast-based transformation in yeast-like fungus Aureobasidium pullulans.
Gene, 709:8-16, 25 May 2019
Cited by: 9 articles | PMID: 31132514
Improved CRISPR/Cas9 gene editing by fluorescence activated cell sorting of green fluorescence protein tagged protoplasts.
BMC Biotechnol, 19(1):36, 17 Jun 2019
Cited by: 15 articles | PMID: 31208390 | PMCID: PMC6580576
CRISPR/Cas9-Mediated Genome Editing of Trichoderma reesei.
Methods Mol Biol, 2234:87-98, 01 Jan 2021
Cited by: 6 articles | PMID: 33165782
Genome editing tools based improved applications in macrofungi.
Mol Biol Rep, 51(1):873, 30 Jul 2024
Cited by: 0 articles | PMID: 39080117
Review