Abstract
Free full text

Selective targeting of myeloid-derived suppressor cells in cancer patients using DS-8273a, an agonistic TRAIL-R2 antibody
Abstract
Purpose
Myeloid-derived suppressor cells (MDSC) one of the major contributors to immune suppression in cancer. We recently have demonstrated in preclinical study that MDSC are sensitive to TRAIL receptor 2 (TRAIL-R2) agonist. The goal of this study was to clinically test the hypothesis that targeting TRAIL-R2 can selectively eliminate MDSC.
Experimental Design
The TRAIL-R2 agonistic antibody (DS-8273a) has been tested in 16 patients with advanced cancers enrolled in a phase 1 trial. The antibody (24 mg/kg) was administered IV once every 3 weeks till disease progression, unacceptable toxicities, or withdrawal of consent. The safety and the presence of various populations of myeloid and lymphoid cells in peripheral blood and tumor tissues were evaluated.
Results
The treatment was well tolerated with only mild to moderate adverse events attributable to the study drug. Treatment with DS-8273a resulted in reduction of the elevated numbers of MDSC in the peripheral blood of most patients to the levels observed in healthy volunteers. However, in several patients, MDSC rebounded back to the pre-treatment level by day 42. In contrast, DS-8273a did not affect the number of neutrophils, monocytes, and other populations of myeloid and lymphoid cells. Decrease in MDSC inversely correlated with the length of progression-free survival. In tumors, DS-8273a treatment resulted in a decrease of MDSC in 50% of the patients who were able to provide pre- and on-treatment biopsies.
Conclusion
Targeting TRAIL-R2 resulted in elimination of different populations of MDSC without affecting mature myeloid or lymphoid cells. These data support the use of this antibody in combination immmunotherapy of cancer.
Trial registration
ClinicalTrials.gov NCT02076451.
Introduction
It is now well established that the myeloid cells play an important role in regulation of tumor progression and metastases as well as in limiting the effects of cancer immunotherapy (1). Consequently, combination of immune checkpoint blockade, or T cell based immunotherapy including treatment with chimeric antigen receptor T (CAR-T) cells, T cell receptor-transduced T (TCR-T) cells or tumor infiltrating lymphocytes (TIL) with therapy that targets the immunosuppressive microenvironment holds great promise (2, 3). Myeloid-derived suppressor cells (MDSC) represent one of the major immune suppressive populations in cancer patients. They consist of populations of polymorphonuclear (PMN-MDSC), monocytic (M-MDSC) and early precursors (eMDSC) (4). In addition to their suppressive abilities, MDSC can also promote tumor survival, angiogenesis and metastasis (5). MDSC accumulation has recently been correlated with tumor burden, as well as overall survival, disease free survival, and recurrence free survival in different tumor types (6, 7). Importantly, MDSC accumulation has been reported to correlate with resistance to ipilimumab or nivolumab treatment in melanoma (8–13). Several strategies to target MDSC have been proposed and are based on inducing depletion or inhibiting their suppressive activity, differentiation or accumulation (14). However, none of these strategies are specific to MDSC and many of them (e.g. chemotherapy) have substantial side effects. We have recently reported that MDSC could be selectively targeted in pre-clinical settings using TRAIL-R2 agonistic antibody (15).
TNF-related apoptosis induced ligand-receptors (TRAIL-R) are members of the TNF receptor superfamily and consist of 2 death receptors, TRAIL-R1 (DR4/CD261) and TRAIL-R2 (DR5/CD262), 2 decoy receptors (DcR1/CD263 and DcR2/CD264) and one soluble receptor (OPG: osteoprotegerin) (16, 17). Ligation of TRAIL with DR4 or DR5 induces trimerization of the receptor, which activates an apoptotic pathway (18). DcR1 is a glycosylphosphatidylinositol (GPI)-linked protein lacking an intracellular domain, and DcR2 contains a truncated death domain. These two receptors can prevent TRAIL-induced apoptosis by competing with DR4 and DR5 for binding to TRAIL or by inhibiting apoptosis via formation of ligand-independent complexes between DR5 and DcR2 (19). In mice, agonistic DR5 antibody potentiated the effect of immune checkpoint blockade (CTLA-4 targeting antibody), which resulted in a 5-fold decrease of the tumor growth (15). This suggests that TRAIL-R targeting antibody can be potentially used in selective elimination of MDSC in cancer patients. We tested this hypothesis in patients with advanced cancers enrolled in a phase I trial of TRAIL-R2 agonistic antibody DS-8273a.
Materials and Methods
Sample collection and preparation
All samples were collected from patients at Sarah Cannon Research Institute, University of Alabama Birmingham and University of Chicago who were enrolled in the phase 1 study registered at clinicaltrials.gov (NCT02076451). Peripheral blood was collected from 16 subjects and pre- and on-treatment tumor tissue from 6 subjects with advanced stage solid tumors. Study was approved by institutional review boards of participating institutions and all patient samples were collected with informed consent. Clinical characteristics are described in Table 1.
Table 1
Main clinical characteristics of patients
Age | Gender | Cancer Type | Histology | Stage | Prior Chemotherapy | |
---|---|---|---|---|---|---|
Pt 0002-0014 | 37 | F | BREAST | ADENOCARCINOMA | IV | Capecitabine / Taxol / MM-121 / Tamoxifen / Docetaxel |
Pt 0003-0012 | 51 | M | COLORECTAL | ADENOCARCINOMA | IV | Oxaliplatin / Bevacizumab / Irinotecan / 5-FU / Mitomycin C / Panitumumab |
Pt 0002-0015 | 63 | F | BREAST | INVASIVE DUCTAL CARCINOMA | IV | Faslodex / Halaven / Tamoxifen / Everolimus |
Pt 0003-0016 | 67 | M | PANCREATIC | ADENOCARCINOMA | IV | Gemcitabine / 5-FU / Irinotecan / Abraxane / Oxaliplatin / Leucovorin |
Pt 0002-0018 | 65 | F | OVARIAN | SEROUS CARCINOMA | IIIC | Topotecan / Filgrastim / Tamoxifen / Paclitaxel / Gemcitabine |
Pt 0002-0019 | 63 | F | ENDOMETRIAL | ADENOCARCINOMA | IIIB | Taxotere / Carboplatin |
Pt 0001-0021 | 50 | M | APPENDICEAL | MUCINOUS ADENOCARCINOMA | IV | |
Pt 0001-0026 | 45 | F | LIPOSARCOMA | MIXED SUBTYPES | IV | MDM2/MDMX inhibitor |
Pt 0002-0023 | 51 | F | COLORECTAL | METASTATIC ADENOCARCINOMA | IV | Leucovorin / Fluorouracil / Avastin / Oxaliplatin / Irinotecan / Zaltrap |
Pt 0002-0025 | 58 | M | COLORECTAL | ADENOCARCINOMA | IV | Zaltrap / Avastin / Xeloda / leucovorin / Irinotecan / Fluorouracil |
Pt 0003-0028 | 76 | F | MELANOMA | ACRAL MALIGNANT | IV | Nivolumab / Ipilimumab / Temozolomide / Lirilumab |
Pt 0002-0027 | 58 | F | COLORECTAL | METASTATIC ADENOCARCINOMA | IIIC | Irinotecan / Fluorouracil / Avastin / Granisetron / Oxaliplatin / Bevacizumab / leucovorin / Regorafnib / Panitumumab |
Pt 0003-0031 | 25 | F | OSTEOSARCOMA | HIGH GRADE OSTEOSARCOMA | IV | Docetaxel / Gemcitabine |
Pt 0002-0033 | 57 | F | OVARIAN | PAPILLARY SEROUS CARCINOMA | IV | Avastin / Gemzar / Carboplatin / Taxotere |
Pt 0003-0034 | 39 | M | LEIOMYOSARCOMA | LEIOMYOSARCOMA | IV | Sirolimus / Gemcitabine / Pazopanib / TH-302 / Doxorubicin / Docetaxel / Dacarzabine |
Pt 0002-0035 | 62 | M | LIVER | HEPATOCELLULAR CARCINOMA | IV | Nexavar / Doxorubicin |
Peripheral blood from 6 patients (5 males and 1 females, age 53–82) with stage III head and neck cancer who were not part of the treatment protocol was collected from Helen F. Graham Cancer Center. That portion of the study was approved by the Institutional Review Boards of the Helen F. Graham Cancer Center, and The Wistar Institute. The patient samples were collected with informed consent. Peripheral blood from 12 healthy donors was used as a control and was obtained at the Wistar Institute in accordance to the institutional IRB protocol. All samples were analyzed within 24–36 hours following collection.
Cell isolation and culture
Human PBMC were isolated on a ficoll gradient following the manufacturer’s recommendation (Amersham). Cells were then cultured in RPMI (Biosource International) supplemented with 10% FBS, 5 mM glutamine, 25 mM HEPES, 50 μM β-mercaptoethanol and 1% antibiotics (Invitrogen). For the experiments where myeloid cells were placed in culture, recombinant GM-CSF was added to the media at a concentration of 10 ng/mL (Peprotech). For MDSC isolation, PBMC and neutrophils were isolated using a histopaque gradient followed by isolation with CD15 antibody and magnetic beads. M-MDSC were sorted by flow cytometry from the PBMC depleted of PMN-MDSC after staining with CD14 and HLA-DR antibodies. The purity of all population was >90% following isolation.
Suppression assay
T cells from one healthy donor were purified using a human T cell enrichment column (R&D) and used as responder cells. Dendritic cells were generated from adherent monocytes in the presence of GM-CSF and IL4 (Peprotech; 20 ng/mL and 10 ng/mL respectively) for 6 days as previously described (20) and used as stimulator cells. Responder and stimulator cells were then mixed at a 10:1 ratio and different subsets of MDSC were added at various ratios (1:1, 1:2, and 1:4 MDSC to T cell ratio). T cell proliferation was assessed after 5 days of culture by thymidine incorporation.
In Vitro Killing Assay
Human PBMC and PMN were isolated by centrifugation over a double density Histopaque (Sigma Aldrich) gradient (1.077 to collect PBMC and 1.119 to collect PMN) from cancer patients. Cells were then culture in RPMI supplemented with 10% FBS, 5 mM glutamine, 25 mM HEPES, 50μM β-mercaptoethanol and 1% antibiotics with 10 ng/ml of rhGM-CSF. DS-8273a was added to culture for overnight incubation followed by flow cytometry analysis of M-MDSC or PMN-MDSC levels for cell recovery. In some studies, healthy donor PMN cells were cultured with or without 1 μM thapsigargin (THG) (Sigma Aldrich) for 4 and 24 hours. Samples were analyzed by flow cytometry for TRAIL-R3 (DcR1), TRAIL-R4 (DcR2), and DR5 (CD262) expression at each time point. After 4 hours, DS-8273a was added to wells at a concentration of 4 μg/ml with or without 2.5 μg/ml of mouse anti-human IgG1 (Thermo Fisher) to facilitate cross-linking. Cell recovery was assessed using a Countess II FL Automated Cell Counter (Thermo Fisher).
Flow cytometry
The antibody panels used in this study are described in supplementary table S1. FoxP3 staining was performed using the human FoxP3 buffer set according to the manufacturer’s recommendations (BD Biosciences). All flow cytometry data were acquired using a BD LSR II flow cytometer and analyzed using FlowJo software (Tree Star).
Immunofluorescence/Immunohistochemistry
Following deparaffinization and rehydration, heat induced antigen retrieval was performed using Tris-EDTA buffer pH 9. Tissues were stained for S100A9 (Novus Biologicals), CD33 (Novocastra), CD8, Neutrophil Elastase, and CD163 (Abcam). PD-1 antibody was obtained from R&D Systems. The following fluorescently conjugated secondary mAb (Life technologies) were used: anti-rabbit IgG AF594 for S100A9 and neutrophil Elastase, anti-mouse IgG AF647 for CD33 and anti-mouse IgG AF594 for CD163. Alexa-594 donkey anti-mouse IgG was used for CD8 and Alexa-647 donkey anti-goat antibody for PD1. Cell nuclei were stained using DAPI (Life technologies). Images were obtained using Leica TCS SP5 Confocal microscope. 16 frames at 63X magnification were used to calculate the cell count/mm2.
IHC Staining was performed on a Bond Max automated staining system (Leica Microsystems). The Bond Refine polymer staining kit (Leica Microsystems) was used. FoxP3 mAb (Biolegend), CD4 mAb (Leica microsystems) and CD8 mAb (DAKO) were used and antigen retrieval was performed with ER2 and ER1 (Leica Microsystems) retrieval solutions. Slides were rinsed, dehydrated through a series of ascending concentrations of ethanol and xylene and then mounted. Images were obtained using Nikon E600 Upright Microscope. 12 frames at 40X magnification were used to calculate the cell count/mm2.
Anti-drug antibody detection (ADA)
ADA against DS-8273a in the patient plasma were assayed using an electrochemiluminescent (ECL) immunoassay in a bridging assay format. Biotin-labeled (B) and ruthenium-labeled (Ru) DS-8273a were used as capture and detection reagents for anti-DS-8273a antibodies, and the ECL signal generated in the assay in the presence of the Ru-DS-8273a/anti-DS-8273a/B-DS-8273a immune complex was measured using the Meso-Scale Discovery (MSD) PRTM 6000 Plate Reader. If a sample was tested ADA-positive, a confirmatory test was performed and considered final.
Statistics
Statistical analysis was performed using a 2-tailed Student’s t-test (unless otherwise stated), correlation analysis using Spearman rank test. GraphPad Prism 5 software (GraphPad Software Inc.) and StataMP13 (StataCorp LP) were used and significance was determined at p < 0.05.
Results
Effect of TRAIL-R2 agonistic antibody on MDSC in vitro
To evaluate the populations of MDSC in peripheral blood mononuclear cell (PBMC) we used previously described criteria (4, 6, 21). MDSC are comprised of a mixed population of early stage precursors and PMN-MDSC (eMDSC) defined as Lin (CD3, 14, 19, 56)−HLA-DR−CD33+, PMN-MDSC defined as CD11b+CD14−CD33+CD15+ cells, and M-MDSC defined as CD14+HLA-DR−/lo cells (Fig. 1A). Other mononuclear cells including various populations of lymphocytes, monocytes, and dendritic cells were also evaluated as described in Fig. S1. In this study, all CD15+ cells present in the PBMC layer were considered as PMN-MDSC. There are currently no fully effective methods or specific markers to distinguish PMN-MDSC from regular neutrophils within the PBMC layer. Due to this fact, we wanted to verify the suppressive activity all CD15+ cells found in the PBMC layer from those found in the high-density PMN fraction. This was confirmed in a direct T-cell suppressive assay using a three-way allogeneic mixed leukocyte reaction using samples from patients with advanced head and neck cancer that were not on the treatment protocol. Fig. 1B demonstrates this comparison of suppressive activity of low-density PMN-MDSC (PBMC fraction) and high-density neutrophils isolated from peripheral blood of the same patients.

A. Phenotype of populations of MDSC evaluated in the study by flow cytometry. Typical example of the analysis of one patient on trial is shown. B. Suppressive activity of PMN-MDSC. PMN-MDSC were isolated from PBMC and neutrophils (PMN) from high density fraction of hystopaque gradient from the same patient followed by magnetic beads isolation with CD15 antibody. Suppressive activity of PMN and PMN-MDSC was evaluated in triplicates in three-way allogeneic MLR. Two patients with HNC cancer (not on trial) were tested and showed similar results. * - p<0.05 from control (no MDSC or PMN added). C. Effect of DS-8273a on survival of cells. Neutrophils, PMN-MDSC, monocytes and M-MDSC were isolated from 4 patients with HNC (not on trial). Cells were cultured for 20 hr with indicated concentration of DS-8273a in the presence of 10 ng/ml rhGM-CSF and viability was assessed by 7AAD staining. * - p<0.05. D. Effect of ER stress inducer THG on TRAIL-Rs expression in PMN. PMN were isolated from 4 different healthy donors and treated for 24 hrs with 20 ng/ml GM-CSF and 1 μM THG. Cells were then collected and analyzed using indicated markers. P values between control (untreated) and THG treated PMN are shown. E. Effect of DS-8273a on THG treated PMN. PMN were isolated from healthy donors and treated with or without 1 μM of THG for 4 hrs in the presence of 10 ng/ml rhGM-CSF followed by the addition of DS-8273a for 24 hours. Untreated – cells incubated with media alone, DS-8273a -4 μg/ml of antibody, DS-8273a + anti-IgG - 4 μg/ml DS-8273a and 2.5 μg/ml anti-IgG1 (to enhance cross-linking of primary antibody). P values of statistically significant differences are shown.
To target MDSC in vitro we used TRAIL-R2 agonistic antibody, DS-8273a developed by Daiichi Sankyo Inc. Cells were isolated from patients with advanced head and neck cancer that were not on the treatment protocol. DS-8273a at concentration 100 μg/ml and higher caused significant killing of PMN-MDSC but not neutrophils from the same patients. Similar results were observed with M-MDSC and monocytes (Fig. 1C).
We have previously implicated ER stress in changes in TRAIL-Rs expression observed in myeloid cells in cancer (15). We asked whether ER stress can make control neutrophils sensitive to DS-8273a. PMN isolated from healthy donors were cultured with GM-CSF and endoplasmic reticulum (ER) stress inducer thapsigargin (THG). THG induced down-regulation of DcR1 and DcR2 on PMN after 4 hours of incubation. The effect became stronger after 24 hours of incubation (Fig. 1D). Importantly, THG had minimal effects on the expression of DR5 consistent with changes observed in human MDSC in a previous study (15). Incubation of PMN for 24 hours with GM-CSF did not sensitize these cells to DS-8273a. However, PMN became sensitive to agonistic TRAIL-R2 antibody after incubation with THG (Fig. 1E). These results indicate that ER stress inducible down-regulation of DcR1 and DcR2 on MDSC make these cells sensitive to the agonistic TRAIL-R2 antibody DS8273a.
Design of the trial and characterization of MDSC targeted by DS-8273a
The effect of DS-8273a was tested in a multicenter two-part phase I clinical trial NCT02076451 in patients with advanced solid tumors. The subjects were treated at escalating doses of single agent DS-8273a starting from 2 mg/kg through 8 mg, 16 mg, and 24 mg per kg in the dose escalation part of the study, followed by a dose expansion part, in which all subjects were treated at the highest dose of 24 mg/kg once every 3 weeks (Q3W) that showed no dose limiting toxicities. The antibody was administered IV Q3W till disease progression, unacceptable toxicities, or withdrawal of consent. The clinical trial demonstrated excellent safety and tolerability of DS-8273a at all the dose levels tested up to 24 mg/kg Q3W (MS submitted). Evaluation of the effect of the antibody on MDSC was performed only in subjects treated with DS-8273a at 24 mg/kg in the dose escalation and the dose expansion cohorts (Fig. S2). The mean Cmax at this dose level after the first infusion of DS-8273a was 621 μg/mL and the Cmin was 132 μg/mL that were both higher than the minimal concentration observed to be effective in inducing apoptosis in the MDSC based on ex vivo experiments mentioned above.
Blood samples were collected from 18 patients who received DS-8273a at 24 mg/kg Q3W. Pre-treatment samples from 2 patients were lost during transportation. Therefore, 16 patients were evaluated (Fig. S3). Most of the patients had stage IV cancer and had failed multiple prior chemotherapies. No concurrent cancer therapy was administrated during the treatment with DS-8273a (Table 1). A risk of anti-drug antibody (ADA) formation affecting continued treatment of the antibody was not identified in the study. Fifteen of the sixteen subjects were ADA-negative at all time points assessed. One patient who developed ADA positivity discontinued the study due to clinical progression, and without a follow up sample for re-testing ADA. PK data showed no appreciable difference in the plasma concentrations of DS-8273a between cycle 1 and cycle 2 (data not shown).
DS-8273a selectively eliminated PMN-MDSC and eMDSC in cancer patients
Samples of blood were collected in heparin tubes at baseline (prior to the treatment), on day 14, 21 (end of cycle 1), 28 and 42 (end of cycle 2) and shipped overnight on ice for analysis (Fig. S2). Control blood samples from healthy volunteers were stored on ice overnight to create comparable conditions. Prior to the beginning of the treatment, patients had lower number of CD4+ T cells, B cells, natural killer (NK) cells, and dendritic cells (DCs) in comparison to healthy donors. In contrast, they had higher numbers of neutrophils, eMDSC and PMN-MDSC (Fig. 2). When various cellular populations were analyzed in all patients during the treatment, no significant effect of DS-8273a was observed on lymphocytes, neutrophils, monocytes, and DCs (Fig. S4). However, a clear trend for a decrease in numbers of MDSC was observed (Fig. S4). To better understand the changes in MDSC, we separated patients based on their pre-treatment levels of eMDSC and PMN-MDSC. In 9 out of 16 patients whose peripheral blood was available for analysis, the MDSC levels were higher than the range established in healthy individuals, whereas in 7 patients it was within the control range (Fig. 3A). In patients with elevated numbers of eMDSC and PMN-MDSC, treatment with DS8273a caused a significant drop in the number of these cells (to a level observed in healthy donors) 14 days after the start of the treatment (Fig. 3B). The presence of these cells remained at the control level on day 21 and started increasing after day 28. A mixed-effects spline model with two knots at 14 and 21 days demonstrated that upward trend was not-significant (p=0.175). A similar trend was continued after the second cycle of treatment; however, the change was not significant (p=0.965). Thus, although populations of PMN-MDSC showed a trend to increase after day 28, it was not statistically significant.

*p<0.05, ** p<0.01, ***p<0.001 from values in healthy donors (HD).
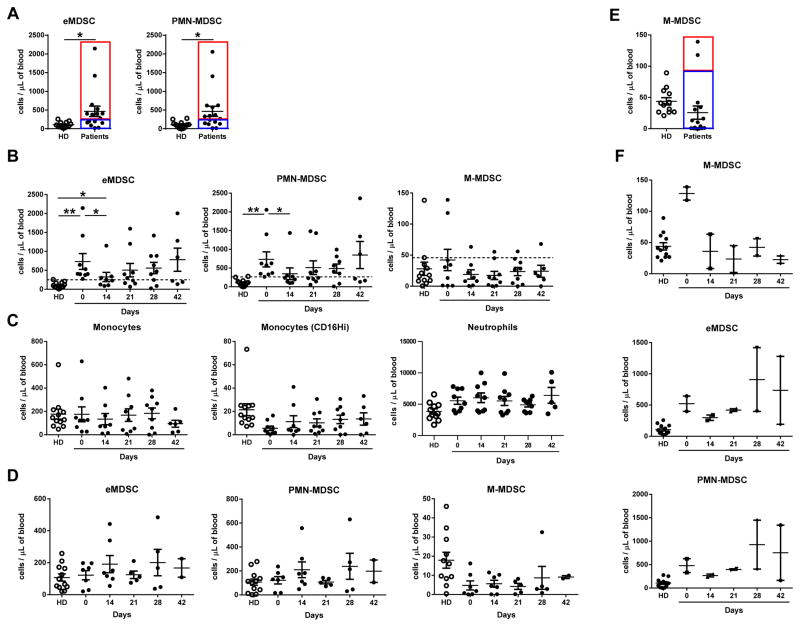
A. Selection of patients with elevated and control level of eMDSC and PMN-MDSC; B. The number of different population of MDSC during the treatment in patients with elevated pre-treatment level of PMN-MDSC; C. The number of monocytes and neutrophils during the treatment in patients with elevated pre-treatment level of PMN-MDSC; D. The number of MDSC in patients with control pre-treatment level of MDSC. E. Selection of patients with elevated and control level of M-MDSC; F. The number of different population of MDSC during the treatment in patients with elevated pre-treatment level of M-MDSC. In this figure *p<0.05, ** p<0.01 from values in healthy donors (HD).
DS-8273a did not affect populations of neutrophils and monocytes (Fig. 3C) nor did it have an impact on other myeloid and lymphoid cells (Fig. S5). Treatment with DS-8273a did not affect the number of MDSC in patients that had pre-treatment eMDSC or PMN-MDSC numbers within the healthy donor range (Fig. 3D).
We performed a similar analysis with the population of M-MDSC. In contrast to PMN-MDSC, only two patients had elevated level of these cells (Fig. 3E). In both patients, DS-8273a dramatically reduced the numbers of M-MDSC to the control level and remained low for the duration of the treatment (Fig. 3F). Thus, DS-8273a specifically target elevated population of MDSC without affecting other myeloid cells and lymphocytes.
Depletion of MDSC in tumor tissue and association with clinical outcome
Tumor biopsies were collected in 10 patients enrolled to the dose expansion cohort. Six of the patients had paired pre- and post-treatment (day 28) biopsies. We evaluated the populations of CD163+ macrophages, elastase positive neutrophils, putative (CD33+S100A9+) MDSC (22) as well as Foxp3 positive regulatory T cells, CD4+, CD8+, and CD8+PD1+ T cells by immunofluorescence staining and immunohistochemistry (Fig. 4A and Fig. S6). Prior to start of the treatment no correlations between the presence of various populations of myeloid cells and T cells were observed. Three out of 6 patients demonstrated a decrease in intratumoral MDSC, whereas one patient had decreased macrophages and neutrophils (Fig. 4B). In those 3 patients who had a decrease in MDSC number within the tumor, the ratios of CD8+: MDSC and CD4+: MDSC, as well as proportion of PD1+ cells among CD8+ T cells: MDSC were increased, whereas in patients with unchanged levels of MDSC, the ratios were either unchanged or decreased (Fig. 4C), indicating that the MDSC decrease was not a reflection of the overall changes in the immune cell infiltration of the tumor and was specific for those cells.

A. Typical examples of staining of tissues. CD33+S100A9+ - immune fluorescent staining (red – S100A9, green – CD33, blue - DAPI). Neutrophil elastase - immune fluorescent staining (red – elastase, blue - DAPI). CD163 – immune fluorescence staining (green – CD163, blue – DAPI). FoxP3, CD4, CD8, PD1+CD8+ - immunohistochemistry staining as described in Methods. B. Changes in the presence of population of myeloid and lymphoid cells in tumor tissues after the treatment with DS-8273a. C. Changes in CD8/MDSC, CD4/MDSC, and proportion of PD1+ out of CD8+ cells/MDSC ratio after the treatment. D. Expression of TRAIL receptors in PMN-MDSC during the treatment. P values of significant differences from healthy donors control are shown. E. Time to progression (months) in patients treated with DS-8273a. Patients on day 28 after start of the treatment were split into two groups: control and elevated number of PMN-MDSC. The control values were established based on the results in healthy donors shown in Fig. 3. P values were calculated in two-way t-test.
Since we have implicated TRAIL-R expression on MDSC as being associated with their sensitivity to agonist antibody, we evaluated the expression of three TRAIL-Rs (DR5, DcR1, DcR2) in PMN-MDSC during the treatment. No changes were observed during the treatment in the expression of DR5 and DcR1 (Fig. 4D). On the other hand, expression of DcR2 was significantly lower on day 14 (p=0.03) with a trend to an increase on days 21 and 28, which follows the pattern of PMN-MDSC changes. Pre-treatment level of DcR2 as well as other TRAIL-Rs did not correlate with subsequent changes in PMN-MDSC numbers.
There were no objective clinical responses (CR and PR) in DS-8273a-treated patients on this trial. We assessed whether the changes in PMN-MDSC during the treatment (ratio between PMN-MDSC numbers during and prior to the treatment) correlated with the time to progression (TTP) or duration of progression-free survival (PFS). Both TTP and PFS were the same in the subjects in whom the MDSCs were analyzed. Changes in PMN-MDSC on day 14 were not associated with PFS. However, on day 21, changes in PMN-MDSC demonstrated a weak but significant inverse correlation with the time to progression. This correlation became stronger on days 28 and day 42 (Table 2). These results indicate that a decrease in MDSC during DS-8273a treatment was associated with longer time to progression in these patients. As described above, the populations of PMN-MDSC increased in some patients on day 28 of the treatment sowe asked whether those changes were associated with clinical outcome. We calculated the time to progression for patients who retained control levels of PMN-MDSC by day 28 as well as for those who had elevated levels of these cells, and patients who retained control levels of MDSC had a significantly longer time to progression than the patients who had elevated levels of PMN-MDSC (Fig. 4E).
Table 2
Correlation between changes in PMN-MDSC and time to progression
Day 14 | Day 21 | Day 28 | Day 42 | |
---|---|---|---|---|
Spearman R | −0.05 | −0.51 | −0.64 | −0.98 |
P Two-Tailed | 0.76 | 0.077 | 0.024 | <0.0001 |
P One-Tailed | 0.039 | |||
Number of Pairs | 11 | 11 | 11 | 7 |
Discussion
We report here the first successful attempt to selectively eliminate MDSC in cancer patients. This study is based on previous observations that MDSC in mice and cancer patients are more sensitive to TRAIL-R2 agonistic antibody or TRAIL than their normal counterparts (15). In mice, this effect was mediated by upregulation of TRAIL-R2 whereas in cancer patients it was mediated mostly by a decrease in DcR2. Targeting of TRAIL-R in mice caused depletion of MDSC and substantially improved the antitumor effect of a CTLA4 blocking antibody (15). These results suggested that targeting TRAIL-R2 could be potentially useful in targeting MDSC in humans. The use of TRAIL-R2 agonist antibodies in cancer patients has been previously reported and demonstrated a very good safety profile (23, 24). However, the antitumor activity of this antibody as monotherapy was limited (25). Tigatuzumab in combination with gemcitabine led to 8 partial responses in pancreatic cancer patients (n=61) and demonstrated no anti-cancer activity in lung cancer patients (n=91), both being phase II clinical trials (26, 27). Another TRAIL-R2 targeting antibody, Conatumab, was also tested in a randomized phase II clinical trial in combination with chemotherapy and did not demonstrate objective clinical response in patients with colorectal, pancreatic, soft tissue sarcoma or lung cancer (28–31). Heterogeneous expression of the DR5 receptor on tumor cells, poor penetration of the antibody to solid tumors and/or the relatively low affinity of the antibody could explain the low clinical efficacy of the treatment. We suggested that TRAIL-R2 antibody could have an effect independent of its ability to directly target solid tumors, by eliminating MDSC in patients with high levels of these cells suppressing host antitumor immunity.
We observed increases in PMN-MDSC and eMDSC in 9 out of 16 patients (56%). This is somewhat lower than we and others have previously reported (32, 33). Overnight shipment of samples before analysis could contribute to this phenomenon. However, we believe that shipment probably played a relatively minor role due to the fact that control samples were also stored overnight and that in previous studies shipment of samples did not have a significant effect on the function of MDSC. Other more likely explanation is that the patients in this trial were heavily pre-treated with chemotherapy prior to enrollment to the study in addition to being heterogeneous in their tumor types. This may also explain the low number of CD4+ cells, B cells, and NK cells in these patients. Increase of M-MDSC in only two patients was not surprising since M-MDSC are largely increased in patients with melanoma, multiple myeloma and to some extent prostate cancer, which were very poorly represented in this trial.
The main finding of this study is that DS-8273a caused rapid elimination of MDSC in patients with elevated levels of these cells at baseline without affecting any other cell populations. It also did not affect MDSC in patients with control levels of the cells. These results describe the first example of highly selective elimination of MDSC in patients. The effect was observed until the end of the first cycle (day 21) and was associated with a decrease in DcR2 expression. However, the second cycle of treatment failed to prolong this effect in all patients. A number of patients had elevated number of PMN-MDSC by day 28 (day 7 of cycle 2) and was associated with an increase in DcR2 expression. Elevated levels of PMN-MDSC at day 28 was associated with a shorter time to disease progression. Overall, changes in PMN-MDSC and eMDSC inversely correlated with clinical outcome supporting the role of these cells in regulation of tumor progression. No direct conclusions could be drawn from these results because of heterogeneous patient population and relatively small number of patients; however, it suggests further that a more detailed study is warranted.
Why did DS-8273a treatment fail to control MDSC levels longer? There are two possible explanations of this phenomenon. Treatment with DS-8273a can cause a compensatory increase in DcR2 expression which could prevent further action of the antibody. However, this explanation is less likely due to the fact that relative up-regulation of DcR2 in MDSC by day 28 just returned it to pre-treatment level. That level did not prevent effective elimination of these cells during first cycle of treatment. The most likely explanation is that in the patients with highly advanced cancer elimination of MDSC by itself was not sufficient to control tumor progression. This phenomenon was previously demonstrated in numerous studies in mice (5). Rapid tumor progression that was observed in the patients on this trial might have resulted in increased production of MDSC which the antibody was unable to control. The fact that patients with control levels of MDSC on day 28 had significantly longer time to progression than patient with elevated levels of MDSC support this explanation.
The presented data demonstrate that DS-8273a maintained selective depletion of MDSC for at least 28 days, which may provide a sufficient window of therapeutic activity in combination with immunotherapies using adoptive T cells transfer, or PD-1 antibody. This data provided the first demonstration of selective elimination of MDSC in patients with advanced cancers that was associated with prolonged time to progression. Our observations open an opportunity for the clinical combination of TRAIL-R2 targeting antibody with various immunotherapeutic strategies.
References
Full text links
Read article at publisher's site: https://doi.org/10.1158/1078-0432.ccr-16-1784
Read article for free, from open access legal sources, via Unpaywall:
https://clincancerres.aacrjournals.org/content/clincanres/23/12/2942.full.pdf
Citations & impact
Impact metrics
Article citations
Targeting Death Receptor 5 (DR5) for the imaging and treatment of primary bone and soft tissue tumors: an update of the literature.
Front Mol Biosci, 11:1384795, 02 Sep 2024
Cited by: 0 articles | PMID: 39286782 | PMCID: PMC11402684
Review Free full text in Europe PMC
Reshaping the tumor immune microenvironment to improve CAR-T cell-based cancer immunotherapy.
Mol Cancer, 23(1):175, 26 Aug 2024
Cited by: 0 articles | PMID: 39187850 | PMCID: PMC11346058
Review Free full text in Europe PMC
Myeloid‑derived suppressor cells: Key immunosuppressive regulators and therapeutic targets in colorectal cancer (Review).
Int J Oncol, 65(3):85, 26 Jul 2024
Cited by: 0 articles | PMID: 39054950 | PMCID: PMC11299769
Review Free full text in Europe PMC
Impact of obesity‑associated myeloid‑derived suppressor cells on cancer risk and progression (Review).
Int J Oncol, 65(2):79, 28 Jun 2024
Cited by: 1 article | PMID: 38940351 | PMCID: PMC11251741
Review Free full text in Europe PMC
Mechanisms of myeloid-derived suppressor cell-mediated immunosuppression in colorectal cancer and related therapies.
World J Gastrointest Oncol, 16(5):1690-1704, 01 May 2024
Cited by: 0 articles | PMID: 38764816 | PMCID: PMC11099432
Review Free full text in Europe PMC
Go to all (109) article citations
Data
Data behind the article
This data has been text mined from the article, or deposited into data resources.
BioStudies: supplemental material and supporting data
Clinical Trials
- (2 citations) ClinicalTrials.gov - NCT02076451
Similar Articles
To arrive at the top five similar articles we use a word-weighted algorithm to compare words from the Title and Abstract of each citation.
First-in-human study of the antibody DR5 agonist DS-8273a in patients with advanced solid tumors.
Invest New Drugs, 35(3):298-306, 03 Jan 2017
Cited by: 18 articles | PMID: 28050790
Hepatic carcinoma-associated fibroblasts enhance immune suppression by facilitating the generation of myeloid-derived suppressor cells.
Oncogene, 36(8):1090-1101, 05 Sep 2016
Cited by: 107 articles | PMID: 27593937
ER stress regulates myeloid-derived suppressor cell fate through TRAIL-R-mediated apoptosis.
J Clin Invest, 124(6):2626-2639, 01 May 2014
Cited by: 232 articles | PMID: 24789911 | PMCID: PMC4038578
Myeloid-Derived Suppressor Cells.
Cancer Immunol Res, 5(1):3-8, 01 Jan 2017
Cited by: 980 articles | PMID: 28052991 | PMCID: PMC5426480
Review Free full text in Europe PMC
Funding
Funders who supported this work.
Daiichi Sankyo NIH (1)
Grant ID: CA084488
NCI NIH HHS (3)
Grant ID: R01 CA100062
Grant ID: P30 CA010815
Grant ID: R01 CA084488
NIH HHS (1)
Grant ID: S10 OD017998