Abstract
Free full text

The srs2 suppressor of UV sensitivity acts specifically on the RAD5- and MMS2-dependent branch of the RAD6 pathway
Abstract
The SRS2 gene encodes a helicase that affects recombination, gene conversion and DNA damage repair in the yeast Saccharomyces cerevisiae. Loss-of-function mutations in srs2 suppress the extreme sensitivity towards UV radiation of rad6 and rad18 mutants, both of which are impaired in post-replication DNA repair and damage-induced mutagenesis. A sub-branch within the RAD6 pathway is mediated by RAD5, UBC13 and MMS2, and a comprehensive analysis of the srs2 effect on other known members of the RAD6 pathway reported here now demonstrates that suppression by srs2 is specific for mutants within this RAD5-dependent sub-system. Further evidence for the concerted action of RAD5 with UBC13 and MMS2 in DNA damage repair is given by examination of the effects of cell cycle stage as well as deletion of other repair systems on the activity of post-replication repair. Finally, it is shown that MMS2, like UBC13 and many other repair genes, is transcriptionally up-regulated in response to DNA damage. The data presented here support the notion that RAD5, UBC13 and MMS2 encode an ensemble of genetically and physically interacting repair factors within the RAD6 pathway that is coordinately affected by SRS2.
INTRODUCTION
Mechanisms of DNA damage repair are highly conserved from bacteria to higher eukaryotes. The yeast Saccharomyces cerevisiae maintains several repair systems that are each responsible for specific types of damage (1,2). The RAD3 group mediates nucleotide excision repair of pyrimidine dimers and other bulky lesions, the RAD52 group encodes the factors for double-strand break repair via homologous recombination and the RAD6 pathway of post-replication repair (PRR) is believed to act upon single-stranded gaps that arise during DNA replication on damaged templates (3). While nucleotide excision repair and homologous recombination are mechanistically quite well understood, little is known about the molecular details of PRR. Extensive genetic analysis, however, has revealed an intricate system of several independent sub-branches. Notably, two fundamentally different modes of repair can be distinguished within the RAD6-dependent repair system: while error-prone repair involves translesion synthesis across a damaged site, error-free repair has been proposed to use information from the undamaged sister chromatid for correct reversal of the damage (3).
RAD6, which is epistatic to all other members of this pathway, encodes a ubiquitin-conjugating enzyme (UBC) whose catalytic activity is essential for function in DNA repair (4,5). Mutations in this gene confer a pleiotropic phenotype, involving a high degree of sensitivity towards DNA-damaging agents, a defect in damage-induced mutagenesis as well as an increased rate of spontaneous mutagenesis, but also defects apparently unrelated to DNA repair, including a reduced growth rate, temperature sensitivity and the inability to sporulate (reviewed in 6). Other members of the RAD6 pathway exhibit different subsets of the repair phenotypes, with varying degrees of UV sensitivity and variable effects on mutagenesis and recombination. Rad18p, a DNA-binding RING finger protein that forms a stable complex with Rad6p and is believed to target the UBC to sites of DNA damage (7), is apparently involved in all RAD6-dependent sub-pathways of DNA repair (8,9). The error-prone system is characterized by a specific damage-tolerant polymerase, Polζ, encoded by REV3 and REV7, which is capable of translesion synthesis (10,11). Another RAD6/RAD18-dependent translesion polymerase is Polη, encoded by RAD30 (12–14). The RAD5 gene acts independently of both sub-pathways, but again depends on RAD6 and RAD18 (15–17). Mutants of RAD5 exhibit a reduced rate of damage-induced mutagenesis in some assays, but since this effect is limited to specific types of mutations, RAD5-dependent repair has also been classified as error free (15–18). RAD5 encodes a DNA-dependent ATPase (19) with homology to the SNF2/SWI2 family of helicases and chromatin remodeling factors (20). Recently, it was shown that there appears to be a second error-free pathway in addition to that mediated by RAD5: a mutation in the POL30 gene encoding the polymerase processivity factor PCNA, pol30-46, which had previously been assigned to the error-free RAD6-dependent sub-system (21), was found to act additively or even synergistically with respect to a rad5 mutation, suggesting their involvement in alternative pathways (22).
A second ubiquitin-conjugating activity has been found associated with the RAD6 pathway: MMS2, encoding a UBC-like protein, was cloned by complementation of a mutant sensitive to the alkylating agent methylmethane sulfonate (MMS) and was assigned to an error-free sub-branch (23). The Mms2p protein was later shown to form a heterodimer with Ubc13p, a genuine UBC (24). This complex assembles multi-ubiquitin chains that are linked in a non-standard way, via Lys63 (K63) of ubiquitin instead of the prevalent Lys48 linkage. In fact, mutants of ubiquitin in which K63 is replaced by arginine (ubiK63R) display a UV sensitivity identical to that of mms2 and ubc13 mutants, indicating that these non-standard multi-ubiquitin chains are relevant to PRR (24,25). A dependence of UBC13 and MMS2 on RAD5 has been established (26), which is supported by the observation that Rad5p protein physically interacts with Ubc13p and recruits the Ubc13p/Mms2p dimer to chromatin, in a manner similar to the recruitment of Rad6p by Rad18p (26).
The SRS2 gene [independently identified as HPR5 (27) and RADH (28)] represents an unusual member of the RAD6 pathway: mutation or deletion of this gene was found to partially suppress the UV sensitivities of rad6 and rad18 mutants (28–30). Suppression depends on a functional recombination pathway (31). SRS2 encodes a helicase with 5′→3′ polarity (32) and is involved in a variety of processes associated with DNA metabolism, such as recombination and gene conversion, as well as double-strand break repair by single-strand annealing and non-homologous end-joining (33–37). It remains to be elucidated how SRS2 acts in PRR. In order to define the relation of SRS2 to the various sub-systems of PRR I have analyzed the effect of an srs2 deletion on the UV sensitivities of a series of RAD6 pathway mutants. I present evidence that the suppression effect of srs2 is specific for the branch mediated by RAD5, UBC13 and MMS2. Further support for a concerted action of these factors within the RAD6 pathway is given by genetic analyses that place MMS2 and UBC13 in the RAD5-dependent repair system under several different experimental conditions. Finally, I present evidence that MMS2, like UBC13 and most other members of the RAD6 pathway, is a damage-inducible gene.
MATERIALS AND METHODS
Yeast strains and plasmids
Standard protocols were followed for the preparation of yeast media, transformation, sporulation and tetrad dissection (38,39). With the exception of UBIK63 and ubiK63R, which correspond to SUB280 and SUB413 and were kindly provided by D. Finley (25), all strains are derivatives of the diploid wild-type (wt) strain DF5 (40) and its isogenic haploid (his3-Δ200, leu2-3,112, lys2-801, trp1-1, ura3-52). Construction of rad18, rad5, rad30, ubc13 and mms2 deletions has been reported previously (26). The construct used to create a rev3 deletion strain is based on the hisG-URA3-hisG geneblaster and was a gift from W. Kramer, a rad2::URA3 construct was provided by K. Madura and a rad52::URA3 plasmid, pSM22, by D. Schild. The pol30-46 mutation was introduced into the DF5 background by deletion of one POL30 allele in diploid cells, introduction of pBL230-46, a centromeric plasmid bearing the pol30-46 allele (21), and sporulation of the transformants. Plasmid pBL230, encoding the unmutated POL30 gene, served for construction of an isogenic control strain. pBL230 and pBL230-46 as well as the pol30::URA3 deletion construct, pBL243, were kindly provided by P. Burgers. The srs2 strain was constructed by exact replacement of the open reading frame with a HisMX cassette, using a PCR strategy (41). Double and triple mutants with srs2 were constructed from the corresponding single mutants by mating and tetrad dissection, with the exception of UBIK63, ubiK63R, POL30 and pol30-46, into which the srs2 deletion was newly introduced using the same PCR strategy. A KanMX cassette (41) served for replacement of the open reading frame in these cases. Expression vectors for the promoter–lacZ fusions are derivatives of YIplac211 (42) carrying the lacZ open reading frame as a BamHI–HindIII fragment and the transcription terminator from pGBT9 (Clontech). The promoter regions of RAD6 and MMS2 were inserted as PCR products (425 and 511 bp upstream of the start codon, respectively).
Determination of UV sensitivities
Unless otherwise noted, cells were grown in liquid YPD medium at 28°C to exponential phase by inoculation from a fresh overnight culture and further incubation with shaking for 4–6 h. For cell cycle experiments, stationary cells were obtained from cultures incubated for 4 days in YPD medium at 28°C. For S phase arrest, exponential cultures were treated with 100 mM hydroxyurea for 4 h, and G2/M-arrested cells were obtained by incubation with 15 µg/ml nocodazole for 4 h. Arrests were confirmed by microscopic inspection of cell morphology. UV sensitivities were determined according to the two protocols outlined below.
Gradient assay
Suspensions of equal cell densities (~107 cells/ml) were applied to a YPD plate in parallel lines by placing a drop of each culture (12 µl for a round plate of 80 mm diameter, 20 µl for a square plate of 120 × 120 mm) at one end and letting it run across the surface by tilting the Petri dish. The streaks were then irradiated with a gradient of UV dosage by gradually uncovering them from one end to the other during the specified time interval. The UV source was a hand-held lamp (254 nm) fixed at a distance corresponding to a dosage of 0.83 J/(m2·s). Plates were incubated in the dark and examined after 2–3 days.
Survival curves
Survival after UV irradiation was determined by spreading out appropriate dilutions of the respective cultures onto YPD plates and irradiation with specified dosages of UV light. Plates were incubated in the dark and colonies were counted after 3 days. The UV source was a hand-held lamp (254 nm) fixed at a distance corresponding to a dosage of either 0.83 or 1.67 J/(m2·s). All assays were performed two to six times. Standard deviations are shown where they exceed 30% of the respective values.
Northern blots
For UV induction assays wt cells grown to logarithmic phase in YPD were washed, resuspended in sterile water at ~2 × 108 cells/ml and irradiated in suspension under constant stirring with a dosage corresponding to ~50% survival (determined by plating a sample before and after irradiation). Cells were harvested, resuspended in YPD prewarmed to 28°C and incubated in the dark. For MMS induction studies MMS was added to a logarithmic culture in YPD to 0.1% (v/v) and incubation was continued for the specified time. Samples of ~5 × 108 cells were harvested at the indicated time points and total RNA was isolated by hot phenol extraction (43). Samples of 15 µg RNA each were glyoxylated and separated on a 1% (w/v) agarose gel. Transfer onto GeneScreen Plus membranes (Amersham) and hybridizations were performed as described (38). PCR products of the entire open reading frames excluding the introns were used as probes for the MMS2 and UBC13 genes and a 500 bp ClaI restriction fragment from a plasmid carrying ACT1 served as probe for the ACT1 gene. Bands were visualized using a Fujix BAS1000 Phosphorimager and quantified with MacBas 2.0 software. Images were edited for better visibility without affecting relative signal strengths.
Measurements of β-galactosidase activities
Yeast cells harboring the promoter–lacZ fusion constructs integrated into the ura3 locus were grown in YPD to logarithmic phase and irradiated with UV light in suspension or treated with 0.1% (v/v) MMS as described above. Samples of 5 × 108 cells were harvested at the indicated times and total protein extracts were prepared by disruption with glass beads in buffer Z (38), followed by low speed centrifugation. β-Galactosidase assays were performed with o-nitrophenyl galactoside as described (38) and specific activities (Miller units) were calculated based on total protein content. All assays were performed in triplicate.
RESULTS
Epistasis of RAD5 to MMS2 and UBC13 is observed throughout the cell cycle
Our previous data indicate that MMS2 and UBC13 are both hypostatic to RAD5 but not to RAD30 with respect to UV sensitivity (26). In contrast, an independent study found additivity between the UV sensitivities of the mms2 and rad5 mutations, while no data were shown for ubc13 (22). In order to shed light on this issue the relationship between RAD5 and MMS2 as well as UBC13 was re-examined under a number of different experimental conditions. A possible dependence on the cell cycle was examined by irradiation of cells arrested in different stages of the cell cycle and subsequent determination of survival. Two different methods were applied for optimal detection of slight differences in UV sensitivities: overall sensitivities were compared qualitatively by applying a gradient of UV dosage to cells streaked in parallel lines across a Petri dish; in addition, exact survival rates were determined by irradiation with specified dosages and colony counting (see Materials and Methods). Figure Figure1A1A shows the survival of rad5 ubc13 and rad5 mms2 in comparison to the wt and single mutants after irradiation of stationary (G0), exponential, hydroxyurea-arrested (S) and nocodazole-treated (G2/M) cells. The wt cells are significantly more sensitive when irradiated in G0 than in S or G2 phase. This situation is reversed in rad5 mutants, confirming recent observations by Friedl et al. (33). Since cells arrested in G1 with α factor show the same sensitivity as stationary cells (data not shown), this variation must be due to cell cycle stage and not to metabolic differences. Nevertheless, within each stage of the cell cycle the sensitivities of rad5, rad5 ubc13 and rad5 mms2 cells were indistinguishable (Fig. (Fig.1A1A and B). As it is conceivable that inactivation of other repair pathways might exacerbate any additional defects of the rad5 ubc13 and rad5 mms2 double mutants, UV sensitivities were also re-examined in rad2 and rad52 backgrounds, defective in nucleotide excision repair and homologous recombination, respectively. Again, identical sensitivities were found for rad5, rad5 ubc13 and rad5 mms2 cells (Fig. (Fig.1C1C and D). These experiments confirm that in the strain background used for this study the function of UBC13 and MMS2 is fully dependent on the presence of RAD5 and thus support our previous assignment of UBC13 and MMS2 to the RAD5-dependent branch of the RAD6 pathway (26).
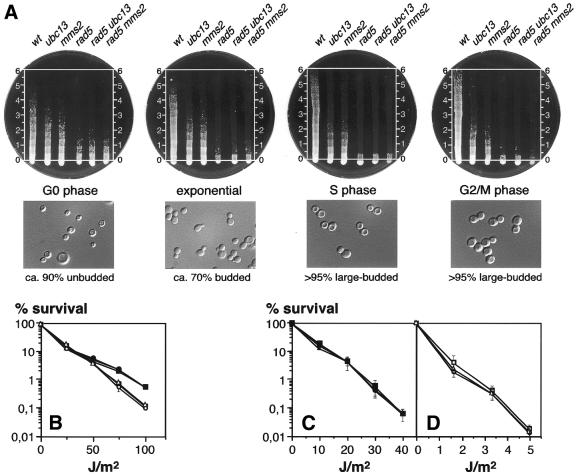
Comparison of the UV sensitivities of rad5, rad5 ubc13 and rad5 mms2 in different stages of the cell cycle. G0 cells were derived from stationary cultures, S phase cells by hydroxyurea treatment and G2/M phase cells by nocodazole treatment. (A) Double mutants were compared to wt and single mutants using the qualitative gradient assay as described in Materials and Methods. Cell morphologies were examined microscopically. Irradiation times (min) are indicated on each plate. (B) Survival after irradiation with specified dosages was determined in G0 (filled symbols) and in S phase (open symbols) for rad5 (squares), rad5 ubc13 (circles) and rad5 mms2 (triangles). (C) Survival in a recombination-deficient background of rad52 rad5 (squares), rad52 rad5 ubc13 (circles) and rad52 rad5 mms2 (triangles). (D) Survival in an excision repair-deficient background of rad2 rad5 (squares), rad2 rad5 ubc13 (circles) and rad2 rad5 mms2 (triangles). Each curve represents the average of two to six independent experiments. Standard deviations are given where they exceed 30% of the respective values.
srs2 acts as a suppressor specific for the RAD5/UBC13/MMS2-dependent branch of the RAD6 pathway
In order to define the position of SRS2 with respect to the different sub-branches of the PRR system, the effect of an srs2 deletion on the UV sensitivities of other RAD6 pathway mutants, rad5, ubc13, mms2, rad30, pol30-46 and rev3, was examined. Since many of the single mutants are only slightly more UV-sensitive than srs2 itself, again the qualitative gradient assay and the colony assay were both applied for optimal detection of a possible suppression effect. Figure Figure2A2A shows that the UV sensitivities of rad5, ubc13 and mms2 are all suppressed by the srs2 deletion. Consequently, all three double mutants, rad5 srs2, ubc13 srs2 and mms2 srs2, display low UV sensitivities very similar to the srs2 single mutant. In contrast, the rad30 srs2 and pol30-46 srs2 double mutants are significantly more UV-sensitive than each of the corresponding single mutants. In the case of rev3, the effect of srs2 is less clear, since the UV sensitivity of the rev3 deletion is even weaker than that of srs2, precluding the identification of a visible suppression effect.

Suppression of UV sensitivity by srs2 within a sub-set of the RAD6 pathway. (A) Gradient assays were performed as described in Materials and Methods. Irradiation times (min) are indicated on each plate. (B–H) Survival after UV irradiation was determined as described in Figure 1 for the wt (filled squares) and an srs2 (open squares) deletion mutant and compared to a series of single mutants in other RAD6 pathway genes (filled circles) as well as the combination of those mutants with srs2 (open circles): (B) rad5; (C) ubc13; (D) mms2; (E) ubiK63R; (F) rad30; (G) pol30-46; (H) rev3. Strains pol30-46 and ubiK63R (SUB413) are plotted in comparison to their respective isogenic wt strains, POL30 and UBIK63 (SUB280), respectively.
Quantitative survival curves for the individual strains are shown in Figure Figure2B–H.2B–H. Again, the srs2 suppression effect on rad5, ubc13 and mms2 is evident (Fig. (Fig.2B–D),2B–D), while additive effects are observed with the rad30 and pol30-46 mutants (Fig. 2F and G). Suppression of the rad5 mutation is in good agreement with recent data (33). Moreover, those double mutants in which suppression is active also display the characteristic curve shape of the srs2 single mutant itself. This S-shaped curve has been observed previously with exponential srs2 cells (28) and is due to a sub-population of highly UV-sensitive cells within the culture that likely corresponds to G1 cells, which are much more sensitive to UV irradiation than G2 cells. Thus, the srs2 mutation behaves epistatic to rad5, mms2 and ubc13 in this respect. Again, the effect of srs2 on the rev3 mutant is not easily interpretable (Fig. (Fig.2H),2H), but the absence of additivity suggests an epistatic relation, which is also supported by the srs2-like shape of the survival curve of the rev3 srs2 double mutant. In conclusion, the srs2 suppressor acts in a manner specific for the RAD5/UBC13/MMS2-dependent branch of the RAD6 pathway.
srs2 acts a suppressor of the ubiK63R mutation
Due to the modest UV sensitivities of ubc13 and mms2 mutants the extent of suppression by srs2 is relatively weak compared to the effect on rad5. In order to confirm that the observed phenomenon is specific for the error-free repair branch, the consequences of an srs2 deletion were examined in the ubiK63R strain, in which all the repair proteins are present and the only modification is a point mutation in the ubiquitin gene (25). As K63 of ubiquitin serves as a substrate for ubiquitin chain polymerization by the Ubc13p–Mms2p dimer (24), the corresponding mutant should display a phenotype identical to the mms2 or ubc13 deletion strains. When compared to the isogenic wt strain, suppression of UV sensitivity by srs2 was indeed observed (Fig. (Fig.2A2A and E).
Suppression by srs2 depends on a functional recombination system
The suppression effect of srs2 is dependent on functional homologous recombination, presumably because of a channeling of lesions into recombinational repair in the absence of SRS2 (31). Thus, if the srs2 effects on ubc13, mms2 and rad5 are functionally related, suppression should be abolished in a rad52 background. Figure Figure33 shows that this is the case: deletion of SRS2 in the rad52 ubc13, rad52 mms2 and rad52 rad5 double mutants did not result in any change in UV sensitivities. The same situation was observed with the rad52 rad18 double mutant. Likewise, no suppression of ubc13 or mms2 by srs2 was observed in stationary phase cells, which predominantly exist with an unreplicated genome (in G0) and should thus be incapable of repair by recombination between sister chromatids (data not shown). In conclusion, the relationship between SRS2 and the members of the RAD5/UBC13/MMS2-dependent branch of PRR is comparable to that between SRS2 and RAD18 or RAD6.

Consequences of defective homologous recombination for suppression of UV sensitivity by srs2. (A) Gradient assay. (B) Survival after UV irradiation for rad52 (filled squares), rad52 srs2 (open squares), rad52 ubc13 (filled circles), rad52 ubc13 srs2 (open circles), rad52 mms2 (filled triangles) and rad52 mms2 srs2 (open triangles). (C) Survival after UV irradiation for rad52 rad18 (filled circles), rad52 rad18 srs2 (open circles), rad52 rad5 (filled diamonds) and rad52 rad5 srs2 (open diamonds).
MMS2 is a damage-inducible gene like UBC13
Most of the genes involved in post-replication DNA repair have been shown to be inducible by DNA damage, such as RAD6 itself (44), RAD18 (45), RAD30 (12), RAD5 (46) and UBC13 (47,48). Considering the close cooperation between Mms2p and the other members of the RAD6 pathway in DNA repair, the MMS2 gene should be expected to be regulated in a similar fashion. I therefore investigated the effect of UV irradiation as well as MMS treatment on transcription of the MMS2 gene by northern analysis. Probes specific for the ACT1 and UBC13 transcripts served as controls for a constitutively expressed and a damage-inducible gene, respectively. Figure Figure4A4A shows that the MMS2 mRNA levels increased by a factor of 3 after UV irradiation, while treatment with 0.1% MMS afforded a 6-fold induction. These values are comparable to the roughly 5-fold induction of the UBC13 transcript (Fig. (Fig.4A;4A; 47,48). Interestingly, however, the induction of MMS2 by MMS followed significantly slower kinetics than that of UBC13, with maximal induction after 2 h compared to <1 h for UBC13. This observation may explain why MMS2 had not been identified as MMS-inducible in two previous studies, in which analysis was limited to 60 or 30 min after treatment, respectively (47,48). In order to confirm the damage-inducibility of the MMS2 gene by an alternative method, its promoter region was fused to the coding sequence of the lacZ gene and the resulting construct was integrated into the ura3 locus. For comparison, the corresponding fusion with the RAD6 promoter was constructed likewise. Assays for β-galactosidase activity show that both promoters are functional and inducible by UV irradiation as well as MMS treatment in this context, and the relative induction of the MMS2 promoter as measured by β-galactosidase activity corresponds well to the values obtained by northern analysis (Fig. (Fig.44B).
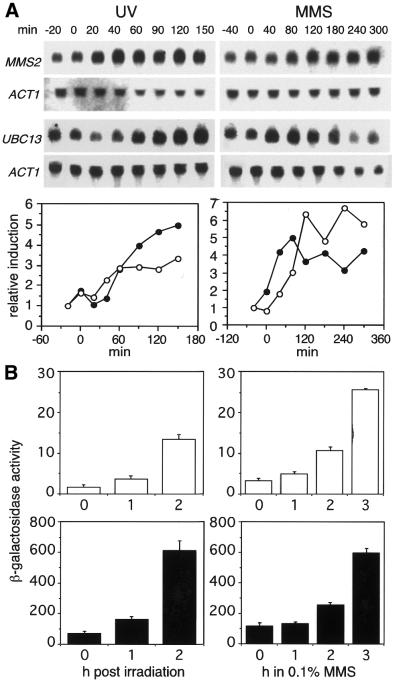
Induction of the MMS2 and UBC13 transcripts by DNA damage. (A) Northern blots were analyzed with probes specific for UBC13 or MMS2 as indicated, stripped and re-hybridized with a probe specific for the ACT1 gene. Numbers above each lane denote the time of sampling (min) relative to irradiation (left) or addition of 0.1% MMS to the cultures (right), respectively. Induction levels for UBC13 (filled circles) and MMS2 (open circles) were calculated relative to the respective ACT1 signals. (B) Activities of β-galactosidase (Miller units based on total protein concentration) in cell extracts prepared after UV irradiation or 0.1% MMS treatment, respectively, of strains bearing a reporter construct of the lacZ gene fused to the promoter of MMS2 (open bars) or RAD6 (solid bars), respectively. Values represent the average of three independent experiments.
DISCUSSION
The relationship between RAD5 and UBC13/MMS2
Rad5p protein physically interacts with Ubc13p via its RING finger domain and in this manner recruits the Ubc13p–Mms2p dimer to chromatin for DNA repair (26). While at this time it is unknown whether Rad5p acts solely as a recruitment factor for the UBC, as a substrate for ubiquitinylation or even as a ubiquitin ligase in cooperation with Ubc13p–Mms2p, the biochemical data are consistent with the observation that the activity of UBC13 and MMS2 within the RAD6 pathway is fully dependent on the presence of the RAD5 gene (26). Since this relationship has important consequences for the organization of the PRR system, its genetic analysis has been expanded here to account for variations in repair capacities during different stages of the cell cycle. While duplication of the genome confers additional UV resistance to wt cells, presumably due to their ability to repair DNA damage by homologous recombination, rad5 mutants are further sensitized during S and G2 phase compared to G1 (Fig. (Fig.1;1; 33). Mutants of ubc13 and mms2 exhibit less dependence on the cell cycle, although as with rad5 the difference from the wt is more pronounced in S and G2 than in G1 (Fig. (Fig.1A). 1A). Nevertheless, the presence or absence of UBC13 and MMS2 was found to have no influence on UV sensitivity in a rad5 background in any stage of the cell cycle, thus further demonstrating their dependence on RAD5 for activity in DNA repair. Similarly, inactivation of other repair systems that might otherwise obscure the importance of the PRR pathway for survival had no effect on the epistatic relationship of RAD5 to UBC13 and MMS2 either (Fig. (Fig.1C1C and D). In summary, the available data all support an assignment of UBC13 and MMS2 to the RAD5-dependent sub-branch of the RAD6 pathway.
The role of SRS2 in post-replication DNA repair
While deletion of SRS2 alone leads to relatively mild defects in haploid cells, combination of srs2 with mutations in the helicase gene SGS1 or one of several recombination factors results in extremely poor growth or even synthetic lethality, which is, however, suppressible by mutations in other recombination or checkpoint factors (35,49–52). Based on these observations it has been proposed that the absence of SRS2 and SGS1 leads to unrestrained recombination and ultimately cell death due to chromosome loss or permanent checkpoint activation, which can only be avoided by preventing the initiation of recombination (53,54).
In PRR SRS2 has been suggested to act upstream of the RAD6-dependent repair machinery. According to this model SRS2 would exert an anti-recombinogenic effect and suppression by srs2 would result from a channeling of lesions into recombinational repair in the absence of PRR (30,31,46). Here I have shown that various members of the RAD6-dependent repair system are affected differentially by deletion of the srs2 gene. While the UV sensitivities of rad5, ubc13 and mms2 mutants are fully suppressed down to the level of the srs2 single mutant itself and suppression depends on functional homologous recombination, additive effects are observed in the case of rad30 and pol30-46 and an epistatic relationship is suggested with rev3 (Fig. (Fig.2).2). These results imply that the action of SRS2 is directed specifically towards the subset of the RAD6 pathway that comprises the branch described above, mediated by RAD5, UBC13 and MMS2. Consistent with this notion is the observation that in contrast to rad5, the UV sensitivities of rad6 and rad18 cells are not fully suppressed by the srs2 mutation, but the double mutants are still significantly more sensitive than the srs2 single mutant (29,31; unpublished results). Hence, the RAD30- and POL30-dependent branches of the RAD6 pathway, which would be crippled by deletion of RAD6 or RAD18, but are not subject to suppression by srs2, are likely responsible for this enhanced sensitivity of rad6 srs2 and rad18 srs2. Based upon the biochemical data that suggest a heteromeric complex in which the Ubc13p–Mms2p UBC is recruited to the Rad6p–Rad18p dimer by means of Rad5p, it is attractive to hypothesize that this complex may actually be the target of Srs2p action in error-free PRR.
The interaction of SRS2 with the REV3-dependent, error-prone branch of the RAD6 pathway is less readily interpretable. Sensitivity of the rev3 mutant is neither suppressed nor significantly enhanced by deletion of srs2. A similar relationship was recently observed between srs2 and a mutant of POL32, which encodes a subunit of polymerase δ and was classified as another member of the error-prone system (55). Likewise, srs2 does not suppress the deficiency of rev3 with respect to induced mutagenesis (29). In fact, the srs2 mutation alone was found to exhibit a significant depression of UV-induced forward mutagenesis, implying that the presence of Srs2p is required for mutagenic translesion synthesis (28). Moreover, a physical interaction between Srs2p and Pol32p was detected in the two-hybrid system (55). Thus, an influence of SRS2 on error-prone repair is apparent.
Based on the strong synergistic effect between mms2 and rev3 with respect to UV-induced killing it has been proposed that Mms2p and Rev3p compete for a common DNA substrate (56). According to the model of SRS2 action in PRR outlined above, the Srs2p helicase would thus be involved in creating these substrates, thereby preventing repair by homologous recombination and allowing either mutagenic translesion synthesis or MMS2-mediated error-free repair. Srs2p may thus be an important factor contributing to the decision between error-free and error-prone PRR. How this decision is reached, however, remains to be determined. Elucidating the events at the sites of damage on molecular terms, particularly establishing the order in which the individual repair factors are recruited there, will undoubtedly provide valuable insight into the complicated mechanism of the RAD6-dependent repair system.
ACKNOWLEDGEMENTS
I would like to thank Regine Kahmann for generous support. Anna Friedl is acknowledged for communicating unpublished data. I also thank Peter Burgers, Dan Finley, Michael Knop, Wilfried Kramer, Kiran Madura and David Schild for plasmids, Sabine Thalmeir for construction of the srs2 deletion strain and Stefan Jentsch, in whose laboratory part of the work was initiated. This work was supported by a grant from the Deutsche Forschungsgemeinschaft.
References
Articles from Nucleic Acids Research are provided here courtesy of Oxford University Press
Full text links
Read article at publisher's site: https://doi.org/10.1093/nar/29.17.3487
Read article for free, from open access legal sources, via Unpaywall:
https://academic.oup.com/nar/article-pdf/29/17/3487/9906038/293487.pdf
Citations & impact
Impact metrics
Citations of article over time
Article citations
PCNA Unloading Is Crucial for the Bypass of DNA Lesions Using Homologous Recombination.
Int J Mol Sci, 25(6):3359, 15 Mar 2024
Cited by: 1 article | PMID: 38542333 | PMCID: PMC10970473
Genetic Dissection of Budding Yeast PCNA Mutations Responsible for the Regulated Recruitment of Srs2 Helicase.
mBio, 14(2):e0031523, 02 Mar 2023
Cited by: 2 articles | PMID: 36861970 | PMCID: PMC10127746
PCNA Loaders and Unloaders-One Ring That Rules Them All.
Genes (Basel), 12(11):1812, 18 Nov 2021
Cited by: 19 articles | PMID: 34828416 | PMCID: PMC8618651
Review Free full text in Europe PMC
The Mgs1/WRNIP1 ATPase is required to prevent a recombination salvage pathway at damaged replication forks.
Sci Adv, 6(15):eaaz3327, 08 Apr 2020
Cited by: 9 articles | PMID: 32285001 | PMCID: PMC7141828
Multifunctional roles of Saccharomyces cerevisiae Srs2 protein in replication, recombination and repair.
FEMS Yeast Res, 17(2), 01 Mar 2017
Cited by: 32 articles | PMID: 28011904 | PMCID: PMC5399913
Review Free full text in Europe PMC
Go to all (41) article citations
Data
Similar Articles
To arrive at the top five similar articles we use a word-weighted algorithm to compare words from the Title and Abstract of each citation.
Suppression of genetic defects within the RAD6 pathway by srs2 is specific for error-free post-replication repair but not for damage-induced mutagenesis.
Nucleic Acids Res, 30(3):732-739, 01 Feb 2002
Cited by: 40 articles | PMID: 11809886 | PMCID: PMC100297
Requirement of RAD5 and MMS2 for postreplication repair of UV-damaged DNA in Saccharomyces cerevisiae.
Mol Cell Biol, 22(7):2419-2426, 01 Apr 2002
Cited by: 125 articles | PMID: 11884624 | PMCID: PMC133702
The Saccharomyces cerevisiae RAD6 group is composed of an error-prone and two error-free postreplication repair pathways.
Genetics, 155(4):1633-1641, 01 Aug 2000
Cited by: 96 articles | PMID: 10924462 | PMCID: PMC1461201
The structure and function of RAD6 and RAD18 DNA repair genes of Saccharomyces cerevisiae.
Genome, 31(2):597-600, 01 Jan 1989
Cited by: 15 articles | PMID: 2698834
Review