Abstract
Free full text

Rescue of exhausted CD8 T cells by PD-1 targeted therapies is CD28-dependent
Abstract
Programmed cell death-1 (PD-1) targeted therapies enhance T cell responses and show efficacy in multiple cancers but the role of costimulatory molecules in this T cell rescue remains elusive. Here we demonstrate that the CD28/B7 costimulatory pathway is essential for effective PD-1 therapy during chronic viral infection of mice. Conditional gene deletion showed a cell-intrinsic requirement of CD28 for CD8 T cell proliferation after PD-1 blockade. B7-costimulation was also necessary for effective PD-1 therapy in tumor-bearing mice. In addition, we found that CD8 T cells proliferating in blood after PD-1 therapy of lung cancer patients were predominantly CD28 positive. Taken together these data demonstrate CD28-costimulation requirement for CD8 T cell rescue and suggest an important role for CD28/B7 pathway in PD-1 therapy of cancer patients.
In recent years, strategies that reinvigorate tumor-specific T cells have uncovered the potential of immunotherapy (1). Sustained expression of the inhibitory receptor PD-1 characterizes exhausted T cells, and PD-1 targeted therapies have shown clinical activity in a wide variety of cancer types (2, 3). However, not all patients experience clinical benefit from PD-1 therapy and there is a critical need to determine the requirements for optimal T cell rescue not only to improve current therapies but also to identify predictive biomarkers. Blockade of inhibitory molecules improves function of exhausted T cells, but it is not known if rescue of exhausted CD8 T cells also requires positive-costimulation. CD28 is a key T cell costimulatory molecule that binds B7 molecules (4). CD28 engagement reduces T cell receptor signaling threshold required for T cell activation, and may provide qualitatively different signals (5). Naïve CD8 T cells are more dependent on CD28 than antigen-experienced cells and the requirement for CD28 signaling varies according to strength and duration of antigen exposure (6–8).
In this study we address the role of the CD28/B7 pathway for rescue of exhausted CD8 T cells following PD-1 therapy using the mouse model of life-long chronic lymphocytic choriomeningitis virus (LCMV) infection (9, 10). Expression of CD28 on LCMV-specific exhausted CD8 T cells was similar to naïve T cells, but lower than LCMV-specific memory CD8 T cells (Fig. S1). CD28 expression did not change significantly on LCMV-specific CD8 T cells following PD-1 therapy of chronically infected mice (Fig. S2). To determine the role of CD28-costimulation in PD-1 mediated rescue of exhausted CD8 T cells, we first blocked the CD28/B7 pathway by CTLA-4-immunoglobin (Ig) fusion protein administration during anti-PD-L1 therapy of chronically infected mice (Fig. 1A). As reported by Barber et. al., PD-1 blockade rescues virus-specific CD8 T cells in LCMV chronically infected mice (11). Rescue was evident by increased frequency and number of LCMV-specific CD8 T cells in anti-PD-L1 treated mice (Fig. 1B and 1C). In contrast, CTLA-4-Ig prevented anti-PD-L1-mediated expansion of LCMV-specific CD8 T cells in multiple tissues (Fig. 1B and 1C, Fig. S3A). CD8 T cells expanded by PD-L1 blockade also regained effector function as evidenced by increased IFN-γ production (Fig. S3B and C). However, when CTLA-4-Ig was combined to anti-PD-L1, IFN-γ production was similar to untreated mice (Fig. S3B and C). Thus, CTLA-4-Ig prevented rescue of LCMV-specific CD8 T cell responses mediated by PD-1 blockade.
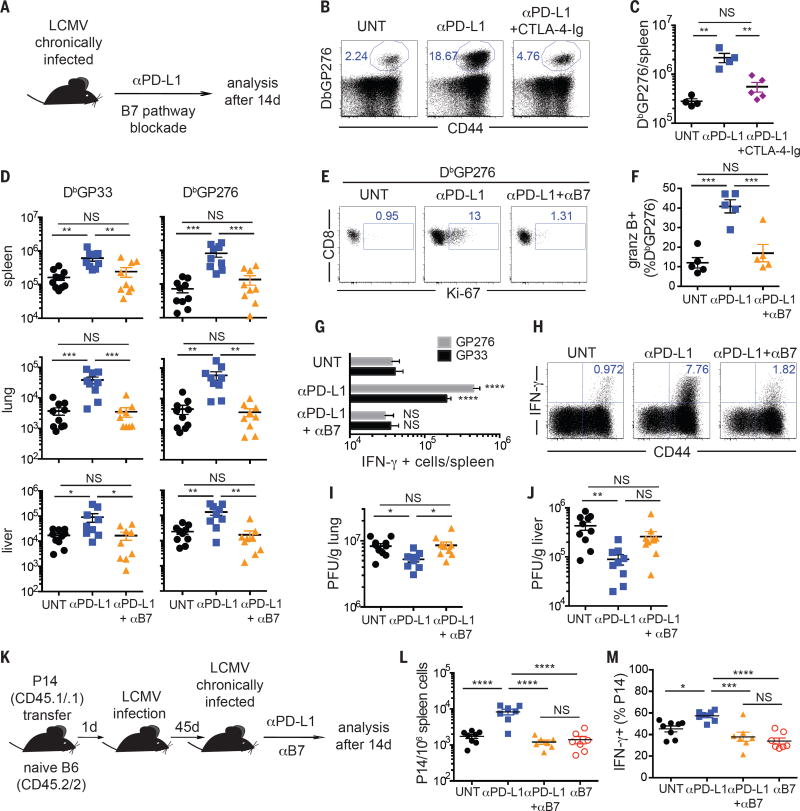
B7-costimulation is necessary for rescue of virus-specific CD8 T cells following PD-1 blockade during chronic LCMV infection. (A) Experimental layout. In B and C mice received CTLA-4-Ig, and in D-J mice received anti-B7-1 and anti-B7-2 blocking antibodies during the course of anti-PD-L1 treatment. (B) Frequencies and (C) numbers of LCMV-DbGP276-specific CD8 T cells in the spleen. (D) Numbers of LCMV-DbGP33 and LCMV-DbGP276-specific CD8 T cells in different organs. (E) Ki-67 expression on LCMV-DbGP276-specific CD8 T cells in the spleen. (F) Frequencies of splenic LCMV-DbGP276-specific CD8 T cells expressing granzyme B. (G) Numbers of IFN-γ–producing CD8 T cells in the spleen after ex vivo restimulation with the indicated peptides. Comparisons are between treated groups and untreated mice. (H) Frequencies of CD8 T cells producing IFN-γ in the spleen after ex vivo restimulation with a pool of LCMV peptides. (I) Viral titer in lung and (J) liver, as quantified by plaque assay. PFU = plaque forming units. (K) Experiment layout for L and M. (L) Frequency of P14 cells in spleen. (M) Frequency of P14 cells producing IFN-γ after ex vivo restimulation with LCMV GP33 peptide. C and F show data from one representative experiment, and D, G, I, J, L and M show combined data from two experiments. Data are representative of at least 3 independent experiments, with 3-5 mice per group. ANOVA with Sidak’s correction for multiple comparisons. *P<0.05, **P<0.01, ***P<0.001. ****P<0.0001. Error bars indicate standard error of the mean (SEM). NS = not significant.
To further extend these observations, we administered blocking antibodies to B7-1 (CD80) and B7-2 (CD86) to chronically infected mice during anti-PD-L1 therapy (Fig. 1A). B7 blockade prevented anti-PD-L1-mediated expansion of LCMV-specific CD8 T cells in spleen, lung and liver (Fig. 1D). Accordingly, B7 blockade precluded cell cycle progression of LCMV-specific CD8 T cells (Fig. 1E). B7 engagement was also necessary for increase in granzyme B expression on LCMV-specific CD8 T cells upon PD-1 blockade (Fig. 1F). In addition, when anti-PD-L1 was combined to B7 blockade, there was no increase in IFN-γ producing cells compared to untreated mice (Fig. 1G and H). Finally, anti-PD-L1 therapy was unable to reduce viral load of chronically infected mice that received B7 blockade (Fig. 1I and J). These data show that B7-costimulation is required for effective PD-1 therapy in LCMV chronically infected mice. To ensure that transient inhibition of the B7 pathway had no significant impact on the maintenance of exhausted CD8 T cells, we used an adoptive transfer of transgenic CD8 T cells specific for LCMV-GP33 (P14 cells) (Fig. 1K). The number of P14 cells was increased by PD-L1 therapy, but remained similar between mice treated with anti-PD-L1/anti-B7, untreated mice or mice receiving just anti-B7 antibodies (Fig. 1L and S4). Likewise, IFN-γ production by P14 cells was similar between mice receiving anti-PD-L1/anti-B7, anti-B7 or untreated mice (Fig. 1M). Hence, transient B7-blockade in mice with established LCMV chronic infection had no major effects on virus-specific CD8 T cells and did not affect viral load (Fig. S5).
PD-L1 can also bind B7-1 and deliver an inhibitory signal, and anti-PD-L1 antibodies used in this study block both PD-L1/PD-1 and PD-L1/B7-1 interactions (12–14). To further clarify and confirm the role of B7/CD28-costimulation in rescuing exhausted CD8 T cells during PD-1 therapy, instead of anti-PD-L1, we used two different clones of anti-PD-1 blocking antibodies (Fig. S6 and S7) (10). Similar to the data obtained with anti-PD-L1, B7-blockade also prevented rescue of LCMV-specific CD8 T cells mediated by administration of anti-PD-1.
To directly determine a cell-intrinsic requirement for CD28 signaling in PD-1 mediated rescue, we examined whether CD28-deficient P14 CD8 T cells could be rescued by anti-PD-L1 blocking antibodies during chronic LCMV infection (Fig. S8). PD-1 blockade resulted in expansion of WT P14 cells, whereas CD28KO P14 cells failed to expand in blood, spleen or lung. These data show that CD28 deficiency prevents expansion of exhausted CD8 T cells by PD-L1 blockade in a cell-intrinsic manner in both lymphoid and non-lymphoid organs of chronically infected mice. However, activation and differentiation of naïve CD28KO P14 cells into exhausted T cells may not appropriately mirror exhaustion of CD28-expressing cells. To overcome this issue, we used an inducible genetic deletion system to investigate whether loss of CD28 expression in already exhausted CD8 T cells would also impair rescue by PD-1 blockade (Fig. 2A). CD28 deletion by tamoxifen was achieved in about 50% of P14 cells expressing CreERT2 and CD28-expressing cells could be easily distinguished from CD28-deficient cells (Fig. 2B). In mice treated with anti-PD-L1 blocking antibodies, Ki-67 expression on P14 cells was largely restricted to CD28-expressing cells (Fig. 2B and C). Thus, PD-1 blockade was ineffective to induce proliferation of exhausted P14 cells that had lost CD28.
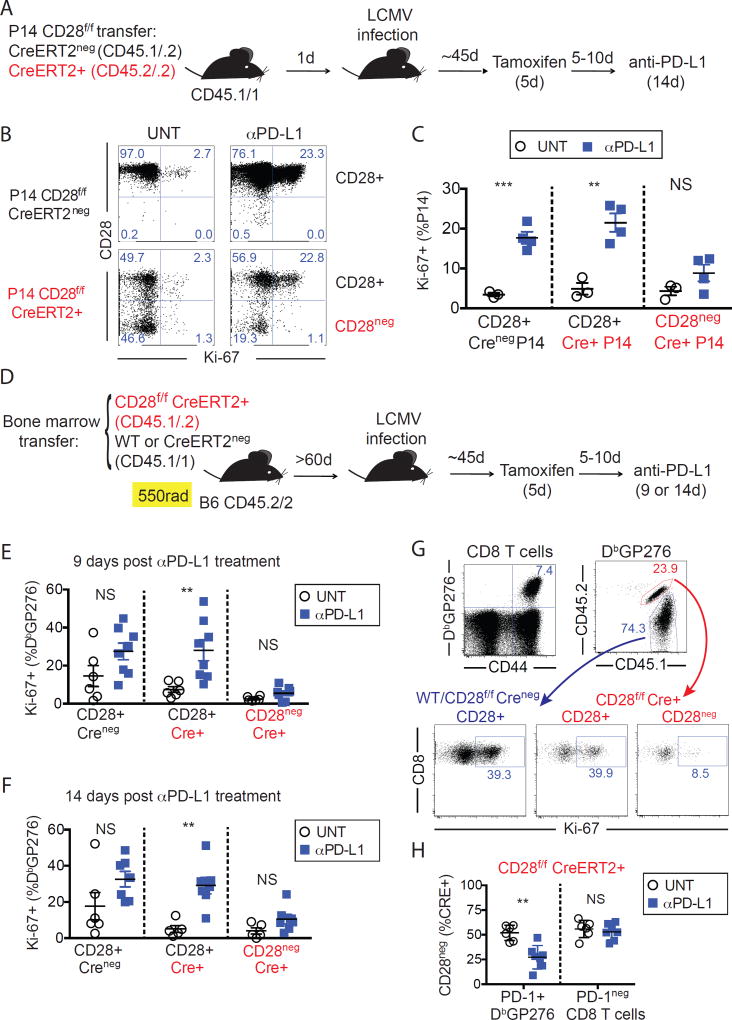
Cell-intrinsic requirement of CD28 expression for exhausted CD8 T cell expansion upon PD-1 blockade. (A) Experimental layout for B and C. (B) CD28 and Ki-67 expression on P14 CD28f/f CreERT2neg and P14 CD28f/f CreERT2+, in the spleen of representative mice. (C) Frequencies of cells expressing Ki-67 in the spleen among each indicated population. Summary of data as in B. Data from B and C are from one representative experiment of 3 independent experiments, with at least 3 mice per group. (D) Experimental layout for E–H. (E) Frequencies of cells expressing Ki-67 in the spleen among each indicated population, 9 days after anti-PD-L1 treatment. (F) as in E, but 14 days after anti-PD-L1 treatment (G) Gating strategy and Ki-67 expression on splenocytes from a representative mouse treated with anti-PD-L1 for 14 days. (H) Frequency of CD28neg cells among each population of Cre+ cells as indicated, 14 days after anti-PD-L1 treatment. Data from E-H are from two or three independent experiments, with at least three mice per group. C, E, F, H unpaired t-test, **P<0.01, ***P<0.001. Error bars indicate SEM. NS = not significant.
To determine whether cell-intrinsic CD28 expression also affects responsiveness of non-transgenic exhausted CD8 T cells to PD-1 blockade, we performed similar experiments in mixed bone marrow chimeric mice (Fig. 2D). We assessed proliferation of LCMV-specific CD8 T cells by Ki-67 expression after anti-PD-L1 treatment and found that proliferation was restricted to CD28+ cells (Fig. 2 E–G). Selective proliferation of CD28-expressing cells by PD-1 therapy resulted in decreased frequency of CD28neg cells among PD-1+ LCMV-specific Cre+ CD8 T cells compared to untreated mice. In contrast, we found no differences in the frequency of CD28neg cells among PD-1neg CD8 T cells between untreated and anti-PD-L1 treated mice (Fig. 2H). In summary, proliferation of PD-1+ CD8 T cells by blockade of the PD-1 pathway during chronic LCMV infection is contingent on CD28 expression.
To determine whether CD28 signaling would also be necessary for reinvigoration of anti-tumor CD8 T cell responses, we analyzed the role of the CD28/B7 pathway for tumor control of CT-26 colon carcinoma by PD-1 therapy. We observed rapid tumor growth in all untreated mice and mice receiving anti-B7, whereas PD-L1 blocking antibodies elicited tumor regression in 8 out of 9 animals. In contrast, 8 out of 10 mice receiving anti-PD-L1 in combination with B7 blocking antibodies showed tumor progression (Fig. 3A). The effectiveness of PD-1 therapy for suppressing CT26 tumor growth resulted in a significant improvement in overall survival of anti-PD-L1 treated mice compared to untreated mice (P<0.001), mice treated with anti-B7 alone (P<0.001) or mice receiving both anti-PD-L1/anti-B7 (P=0.0169) (Fig 3B). Also, there was no significant improvement in the survival of mice treated with anti-PD-L1/anti-B7 compared to untreated mice (P=0.1092). Figure 3C shows the summary of three independent experiments. In accordance to our findings, PD-1 blockade failed to control growth of YUMM2.1 melanoma tumor in mice deficient for CD28 or B7-1/B7-2 (15). In summary, our experiments show that CD28-costimulation is necessary for effective PD-1 therapy in a murine tumor model.
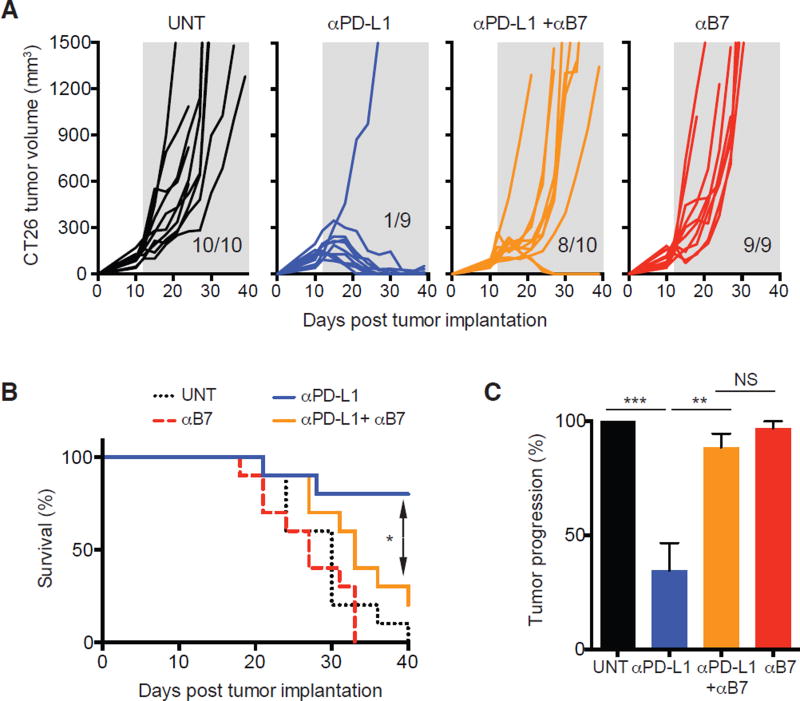
Effectiveness of PD-1 therapy for control of CT-26 tumor relies on the CD28/B7 pathway. Mice were depleted of CD4 T cells for the duration of the experiment. CT-26 tumor bearing mice (mean volume of about 150 mm3) were enrolled into different treatment groups as indicated. (A) Individual tumor growth, represented by tumor volume. Insets on each panel show ratio of mice that experienced tumor progression. Shaded grey area indicates duration of treatment. (B) Survival curves from data in A. Comparisons are by log-rank (Mantel-Cox) test *P<0.05. A and B show one representative experiment out of three. (C) Percentage of mice unable to control tumor growth. Summary of three independent experiments with 7–12 mice per group in each experiment. ANOVA with Sidak’s correction for multiple comparisons. *P<0.05, **P<0.01, ***P<0.001. Error bars indicate SEM. NS = not significant.
To further explore the role of the CD28/B7 pathway in cancer immunotherapy we analyzed samples from advanced lung cancer patients receiving PD-1 therapies. We hypothesized that human PD-1+ CD8 T cells would need to express CD28 to efficiently expand upon PD-1 therapy. Human effector CD8 T cells induced by vaccination have been identified by HLA-DR, CD38 and Ki-67 expression (16). Hence we analyzed proliferation (Ki-67) and activation (HLA-DR, CD38) of peripheral blood PD-1+ CD8 T cells during PD-1 therapy in non-small cell lung cancer (NSCLC) patients (Fig. 4A). Following therapy initiation, in about half of patients we observed an increase in Ki-67-expressing CD8 T cells, largely restricted to PD-1-positive cells (17) (Fig. 4B). These CD8 T cells also expressed high levels of CD38 and HLA-DR (Fig. 4C). To assess expression of CD28 on CD8 T cells responding to PD-1 therapy, we focused our analysis on patients that had at least a two-fold increase in the frequency of Ki-67+ PD-1+ CD8 T cells (Fig. 4D). In accordance with our predictions, PD-1+ CD8 T cells activated by PD-1 therapy in NSCLC patients were mostly CD28+. These data suggest that CD28 signals may also be important for proliferation of PD-1+ CD8 T cells during PD-1 therapy in cancer patients.
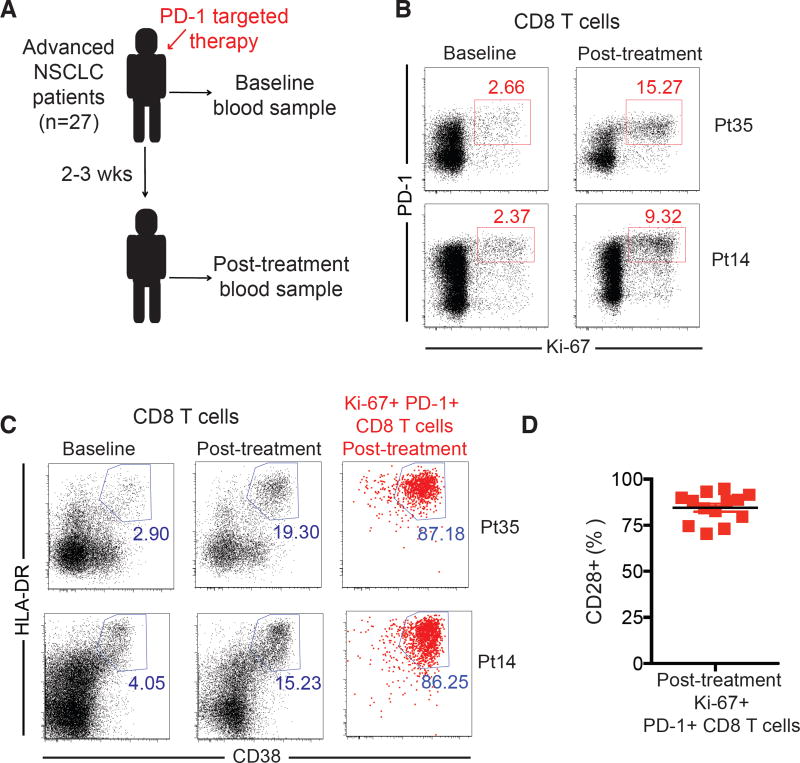
PD-1+ CD8 T cells that proliferate in the peripheral blood of lung cancer patients receiving PD-1 therapy express CD28. (A) Overview of study design. (B) Ki-67 and PD-1 expression on CD8 T cells from two representative patients (Pt) with significant CD8 T cell responses after PD-1 targeted therapy. (C) as in B, but showing HLA-DR and CD38 expression. Dot plots in the right were gated on post-treatment Ki-67+ PD-1+ CD8 T cells as indicated in B. (D) CD28 expression on Ki-67+ PD-1+ CD8 T cells in post-treatment samples as gated in B. N=13, patients with least a two-fold increase from baseline in the frequency of Ki-67+PD-1+ CD8 T cells.
Many studies have assessed expression of inhibitory receptors on exhausted CD8 T cells, but positive costimulatory molecules have not been a major focus. In humans, antigen-experienced CD8 T cells can lose CD28 expression, and loss of CD28 has been associated with chronic stimulation (18). We obtained tumor samples from early stage NSCLC patients and examined CD28 expression on freshly isolated CD8 T cells (Fig. S9A). Confirming previous reports (19, 20), we found variable CD28 expression, ranging from 20 to 90% of the CD8 tumor infiltrating lymphocyte (TIL) population, in individual NSCLC patient samples (Fig. S9B and C). Among NSCLC TILs, TIM-3neg PD1+ CD8 T cells contained a higher proportion of CD28-positive cells when compared to TIM-3+ PD-1+ TILs in the same tumor (Fig. S9D and E). Thus, many human CD8 TILs do not express CD28 and thus, according to our data and hypothesis, as well as with previous studies on T cell senescence, may be less responsive to proliferate upon PD-1 blockade (18, 19). Our laboratory recently identified in chronically infected mice the LCMV-specific CD8 T cell subpopulation that provides the proliferative burst after PD-1 therapy (21). These stem-cell-like TIM-3neg PD-1+ TCF-1+ virus-specific CD8 T cells express higher levels of positive costimulatory molecules (CD28, ICOS, OX40 and LIGHT) and lower levels of inhibitory receptors compared to TIM-3+ PD-1+ TCF-1neg counterparts that do not expand after PD-1 therapy. Yet, in spite of the expression of several positive co-stimulatory molecules by TIM-3neg PD-1+ TCF-1+ virus-specific CD8 T cells, we show that CD28-signaling plays a major and non-redundant role for response to PD-1 blockade. This highlights the dominant role of CD28 signaling in the proliferative response to PD-1 blockade.
Since most tumors (and many virus-infected cells) do not express B7 molecules, our model implicates participation of B7-expressing antigen-presenting cells in the efficacy of PD-1 therapy. Indeed, recent studies found associations between dendritic cell infiltration and maturation and response to PD-1 blockade (22, 23). It is important to highlight, that the PD-1 pathway can modulate T cell responses at two different levels: (i) reducing T cell activation by antigen-presenting cells, and (ii) inhibiting target cell elimination (infected cells or tumors) (24). Since most target cells do not express B7, it is conceivable that local elimination of target cells may be enhanced by PD-1 blockade in a CD28-independent manner. In contrast, we show that T cell expansion that follows PD-1 therapy requires CD28-costimulation. Efficacy of PD-1 therapy in cancer patients has been associated to proliferation of CD8 TILs (25). Therefore systemic effects of PD-1 blockade that result in clinical benefit most likely require amplification of T cell responses by proliferation of PD-1+ CD8 T cells and thus CD28-costimulation.
In conclusion, we show a cell-intrinsic requirement for CD28-costimulation in the expansion of PD-1+ CD8 T cells and effectiveness of PD-1 therapy in murine models of chronic viral infection and cancer. In lung cancer patients, PD-1+ CD8 T cells that proliferate in the peripheral blood after PD-1 blockade express CD28. Our data imply selective proliferation of CD28+ cells by PD-1 therapy and suggest further evaluation of CD28 as a potential biomarker to predict CD8 T cell responses in cancer patients. In addition, Hui et. al. show that PD-1 directly targets CD28 cytoplasmic tail, with higher affinity than T cell receptor downstream molecules, further emphasizing the interplay between the CD28 and the PD-1 pathway (26). Taken together these data provide greater insight into the molecules and interactions involved in T cell exhaustion and PD-1 directed immunotherapy.
Acknowledgments
This work was supported by Merck pre-clinical grant 52507 (RA and AOK), and the National Institutes of Health grants R01 AI30048 (RA), P01 AI080192 (RA and GJF), P01 AI056299 (AHS, RA, GJF and BRB), P01 AI054456 (AHS), R01 AI089955 (GJF), R01 CA72669 (BRB), R01 AI037691 (LAT and RZ). RA, DLB, GJF and AHS are inventors on patent numbers US 8552154 B2, US 8652465 B2, and US 9102727 B2, held by Emory University (Atlanta), Dana-Farber Cancer Institute (Boston), Brigham and Women’s Hospital (Boston) and Harvard University (Cambridge), that cover the topic of PD-1 directed immunotherapy. We thank Mandy Ford (Emory University) for reagents, and Evgeniy Eruslanov (University of Pennsylvania School of Medicine) for experimental advice.
References and Notes
Full text links
Read article at publisher's site: https://doi.org/10.1126/science.aaf0683
Read article for free, from open access legal sources, via Unpaywall:
https://science.sciencemag.org/content/sci/355/6332/1423.full.pdf
Citations & impact
Impact metrics
Citations of article over time
Alternative metrics
Smart citations by scite.ai
Explore citation contexts and check if this article has been
supported or disputed.
https://scite.ai/reports/10.1126/science.aaf0683
Article citations
Targeted Drug Delivery in Periorbital Non-Melanocytic Skin Malignancies.
Bioengineering (Basel), 11(10):1029, 15 Oct 2024
Cited by: 0 articles | PMID: 39451404 | PMCID: PMC11504966
Review Free full text in Europe PMC
PD-1 and CTLA-4 serve as major gatekeepers for effector and cytotoxic T-cell potentiation by limiting a CXCL9/10-CXCR3-IFNγ positive feedback loop.
Front Immunol, 15:1452212, 15 Oct 2024
Cited by: 0 articles | PMID: 39474427 | PMCID: PMC11519525
Pre-operative stereotactic radiosurgery and peri-operative dexamethasone for resectable brain metastases: a two-arm pilot study evaluating clinical outcomes and immunological correlates.
Nat Commun, 15(1):8854, 14 Oct 2024
Cited by: 0 articles | PMID: 39402027 | PMCID: PMC11473782
Emerging druggable targets for immune checkpoint modulation in cancer immunotherapy: the iceberg lies beneath the surface.
Apoptosis, 29(11-12):1879-1913, 01 Oct 2024
Cited by: 0 articles | PMID: 39354213
Review
Analysis of the implication of steroid 5 alpha-reductase 3 on prognosis and immune microenvironment in Liver Hepatocellular Carcinoma.
Ann Med, 56(1):2408463, 28 Sep 2024
Cited by: 0 articles | PMID: 39340288 | PMCID: PMC11441025
Go to all (525) article citations
Data
Data behind the article
This data has been text mined from the article, or deposited into data resources.
BioStudies: supplemental material and supporting data
Similar Articles
To arrive at the top five similar articles we use a word-weighted algorithm to compare words from the Title and Abstract of each citation.
Analysis of the role of negative T cell costimulatory pathways in CD4 and CD8 T cell-mediated alloimmune responses in vivo.
J Immunol, 174(11):6648-6656, 01 Jun 2005
Cited by: 115 articles | PMID: 15905503
PD-1 blockade-unresponsive human tumor-infiltrating CD8+ T cells are marked by loss of CD28 expression and rescued by IL-15.
Cell Mol Immunol, 18(2):385-397, 24 Apr 2020
Cited by: 32 articles | PMID: 32332901 | PMCID: PMC8027446
Defining CD8+ T cells that provide the proliferative burst after PD-1 therapy.
Nature, 537(7620):417-421, 02 Aug 2016
Cited by: 1080 articles | PMID: 27501248 | PMCID: PMC5297183
The PD-1/PD-L1 (B7-H1) pathway in chronic infection-induced cytotoxic T lymphocyte exhaustion.
J Biomed Biotechnol, 2011:451694, 25 Sep 2011
Cited by: 87 articles | PMID: 21960736 | PMCID: PMC3180079
Review Free full text in Europe PMC
Funding
Funders who supported this work.
Merck (2)
Grant ID: award296711
Grant ID: 52507
NCI NIH HHS (2)
Grant ID: P01 CA065493
Grant ID: R01 CA072669
NHLBI NIH HHS (1)
Grant ID: R01 HL056067
NIAID NIH HHS (9)
Grant ID: R01 AI030048
Grant ID: P01 AI054456
Grant ID: R01 AI089955
Grant ID: R37 AI034495
Grant ID: P01 AI080192
Grant ID: P01 AI056299
Grant ID: R01 AI037691
Grant ID: R01 AI139675
Grant ID: R01 AI034495
National Institutes of Health (2)
Grant ID: award296710
Grant ID: AI30048,AI080192,AI056299,AI054456,AI089955,CA72669,AI037691