Abstract
Free full text

Later circadian timing of food intake is associated with increased body fat
Abstract
Background: Weight gain and obesity have reached alarming levels. Eating at a later clock hour is a newly described risk factor for adverse metabolic health; yet, how eating at a later circadian time influences body composition is unknown. Using clock hour to document eating times may be misleading owing to individual differences in circadian timing relative to clock hour.
Objective: This study examined the relations between the timing of food consumption relative to clock hour and endogenous circadian time, content of food intake, and body composition.
Design: We enrolled 110 participants, aged 18–22 y, in a 30-d cross-sectional study to document sleep and circadian behaviors within their regular daily routines. We used a time-stamped-picture mobile phone application to record all food intake across 7 consecutive days during a participant’s regular daily routines and assessed their body composition and timing of melatonin release during an in-laboratory assessment.
Results: Nonlean individuals (high body fat) consumed most of their calories 1.1 h closer to melatonin onset, which heralds the beginning of the biological night, than did lean individuals (low body fat) (log-rank P = 0.009). In contrast, there were no differences between lean and nonlean individuals in the clock hour of food consumption (P = 0.72). Multiple regression analysis showed that the timing of food intake relative to melatonin onset was significantly associated with the percentage of body fat and body mass index (both P < 0.05) while controlling for sex, whereas no relations were found between the clock hour of food intake, caloric amount, meal macronutrient composition, activity or exercise level, or sleep duration and either of these body composition measures (all P > 0.72).
Conclusions: These results provide evidence that the consumption of food during the circadian evening and/or night, independent of more traditional risk factors such as amount or content of food intake and activity level, plays an important role in body composition. This trial was registered at clinicaltrials.gov as NCT02846077.
See corresponding editorial on page 1171.
INTRODUCTION
The coordination and timing of specific behaviors and biological processes in the brain and body are necessary for healthy physiologic functioning. Human circadian physiology has evolved to promote certain behaviors and activities during the day (e.g., physical activity, arousal, and metabolism) or night (e.g., sleep and fasting) (1). In mammals, the internal circadian timing system is entrained to the 24-h light-dark cycle through light signals reaching a master clock located within the hypothalamus (2). With the relatively recent widespread availability of electrical lighting, humans have the ability to self-select their light-dark cycles and extend wakefulness activities far into the night (3). This capability to alter the timing of wakefulness can result in a misalignment between behavior and endogenous circadian physiology (3, 4) and has been associated with adverse health outcomes such as decreased energy metabolism (5, 6), impaired glucose metabolism (7–9), and increased cardiovascular disease risk (7, 10).
One potential mechanism for adverse health from the misalignment between behavior and physiology is food consumption at an inappropriate circadian time (11, 12). There have been numerous reports in animal models (13, 14) and human studies (15, 16) that eating during a time normally reserved for sleeping can result in weight gain and adverse metabolic health. Populations that are susceptible to pushing activities to a later clock hour, such as night or rotating shift workers (17) or adolescents and college-aged individuals (18–20), also have a higher incidence of weight gain and obesity (21–23). Therefore, understanding the interaction of the timing of dietary intake with an individual’s circadian rhythms is important, particularly because using clock hour to document eating times may not be physiologically relevant due to individual differences in circadian timing relative to clock hour (24).
In this cross-sectional study (registered at clinicaltrials.gov as NCT02846077), we characterized the eating patterns of college-aged individuals during a week of their regular daily activities by documenting the timing and content of their entire dietary intake with the use of a mobile phone application (Figure 1). The relation of these patterns was then quantified relative to circadian timing, habitual sleep timing and duration, and wakefulness behaviors.
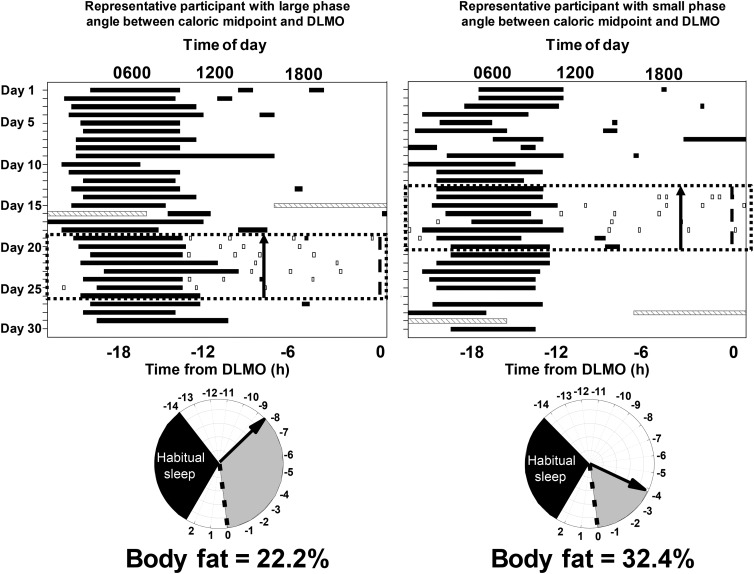
Raster plots (top) of the ~30-d protocol from 2 participants: 1 with a large phase angle (time difference) between caloric midpoint (average time at which 50% of daily calories were consumed) and DLMO timing (left) and 1 with a small phase angle between caloric midpoint and DLMO timing (right). The x-axis represents the time of day, and the y-axis represents days into the protocol. The black bars represent sleep episodes, gray hatched bars represent the in-laboratory overnight stay for recording percentage of body fat and a DLMO phase assessment (light <4 lux), the black dotted box represents the week of monitoring caloric intake via the time-stamped mobile phone picture food diary, and the open boxes represent caloric events (i.e., any food or beverage that is not water). Note that the in-laboratory assessment could occur before or after the week of monitoring caloric intake but was during the ~30 d of the protocol. The black arrow on the top and bottom panels denotes the timing of caloric midpoint relative to DLMO. Relative timing of physiologic events in the 2 participants (bottom). The dotted line is the timing of the DLMO, the gray shaded area denotes the phase angle, and the black shaded area denotes habitual sleep timing for that participant relative to DLMO. DLMO, dim-light melatonin onset.
METHODS
Participants
Participants (n = 110, 64 males; Supplemental Figure 1 and Supplemental Table 1) with an age range of 18–22 y were enrolled in a cross-sectional 30-d protocol to document sleep and circadian behaviors within their regular daily routines (Figure 1). Participants were of mixed ethnicity (21.0% Hispanic, 76.0% non-Hispanic, and 3.0% unknown) and race (1.0% American Indian, 30.9% Asian, 12.7% African American, 41.8% white, 10.0% reporting >1, and 3.6% not reported). They were recruited through e-mail, paper flyers, and verbal communication. Exclusion criteria consisted of inability to wear actigraphy monitors, inability to download phone application, and travel across >1 time zone within a 3-mo period before and during the protocol. No participants reported night work during the protocol. All participants provided written informed consent, and the Partners Health Care (#2012P001631) and Massachusetts Institute of Technology (#1209005240) Institutional Review Boards approved all study procedures.
Sleep and wakefulness actigraphy monitoring
On the day before starting the ~30-d sleep-wake monitoring protocol, participants met with study staff to learn about specific study procedures, obtain study materials, and complete a series of questionnaires to determine habitual sleep and wakefulness patterns. At the conclusion of the meeting, participants were given a wrist actigraphy monitor (Motionlogger; Ambulatory Monitoring) and instructed to wear the device on their nondominant arm at all times for the duration of the protocol except for when the monitor might get wet or damaged. Participants also completed electronic sleep-wake and exercise diaries during the ~30-d protocol. Diaries were sent to the participant’s e-mail once each morning (0700) and once each evening (2000). Actigraphy monitors were downloaded and checked at weekly meetings, and electronic diaries were checked daily to ensure completion and accuracy of sleep-wake timing. Sleep timing and duration were manually scored from actigraphy and corroborated with diary sleep-wake times (25).
Meal documentation
For 7 consecutive days, participants were asked to record all food and beverages they consumed, with the exception of water, by the use of the mobile phone application MealLogger (Wellness Foundry). MealLogger allowed participants to take a time-stamped photograph of their meal, include a detailed description of the meal content, and identify which meal they were eating (i.e., breakfast, lunch, dinner, beverage, or snack). The 7-d monitoring period for meal timing and content began ≥1 wk after the start of the protocol to ensure participants were familiar with other study procedures. Participants were instructed via detailed presentations how to accurately identify what meal they were eating, how to take a photograph of the meal with an item of known size (e.g., fork, pen) in the frame of reference for portion size estimation, and how to add a detailed description of each meal to identify correct nutrient content (e.g., what type of dressing on a salad, mayonnaise on a bun, or sugar in coffee). Immediately after the photograph was taken, the data were available to study staff via Web access. Dietary and study staff followed up with participants within 24-h after the meal was documented if any clarification of meal composition was needed. If meals were not fully consumed, participants took a second photograph to display what remained.
Inpatient study procedures
For calculation of circadian phase, participants were admitted to the Brigham and Women’s Hospital Center for Clinical Investigation Intensive Physiologic Monitoring Unit for one ~16-h overnight stay. Participants arrived at the laboratory at ~1400 and were admitted to a sound-attenuated, temperature-controlled suite with dim-lighting conditions (<4 lux). The use of any personal electronic devices (e.g., cell phone, tablet, or computer) was prohibited due to the influence of artificial lighting on circadian outcomes (3, 26). Beginning at 1600, salivary melatonin samples were collected hourly until 0700 the next morning. Participants were instructed to remain seated in a constant posture and not eat or drink any foods for 20 min before saliva collection to limit any potential influences on salivary melatonin assessments. Between the hourly saliva collections, participants were allowed to be ambulatory, sleep in a seated position, or sit quietly. If participants were asleep, they were awakened by research staff to collect saliva. Dim-light melatonin onset (DLMO), an established marker of circadian phase and onset of biological night, was calculated as the linear interpolated point in time at which melatonin concentrations crossed and maintained concentrations above a 5-pg/mL threshold (27).
Body composition was assessed via 4-lead bioelectrical impedance (Quantum II BIA analyzer; RJL Systems). On arrival to the laboratory, participants removed jewelry and electrical devices, laid flat on a hospital stretcher, and electrodes were placed on the participant’s right hand and foot. Each impedance measurement was calculated 3 times to provide consistent results; an average of these 3 readings was used for analysis.
Analysis
Meals were scored by the Brigham and Women’s Hospital Center for Clinical Investigation dietary staff. All meals were coded in duplicate (i.e., scored by 2 nutritionists) to determine food item and portion size, and any discrepancies were solved to minimize error before scoring for nutrient content by using the University of Minnesota Nutrition Data System for Research software (28, 29). Meals that were identified by the participant as the same type of meal (e.g., dinner) and consumed within 15 min of each other were combined into 1 event (30), with the latest meal documented used for time of the meal to better estimate how late calories were consumed. Participants needed to complete a minimum of 4 d of meal recording to be included in the analysis or otherwise were identified as declining participation in meal logging (Supplemental Figure 1).
We calculated the timing of calories consumed relative to clock hour and relative to the timing of DLMO (Figure 1). Because of the physiologic differences in the percentage of body fat between men and women (31), participants were grouped by sex-dependent criteria of percentage of body fat into lean or nonlean groups (32), which have been used in similarly aged populations (33). The lean group was defined as 5–20% body fat for men and 8–30% body fat for women, and the nonlean group was defined as >21% body fat for men and >31% for women (32). The activity level for each participant was determined from the reported exercise activity from the daily diaries by using percentage of days exercised within the protocol. We also examined activity level by using predefined levels for sedentary (activity factor: 1.20), mild (activity factor: 1.375), moderate (activity factor: 1.55), or heavy activity (activity factor: 1.725) (34). To determine potential misalignment between sleep and wakefulness timing on free days (e.g., weekends) compared with work and school days (e.g., weekdays), social jetlag was calculated as the difference between the midpoint of sleep on free days and on work and school days as quantified by the Munich Chronotype Questionnaire (35).
All group comparisons for men compared with women were performed by using unpaired t tests and for weekday compared with weekend by using paired t tests. Kaplan-Meier survival curves were compared with log-rank tests (36). Multiple linear regressions were performed for associations with percentage body fat or BMI as the dependent variable and sex, caloric midpoint relative to DLMO, 24-h total sleep time, caloric intake, and percentage of calories from fat, carbohydrates, and proteins as independent variables. Pearson correlations were used for associations. All statistics were performed with SAS 9.4 software (SAS Institute Inc.). Of the 110 participants included in the analysis, 4 (3 lean and 1 nonlean) did not have usable DLMO data, 6 did not have usable actigraphy data, and 1 did not have usable social jetlag data.
RESULTS
Daily eating habits
More than 2300 caloric events (food or beverage other than water) were recorded. Approximately 93% of participants documented caloric events for 7 d and 100% for 6 d of monitoring, with a mean ± SD (range) of 3.1 ± 1.4 (1.5–9.3) caloric events/d. The participants denoted ~19% of the caloric events as breakfast, 20% as lunch, 28% as dinner, 23% as beverages or snacks consumed before a recorded dinner, and 10% as beverages or snacks consumed after a recorded dinner (Supplemental Table 2). The timing of breakfast was 1026 ± 0144 (0457–1757); lunch, 1401 ± 0207 (0900–0127); predinner snacks, 1554 ± 0457 (0612–0439); dinner, 1945 ± 0157 (1532–336); and after-dinner snacks, 2245 ± 0228 (1715–0543), with men having significantly later after-dinner snacks compared with women (P = 0.003; Supplemental Figure 2A). There was no significant difference (P = 0.12) in the clock times at which men and women reached their caloric midpoint (i.e., average time at which 50% of daily calories were consumed). Participants ate significantly later breakfast, lunch, predinner snacks, and dinner on Saturday and Sunday (weekend) than on all other days (weekdays) (all P < 0.05; Supplemental Figure 2B).
Timing of food intake and body fat composition
The lean and nonlean groups both had a similar timing of DLMO of 2325 ± 0148 (1752–0338) and 2303 ± 0130 (1946–0135), respectively (P = 0.29). When the timing of calories was examined relative to the timing of the individuals’ DLMO, the nonlean group consumed their caloric midpoint 1.1 h closer to DLMO (i.e., at a later biological time) than the lean group (HR: 1.7; 95% CI: 1.1, 2.6; log-rank P = 0.009; Figure 2A). This was also true for the timing of the latest daily caloric event: the nonlean group ate their latest daily calories at a later time relative to DLMO (0.9 h; HR: 1.6; 95% CI: 1.0, 2.5; log-rank P = 0.03). There was no significant difference in clock time of the 2 groups’ caloric midpoints (1614 compared with 1641; HR: 0.9; 95% CI: 0.6, 1.4; log-rank P = 0.72; Figure 2B) or clock time at which the 2 groups consumed their latest daily calories (HR: 1.0; 95% CI: 0.7, 1.5; log-rank P = 0.98).
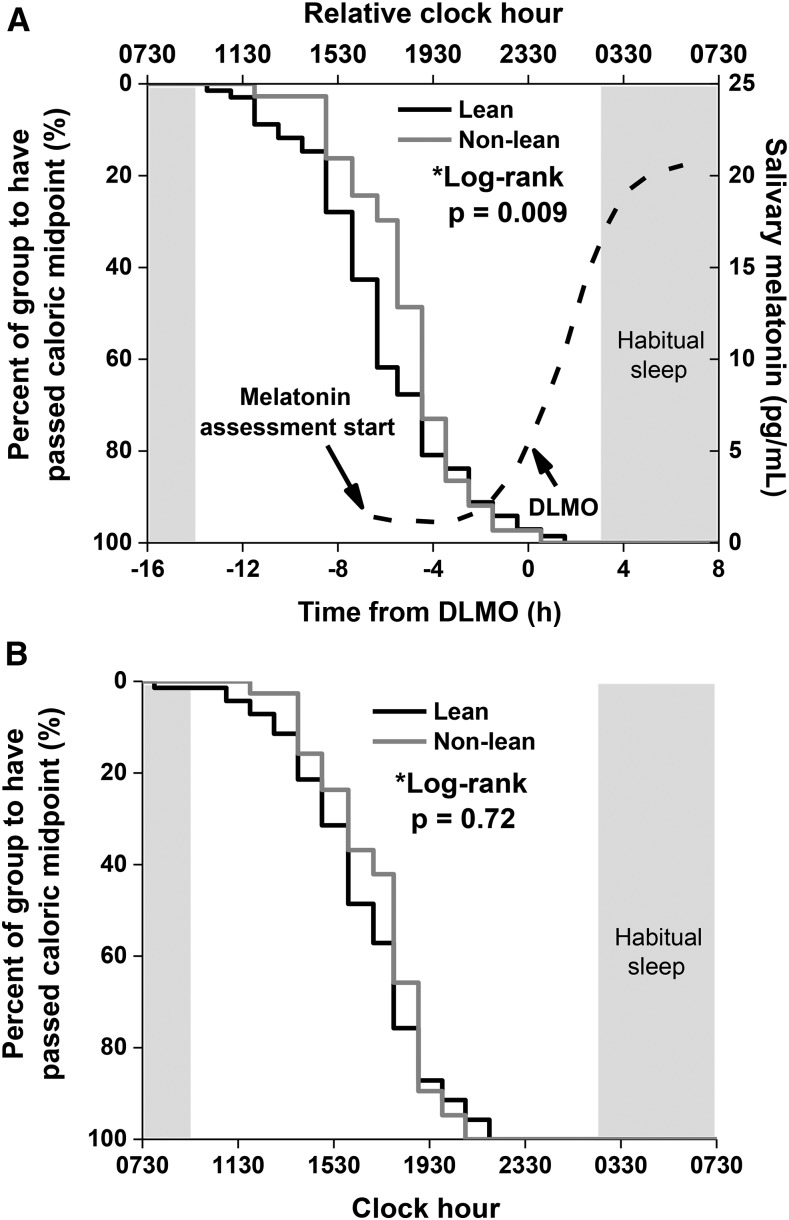
Kaplan-Meier survival curves of lean (n = 67; black line) and nonlean (n = 39; gray line) participants’ time to pass caloric midpoint (average time at which 50% of daily calories were consumed) relative to DLMO (A) and clock hour (B). Lean and nonlean participants were defined by their percentage of body fat and by sex (32). The dashed curve and corresponding right y axis represent the average melatonin concentrations of all participants, and the shaded box represents the average habitual timing of sleep of the whole population. P values were derived from log-rank tests. DLMO, dim-light melatonin onset.
The postprandial energetic response to food intake (diet-induced thermogenesis) can last >6 h; however, >90% of that response is observed within 5 h of food intake when meals of varying sizes and compositions are examined (37). Therefore, we examined the relation between the caloric midpoint and the timing of caloric events between 4 h before DLMO and sleep onset. By measuring calories consumed between 4 h before DLMO and sleep onset, we expect a portion of those calories will still be digested during and after DLMO (i.e., during the biological night). Individuals who consumed a greater percentage of their daily calories between 4 h before DLMO and sleep onset had a higher percentage of body fat (P = 0.006; Figure 3). Furthermore, individuals with a caloric midpoint at a later clock hour had a greater number of caloric events between 4 h before DLMO and sleep onset (P < 0.001).
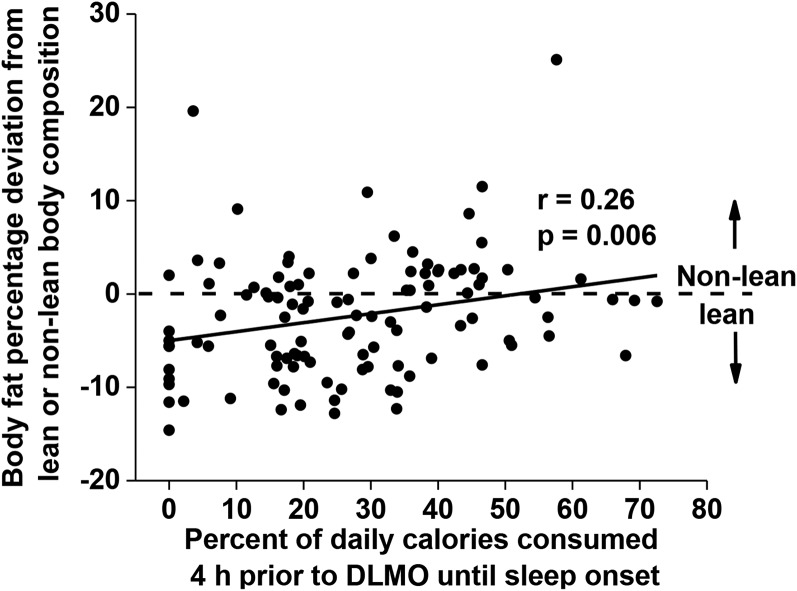
Correlation between the percentage of calories consumed between 4 h before DLMO and sleep onset (x-axis) and body fat percentage represented as a deviation from the sex-specific criteria of lean (n = 67) or nonlean (n = 39) body composition (y-axis). The horizontal dashed line at 0 represents the threshold to be categorized as lean (<0) or nonlean (>0). Data were analyzed with the Pearson correlation. DLMO, dim-light melatonin onset.
Relations between food intake, sleep, circadian measures, and body composition
Female sex (31), positive energy balance (calories consumed more than calories expended) (38), and short sleep durations (39) have previously been associated with a higher body fat percentage. To our knowledge, how these variables contribute to body composition when accounting for the circadian timing of food intake has been not studied. Thus, we tested the contribution of the circadian timing of food intake, caloric amount, macronutrient composition (i.e., percentage of calories from carbohydrates, fats, or proteins), sleep duration, and activity level by using multiple linear regression models that controlled for sex. The timing of the caloric midpoint relative to DLMO was the only variable significantly associated with the percentage of body fat (β = −0.15; 95% CI: −0.27, −0.02; P = 0.02) when controlling for sex. This was also true when we used BMI as our dependent variable (β = −0.23; 95% CI: −0.45, −0.01; P = 0.04).
There was a significant association between the timing of the latest daily food intake relative to DLMO and 24-h total sleep duration (i.e., major sleep plus naps), such that individuals with their latest daily food intake closer to DLMO had less sleep (Figure 4A). No association, however, was found between clock hour of the latest food intake and 24-h total sleep duration (Figure 4B). In this cohort, we found no association between social jetlag and body composition (Supplemental Figure 3A) or between eating duration (the length between the first and last caloric event each day) and body composition (Supplemental Figure 3B). There was also no association between social jetlag and the percentage of daily calories consumed 4 h before DLMO until sleep onset (Supplemental Figure 3C), but there was a positive association between eating duration and the percentage of calories consumed 4 h before DLMO until sleep onset, such that individuals who had a longer eating duration consumed a greater percentage of their calories during that time (Supplemental Figure 3D).
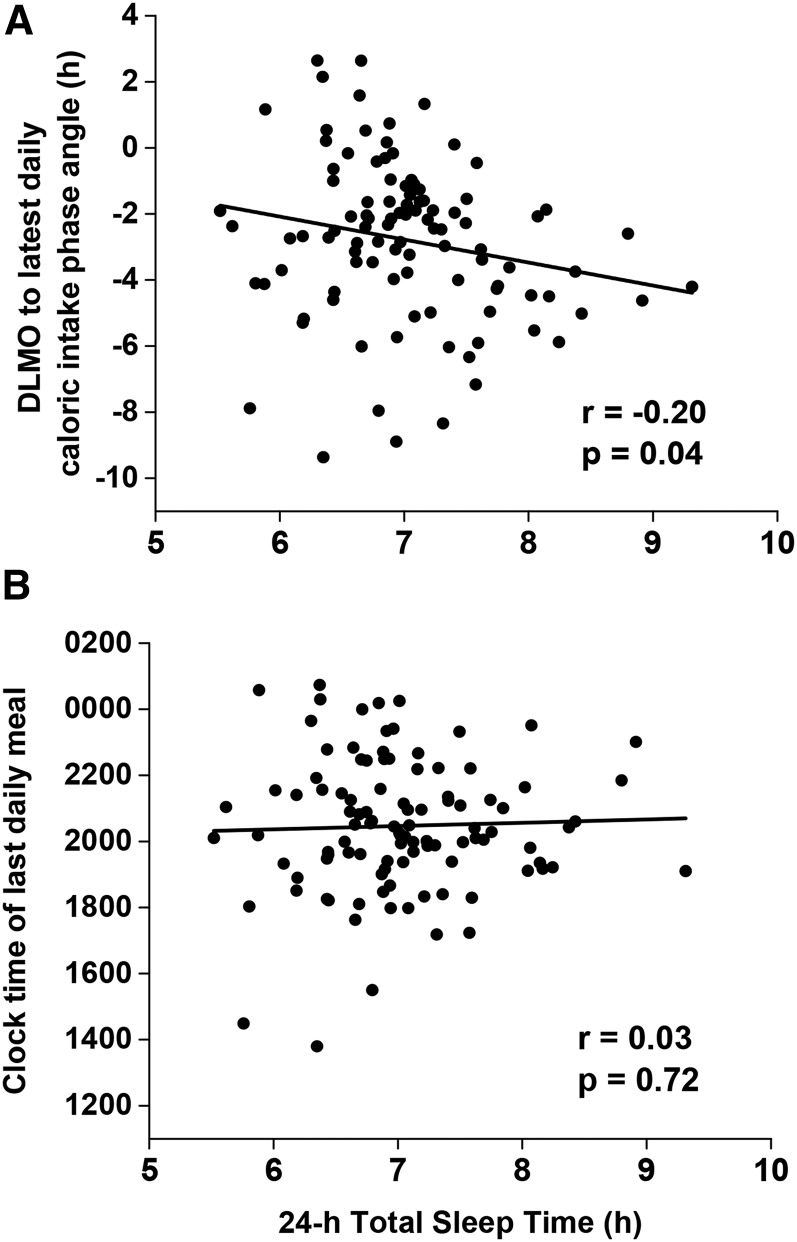
Correlations between 24-h total sleep time (x-axis) and the time difference of the latest daily calories relative to DLMO timing (i.e., time of latest calories minus DLMO) represented as phase angle (n = 100) (y-axis) (A) and clock hour of the latest daily calories (n = 104) (y-axis) (B). Data were analyzed with the Pearson correlation. DLMO, dim-light melatonin onset.
DISCUSSION
Weight gain and obesity have reached alarming levels throughout the developed world. Because overweight or obese adolescents have double the risk for high weight status in adulthood (40), and college is a critical developmental time for establishing long-term health behaviors (41), understanding behaviors that may promote improved health is clinically relevant and vital to this population. By studying participants daily across an entire month, we were able to identify habitual patterns that may advance unhealthy metabolic behavior. Accurate assessment of the circadian phase also distinguishes this study from prior research. The synthesis of real-world observations with precise laboratory data enabled us to identify a novel potential risk factor for weight gain and disease: caloric intake relative to the central circadian phase. Previous observations have shown that eating after 2000 in humans is associated with higher BMI (42) and that restricting eating to typical waking hours in humans and rodents decreases weight (14, 30, 43). We here show how these observations are more directly correlated with circadian timing rather than clock time.
A potential mechanism for increased body fat in response to later meal timing may be a decreased thermic effect of food (TEF) (5, 44, 45), which is the energy expended in response to a meal. In controlled laboratory settings using whole-room indirect calorimetry, the TEF response to a meal consumed at ~2230 decreased by ~4% relative to the same meal at ~1830 (5). Other findings also indicate a possible time-of-day variation in the TEF (44). Specifically, consumption of a snack containing 20% of the total daily intake at 0100 produced a smaller TEF than the same snack at 0900 and 1700 (44). Of special relevance here, given the associations of body composition with meal timing relative to the circadian phase and not clock time, we previously showed the morning-evening difference in the TEF was caused primarily by the circadian system (45). Thus, one possible consequence of eating closer to or after DLMO may be a lower TEF response, which would contribute to a positive energy balance and weight gain over time. When we examined the percentage of total daily calories consumed from 4 h before DLMO until sleep onset, a time in which we would expect the TEF to be decreased, we found it was significantly associated with increased body fat. Although we did not have a direct measurement of energy expenditure or energy balance, making it difficult to demonstrate causality (i.e., that eating at a later circadian phase influences body composition), future work should take into account actual measures of energy expenditure in response to real-world food choices.
The absence of a relation between sleep duration and percentage of body fat in our data was unexpected, because insufficient sleep has been associated with higher weight (39, 46, 47). However, in those previous studies, when sleep is restricted in controlled laboratory settings and food is provided ad libitum, participants increase their caloric intake (48–50) to exceed the additional energy demands required to maintain wakefulness (5), and this excess consumption of calories occurs after dinner and closer to or after DLMO (49, 51). This eating pattern mirrors what we observed in this real-world setting when food was easily accessible in the college environment, because the shortest sleepers consumed their last daily calories closer to or after DLMO. Thus, short sleep may not directly contribute to weight gain per se, but rather when sleep is shortened because of a delayed bedtime or night and/or shift work, it may increase food consumption and enable dietary intake closer to DLMO.
Conversely, the weight loss observed during time-restricted feeding schedules (30) may also result from a change in the timing of consumption relative to DLMO. It was notable in the current study with well-characterized behaviors that although we did see a relation between the circadian timing of caloric intake and body composition, we did not see any associations between social jetlag and BMI, as have been previously reported (35). It is possible that our limited sample size did not provide sufficient power for a significant correlation between social jetlag and body composition. Alternatively, the timing of meals with respect to DLMO may have been the underlying mechanism in the correlations observed in the literature, and social jetlag may be a proxy measure for individuals eating later meals. Further, we did not see a relation between eating duration and body composition, similar to what has been reported in the literature (30). When eating durations are restricted, however, weight loss ensues (30). We again hypothesize that one underlying mechanism for this weight loss may be reducing the amount of calories consumed close to DLMO timing, because participants in the current study with longer eating durations ate a greater number of calories closer to DLMO. Before the translation of this work to clinical settings, randomized controlled trials based on our findings are needed. One such study would include altering the timing of meals of the exact same food content in relation to melatonin timing (e.g., providing meals within 4 h of melatonin or restricting calories to when melatonin concentrations are low).
Our study has several limitations that need to be considered in future work. First, we cannot dismiss the potential for underreporting of calories consumed or participants changing their eating habits during the meal-tracking protocol. To help avoid underreporting, the meal-tracking protocol was designed to occur when participants were in the midst of 30 d of data collection, reducing potential alteration of habitual patterns due to novelty. Furthermore, participants who recorded ≤2 meals within a 24-h period were sent an e-mail to confirm they had recorded all food or beverages consumed for that day. Second, and as noted above, we did not have a direct measure of energy expenditure to capture the full scope of energy balance. We attempted to account for this by including activity (measured as percentage of days with exercise) into our regression models. Third, across the 30 d of data collection, we only had 1 overnight assessment of the circadian phase, thereby limiting our ability to identify the relation between slight changes in the daily timing of DLMO and the timing of food consumption for each meal. However, because typical room lighting at night can produce only a small shift of ±1 h in the circadian phase (52), we do not anticipate a shift significantly impaired our conclusions. Lastly, we acknowledge that our population of college-aged individuals may not be representative of the entire population in food choice or timing. Our college population most likely had a delayed circadian phase compared with children and adults (18–20). We note that those other populations, who are expected to have earlier circadian timing, may be more vulnerable to the effect of eating meals at a later clock hour, more likely on weekends (30), because it would be closer to their (earlier) melatonin onset. Other populations with known late eating are night and shift workers, who also have increased rates of obesity (23). Examination of the timing of caloric consumption relative to melatonin onset in non–college-aged populations is needed to extend our findings.
In summary, our findings are the first, to our knowledge, to highlight eating patterns in real-world settings by using advanced food diary techniques and reveal that the timing of caloric consumption relative to melatonin onset is associated with increased body fat percentage, whereas caloric intake, the macronutrient content of the calories consumed, the amount of sleep obtained, and clock hour of the caloric consumption were not associated with body composition. These findings emphasize the importance of considering internal circadian timing of food consumption, in addition to the traditional risk factors of diet and exercise, for understanding healthy metabolic behavior, particularly as our society moves toward individualized health care strategies.
Acknowledgments
We thank the MIT Media Laboratory Affective Computing and the Brigham and Women’s Hospital Center for Clinical Investigation staff.
The authors’ responsibilities were as follows—AWM, CAC, and EBK: designed and conducted the research, analyzed the data, wrote the manuscript, and had primary responsibility for the final content; MG and FAJLS: designed the research, analyzed the data, and wrote the manuscript; AJKP, LK, KY, and LKB: conducted the research, analyzed the data, and wrote the manuscript; and all authors: read and approved the final manuscript. CAC has received consulting fees from or served as a paid member of scientific advisory boards for Bose Corporation, Boston Celtics, Boston Red Sox, Columbia River Bar Pilots, Institute of Digital Media and Child Development, Klarman Family Foundation, Koninklijke Philips Electronics, N.V., Samsung Electronics, Sleep Multimedia Inc., Vanda Pharmaceuticals, and V-Watch/PPRS. CAC has also received education/research support from Cephalon Inc., Mary Ann & Stanley Snider via Combined Jewish Philanthropies, Optum, Philips Respironics Inc., ResMed Foundation, San Francisco Bar Pilots, Schneider Inc., and Sysco and has received lecture fees from Annual Congress of the German Sleep Society (DGSM), CurtCo Media Laboratories LLC, Global Council on Brain Health/AARP, Harvard School of Public Health, Integritas Communications Group, Maryland Sleep Society, National Sleep Foundation, University of Michigan, and Zurich Insurance Company Ltd. The Sleep and Health Education Program of the Harvard Medical School Division of Sleep Medicine (which CAC directs) has received educational grant funding from Cephalon Inc., Jazz Pharmaceuticals, Takeda Pharmaceuticals, Teva Pharmaceuticals Industries Ltd., Sanofi, Inc., Sepracor Inc., and Wake Up Narcolepsy. He is the incumbent of an endowed professorship provided to Harvard University by Cephalon Inc. and holds a number of process patents in the field of sleep/circadian rhythms (e.g., photic resetting of the human circadian pacemaker). Since 1985, CAC has also served as an expert on various legal and technical cases related to sleep and/or circadian rhythms, including those involving the following commercial entities: Bombardier Inc., Continental Airlines, FedEx, Greyhound, and United Parcel Service. CAC owns or owned an equity interest in Lifetrac Inc., Somnus Therapeutics Inc., and Vanda Pharmaceuticals, and received royalties from McGraw Hill, Houghton Mifflin Harcourt, and Philips Respironics Inc. for the Actiwatch-2 and Actiwatch-Spectrum devices. FAJLS has received lecture fees from Bayer HealthCare, Sentara Healthcare, and Philips. EBK has received travel reimbursement from the Sleep Technology Council, Wire In-Brain Conference, Free Health LLC, Employer Health Benefit Congress, and the Associated Professional Sleep Society, and has served as consultant in cases involving transportation safety and sleep deprivation. The remaining authors had no conflicts to disclose.
REFERENCES
Articles from The American Journal of Clinical Nutrition are provided here courtesy of American Society for Nutrition
Full text links
Read article at publisher's site: https://doi.org/10.3945/ajcn.117.161588
Read article for free, from open access legal sources, via Unpaywall:
https://academic.oup.com/ajcn/article-pdf/106/5/1213/24209740/ajcn161588.pdf
Citations & impact
Impact metrics
Article citations
The metabolic and circadian signatures of gestational diabetes in the postpartum period characterised using multiple wearable devices.
Diabetologia, 12 Nov 2024
Cited by: 0 articles | PMID: 39531039
Late eating is associated with poor glucose tolerance, independent of body weight, fat mass, energy intake and diet composition in prediabetes or early onset type 2 diabetes.
Nutr Diabetes, 14(1):90, 25 Oct 2024
Cited by: 0 articles | PMID: 39455572 | PMCID: PMC11511895
The organization of sleep-wake patterns around daily schedules in college students.
Sleep, 47(9):zsad278, 01 Sep 2024
Cited by: 0 articles | PMID: 37930792 | PMCID: PMC11381563
Preventing and treating childhood obesity by sleeping better: a systematic review.
Front Endocrinol (Lausanne), 15:1426021, 19 Sep 2024
Cited by: 0 articles | PMID: 39363896 | PMCID: PMC11446760
Review Free full text in Europe PMC
Older adults exercising ON TIME: protocol for a randomized controlled cross-over study to assess the effect of physical activity timing on insomnia severity.
Trials, 25(1):523, 05 Aug 2024
Cited by: 0 articles | PMID: 39103937 | PMCID: PMC11299269
Go to all (189) article citations
Data
Data behind the article
This data has been text mined from the article, or deposited into data resources.
BioStudies: supplemental material and supporting data
Clinical Trials
- (2 citations) ClinicalTrials.gov - NCT02846077
Similar Articles
To arrive at the top five similar articles we use a word-weighted algorithm to compare words from the Title and Abstract of each citation.
Circadian timing and alignment in healthy adults: associations with BMI, body fat, caloric intake and physical activity.
Int J Obes (Lond), 41(2):203-209, 31 Oct 2016
Cited by: 37 articles | PMID: 27795550 | PMCID: PMC5296236
Caloric and Macronutrient Intake Differ with Circadian Phase and between Lean and Overweight Young Adults.
Nutrients, 11(3):E587, 11 Mar 2019
Cited by: 29 articles | PMID: 30862011 | PMCID: PMC6471585
The Effect of Exogenous Melatonin on Eating Habits of Female Night Workers with Excessive Weight.
Nutrients, 14(16):3420, 19 Aug 2022
Cited by: 3 articles | PMID: 36014925 | PMCID: PMC9412377
Chronotype Differences in Body Composition, Dietary Intake and Eating Behavior Outcomes: A Scoping Systematic Review.
Adv Nutr, 13(6):2357-2405, 01 Dec 2022
Cited by: 23 articles | PMID: 36041181 | PMCID: PMC9776742
Review Free full text in Europe PMC
Funding
Funders who supported this work.
NCRR NIH HHS (1)
Grant ID: UL1 RR025758
NHLBI NIH HHS (4)
Grant ID: R01 HL128538
Grant ID: K24 HL105664
Grant ID: T32 HL007901
Grant ID: R01 HL114088
NIA NIH HHS (1)
Grant ID: P01 AG009975
NIGMS NIH HHS (1)
Grant ID: R01 GM105018