Abstract
Free full text

Use of dual energy computed tomography to measure skeletal-wide marrow composition and cancellous bone mineral density
Abstract
Temporal and spatial variations in bone marrow adipose tissue (MAT) can be indicative of several pathologies and confound current methods of assessing immediate changes in bone mineral remodeling. We present a novel dual energy computed tomography (DECT) method to monitor MAT and marrow corrected volumetric BMD (mcvBMD) throughout the body. Twenty-three cancellous skeletal sites in 20 adult female cadavers aged 40–80 years old were measured using DECT (80kVp and 140kVp). vBMD was simultaneous recorded using QCT. MAT was further sampled using MRI. Thirteen lumbar vertebrae were then excised from the MRI-imaged donors and examined by microCT.
After MAT correction throughout the skeleton, significant differences (p < 0.05) were found between QCT-derived vBMD and DECT-derived mcvBMD results. McvBMD was highly heterogeneous with a maximum at the posterior skull and minimum in the proximal humerus (574 mg/cc and 0.7 mg/cc, respectively). BV/TV and BMC have a nearly significant correlation with mcvBMD (r = 0.545, p=0.057 and r = 0.539, p=0.061, respectively). MAT assessed by DECT showed a significant correlation with MRI MAT results (r = 0.881, p<0.0001). Both DECT- and MRI-derived MAT had a significant influence on uncorrected vBMD (r = −0.86 and r = −0.818, p≤0.0001, respectively). Conversely, mcvBMD had no correlation with DECT- or MRI- derived MAT (r = 0.261 and r = 0.067).
DECT can be used to assess MAT while simultaneously collecting mcvBMD values at each skeletal site. MAT is heterogeneous throughout the skeleton, highly variable, and should be accounted for in longitudinal mcvBMD studies. McvBMD accurately reflects the calcified tissue in cancellous bone.
1. Introduction
Cancellous bone can have sudden, drastic, and simultaneous changes in both calcified and marrow adipose tissue (MAT) [1, 2]. These changes can arise throughout the entire skeleton and be caused by a systemic stimulus or specific insult [3, 4]. Such responses are common from cytotoxic treatments such as radiation therapy and chemotherapy [5, 6]. MAT variations have recently been shown to indicate other skeletal and metabolic pathologies [7]. Scheller et al. have shown that in the mammalian skeleton, subsections of MAT can behave differently in response to a stimulus even within a single bone [8]. Identifing areas of global minima or comparing cancellous bone of different sites could highlight areas for further examination. Therefore, it is necessary to employ a modality that can accurately measure cancellous bone metrics throughout the entire body. Although the use of quantitative computed tomography (QCT) is useful to evaluate 3D cancellous bone, it has trouble comparing skeletal sites with significantly different bone marrow composition. For instance, hematopoietic bone marrow tissue has a higher linear attenuation coefficient than MAT. Therefore, sites with significant quantities of MAT compared to sites of hematopoietic tissue would show a lower vBMD, even if the same amount of mineralized tissue were present [9–12].
We have already shown dual energy computed tomography’s (DECT) ability to simultaneously measure MAT quantities[13] and provide marrow corrected vBMD (mcvBMD) values that better reflect mineralized tissue [14]. Whole-body CT imaging is already commonly prescribed for the cancer patient for staging, treatment, and treatment follow-up (e.g. as part of a PET-CT procedure). DECT is feasible for clinical studies since, against conventional wisdom, doses from DECT have been shown to be similar to single energy CT [15, 16]. A quick, accurate, and efficient method to examine both MAT and marrow corrected vBMD would be beneficial to the cancer survivor [17]. The current study is the first to characterize both MAT and accurate vBMD of mineralized tissue throughout the whole body.
The primary goal of the current study is to accurately image the entire skeleton in order to characterize the heterogeneity of cancellous bone in terms of its bone mineral density and marrow composition (percentage of marrow space occupied by adipocytes). All aims were conducted using 20 female cadavers, permitting excision of cancellous bone for ex vivo high resolution imaging.
2. Materials and Methods
2.1. Subjects
Approval for study was given by the University of Minnesota Anatomy Bequest program in accordance with the Minnesota Darlene Luther Revised Uniform Anatomical Gift Act. Over a 10 month period, twenty recently deceased human donors were accepted in the study. Women between the ages of 40 and 80 years old were selected to reduce confounding factors associated with gender and youth (e.g. unfused epiphyses) while still providing a range of marrow compositions. Donors were included if they were less than 190cm tall, had no history of anti-osteoporotic treatment, and could be imaged within 24 hours postmortem. The height restriction ensured that the body could fit within the longitudinal length of the CT scanner.
The mean (SD) age for all 20 subjects was 62 (13) years. The mean (SD) height and weight for subjects was 165cm (7cm) and 63kg (20kg), respectively, resulting in a BMI of 23.0 (6.4 kg/m2). Causes of death included cancer (n=11), Parkinson Disease (n=2), chronic obstructive pulmonary disease (n=2), hepatitis (n=2), sudden cerebrovascular accident (n=2), and heart failure (n=1). One subject sustained a proximal femur fracture approximately one month before death but was never surgically treated, while another donor had prior bilateral knee replacement and shoulder reconstructive surgery. The high density material and resulting streak artifact made assessment of those regions unattainable in those two subjects. Any areas of obvious disease (e.g. metastases) or low signal-to-noise ratio were similarly avoided. In total, 14 of 460 sites were excluded from the analysis, mainly from the distal radius due to poor image quality (n=8).
2.2. Imaging
Within 20 hours of death, bodies were taken to the radiology department at the University of Minnesota Medical Center – Fairview for whole body dual energy CT scanning. Imaging was obtained using a Siemens® SOMATOM Definition Flash Dual Source CT scanner (Siemens Healthcare, Forchheim, Germany) at energies of 80kVp, 500mm SFOV, and 306–308mAs for Source A, and 140kVp, 328mm SFOV and 110–150mAs with a tin filter for Source B. During imaging, subjects were placed in a supine position with arms at the sides. The SOMATOM DECT unit was calibrated for both energies using the Mindways Software, Inc. (Mindways Software, Austin, TX, USA) QCTPro calibration Phantom. The table height was varied to ensure that the patient was in the center of the imaging field, regardless of body size. A calibration was performed for each table height.
2.3. Region of Interest analysis
Using anatomic landmarks and rigid registration software developed by Mindways Software, Inc. (Austin, Texas, USA), regions of interest (ROIs) were placed in the same location on the 80kVp and Sn140kVp CT scans. 446 cancellous bone locations between 20 donors were analyzed (Fig. 1) including the skull, twelve sites from the spine, two from the arm, three from the hip, two from the knee, and three from the ankle/foot.
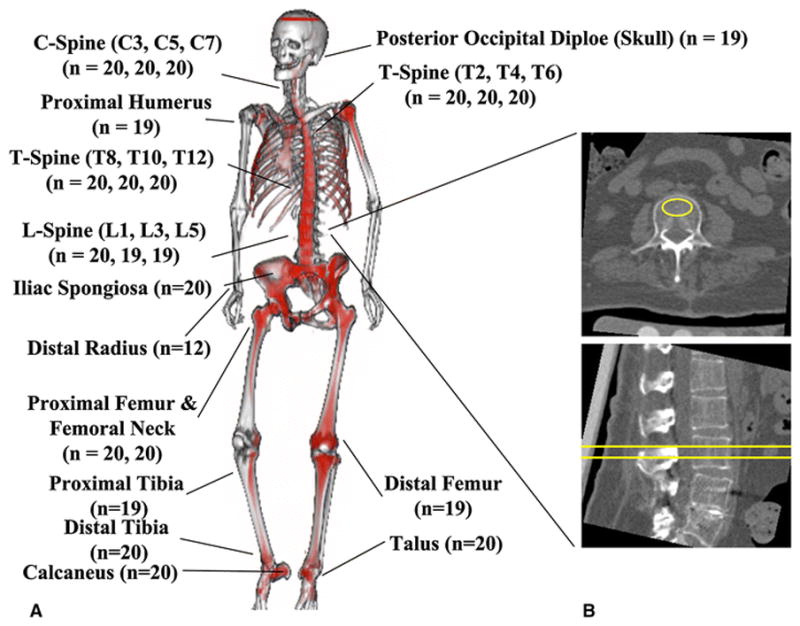
23 regions of interest representing areas of analyzed cancellous bone. a) First conceptual illustration of whole-body DECT image where less fatty marrow (i.e. more hematopoietically active) regions are highlighted in red and merged with a single energy CT skeletal contour. Apparent asymmetry caused by resampling limitations. b) Sample ROI placement for L3 (yellow)
The size of each ROI depended on the bone being measured but ranged from a cylinder of 20 mm2 × 3 mm for the posterior skull to 300 mm2 × 9 mm for the lumbar spine (Fig. 2). Care was taken to exclude areas of prior fracture. ROIs were placed at each skeletal site for all donors and ROI sizes were recorded. Vertebrae were analyzed at the center of the vertebral body in the superior-inferior axis.
2.4. Basis Material Composition
To assess material composition of bone, a commercially available CT calibration phantom was included in each imaging procedure. The phantom was composed of five rods of different water and K2HPO4 concentrations (Mindways Software, Inc. Austin, TX) and placed underneath the lumbar spine and pelvis of the donor. Aqueous K2HPO4 is a commonly used physical standard for assessing bone mineral density (BMD). Relative to this standard, the basis material for bone as a function of BMD (ρK2HPO4) is described by the following relationship derived from conventional methods for estimating bone mineral density from single-energy CT data:
The factor of 0.2174 appearing in this equation describes how water is displaced from a fixed volume by way of addition of K2HPO4.
Using the publically available XCOM database to estimate energy-dependent, mass attenuation coefficients, we estimated the average atomic composition for basis materials (ρH2O, ρK2HPO4) [18]. We then employed the principle that when scanned with two different energies in the diagnostic range, the x-ray attenuation of biologic tissue can be described by the linear combination of the x-ray attenuation properties of H2O and K2HPO4 [19, 20]. We estimated the average basis material for identically placed ROIs in a phantom acquired at 80 kVp and 140 kVp using the following equations:
where CT80 and CT140 were the average CT values within the defined ROIs and βH2O,80, βH2O,140, βK2HPO4,80, βK2HPO4,140 were calibration slopes determined from CT calibration phantom measurements.
The International Commission on Radiation Units and Measures has documented average atomic compositions of adipose tissue, yellow and red marrow (0.91 g/cm3, 0.93 g/cm3, and 1.03g/cm3, respectively)[21]. When combined with XCOM [18], these values were used as a reference range for the basis material compositions in our ROIs. This information, along with the aqueous K2HPO4 bone density standard described earlier was used to define a window of viable bone density estimates spanning a range of marrow compositions from 0% to 100% MAT. The porosity for this bone model is defined relative to the aqueous K2HPO4 bone density standard with 0 mg/cm3 bone density on this scale assumed to correspond to a porosity of 1 and 1200 mg/cm3 bone density assumed to correspond to a porosity of 0.
This technique of marrow composition estimation has been validated by a previous study [13]. Bone marrow composition and mcvBMD values were then estimated at each skeletal site using calibration slopes calculated for the whole body, similar to our previous investigation [14].
2.5. MRI analysis
In order to validate MAT assessment by DECT, immediately following DECT imaging, five random donors without history of fracture or prosthesis were scanned with Siemens MRI for marrow validation as previously described [22]. These donors were 47, 53, 54, 62, and 68 years old with a BMI of 20.7, 22.9, 22.1, 22.8, and 23.5, respectively. Briefly, after DECT imaging, the donor was imaged on a 3 Tesla MRI scanner (Tim TRIO, Siemens Medical Solutions, Malvern, PA, USA) using a three-point Dixon technique. Images were acquired in the supine position using the vendor’s spine array receive coil combined with an anterior body matrix coil centered over the lumbar spine. After localizer scans, a series of 3D gradient echo images were acquired with TR = 9 microseconds, flip = 1°, matrix 448×266, 256 slices, 1.5 mm slice thickness, acquisition time 225 seconds. Three consecutive images were acquired with TE = 2, 3, and 4 microseconds. The raw image data were reconstructed offline in Matlab (MathWorks Inc., Natick, MA) using the method of Berglund et al. [23], as implemented in the ISMRM Fat-Water Toolbox [24], and using a 9-peak model for the lipid spectral peaks [25]. The water and fat images produced by the reconstruction were used to calculate the signal fat fraction (sFF), defined as sFF=fat/(fat+water). 20 vertebrae were imaged with adequate resolution and acceptable noise. Manual rigid registration was performed to anatomically match MRI and DECT/QCT ROIs.
2.6. Ex vivo analysis
Less than 12 hours after MRI imaging, the lumbar vertebrae, L1–L5, were removed, separated, and placed in formalin. Afterwards, 13 individual vertebrae were imaged using a Siemens Inveon® MicroCT at the Center for Magnetic Resonance Research at the University of Minnesota. The μCT parameters used included 1.3x magnification, 4 binning, 0.5 mmAl filter, and 80kVp to produce 95 μm/voxel images (Fig. 2b).
μCT DICOM images were analyzed using the BoneJ plugin [26] on ImageJ[27]. A threshold of approximately 0.8 grams hydroxyapatite/cc was used to separate out mineralized tissue. Manual rigid registration was performed to anatomically match microCT and DECT/QCT data. A region of interest similar to the DECT imaging was applied in identical locations of the lumbar vertebrae. Bone mineral content (BMC) and bone volume per total volume (BV/TV) were measured.
2.7. Statistical Analysis
Mean QCT-derived vBMD, MAT, and mcvBMD values were determined at 446 skeletal sites. To compare in vivo imaging results (i.e. QCT and DECT) to ex vivo imaging results (i.e. BMC and BV/TV), as well as to MAT results (i.e. DECT-derived MAT and MRI-derived sFF), spearman nonparametric correlation coefficients and two-tailed P value were were determined using Graph Pad Prism software (Version 6.02). Significance of within-donor differences in QCT-derived vBMD and mcvBMD were assessed by a paired t-test for each skeletal site.
3. Results
When comparing MAT between DECT and MRI, regions of interest of matching size were created in the vertebral bodies and anatomically placed in approximately identical locations using manual rigid registration. The sFF (i.e. the intensity of marrow fat as assessed by MRI) of 20 vertebral bodies were recorded. Between the 5 patients, the values ranged from 23% to 79% marrow fat. Variations within each individual lumbar spine were far less with an average difference of 14% between max and min sFF. DECT-derived MAT was shown to be highly correlated with sFF results (r = 0.881, p < 0.0001). There was no correlation identified between MAT, assessed by either MRI sFF or DECT, and mcvBMD (r = 0.067 and = 0.261, respectively, p>0.3). However, a significant inverse correlation was observed between MAT, assessed by either MRI sFF or DECT, and uncorrected vBMD (r = −0.818 and = −0.86, respectively, p<0.0001) (see Fig. 3a).
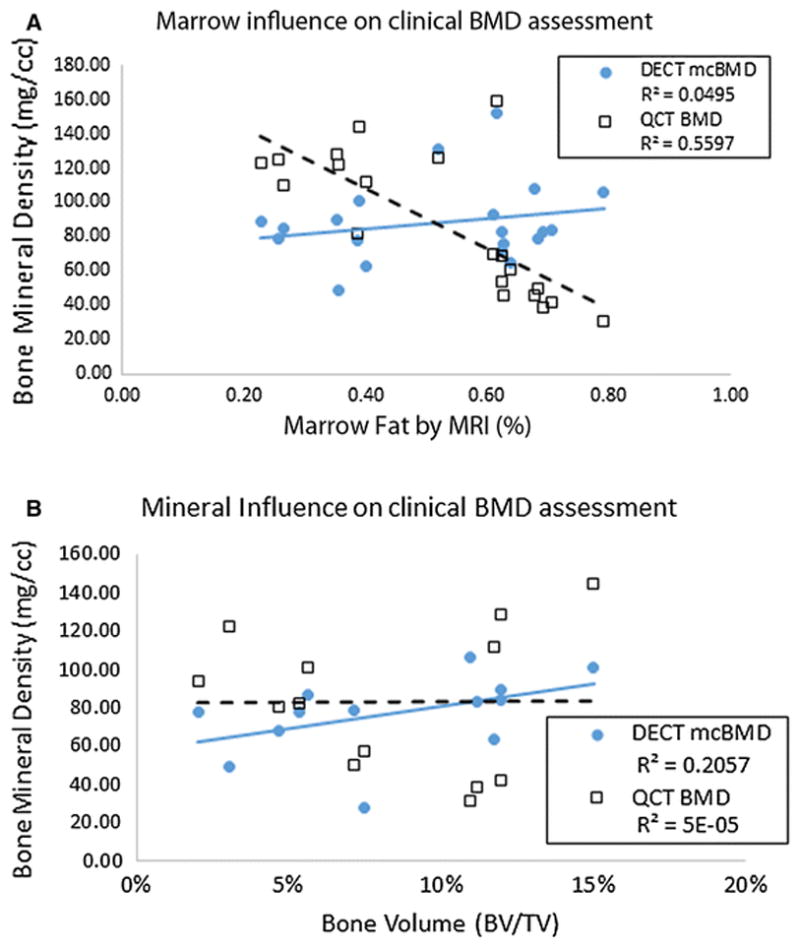
Marrow adiposity and mineral influences on in vivo imaging of cancellous bone a) percent of marrow adipose tissue (MAT) in marrow compartment assessed by MRI for 20 vertebral bodies. MAT is compared to volumetric BMD derived by marrow-corrected DECT and QCT. QCT-derived vBMD shows a significant inverse correlation with marrow fat than DECT-derived marrow corrected vBMD and b) Ex vivo analysis of bone volume to total volume (BV/TV) using μCT taken from 13 of the 20 vertebral bodies. Notice better correlation with BV/TV for DECT than QCT
The results from ex vivo analysis revealed heterogeneous characteristics among the 13 lumbar samples. BV/TV values ranged from 3.1% to 15% and BMC ranged from 0.016 to 0.223 mg of hydroxyapatite. The BV/TV and BMC values for the 13 vertebrae were compared to the vBMD and mcvBMD values of the QCT and DECT, respectively. In Fig. 3b, a slightly better correlation with BV/TV is seen when vBMD is corrected for marrow composition than when assessed by QCT (r = 0.545, p = 0.057 and r = 0.058, p = 0.850, respectively). When BMC was correlated to DECT and QCT vBMD, there was a similar noteable difference (r = 0.539, p=0.061 and r = 0.093, p=0.765 respectively, Spearman correlation and two tailed p value estimation).
For the 23 skeletal sites, the average uncorrected vBMD using 140kVp QCT ranged from 783 mg/cm3 to −44 mg/cm3 (skull and proximal humerus, respectively). Fig. 4a compares these results to the marrow-corrected DECT dataset. QCT significantly overestimated the BMD for the vertebral bodies of the cervical and thoracic spine. By contrast, the BMD values were lower when assessed by QCT than by DECT in more caudal sites (e.g. tibia). At 14 of the 23 skeletal sites, the average difference between QCT-derived vBMD and mcvBMD was significant at the p < 0.05 level (marked with * in Fig. 4a). MAT ratios at each skeletal site are shown in Fig. 4b, excluding the posterior occipital diploë due to large uncertainty with small marrow space. At sites with a higher ratio of yellow to red marrow, the difference between mean mcvBMD and vBMD had a high correlation with the mean marrow adiposity of each site (r = 0.93, p <0.0001).
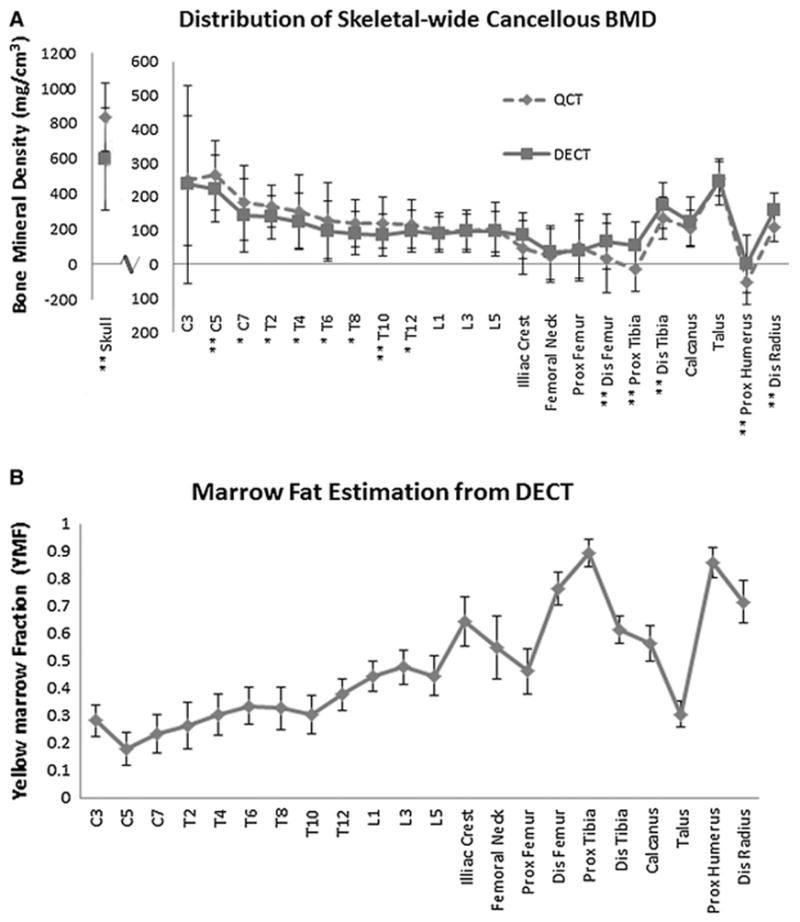
Distribution of cancellous bone throughout entire skeleton. Error bars are standard deviation for 20 female donors. a) Average Trabecular Bone Mineral Density distribution for 23 skeletal sites (skull separately). (* = p<0.05; ** = p<0.01). Notice lower BMD estimations in proximal tibia and humerus for QCT than DECT. b) Average Marrow fat (MAT) distribution from cervical spine to calcaneus
Higher marrow fat ratios were seen in proximal tibia and humerus. These two sites were also the only values that QCT determined to have a negative BMD (−11.9 and −44.3 mg/cc, respectively). Excluding the skull, the average (±SD) mcvBMD derived by DECT was 106 mg/cm3 (±85.6 mg/cm3) with the densest site being the talus (229 mg/cm3). We also observed a gradual decline in mean mcvBMD in the axial skeleton from the top of the spinal column to the bottom (233 mg/cm3 for C3; 93 mg/cm3 for L5). Local differences in mcvBMD were also seen within individual bones such as the femur and tibia. Cancellous mcvBMD was lower in the proximal femur than the distal femur (40 mg/cm3 and 62 mg/cm3, respectively), and significantly lower (p < 0.001) in the proximal tibia than distal tibia (50 mg/cm3 and 158 mg/cm3, respectively).
4. Discussion
Traditionally, clinicians assess fracture risk by measuring areal BMD using DXA. However, DXA superimposes thick cortical with trabecular bone, and cannot quantify marrow fat. Since radiation therapy and chemotherapy cause rapid increases in marrow fat, uncorrected BMD measurements can lead to significant errors in BMD when measured by DXA or QCT. In this study, we imaged the entire skeleton in vivo and assessed marrow composition using DECT. A range of MRI sFF values (i.e. 23% to 79%), were used to validate the DECT modality for MAT assessment. We demonstrate that vBMD and MAT vary across skeletal sites. Additionally, we show that marrow fat, determined by either MRI sFF or DECT, is inversely correlated with vBMD when measured by QCT. This suggests an influence of MAT on uncorrected vBMD. We propose that DECT can be a useful imaging method to discern the adverse skeletal effects of cancer therapy. A more sensitive and accurate tool such as DECT might lead to greater understanding of the site-specific changes in fracture risk, such as greater risk of pelvic fractures after radiation therapy for gynecologic malignancy.
An increasing number of studies have investigated calcified cancellous tissue separate from marrow composition using high resolution imaging (e.g. peripheral quantitative computed tomography, micro-computed tomography (μCT), and quantitative ultrasound) [28–30]. However, their work is limited to ex vivo samples and peripheral skeletal sites. Poole et al. has established the importance of assessing 3D skeletal parameters for the evaluation of structural strength [31]. Another QCT-based study attempted to correct the vBMD results with microCT values [32]. However, both studies were not concerned with the influence of inherent marrow composition. The current work furthers the use of advanced CT techniques to simultaneously measure the metabolic changes in the two compartments of cancellous bone.
Our study adds to a growing body of knowledge by extending the observations to the entire skeleton in vivo and assess marrow composition using DECT (Fig. 1a). A method of assessing the two components of cancellous bone throughout the skeletal system might improve the ability to identify and monitor patients at high risk of fractures. A previous attempt to assess skeletal-wide heterogeneity of more metabolically-active (i.e. cancellous) bone was unable to separate adjacent skeletal regions, much less cortical from cancellous bone, due to technical limitations [33]. More recently, work by Frost et al.[3] and Yagi et al.[34] showed the functional heterogeneity of both osteoblastic activity and active marrow using 18F labeled FDG and NaF. Recent improvements in CT scanners, including dual energy, large bore, and larger couch travel, have permitted accurate and efficient investigations of cancellous bone throughout the whole body, including quantification of marrow fat [35, 36]. Correcting cancellous bone for MAT (i.e. mcvBMD) resulted with a moderate correlation to both BV/TV and BMC whereas no correlation was observed between either BV/TV or BMC and uncorrected vBMD. Therefore, using DECT to monitor bone health has advantages of being able to identify vBMD of calcified tissue while simultaneously characterizing marrow composition.
Although CT imaging increases radiation exposure to patients, whole body CT scans are already performed as part of a positron emission tomography (PET)/CT. Also, since radiation treatment planning CT scans are regularly given as part of the diagnosis, treatment and follow-up process, DECT could become very useful to the study of cancer survivors’ bone health. To date, such images are taken with single energy CT and only used for anatomical referencing. Another benefit of whole body DECT would be for applications such as evaluating radiation dose from cancer treatment to the active marrow without assuming marrow composition from surrogate anatomy [37]. We expect these imaging studies to soon take advantage of reduced radiation exposure from DECT scans [38–40] and be able to monitor bone health.
4.1. Limitations
The present study was a feasibility study and did not have a high sample size. Although the correlation between DECT and MRI for MAT assessment is good, our study was limited in only validating regions with high marrow content, i.e. the lumbar vertebrae. We limited participants to subjects who had pre-arranged anatomical donation contracts with the University of Minnesota Bequest Program. Only Caucasian women were imaged, and cannot state whether skeletal heterogeneity would also exist in men or in other racial groups. Since we were performing a feasibility study, we did not restrict our cancer donors to those with a certain form of cancer, certain type of chemotherapy, or a standard dose or location of radiation therapy.
5. Conclusion
Bone mineral density and marrow composition vary among cancellous bone sites along the entire skeleton. With the ability to correct for marrow composition, DECT imaging of the entire human body can accurately assess the calcified vBMD of cancellous bone. The trend of cancellous vBMD among skeletal sites was similar for QCT and DECT. However, marrow composition heterogeneity did reveal dynamic differences in some skeletal sites. Further validation with periphery skeletal sites would increase significance of DECT whole body assessment for bone health. These findings suggest potential for application in further prospective studies where accurate and longitudinal vBMD and marrow assessments are necessary (e.g. age related studies). This method could also be beneficial to longitudinal clinical studies where monitoring abscopal bone health changes in short time periods is desirable.
Acknowledgments
This work was supported by the National Institute of Health grants (1R01CA154491-01; RO3 AR055333-02), National Institute of Child Health and Human Development (1K12-HD055887-01), the by the National Center for Advancing Translational Sciences of the National Institutes of Health Award Number 8UL1TR000114-02, and Cancer Center Support Grant P30 CA77398. Other grant support includes the Minnesota Medical Foundation and seed grants from the university (Academic Health Center, Grant in Aid, Masonic Cancer Center breast cancer research). Susanta K Hui is a scholar of the BIRCWH (Building Interdisciplinary Careers in Women’s Health) program. This work was supported by Japan Society for the Promotion of Science Core to Core Program (23003). Karen Hansen serves as a medical monitor and consultant to Deltanoid Pharmaceuticals. All other authors have no conflicts of interest.
The authors wish to thank the women who donated their bodies for the advancement of education and research.
Footnotes
5.1. Conflicts of Interest
Karen Hansen is a medical monitor and consultant to Deltanoid Pharmaceuticals. Luke Arentsen, Masashi Yagi, Yutaka Takahashi, Ryan Shanley, Angela McArthur, Taiki Magome, Douglas Yee, Jerry Froelich, and Susanta Hui declare no conflict of interest.
References
Full text links
Read article at publisher's site: https://doi.org/10.1007/s00774-016-0796-1
Read article for free, from open access legal sources, via Unpaywall:
https://europepmc.org/articles/pmc5689468?pdf=render
Citations & impact
Impact metrics
Article citations
Multi-site phantomless bone mineral density from clinical quantitative computed tomography in males.
JBMR Plus, 8(10):ziae106, 03 Aug 2024
Cited by: 0 articles | PMID: 39224571 | PMCID: PMC11366047
The influence of contrast media on calcium-based imaging of the spine in dual-layer CT.
Sci Rep, 14(1):18898, 14 Aug 2024
Cited by: 0 articles | PMID: 39143146 | PMCID: PMC11324893
Young minds, deeper insights: a recap of the BMAS Summer School 2023, ranging from basic research to clinical implications of bone marrow adipose tissue.
Biol Open, 13(2):bio060263, 30 Jan 2024
Cited by: 0 articles | PMID: 38288785 | PMCID: PMC10855210
Semi-automatic proximal humeral trabecular bone density assessment tool: technique application and clinical validation.
Osteoporos Int, 35(6):1049-1059, 09 Mar 2024
Cited by: 0 articles | PMID: 38459138
Dual-energy computed tomography and micro-computed tomography for assessing bone regeneration in a rabbit tibia model.
Sci Rep, 14(1):5967, 12 Mar 2024
Cited by: 0 articles | PMID: 38472263 | PMCID: PMC10933353
Go to all (19) article citations
Similar Articles
To arrive at the top five similar articles we use a word-weighted algorithm to compare words from the Title and Abstract of each citation.
A phase I feasibility study of multi-modality imaging assessing rapid expansion of marrow fat and decreased bone mineral density in cancer patients.
Bone, 73:90-97, 20 Dec 2014
Cited by: 16 articles | PMID: 25536285 | PMCID: PMC4336831
Correction of QCT vBMD using MRI measurements of marrow adipose tissue.
Bone, 120:504-511, 21 Dec 2018
Cited by: 4 articles | PMID: 30583123
Evaluation of cross-sectional and longitudinal changes in volumetric bone mineral density in postmenopausal women using single- versus dual-energy quantitative computed tomography.
Bone, 112:145-152, 25 Apr 2018
Cited by: 11 articles | PMID: 29704696 | PMCID: PMC5970096
Marrow adipose tissue imaging in humans.
Bone, 118:69-76, 10 Jan 2018
Cited by: 29 articles | PMID: 29331301 | PMCID: PMC6039291
Review Free full text in Europe PMC
Funding
Funders who supported this work.
Cancer Center Support Grant (1)
Grant ID: P30 CA77398
NCATS NIH HHS (1)
Grant ID: UL1 TR000114
NCI NIH HHS (2)
Grant ID: R01 CA077398
Grant ID: R01 CA154491
NIAMS NIH HHS (1)
Grant ID: R03 AR055333
NICHD NIH HHS (1)
Grant ID: K12 HD055887
National Center for Advancing Translational Sciences (1)
Grant ID: 8ul1tr000114-02
National Institute of Child Health and Human Development (1)
Grant ID: 1k12-hd055887-01
National Institutes of Health (2)
Grant ID: 1R01CA154491-01
Grant ID: ar055333-02