Abstract
Free full text

In Vitro Comparison of Ceftolozane-Tazobactam to Traditional Beta-Lactams and Ceftolozane-Tazobactam as an Alternative to Combination Antimicrobial Therapy for Pseudomonas aeruginosa
ABSTRACT
Guidelines for the treatment of sepsis, febrile neutropenia, and hospital-acquired pneumonia caused by Pseudomonas aeruginosa include empirical regimens incorporating two antibiotics from different classes with activity against P. aeruginosa for select at-risk patients to increase the likelihood that the organism will be susceptible to at least one agent. The activity against P. aeruginosa and the rates of cross-resistance of ceftolozane-tazobactam were compared to those of the β-lactam comparators cefepime, ceftazidime, piperacillin-tazobactam, and meropenem alone and cumulatively with ciprofloxacin or tobramycin. Nonurine P. aeruginosa isolates were collected from adult inpatients at 44 geographically diverse U.S. hospitals. MICs were determined using reference broth microdilution methods. Of the 1,257 isolates collected, 29% were from patients in intensive care units and 39% were from respiratory sites. The overall rate of susceptibility to ceftolozane-tazobactam was high at 97%, whereas it was 72 to 76% for cefepime, ceftazidime, piperacillin-tazobactam, and meropenem. The rate of nonsusceptibility to all four comparator β-lactams was 11%; of the isolates nonsusceptible to the four comparator β-lactams, 80% remained susceptible to ceftolozane-tazobactam. Among the isolates nonsusceptible to the tested β-lactam comparators, less than half were susceptible to ciprofloxacin. By comparison, approximately 80% of the β-lactam-nonsusceptible isolates were susceptible to tobramycin, for overall cumulative susceptibility rates of 94 to 95%, nearly 10% higher than that of the ciprofloxacin–β-lactam combinations and approaching that of ceftolozane-tazobactam as a single agent. The rates of susceptibility to ceftolozane-tazobactam were consistently high, with little observable cross-resistance. Ceftolozane-tazobactam monotherapy performed at or above the level of commonly utilized combination therapies on the basis of in vitro susceptibilities. Ceftolozane-tazobactam should be considered for use in patients at high risk for resistant P. aeruginosa infection and as an alternative to empirical combination therapy, especially for patients unable to tolerate aminoglycosides.
INTRODUCTION
Pseudomonas aeruginosa is a notorious cause of nosocomial infection both within the United States and around the world. The CDC's National Healthcare Safety Network lists P. aeruginosa as the seventh most common cause of health care-associated infection, accounting for 8% of all reported cases (1), while other surveillance data from both U.S. and European centers place it even higher as the third most common isolate causing health care-associated infections in both intensive care unit (ICU) and non-ICU patients (18% and 12% of all isolates, respectively) (2). Within the ICU, P. aeruginosa has been implicated in 16% of nosocomial pneumonias, 10% of urinary tract infections, 10% of surgical site infections, and 4% of bloodstream infections (3) and was noted in one European study to be the pathogen responsible for the greatest burden of health care-acquired infections (4).
The mainstay of therapy against P. aeruginosa infections involves the administration of an active antibiotic of the β-lactam class; however, the propensity of P. aeruginosa to develop resistance to multiple antibiotic agents can pose significant treatment challenges. Resistance to β-lactams may result from a variety of mechanisms (Table 1) that may act alone or in combination to produce a phenotypic profile of resistance (5). Resistance to only a single β-lactam or to the entire class may be conferred depending on the mechanism. Large surveillance studies have demonstrated baseline rates of resistance to traditional β-lactams (e.g., cefepime, ceftazidime, piperacillin-tazobactam, and meropenem) of 15 to 20% or more, regardless of the agent selected (6,–8). Additionally, critically ill patients within the ICU are at increased risk of harboring resistant isolates, with these isolates having approximately 10% declines in susceptibility compared to those of isolates from ward patients (2).
TABLE 1
Mechanisms of Pseudomonas aeruginosa resistance to β-lactam antibiotics
Mechanism of resistance | Example(s) |
---|---|
Enzymatic inactivation via β-lactamase production | Expanded-spectrum β-lactamases (TEM, SHV, CTX-M, PER, VEB, GES, IBC, AmpC) and carbapenemases (KPC, IMP, VIM, SPM, GIM, OXA-40) |
Alterations in outer membrane permeability via porin alteration | Decreased production or loss of functional OprD |
Active efflux via overproduction of efflux pumps | mexAB-OprM, mexCD-OprJ, mexXY-OprM |
Given this risk for resistance, many international guidelines allow or advocate for the empirical use of two agents from different antibiotic classes with activity against P. aeruginosa in order to better capture a susceptible result (Table 2) (9,–17). In particular, the most recent guidelines for the treatment of ventilator-associated pneumonia (VAP) recommend combination therapy when the local rate of resistance of Gram-negative bacteria to an agent being considered for monotherapy exceeds 10% (9). These combination regimens have traditionally been composed of a β-lactam plus either an aminoglycoside or a fluoroquinolone. This results in expanded anti-P. aeruginosa activity, but at the cost of a potential increased risk for adverse effects, including nephrotoxicity and ototoxicity (aminoglycosides) or neuropathies and central nervous system effects (fluoroquinolones).
TABLE 2
International guidelines allowing empirical dual-coverage therapy against Pseudomonas aeruginosaa
Guideline (reference) | Organization | Yr | Recommendations |
---|---|---|---|
Management of adults with hospital-acquired and ventilator-associated pneumonia (9) | IDSA, ATS | 2016 | For VAP, 2 antibiotics with anti-P. aeruginosa activity are recommended if any of the following are present: (i) a risk factor increasing the likelihood of Gram-negative bacterial infection or antimicrobial resistance, (ii) >10% resistance of Gram-negative bacterial isolates on a hospital unit to an agent being considered for monotherapy, (iii) the lack of availability of local antimicrobial susceptibility rates for the ICU, and (iv) an increase in the risk of Gram-negative bacterial infection because of structural lung disease (i.e., bronchiectasis or cystic fibrosis). For HAP, 2 antibiotics with anti-P. aeruginosa activity are recommended if any of the following are present: (i) risk factors increasing the likelihood for P. aeruginosa or other Gram-negative bacterial infection, and (ii) patients are at high risk for mortality (e.g., need for ventilator support and septic shock). |
Guidelines for the management of adult lower respiratory tract infections (10) | ESCMID, ERS | 2011 | The use of 2 antibiotics with anti-P. aeruginosa activity is recommended for CAP patients requiring ICU or immediate care when risk factors for P. aeruginosa infection are present. |
Use of antimicrobial agents in neutropenic patients with cancer (11) | IDSA | 2010 | Additional antibiotics with anti-P. aeruginosa activity may be added for management of complications (e.g., hypotension and pneumonia) or if antimicrobial resistance is suspected or proven. |
Prevention and treatment of cancer-related infections (12) | NCCN | 2017 | Initial combination therapy should be considered for febrile patients at high risk for P. aeruginosa infections. Combination therapy may also be considered in complicated or resistant cases. |
European guidelines for empirical antibacterial therapy for febrile neutropenic patients (13) | ECIL | 2013 | A deescalation treatment approach with the empirical use of 2 antibiotics with anti-P. aeruginosa activity may be considered if one of the following criteria are present: (i) known prior colonization or infection with resistant pathogens, (ii) complicated presentation (e.g., severe sepsis, septic shock), (iii) high rates of resistant nonfermenters on the basis of local epidemiology, or (iv) carbapenem use within the past month. |
Diagnosis and management of prosthetic joint infection (14) | IDSA | 2013 | The use of 2 antibiotics with anti-P. aeruginosa activity may be considered on the basis of the clinical circumstances of the patient. |
Clinical practice guidelines for the diagnosis and treatment of native vertebral osteomyelitis in adults (15) | IDSA | 2015 | The use of 2 antibiotics with anti-P. aeruginosa activity may be considered. |
International guidelines for management of sepsis and septic shock (16) | SSC | 2016 | Supplemental use of an agent with activity against Gram-negative bacteria is recommended for critically ill septic patients at high risk of infection with multidrug-resistant pathogens (including P. aeruginosa). |
Combination antibiotic therapy for empirical and definitive treatment of Gram-negative bacterial infections (17) | SIDP | 2011 | The use of 2 antibiotics with anti-P. aeruginosa activity is reasonable for empirical treatment of suspected health care-associated infections, particularly when risk factors for multidrug-resistant organisms are present. |
Alternatively, new antibiotic agents with enhanced activity against P. aeruginosa may provide a promising substitute for combination therapy. Ceftolozane-tazobactam (Zerbaxa; Merck & Co., Kenilworth, NJ, USA) is a novel cephalosporin–β-lactamase inhibitor engineered to have increased stability against efflux and degradation by multiple classes of β-lactamases. Current FDA approvals for ceftolozane-tazobactam include complicated intra-abdominal infections in combination with metronidazole and complicated urinary tract infections, including pyelonephritis, with clinical trials evaluating its use for bacterial pneumonia currently in progress. Ceftolozane-tazobactam has demonstrated consistently high in vitro activity against P. aeruginosa in large surveillance studies, with overall rates of susceptibility being >90% (6).
The purpose of this study was to describe the susceptibilities of P. aeruginosa isolates to ceftolozane-tazobactam and to compare those to the susceptibilities to traditionally utilized β-lactams alone and the cumulative susceptibilities of each β-lactam used with either ciprofloxacin or tobramycin. Additionally, the rates of cross-resistance among the β-lactam comparators and to ceftolozane-tazobactam were assessed. The rates of susceptibility of isolates nonsusceptible to one, two, three, or all four comparator β-lactams to ceftolozane-tazobactam were determined as well.
RESULTS
A total of 1,257 P. aeruginosa isolates were evaluated. Approximately 29% of the isolates were obtained from patients residing in an ICU. Over one-third (39%) were isolated from respiratory sources, and 61% were isolated from nonrespiratory sources. Nonrespiratory sources included blood, wounds, and miscellaneous body fluids (e.g., ascites).
The rate of susceptibility to ceftolozane-tazobactam was >90% across all locations (ICU or ward) and sources (respiratory or nonrespiratory) (Table 3). Ceftolozane-tazobactam was the most active agent tested against P. aeruginosa, with the rates of in vitro susceptibility being 20 to 25% higher than those to cefepime, ceftazidime, piperacillin-tazobactam, or meropenem. The trends for susceptibility to ceftolozane-tazobactam tended to follow those to the other antibiotics, with ICU isolates being overall less susceptible than non-ICU isolates and respiratory isolates being less susceptible than nonrespiratory isolates. The least susceptible isolates were respiratory isolates from the ICU (92% were susceptible to ceftolozane-tazobactam); however, this decline in susceptibility was less pronounced than that for the β-lactam comparators, with the rates of susceptibility to ceftolozane-tazobactam being 32 to 42% higher for this subgroup.
TABLE 3
Susceptibilities of P. aeruginosa isolates to single agents stratified by location and/or source and percentage of nonsusceptible isolates remaining susceptible to ceftolozane-tazobactama
Single agent | All patient isolates (n = 1,257) | ICU patient isolates (n = 359, 28.6%) | Non-ICU patient isolates (n = 898, 71.4%) | Respiratory isolates (n = 490, 39.0%) | Nonrespiratory isolates (n = 767, 61.0%) | ICU patient respiratory isolates (n = 156, 12.4%) | ICU patient nonrespiratory isolates (n = 203, 16.1%) | Non-ICU patient respiratory isolates (n = 334, 26.6%) | Non-ICU patient nonrespiratory isolates (n = 564, 44.9%) | |||||||||
---|---|---|---|---|---|---|---|---|---|---|---|---|---|---|---|---|---|---|
% S | Of non-S, % S to C-T | % S | Of non-S, % S to C-T | % S | Of non-S, % S to C-T | % S | Of non-S, % S to C-T | % S | Of non-S, % S to C-T | % S | Of non-S, % S to C-T | % S | Of non-S, % S to C-T | % S | Of non-S, % S to C-T | % S | Of non-S, % S to C-T | |
Ceftolozane-tazobactam | 96.6 | NA | 95.0 | NA | 97.2 | NA | 94.7 | NA | 97.8 | NA | 92.3 | NA | 97.0 | NA | 95.8 | NA | 98.0 | NA |
Cefepime | 77.0 | 87.5 | 71.6 | 84.3 | 79.2 | 89.3 | 69.6 | 83.2 | 81.7 | 92.1 | 59.6 | 81.0 | 80.8 | 89.7 | 74.3 | 84.9 | 82.1 | 93.1 |
Ceftazidime | 77.0 | 86.2 | 68.8 | 83.9 | 80.3 | 87.6 | 70.0 | 83.0 | 81.5 | 89.4 | 60.3 | 80.6 | 75.4 | 88.0 | 74.6 | 84.7 | 73.7 | 90.2 |
Piperacillin-tazobactam | 71.8 | 90.1 | 63.0 | 88.0 | 75.4 | 91.4 | 63.1 | 87.3 | 77.4 | 93.1 | 50.6 | 85.7 | 72.4 | 91.1 | 68.9 | 88.5 | 79.3 | 94.0 |
Meropenem | 76.0 | 90.1 | 69.9 | 87.0 | 78.4 | 91.8 | 70.4 | 85.5 | 79.5 | 94.3 | 59.0 | 82.8 | 78.3 | 93.2 | 75.7 | 87.7 | 80.0 | 94.7 |
Tobramycin | 91.5 | 83.2 | 88.0 | 76.7 | 92.9 | 87.5 | 90.4 | 74.5 | 92.2 | 90.0 | 84.0 | 68.0 | 91.1 | 88.9 | 93.4 | 81.8 | 92.6 | 90.5 |
Ciprofloxacin | 72.3 | 91.1 | 71.0 | 87.5 | 72.8 | 92.6 | 68.0 | 88.5 | 75.1 | 93.2 | 64.7 | 83.6 | 75.9 | 91.8 | 69.5 | 91.2 | 74.8 | 93.7 |
The rates of susceptibility of P. aeruginosa isolates to cefepime, ceftazidime, piperacillin-tazobactam, and meropenem had limited variation and were generally within 10% of each other. Among all P. aeruginosa isolates, 24 to 28% were nonsusceptible to any one agent, with the rates of nonsusceptibility being the highest for respiratory isolates in the ICU, at 40 to 49% (Table 3).
Cross-resistance among the β-lactam comparators was common (Table 4). Meropenem was the comparator that was the most likely to be active in the case of resistance to one of the other β-lactams, with a susceptible result being obtained for 39 to 45% of the isolates. Similarly, in the case of meropenem nonsusceptibility, the rates of susceptibility to the other β-lactam comparators ranged from 36 to 44%. By comparison, with cefepime, ceftazidime, or piperacillin-tazobactam nonsusceptibility, the likelihood of activity of one of the other two agents ranged from 15 to 35%. These trends were similar among the subgroups of isolates. In contrast, of the isolates nonsusceptible to one of the other β-lactams, the rates of ceftolozane-tazobactam susceptibility ranged from 86 to 90% overall and from 81 to 86% for respiratory isolates from patients in an ICU. Even in the case of resistance to two, three, or all four β-lactam comparators, the overall rates of ceftolozane-tazobactam susceptibility were ≥80% (Fig. 1). Eleven percent of all isolates and 23% of respiratory isolates from patients in an ICU were resistant to all four β-lactam comparators. Ceftolozane-tazobactam remained active against 80% and 69% of all isolates and respiratory isolates from patients in an ICU with this resistance phenotype, respectively.
TABLE 4
Cross-resistance of P. aeruginosa isolates to β-lactams and ceftolozane-tazobactama
Isolate and β-lactam | % NS isolates | Of NS isolates, % S to: | ||||
---|---|---|---|---|---|---|
FEP | CAZ | TZP | MEM | C-T | ||
All patient isolates | ||||||
![]() ![]() ![]() ![]() | 23.0 | NA | 23.9 | 20.8 | 39.4 | 87.5 |
![]() ![]() ![]() ![]() | 23.0 | 23.9 | NA | 15.2 | 41.2 | 86.2 |
![]() ![]() ![]() ![]() | 28.2 | 35.3 | 30.8 | NA | 45.2 | 90.1 |
![]() ![]() ![]() ![]() | 24.0 | 42.1 | 43.7 | 35.8 | NA | 90.1 |
ICU patient isolates | ||||||
![]() ![]() ![]() ![]() | 28.4 | NA | 16.7 | 13.7 | 37.3 | 84.3 |
![]() ![]() ![]() ![]() | 31.2 | 24.1 | NA | 11.6 | 37.5 | 83.9 |
![]() ![]() ![]() ![]() | 37.0 | 33.8 | 25.6 | NA | 41.4 | 88.0 |
![]() ![]() ![]() ![]() | 30.1 | 40.7 | 35.2 | 27.8 | NA | 87.0 |
Non-ICU patient isolates | ||||||
![]() ![]() ![]() ![]() | 20.8 | NA | 27.8 | 24.6 | 40.6 | 89.3 |
![]() ![]() ![]() ![]() | 19.7 | 23.7 | NA | 17.5 | 43.5 | 87.6 |
![]() ![]() ![]() ![]() | 24.6 | 36.2 | 33.9 | NA | 47.5 | 91.4 |
![]() ![]() ![]() ![]() | 21.6 | 42.8 | 48.5 | 40.2 | NA | 91.8 |
Respiratory isolates | ||||||
![]() ![]() ![]() ![]() | 30.4 | NA | 18.1 | 16.1 | 41.6 | 83.2 |
![]() ![]() ![]() ![]() | 30.0 | 17.0 | NA | 10.9 | 40.8 | 83.0 |
![]() ![]() ![]() ![]() | 36.9 | 30.9 | 27.6 | NA | 45.9 | 87.3 |
![]() ![]() ![]() ![]() | 29.6 | 40.0 | 40.0 | 32.4 | NA | 85.5 |
Nonrespiratory isolates | ||||||
![]() ![]() ![]() ![]() | 18.3 | NA | 30.0 | 25.7 | 37.1 | 92.1 |
![]() ![]() ![]() ![]() | 18.5 | 31.0 | NA | 19.7 | 41.5 | 89.4 |
![]() ![]() ![]() ![]() | 22.6 | 39.9 | 34.1 | NA | 44.5 | 93.1 |
![]() ![]() ![]() ![]() | 20.5 | 43.9 | 47.1 | 38.9 | NA | 94.3 |
ICU patient respiratory isolates | ||||||
![]() ![]() ![]() ![]() | 40.4 | NA | 17.5 | 11.1 | 31.7 | 81.0 |
![]() ![]() ![]() ![]() | 39.7 | 16.1 | NA | 8.1 | 32.3 | 80.6 |
![]() ![]() ![]() ![]() | 49.4 | 27.3 | 26.0 | NA | 32.5 | 83.6 |
![]() ![]() ![]() ![]() | 41.0 | 32.8 | 34.4 | 18.8 | NA | 85.7 |
ICU patient nonrespiratory isolates | ||||||
![]() ![]() ![]() ![]() | 19.2 | NA | 15.4 | 17.9 | 46.2 | 89.7 |
![]() ![]() ![]() ![]() | 24.6 | 34.0 | NA | 16.0 | 44.0 | 88.0 |
![]() ![]() ![]() ![]() | 27.6 | 42.9 | 25.0 | NA | 53.6 | 91.1 |
![]() ![]() ![]() ![]() | 21.7 | 52.3 | 36.4 | 40.9 | NA | 93.2 |
Non-ICU patient respiratory isolates | ||||||
![]() ![]() ![]() ![]() | 25.7 | NA | 18.6 | 19.8 | 48.8 | 84.9 |
![]() ![]() ![]() ![]() | 25.4 | 17.6 | NA | 12.9 | 47.1 | 84.7 |
![]() ![]() ![]() ![]() | 31.1 | 33.7 | 28.8 | NA | 55.8 | 88.5 |
![]() ![]() ![]() ![]() | 24.3 | 45.7 | 44.4 | 43.2 | NA | 87.7 |
Non-ICU patient nonrespiratory isolates | ||||||
![]() ![]() ![]() ![]() | 17.9 | NA | 35.6 | 28.7 | 33.7 | 93.1 |
![]() ![]() ![]() ![]() | 16.3 | 29.3 | NA | 21.7 | 40.2 | 90.2 |
![]() ![]() ![]() ![]() | 20.7 | 38.5 | 38.5 | NA | 40.2 | 94.0 |
![]() ![]() ![]() ![]() | 20.0 | 40.7 | 51.3 | 38.1 | NA | 94.7 |
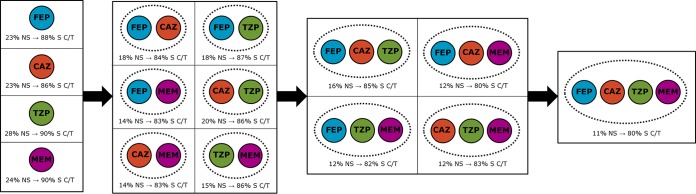
Rates of nonsusceptibility of P. aeruginosa isolates to one, two, three, or four β-lactam antibiotics and percentage of nonsusceptible isolates remaining susceptible to ceftolozane-tazobactam. Definitions: CAZ, ceftazidime; C/T, ceftolozane-tazobactam; FEP, cefepime; MEM, meropenem; NS, nonsusceptible; TZP, piperacillin-tazobactam; S, susceptible.
With respect to combination therapy, although the overall rates of ciprofloxacin susceptibility were comparable to those for the β-lactams, its activity against those isolates nonsusceptible to the primary β-lactam was significantly reduced (37 to 49% active) (Table 5). Therefore, only 9 to 14% improvements in susceptibility to the β-lactam and ciprofloxacin combination regimens compared to that to the primary β-lactam alone were seen, with these regimens being active against the tested isolates 85 to 88% of the time (Fig. 2). In contrast, tobramycin remained highly active (susceptibility rate, 76 to 80%) against the β-lactam-nonsusceptible isolates, which translated to 14 to 18% gains in susceptibility with tobramycin-containing combinations. The tobramycin-based regimens were active against 94 to 95% of all tested isolates, with the rates of susceptibility to the tobramycin-based regimens consistently being higher than those to the ciprofloxacin-based regimens. These differences were even more pronounced for ICU patient respiratory isolates, with 16 to 25% gains in susceptibility being achieved when ciprofloxacin was added and 28 to 37% gains in susceptibility being achieved when tobramycin was added (Fig. 3). Rates of susceptibility to ceftolozane-tazobactam monotherapy ranged from 92 to 98% across all subgroups of isolates, equaling or surpassing those achieved with the tobramycin-based combination regimens. The gains in susceptibility with the addition of either ciprofloxacin or tobramycin to ceftolozane-tazobactam were negligible (≤3% across all subgroups).
TABLE 5
Susceptibility of P. aeruginosa isolates to β-lactams alone and in combination with ciprofloxacin or tobramycin compared to susceptibility to ceftolozane-tazobactam monotherapya
Isolate and β-lactam | % S isolates | Of BL NS isolates, % CIP S | % S to BL-CIP | Of BL NS isolates, % TOB S | % S to BL-TOB | % C-T S |
---|---|---|---|---|---|---|
All patient isolates | ||||||
![]() ![]() ![]() ![]() | 77.0 | 39.8 | 86.2 | 78.2 | 95.0 | 96.6 |
![]() ![]() ![]() ![]() | 77.0 | 45.7 | 87.5 | 78.9 | 95.1 | |
![]() ![]() ![]() ![]() | 71.8 | 49.2 | 85.7 | 80.2 | 94.4 | |
![]() ![]() ![]() ![]() | 76.0 | 37.4 | 85.0 | 76.2 | 94.3 | |
ICU patient isolates | ||||||
![]() ![]() ![]() ![]() | 71.6 | 44.1 | 84.1 | 69.6 | 91.4 | 95.0 |
![]() ![]() ![]() ![]() | 68.8 | 49.1 | 84.1 | 74.1 | 91.9 | |
![]() ![]() ![]() ![]() | 63.0 | 51.1 | 81.9 | 75.2 | 90.8 | |
![]() ![]() ![]() ![]() | 69.9 | 38.9 | 81.6 | 69.4 | 90.8 | |
Non-ICU patient isolates | ||||||
![]() ![]() ![]() ![]() | 79.2 | 37.4 | 87.0 | 82.9 | 96.4 | 97.2 |
![]() ![]() ![]() ![]() | 80.3 | 43.5 | 88.9 | 81.9 | 96.4 | |
![]() ![]() ![]() ![]() | 75.4 | 48.0 | 87.2 | 83.3 | 95.9 | |
![]() ![]() ![]() ![]() | 78.4 | 36.6 | 86.3 | 79.9 | 95.7 | |
Respiratory isolates | ||||||
![]() ![]() ![]() ![]() | 69.6 | 41.6 | 82.7 | 81.2 | 94.7 | 94.7 |
![]() ![]() ![]() ![]() | 70.0 | 45.6 | 81.6 | 81.6 | 92.4 | |
![]() ![]() ![]() ![]() | 63.1 | 50.3 | 81.6 | 82.9 | 93.7 | |
![]() ![]() ![]() ![]() | 70.4 | 37.2 | 81.4 | 74.5 | 92.4 | |
Nonrespiratory isolates | ||||||
![]() ![]() ![]() ![]() | 81.7 | 37.9 | 88.7 | 75.0 | 95.4 | 97.8 |
![]() ![]() ![]() ![]() | 81.5 | 45.8 | 90.0 | 76.1 | 95.6 | |
![]() ![]() ![]() ![]() | 77.4 | 48.0 | 88.3 | 77.5 | 94.9 | |
![]() ![]() ![]() ![]() | 79.5 | 37.6 | 87.2 | 77.7 | 95.4 | |
ICU patient respiratory isolates | ||||||
![]() ![]() ![]() ![]() | 59.6 | 47.6 | 78.8 | 74.6 | 89.7 | 92.3 |
![]() ![]() ![]() ![]() | 60.3 | 51.6 | 80.8 | 77.4 | 91.0 | |
![]() ![]() ![]() ![]() | 50.6 | 50.6 | 75.6 | 75.3 | 87.8 | |
![]() ![]() ![]() ![]() | 59.0 | 39.1 | 75.0 | 67.2 | 86.5 | |
ICU patient nonrespiratory isolates | ||||||
![]() ![]() ![]() ![]() | 80.8 | 38.5 | 88.2 | 61.5 | 92.6 | 97.0 |
![]() ![]() ![]() ![]() | 75.4 | 46.0 | 86.7 | 70.0 | 92.6 | |
![]() ![]() ![]() ![]() | 72.4 | 51.8 | 86.7 | 75.0 | 93.1 | |
![]() ![]() ![]() ![]() | 78.3 | 38.6 | 86.7 | 72.7 | 94.1 | |
Non-ICU patient respiratory isolates | ||||||
![]() ![]() ![]() ![]() | 74.3 | 37.2 | 83.8 | 86.0 | 96.4 | 95.8 |
![]() ![]() ![]() ![]() | 74.6 | 41.2 | 85.0 | 84.7 | 96.1 | |
![]() ![]() ![]() ![]() | 68.9 | 50.0 | 84.4 | 88.5 | 96.4 | |
![]() ![]() ![]() ![]() | 75.7 | 35.8 | 84.4 | 80.2 | 95.2 | |
Non-ICU patient nonrespiratory isolates | ||||||
![]() ![]() ![]() ![]() | 82.1 | 37.6 | 88.8 | 80.2 | 96.5 | 98.0 |
![]() ![]() ![]() ![]() | 83.7 | 45.7 | 91.1 | 79.3 | 96.6 | |
![]() ![]() ![]() ![]() | 79.3 | 46.2 | 88.8 | 78.6 | 95.6 | |
![]() ![]() ![]() ![]() | 80.0 | 37.2 | 87.4 | 79.6 | 95.9 |

Percent susceptibility of all P. aeruginosa isolates (n = 1,257) to ceftolozane-tazobactam (pink bar) compared to that to β-lactams alone (light-blue bars) or in combination with ciprofloxacin (dark-blue bars) or tobramycin (green bars). Definitions: CAZ, ceftazidime; C/T, ceftolozane-tazobactam; FEP, cefepime; MEM, meropenem; TZP, piperacillin-tazobactam.

Percent susceptibility of ICU patient respiratory P. aeruginosa isolates (n = 156) to ceftolozane-tazobactam (pink bar) compared to that to β-lactams alone (orange bars) or in combination with ciprofloxacin (blue bars) or tobramycin (green bars). Definitions: CAZ, ceftazidime; C/T, ceftolozane-tazobactam; FEP, cefepime; MEM, meropenem; TZP, piperacillin-tazobactam.
DISCUSSION
Selection of an optimal empirical regimen for the treatment of P. aeruginosa infections in the face of an evolving resistance profile remains a continual clinical challenge. A delay of appropriate antibiotic therapy has been associated with detrimental therapeutic outcomes, resulting in prolonged hospital stays and increased mortality (18,–20). Thus, ensuring adequate initial therapy should be a high priority. Resistance to β-lactams is commonplace among P. aeruginosa isolates, with the rates of nonsusceptibility being 18 to 24% in this study, in line with previously reported data (6, 7). The β-lactam comparators performed particularly poorly against the ICU patient respiratory isolates, where the rates of susceptibility did not exceed 60%. This was in contrast to the findings for ceftolozane-tazobactam, which demonstrated consistently high levels of activity (>90%) regardless of isolate location or source.
In the empirical setting, many institutions employ a workhorse β-lactam, such as cefepime or meropenem, to be utilized in cases where P. aeruginosa infection is suspected. However, these data demonstrate that ≥20% of patients with P. aeruginosa infection have an isolate that is resistant and missed by this initial empirical regimen. Resistance to one β-lactam does not always confer resistance to the entire class, and these study data suggest that in approximately 50% of cases an alternative β-lactam will have activity. This discordance in β-lactam susceptibility profiles is primarily due to alterations in membrane permeability or efflux pumps with different affinities for the various β-lactams. For example, overexpression of the multidrug efflux pump mexXY-OprM was previously associated with an increase in the incidence of P. aeruginosa strains with a cefepime-resistant, ceftazidime-susceptible phenotype at one institution (21). Therefore, in cases where susceptibilities are known, if an isolate is reported to be susceptible to an alternative β-lactam, it is reasonable to switch therapy to that agent. However, prior to the reporting of susceptibilities or when a sample for culture is unobtainable, the decision of how to manage clinically failing patients is less clear. The use of rapid diagnostic tests to more quickly and accurately detect resistance is a promising future strategy for identifying patients who may benefit from ceftolozane-tazobactam therapy; however, the various technologies are still being developed.
These data indicate that switching to an alternative β-lactam in the absence of susceptibilities is expected to be effective only 20 to 40% of the time due to high rates of cross-resistance. In contrast, ceftolozane-tazobactam would be expected to have activity against approximately 90% of strains resistant to the primary β-lactam, making it a far more reliable therapeutic option. Additionally, in this study P. aeruginosa was nonsusceptible to all four β-lactams 11% of the time; however, 80% of these isolates remained ceftolozane-tazobactam susceptible. These data demonstrate that ceftolozane-tazobactam is a viable therapeutic option for the treatment of infections caused by β-lactam-resistant isolates; however, verification of susceptibility using an FDA-cleared test should still be performed when feasible. Additionally, little is known at this time about the potential for the emergence of resistance while on ceftolozane-tazobactam therapy. Although molecular characterization of the mechanisms of β-lactam resistance was not performed in this study, certain mutations in AmpC resulting in overexpression and structural modification have been shown to confer high-level resistance to ceftolozane, even in the presence of tazobactam (22, 23). As with all antibiotics, clinicians should aim to treat patients for the shortest duration necessary in order to ameliorate the potential for the propagation of resistant isolates.
The use of combination therapy is a commonly employed strategy when infection with a resistant P. aeruginosa strain is suspected. Although synergistic interactions between antimicrobials play an important role in some infections (e.g., ampicillin and ceftriaxone for enterococcal endocarditis), clinical studies with P. aeruginosa have failed to demonstrate superior outcomes with combination therapy over active monotherapy. Therefore, the primary purpose of dual therapy against P. aeruginosa is to increase the probability of having at least one active agent and enhance initial adequate therapy (17). In applying the most recent Infectious Diseases Society of America (IDSA) guidelines for the treatment of VAP, dual therapy with two agents active against Gram-negative bacteria is recommended when the ICU-level incidence-weighted resistance rates of Gram-negative bacteria exceed 10% (9). Although P. aeruginosa represents approximately 20% of VAP isolates, enteric Gram-negative bacteria, such as Klebsiella pneumoniae and Escherichia coli, are collectively more common causes of VAP (20 to 40%) (24, 25) and, within the United States, where the rates of bacterial extended-spectrum β-lactamase production are low, are often more susceptible than P. aeruginosa isolates to the comparator β-lactams examined in this study. However, not all facilities have the capacity to calculate a pooled rate of Gram-negative bacterial resistance to each potential empirical antibiotic choice. Therefore, use of the rate of susceptibility of P. aeruginosa as a conservative proxy value for the rate of susceptibility of Gram-negative bacteria overall is a common, guideline-endorsed strategy.
The low rates of susceptibility to the comparator β-lactams observed in this study would warrant initial combination therapy; however, a concerning finding of this study was that even when representative combination regimens were utilized, the rates of susceptibility were still below or barely exceeded the threshold of 90% for the ICU patient respiratory isolates. Ciprofloxacin-based combinations performed particularly poorly, with the rates of susceptibility of this resistant subgroup being only 75 to 81%. This observed inferiority of fluoroquinolone-based combinations compared to aminoglycoside-based regimens is in line with previous data, with β-lactam–fluoroquinolone cross-resistance being more common than β-lactam–aminoglycoside cross-resistance (26, 27). This is a limitation of standard antibiograms, which do not account for cross-resistance and may cause clinicians to overestimate the effectiveness of dual therapy. Combination antibiograms, which report in vitro susceptibilities to the select antibiotic combinations, may be of enhanced utility when dual therapy is being considered by providing a more accurate picture of the expected benefit (27). Although these data imply that tobramycin-based combination regimens should be preferred, it must be considered that patients with β-lactam-resistant, tobramycin-susceptible isolates would essentially be treated only with an aminoglycoside, at least until the final reporting of susceptibilities. The IDSA guidelines for the treatment of hospital-acquired pneumonia and VAP specifically recommend against aminoglycoside monotherapy, citing meta-analysis data demonstrating lower clinical response rates with aminoglycoside-containing regimens than with aminoglycoside-free regimens (56% versus 68%; relative risk, 0.82; 95% confidence interval, 0.71 to 0.95), though without a corresponding increase in mortality (9). In contrast, ceftolozane-tazobactam susceptibilities were sufficient to allow empirical monotherapy; in addition, ceftolozane-tazobactam had a more favorable side effect profile than either the aminoglycosides or fluoroquinolones. Robust clinical data assessing the efficacy of ceftolozane-tazobactam for the treatment of nosocomial pneumonia are still needed; however, early data from a retrospective study are promising (28). Currently, a phase 3 randomized controlled trial (ClinicalTrials.gov registration number NCT02070757) of ceftolozane-tazobactam versus meropenem is in the recruitment phase and should help to further define ceftolozane-tazobactam's efficacy for this indication.
Although this study's results suggest a significant potential role for ceftolozane-tazobactam within empirical and definitive therapy regimens, it should be noted that local resistance rates are key to determining the utility of ceftolozane-tazobactam for a specific institution. For example, at an institution where the rates of P. aeruginosa susceptibility to cefepime, ceftazidime, and piperacillin-tazobactam approach or exceed 90%, including within the ICU, empirical therapy with ceftolozane-tazobactam would be rarely indicated in the absence of patient-specific risk factors indicating a high probability of multidrug-resistant P. aeruginosa infection and the use of ceftolozane-tazobactam should not be routine. However, in regions where resistant P. aeruginosa is endemic, such as southern and southeastern Europe (8), the use of ceftolozane-tazobactam as empirical therapy for at-risk patients may be a promising strategy. With the increased utilization of hospital order sets, members of hospitals' antimicrobial stewardship committees and pharmacy and therapeutics committees are encouraged to utilize antibiogram results in building disease-specific order sets to help direct clinicians to the most appropriate antibiotic on the basis of local resistance trends and risk factors.
Whether these high levels of susceptibility to ceftolozane-tazobactam can be maintained over time remains to be seen. As noted above, resistance already exists among P. aeruginosa isolates, albeit at very low levels. Active and ongoing antimicrobial stewardship efforts, including dose optimization and prompt deescalation to alternative antibiotics as susceptibilities become available, will likely be required to preserve the activity of ceftolozane-tazobactam in the future.
Conclusions.
The rates of P. aeruginosa resistance to commonly utilized antibiotics can be substantial, and the risk appears to be the highest for the most critically ill patients. Ceftolozane-tazobactam demonstrated significantly enhanced activity against P. aeruginosa compared to the other β-lactams, with its potency against ICU isolates being maintained and cross-resistance being limited. Additionally, ceftolozane-tazobactam monotherapy may provide a safe and effective alternative to combination antimicrobial therapy. Although clinical data are needed to substantiate these results, ceftolozane-tazobactam appears to be a highly active option for the treatment of documented or suspected P. aeruginosa infections.
MATERIALS AND METHODS
Between June 2013 and September 2014, 1,257 nonduplicate, nonurine isolates of P. aeruginosa were obtained from adult inpatients in 44 geographically diverse hospitals. The isolates were processed at the Center for Anti-Infective Research and Development (Hartford Hospital, Hartford, Connecticut) and transferred onto Trypticase soy agar plates (5% blood) for MIC determination. The β-lactam comparators investigated were cefepime, ceftazidime, piperacillin-tazobactam, and meropenem. Ciprofloxacin and tobramycin were additionally assessed. MICs for ceftolozane-tazobactam and comparator antimicrobials were determined with CLSI broth microdilution methods and interpreted according to 2017 CLSI breakpoints. An isolate was classified as susceptible to a combination regimen if it was susceptible to one or both antibiotics. Ceftolozane-tazobactam was provided courtesy of Cubist Pharmaceuticals (Lexington, MA); other antibiotics were purchased from Sigma (St. Louis, MO). Additional laboratory methods are further described in detail in a previous publication (29).
ACKNOWLEDGMENTS
This work was supported by Cubist Pharmaceuticals (Lexington, MA) for collection of the original surveillance data. No additional funds were provided for the current analysis.
The funders had no role in study design, data collection and interpretation, or the decision to submit the work for publication.
During 2016 and 2017, K. J. Goodlet received grant support from Merck. D. P. Nicolau has received research grants and is on the speakers' bureau of Cubist Pharmaceuticals. M. D. Nailor has received grant support or honorarium from Merck and Astellas.
REFERENCES
Articles from Antimicrobial Agents and Chemotherapy are provided here courtesy of American Society for Microbiology (ASM)
Full text links
Read article at publisher's site: https://doi.org/10.1128/aac.01350-17
Read article for free, from open access legal sources, via Unpaywall:
https://aac.asm.org/content/aac/61/12/e01350-17.full.pdf
Citations & impact
Impact metrics
Citations of article over time
Alternative metrics
Article citations
Ten Issues to Update in Nosocomial or Hospital-Acquired Pneumonia: An Expert Review.
J Clin Med, 12(20):6526, 14 Oct 2023
Cited by: 2 articles | PMID: 37892664 | PMCID: PMC10607368
Review Free full text in Europe PMC
Tazobactam/ceftolozane and tobramycin combination therapy in extensively drug-resistant Pseudomonas aeruginosa infections in severe burn injury: a case report.
J Pharm Health Care Sci, 9(1):25, 08 Aug 2023
Cited by: 0 articles | PMID: 37550794 | PMCID: PMC10408163
How to Manage Pseudomonas aeruginosa Infections.
Adv Exp Med Biol, 1386:425-445, 01 Jan 2022
Cited by: 4 articles | PMID: 36258082
Collective assessment of antimicrobial susceptibility among the most common Gram-negative respiratory pathogens driving therapy in the ICU.
JAC Antimicrob Resist, 3(1):dlaa129, 19 Feb 2021
Cited by: 8 articles | PMID: 34223078 | PMCID: PMC8209971
Ceftolozane-Tazobactam Treatment of Hypervirulent Multidrug Resistant Pseudomonas aeruginosa Infections in Neutropenic Patients.
Microorganisms, 8(12):E2055, 21 Dec 2020
Cited by: 3 articles | PMID: 33371496 | PMCID: PMC7767535
Go to all (19) article citations
Data
Data behind the article
This data has been text mined from the article, or deposited into data resources.
BioStudies: supplemental material and supporting data
Clinical Trials
- (1 citation) ClinicalTrials.gov - NCT02070757
Similar Articles
To arrive at the top five similar articles we use a word-weighted algorithm to compare words from the Title and Abstract of each citation.
Antimicrobial Activity of Ceftolozane-Tazobactam Tested Against Enterobacteriaceae and Pseudomonas aeruginosa with Various Resistance Patterns Isolated in U.S. Hospitals (2013-2016) as Part of the Surveillance Program: Program to Assess Ceftolozane-Tazobactam Susceptibility.
Microb Drug Resist, 24(5):563-577, 17 Oct 2017
Cited by: 28 articles | PMID: 29039729
Activity of Ceftolozane-Tazobactam against Pseudomonas aeruginosa and Enterobacteriaceae Isolates Collected from Respiratory Tract Specimens of Hospitalized Patients in the United States during 2013 to 2015.
Antimicrob Agents Chemother, 62(3):e02125-17, 23 Feb 2018
Cited by: 31 articles | PMID: 29263073 | PMCID: PMC5826161
Activity of Ceftolozane-Tazobactam and Ceftazidime-Avibactam against Beta-Lactam-Resistant Pseudomonas aeruginosa Isolates.
Antimicrob Agents Chemother, 61(12):e01858-17, 22 Nov 2017
Cited by: 37 articles | PMID: 28993338 | PMCID: PMC5700315
Ceftolozane/tazobactam: a novel cephalosporin/β-lactamase inhibitor combination with activity against multidrug-resistant gram-negative bacilli.
Drugs, 74(1):31-51, 01 Jan 2014
Cited by: 165 articles | PMID: 24352909
Review