Abstract
Free full text

Regulation of the immune response by the aryl hydrocarbon receptor
Abstract
The aryl hydrocarbon receptor (AhR) is a ligand-activated transcription factor that is activated by small molecules provided by the diet, microorganisms, metabolism and pollutants. AhR is expressed by a number of immune cells, and thus, AhR signaling provides a molecular pathway that integrates the effects of the environment and metabolism on the immune response. Studies have shown that AhR signaling plays important roles in the immune system in health and disease. As its activity is regulated by small molecules, AhR also constitutes a potential target for therapeutic immunomodulation. In this review we discuss the role of AhR in the regulation of the immune response in the context of autoimmunity, infection and cancer, as well as the potential opportunities and challenges of developing AhR targeted therapeutics.
INTRODUCTION
The Aryl Hydrocarbon Receptor (AhR) is a member of the Pern-Arnt-Sim (PAS) superfamily of transcription factors that are involved in sensing environmental signals such as changes in the circadian rhythm (BMAL1 and BMAL2), oxygen tension or redox potential (HIF-1α, HIF-2α, HIF-3α) among others (Kewley et al., 2004). In response to activation by a ligand, AhR translocates from the cytoplasm to the nucleus where it controls the transcription of a wide variety of target genes. Although AhR was initially recognized as the mediator of the toxic effects of dioxins, multiple physiologic ligands are provided by the diet, the commensal flora and also the host metabolism. The identification of these natural ligands and the analysis of AhR-deficient mice has revealed important physiological roles for AhR.
Both genetic and environmental factors contribute to the regulation of the immune system in autoimmunity, infections and cancer. Although significant advances have been made in the identification of the genetic control of the immune response, limited information is still available regarding the contribution of environmental factors to immune regulation and the mechanisms involved. In this context, AhR provides a model signaling pathway to investigate the molecular mechanisms through which the environment modulates the immune response in health and disease. Moreover, as AhR activity is regulated by small molecules, a detailed understanding of the mechanisms through which AhR controls the immune response is likely to guide new approaches for therapeutic immunomodulation. In this review, we discuss current knowledge on the multiple roles of AhR signaling in T cells and dendritic cells (DCs), and its relevance for the regulation of the immune response in health and disease.
AhR-DEPENDENT SIGNALING PATHWAYS
When inactive, AhR is localized in the cytoplasm as part of a complex formed by a dimer of the 90-kDa heat shock protein (HSP90) (Denis et al., 1988; Perdew, 1988), the AhR-interacting protein (AIP, also known as XAP2 or Ara9) (Carver and Bradfield, 1997; Meyer and Perdew, 1999), the cochaperone p23 (Grenert et al., 1997; Nair et al., 1996) and the c-SRC protein kinase (Dong et al., 2011) (Figure 1). HSP90 stabilizes AhR in a conformation of high affinity for its ligands (Pongratz et al., 1992). In addition, AIP prevents AhR ubiquitination and degradation, maintaining AhR steady state cellular levels (Lees et al., 2003). Ligand binding releases AIP from the complex and triggers conformational changes in AhR that expose its nuclear localization signal, leading to AhR translocation to the nucleus (Ikuta et al., 1998). These conformational changes also expose a protein kinase C target site that when phosphorylated interferes with AhR nuclear translocation (Ikuta et al., 2004), constituting one of several mechanisms to control AhR. Of note, the regulation of AhR translocation to the nucleus is a potential target to for the specific modulation of the non-genomic AhR signaling discussed subsequently.
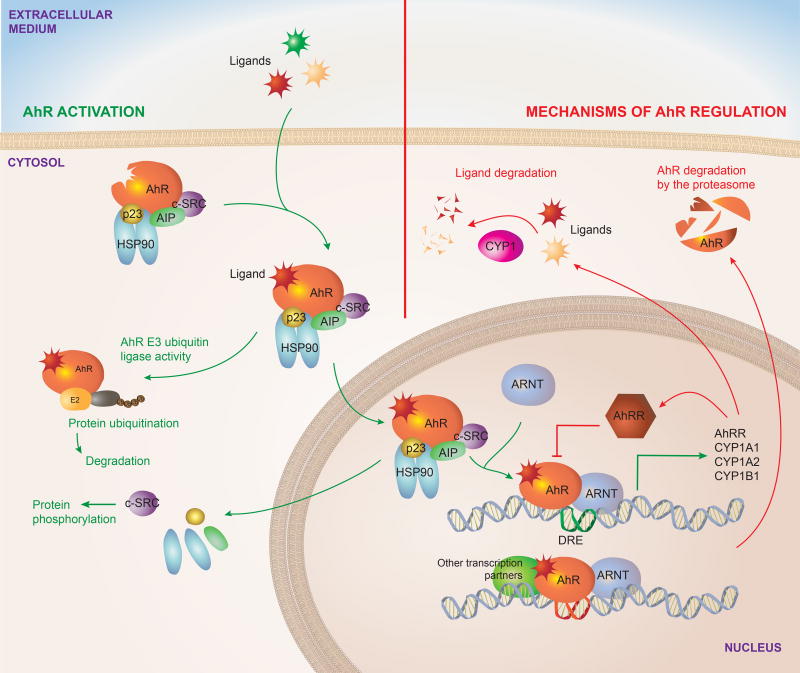
Inactive AhR is localized in the cytosol complexed to HSP90, AIP, p23 and c-SRC. Upon interaction with an agonist, conformational changes result in the translocation of the complex to the nucleus and the interaction of AhR with ARNT after the dissociation of the cytoplasmic complex. The AhR-ARNT heterodimer controls the transcription of DRE containing genes. AhR signaling also includes non-genomic pathways, for example AhR functions as an E3 ubiquitin ligase, while the release of the c-SRC kinase results in the phosphorylation of multiple targets. AhR activation is limited by regulatory mechanisms, some of which are actually triggered by AhR activation. AhR drives the expression of CYP enzymes, which degrade AhR ligands. AhR also induces the expression of its repressor AhRR, which inhibits the formation of AhR/ARNT complex required for AhR signaling.
Data obtained in HeLa cells suggest that AhR translocates to the nucleus while still bound to HSP90 (Tsuji et al., 2014). However, it still remains to be seen whether this observation can be extrapolated to other cellular contexts and to all AhR agonists (Davarinos and Pollenz, 1999; Reyes et al., 1992). Once in the nucleus, the association of AhR with the AhR nuclear translocator (ARNT) results in the transcriptional control of multiple target genes (Furman et al., 2009). These genes include several xenobiotic metabolizing enzymes including the microsomal cytochrome P450-dependent monooxygenases including cytochrome P450 family-1 subfamily-A polypeptide-1 (CYP1A1), cytochrome P450 family-1 subfamily-A Polypeptide-2 (CYP1A2), cytochrome P450 family-1 subfamily-B polypeptide-1 (CYP1B1) and NAD(P)H-quinone oxidoreductase.
The genomic regulatory regions of AhR target genes contain the AhR binding DNA consensus motif (5’-TNGCGTG-3’), referred to as the dioxin- or xenobiotic-responsive element (DRE or XRE), (Durrin et al., 1987). The AhR/ARNT complex recognizes DREs, while it also interacts with other transcriptional regulators to control the expression of target genes. AhR has also been shown to influence chromatin remodeling by interacting with the SWI/SNF chromatin-remodeling complex (Wang and Hankinson, 2002), the steroid receptor coactivator-1 complex (SRC-1) (Beischlag et al., 2002) and by displacing histone deacetylase (HDAC) complexes (Schnekenburger et al., 2007). Additional epigenetic mechanisms involved in the genomic control of biological processes by AhR include the regulation of retrotransposons, micro-RNAs and long non-coding RNAs, which can regulate the expression of multiple target genes (Chinen et al., 2015; Garcia et al., 2017; Goldstone et al., 2012; Gordon et al., 2015; Hanieh and Alzahrani, 2013; Hecht et al., 2014; Morales-Hernandez et al., 2016; Nakahama et al., 2013; Okudaira et al., 2010; Roman et al., 2008; Singh et al., 2016; Wragg et al., 2013; Zhang et al., 2012). In addition, AhR can also repress gene expression directly by interacting with target DNA sequences or indirectly by controlling the activity of additional molecules involved in the control of gene expression, such as transcription factors and regulatory RNAs.
AhR interacts with other transcription factors such as NF-κB, c-Maf, the retinoic acid receptor, the estrogen receptor, E2F and retinoblastoma protein (Rb) to modulate their activity and consequently, the expression of their target genes (Hankinson, 2005). Some of these interactions may participate on regulatory feedback loops highly relevant for immune regulation. For example, NF-κB induces AhR expression, but AhR can then regulate NF-κB signaling (Kimura et al., 2009; Rothhammer et al., 2016; Vogel et al., 2014; Vogel and Matsumura, 2009; Yeste et al., 2016).
The association of AhR to other transcription factors can direct its recruitment to target DNA sequences different from canonical DREs. For example, AhR dimerizes with RelA and RelB leading to its recruitment to NF-κB responding sites (Kim et al., 2000; Vogel et al., 2007b) and with KLF6, leading to its recruitment to non-consensus DNA elements (Wilson et al., 2013). Interestingly, different ligands have been suggested to induce the association of AhR with different proteins (Murray et al., 2010), potentially leading to AhR recruitment to different target DNA sequences and consequently triggering ligand-specific biologic responses.
In addition to the genomic mechanisms through which AhR regulates gene expression, AhR can also control biological processes through non-genomic signaling events. For example, the release of c-SRC from the AhR cytoplasmic complex results in the phosphorylation of multiple c-SRC target proteins (Dong et al., 2011; Matsumura, 2009). AhR can also act as an E3 ubiquitin ligase that targets substrates for ubiquitination and degradation by the proteasome, for example triggering the degradation of the estrogen receptor (Ohtake et al., 2007). Although the relevance of these non-genomic pathways has not been yet evaluated in depth in the immune system, their importance for immune regulation is starting to emerge, as shown in a recent report by Bessede et al that describes the role of AhR-controlled activation of c-SRC in limiting macrophage responses to inflammatory stimuli (Bessede et al., 2014; Quintana, 2014).
Finally, it has been proposed that AhR can act independently of ligand activation under certain circumstances (Han et al., 2008; Ikuta et al., 2000; Murray et al., 2005), but the physiological relevance of these observations remains to be evaluated.
Inhibitory mechanisms target AhR signaling at multiple levels. As mentioned, AhR is a ligand-activated transcription factor. Thus, ligand availability effectively controls AhR-dependent signaling as recently shown by studies in which the overexpression of CYP1A1 led to the depletion of physiologic AhR agonists, resulting in a phenotype that resembles that of AhR-deficient mice with regards to intestinal immunity (Schiering et al., 2017). In addition, the AhR repressor (AhRR) binds to ARNT, inhibiting the transcriptional activity of the AhR/ARNT complex (Mimura et al., 1999). Finally, AhR is degraded via the 26S proteasome (Davarinos and Pollenz, 1999; Ma and Baldwin, 2000). Interestingly, Cyp1a1 and Ahrr are transcriptional targets of AhR, and 26S proteasome-driven degradation is triggered by AhR activation, highlighting the importance of negative feedback loops that limit AhR activation to avoid potential deleterious effects of unrestricted chronic AhR signaling.
AhR LIGANDS
AhR was first identified as the receptor for 2,3,7,8-tetracholrodibenzo-p-dioxin (TCDD) (Poland and Knutson, 1982). Additional xenobiotic AhR ligands have been identified, differing in their structures and affinities for the receptor as extensively reviewed elsewhere (Nguyen and Bradfield, 2008). However, in spite of the initial association of AhR with these xenobiotic compounds, natural AhR ligands have also been identified. The physiological relevance of natural AhR ligands is highlighted by the upregulation of AhR-target genes during development (Campbell et al., 2005; Choudhary et al., 2003), and reports of defects in the development and physiology of AhR deficient mice (Fernandez-Salguero et al., 1995; Lahvis et al., 2005; Schmidt et al., 1996).
Diverse sources of physiological AhR ligands have been identified so far, including ligands provided by the diet, free radical formation and enzymatic activities in the host and the commensal flora (Quintana, 2013, 2014; Quintana and Sherr, 2013). In Table 1 we highlight major sources of physiological AhR ligands relevant to the immune system
TABLE 1
AhR Endogenous Ligands
Group | Compound | Origin |
---|---|---|
Indole Metabolites | Indole | Dietary metabolite and microbiota metabolism |
Indolo[3,2-b]carbazole (ICZ) | Dietary metabolite | |
2-(Indol-3-ylmethyl)-3,3’-diindolylmethane (Ltr-1) | Dietary metabolite | |
3,3’-Diindolylmethane (DIM) | Dietary metabolite | |
2-(1’H-indole-3’-carbonyl)-thiazole-4-carboxylic acid methyl ester (ITE) | Endogenous/Chemical process | |
Phytochemicals | Indigorubin | Plants |
Indigo | Plants | |
Tryptophan Metabolites | Kynurenine (Kyn) | Host Metabolism |
Kynyrenic acid (KA) | Host Metabolism | |
Zanthurenic acid | Host Metabolism | |
Cinnabarinic acid (CA | Host Metabolism | |
6-Formylindolo[3,2-b]carbazole (FICZ) | Photo-oxidation | |
5-hydroxy-tryptophan (5HTP) | Host Metabolism | |
Tryptamine (TA) | Microbiota metabolism | |
Indol-3-acetic Acid (IAA) | Microbiota metabolism | |
3-methylindole (Skatole) | Microbiota metabolism | |
Indole-3-aldehyde (IAld) | Microbiota metabolism | |
Indoxyl-3-sulfate (I3S) | Microbiota and Host Metabolite | |
Heme-derived | Bilirubin | Host Metabolism |
Biliverdin | Host Metabolism | |
Arachidonic Acid Metabolites | Lipoxin 4A | Host Metabolism |
Prostaglandin PGG2 | Host Metabolism | |
Hydroxyeicosatrienoic acid ([12(R)-HETE]) | Host Metabolism |
An abundant source of AhR agonists is the diet. The Brassica genus (broccoli, cauliflower, Brussel sprouts and cabbages) contains significant amounts of indole-based glucobrassicin. Myrosinases, enzymes present in plants, the gut flora, and also induced by chewing, metabolize glucobrassicin into the AhR agonist precursors indole-3-carbinol (I3C) and indole-3-acetonitrile (I3ACN) (Loub et al., 1975). I3C and I3ACN are further transformed by nonenzymatic acid condensation into AhR agonists including 3,3’diindolylmethane (DIM), 2-(indol-3-ylmethyl)-3,3’-diindolylmetane, and indolo[3,4-b]carbazole (ICZ) (Bjeldanes et al., 1991; Chen et al., 1996; Degner et al., 2009; Ociepa-Zawal et al., 2007) (Figure 2A). Among them, ICZ shows the highest AhR agonistic activity. Dietary I3C and its condensation products are potentially important in the context of autoimmunity and inflammation since they have been shown to have therapeutic activity in experimental murine models of multiple sclerosis and colitis through the AhR-dependent induction of FoxP3+ regulatory T cells (Tregs) (Huang et al., 2013; Rouse et al., 2013). Moreover, I3C and its condensation products are also critical for the development of gut-associated intraepithelial lymphocytes and the maintenance of gut epithelial barrier integrity (Kiss et al., 2011; Li et al., 2011).
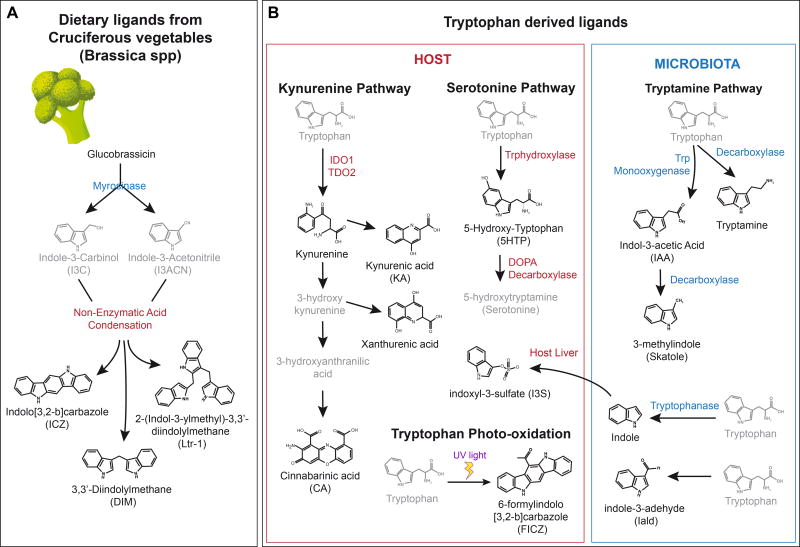
A. Indole-derived AhR agonists provided by the diet. B. Tryptophan-derived AhR agonists. Both the host and the microbiota are implicated in the generation of these agonists.
Additional AhR ligands are originated in plants. Indirubin and indigo are plant-derived phytochemicals with mild AhR agonistic activity; they have also been proposed to be produced by human cytochrome P450 (Gillam et al., 2000). However, despite being detectable in human urine (Adachi et al., 2001), their concentrations are too low to be considered important under physiological conditions unless specific mechanisms increase their production and/or accumulation in microenvironments that may benefit from AhR activation, for example to maintain the integrity of intestinal tissues.
The metabolism of tryptophan (Trp) is also a physiological source of AhR agonists. The primary route of Trp metabolism is the kynurenine (Kyn) pathway, which transforms Trp into nicotinamide adenine dinucleotide and different intermediate byproducts, some of which activate AhR (Figure 2B). Indoleamine-2,3-dioxygenase (IDO) and Tryptophan-2,3-dioxygenase (TDO) are the two main enzymes that catalyze the conversion of Trp into Kyn in the context of inflammation and cancer with important immune effects. Kyn, for example, is produced by glioma cells (Opitz et al., 2011) and has been described to boost the differentiation of Tregs (Mezrich et al., 2010). Thus, AhR activation by Kyn produced in the tumor microenvironment has been linked to glioma-associated immunosuppression (Gabriely et al., 2017; Takenaka et al., 2016). Kyn can also be metabolized into additional potent AhR ligands like kynurenic acid, xanthirenic acid or cinnabarinic acid.
Tryptamine and serotonin metabolism can also provide AhR agonists derived from Trp through a pathway independent of IDO/TDO. Indeed, tryptamine itself and its downstream metabolite indole acetic acid may act as direct AhR agonists (Heath-Pagliuso et al., 1998; Vikstrom Bergander et al., 2012). Interestingly, trypatmine is also a CYP1A1 substrate (Chiaro et al., 2007). In addition, 5-Hydroxy-Trp, a proximal serotonine metabolite, has been identified as a mild AhR ligand (Bittinger et al., 2003) (Figure 2B).
Trp is also thought to provide AhR agonists through additional mechanisms. The AhR agonist 2-(1’H-indole-3’-carbonyl)-thiazole-4-carboxylic acid methyl ester (ITE), purified from porcine lung, has been hypothesized to be formed through a condensation reaction between Trp and cysteine (Song et al., 2002). The pathways involved in ITE synthesis and metabolism, however, are still unknown and its synthesis under physiological conditions is still debatable.
Finally, exposure of Trp to ultraviolet light triggers its photo-oxidation to 6-formylindolo[3,2-b]carbazole (FICZ) a potent AhR ligand (Rannug et al., 1987; Wincent et al., 2009) with broad implications in UV light response, genomic stability, circadian rhythms and the immune response (Quintana et al., 2008; Veldhoen et al., 2008; Wincent et al., 2012). Additional Trp photometabolites exist, such as 1-(1H-indol-3-yl)-9H-pyrido[3,4-b]indole which exhibits a potency similar to that of FICZ (Diani-Moore et al., 2011). These photometabolites may have both local effects in the skin, and also systemic effects because FICZ has been detected in human urine (Wincent et al., 2012). In fact, the generation of FICZ through light-independent pathways has been recently reported (Smirnova et al., 2016).
The microbial metabolism of dietary Trp also produces additional AhR ligands such as indole-3-acetic acid (IAA) (Jin et al., 2014b; Miller, 1997), tryptamine (TA) and 3-methyl indole (skatole) (Hubbard et al., 2015; Weems and Yost, 2010). Bacterial tryptophanase converts dietary Trp to indole, and is indeed essential for the subsequent production of indoxyl-3-sulfate (I3S) by the liver (Schroeder et al., 2010; Wikoff et al., 2009). Other derivatives of Trp catabolism produced by commensal Lacobacillus species (e.g. indole-3-adehyde (Iald)) maintain intestinal homeostasis preventing not only colonization by pathogenic microorganisms like Candida albicans (Zelante et al., 2013) but also the worsening of inflammatory disorders affecting the intestinal tract such as inflammatory bowel disease (IBD) and cancer. Indeed, it has been recently reported that deficits in commensal bacteria that produce Trp-derived AhR agonists may contribute to the pathogenesis of human IBD (Lamas et al., 2016). Accordingly, microbiota-based interventions may provide an efficacious therapeutic approach to deliver AhR ligands and re-establish intestinal homeostasis. Finally, it is worth to mention that novel AhR ligands have been recently identified in bacteria. For example, pigmented virulence factors from pulmonary pathogens activate AhR as we will discuss later (Moura-Alves et al., 2014).
Some Heme-derived metabolites present AhR agonistic activities. Bilirubin induces the expression of the AhR-target gene CYP1A1 in hepatoma cells (Sinal and Bend, 1997); biliverdin also activates AhR (Phelan et al., 1998). AhR activation by bilirubin was recently reported to suppress experimental colitis, suggesting that bilirubin contributes to AhR-dependent anti-inflammatory effects in vivo (Longhi et al., 2017). Interestingly, the deficiency in UDP-glucuronosyltransferase, which degrades bilirubin, results in increased CYP1A1 expression likely as a result of bilirubin-triggered AhR activation (Kapitulnik and Gonzalez, 1993). Indeed, CYP1A1, CYP1A2 and UDP-glucuronosyltransferase participate in bilirubin catabolism as part of a negative feedback loop that can potentially limit AhR activation by removing physiologically relevant agonists.
Finally, some arachidonic acid derivatives have been shown to activate AhR. Prostaglandins and leukotrienes activate AhR, being prostaglandin G2 the most potent agonist (Seidel et al., 2001). Interestingly, AhR activation induces the expression of cyclooxygenase 2 (Degner et al., 2009; Vogel et al., 2007a), which is responsible of the synthesis of prostaglandin. Moreover the product of the AhR-induced gene CYP1A1 catalyzes the generation of eicosanoids from arachidonic acid (Capdevila et al., 2002). However, it should be noted that the molecular structure of these compounds differs significantly from the structure of other AhR ligands and their agonistic activity is generally weak. Thus, it is still unclear whether arachidonic acid derivatives play a physiological role as AhR agonists unless their local levels are highly increased by specific mechanisms, for example in the microenvironment of inflamed tissues.
AhR IN T CELLS
AhR has significant effects in the control of adaptive immunity, modulating T-cell differentiation and function directly and indirectly through its effects on antigen presenting cells. AhR activation by its potent xenobiotic ligand TCDD was found to inhibit immune responses (Kerkvliet et al., 1990), an effect that was later linked to the induction of CD4+ T cells with a regulatory phenotype (Funatake et al., 2005; Kerkvliet et al., 2002; Marshall et al., 2008). Additionally, a role for AhR in Th17 biology and the control of IL-22 production by T cells was also established (Quintana et al., 2008; Rutz et al., 2011; Veldhoen et al., 2009; Veldhoen et al., 2008; Yeste et al., 2014). In the following sections we discuss illustrative examples of the multiple roles of AhR on effector and regulatory T cells.
AhR in Treg cells
FoxP3+ Treg cells (Fontenot et al., 2003; Hori et al., 2003) and IL-10 producing type 1 regulatory T cells (Tr1 cells) (Groux et al., 1997) are the best-characterized regulatory T-cell populations. Both Foxp3+ Tregs and Tr1 cells have been linked to AhR.
AhR activation by TCDD, ITE, Kyn or Laquinimod metabolites increases FoxP3+ Tregs trough different mechanisms, including direct transactivation and the induction of epigenetic modifications that control Foxp3 transcription, and also through the modulation of DCs (Goettel et al., 2016; Kaye et al., 2016; Kerkvliet et al., 2009; Mezrich et al., 2010; Quintana et al., 2008; Singh et al., 2016; Singh et al., 2011). AhR activation with TCDD in the presence of TGF-β1 also induces the expression of SMAD1 in human Tregs, stabilizing FoxP3 expression (Gandhi et al., 2010). The analysis of murine AhR deficient T cells demonstrated that AhR also prevents STAT1 activation, which can inhibit FoxP3+ Treg differentiation (Kimura et al., 2008; Quintana et al., 2010). Additionally, AhR drives the expression of the epigenetic modifier Aiolos, which suppresses the expression of genes associated to effector T-cell function like IL-2 (Gandhi et al., 2010; Goettel et al., 2016; Quintana et al., 2012). The effects of AhR on FoxP3+ Tregs, however, may be influenced by the experimental model used, probably reflecting tissue specific effects and/or the differential contribution of AhR agonists provided by the commensal flora (Dant et al., 2017). In addition, it has been also suggested that xenobiotic AhR agonists may selectively induce effector T-cell death, contributing to the relative increase in FoxP3+ Tregs observed after AhR activation (Stockinger et al., 2009). Collectively, these findings suggest a role for AhR agonists in the control of functional FoxP3+ Tregs, as indicated by the protective effects of AhR activation in experimental models of autoimmune disease like EAE (Quintana et al., 2008; Quintana et al., 2010), colitis (Goettel et al., 2016), uveitis (Nugent et al., 2013) and diabetes (Kerkvliet et al., 2009). It is not clear yet whether specific subpopulations of FoxP3+ Tregs express higher levels of AhR, as recently described for TIGIT+ FoxP3+ Tregs (Joller et al., 2014), making them more susceptible to modulation by AhR agonists.
Tr1 cells are induced Foxp3− IL-10+ CD4+ Tregs implicated in the control of tissue inflammation through their capacity to secrete IL-10 among other mechanisms (Groux et al., 1997). IL-27 promotes Tr1 cell differentiation (Awasthi et al., 2007; Fitzgerald et al., 2007; Stumhofer et al., 2006), while IL-21 acts in an autocrine manner to stabilize these cells (Pot et al., 2009; Spolski et al., 2009). IL-27 induces AhR expression in Tr1 cells through a mechanism driven by STAT3; AhR expression is then maintained by the transactivation of the Ahr promoter by AhR (Apetoh et al., 2010; Gandhi et al., 2010; Mascanfroni et al., 2015; Wu et al., 2011). The importance of AhR for Tr1 cells in vivo is highlighted by the deficient differentiation of Tr1 cells triggered by chronic administration of anti-CD3 to AhR mutant mice (Apetoh et al., 2010; Wu et al., 2011). Interestingly, additional factors controlled by the environment, such as melatonin, boost AhR expression in Tr1 cells (Farez et al., 2015).
AhR influences the transcriptional program of human (Gandhi et al., 2010) and mouse (Apetoh et al., 2010; Mascanfroni et al., 2015; Wu et al., 2011) Tr1 cells at multiple levels (Apetoh et al., 2010; Gandhi et al., 2010; Mascanfroni et al., 2015; Wu et al., 2011) (Figure 3). AhR synergizes with cMaf to drive the expression of IL-10 and IL-21 in Tr1 cells (Apetoh et al., 2010; Gandhi et al., 2010; Wu et al., 2011). In addition, AhR and STAT3 in Tr1 cells cooperate to drive the expression of CD39, an ectonucleotidase that depletes proinflammatory extracellular ATP (eATP), while cooperating with CD73 to generate immunosuppressive adenosine (Gandhi et al., 2010; Goettel et al., 2016; Mascanfroni et al., 2015; Takenaka et al., 2016). Indeed, CD39 contributes to the suppressive activity of Tr1 cells in vivo and in vitro (Gandhi et al., 2010; Goettel et al., 2016; Mascanfroni et al., 2015).
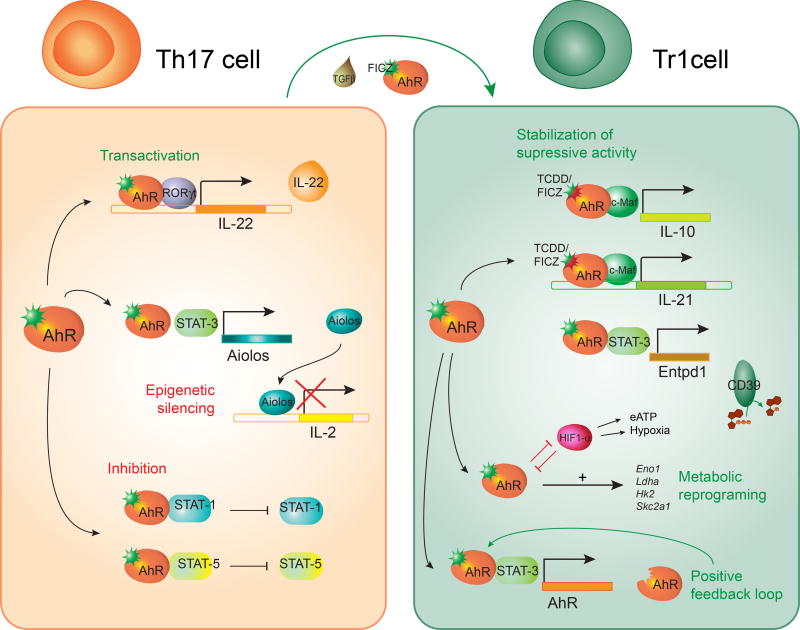
In Tr1 cells, AhR cooperates with c-Maf to induce the expression of IL-10 and IL-21 and with STAT 3 to drive CD39 expression; AhR also drives its own expression. AhR mediates the metabolic reprogramming of Tr1 cells by controlling the expression of enzymes ivolved in glucose metabolism. In Th17 cells, AhR induces IL-22 expression in cooperation with RORγt. AhR also inhibits STAT1 and STAT5 activation, as well as IL-2 expression by inducing the epigenetic modifier Aiolos in cooperation with STAT3. AhR has been implicated in the plasticity of Th17 by driving their trans-differentiation into Tr1 cells.
AhR-induced CD39 also affects Tr1 cell differentiation. Following activation, T cells release eATP (Takenaka et al., 2016). eATP interferes with Tr1 cell differentiation by signaling via the hypoxia inducible factor-1α (HIF1-α). HIF1-α outcompetes AhR for its interaction with ARNT and promotes AhR degradation via the immunoproteosome, suppressing AhR-dependent Tr1 cell differentiation (Mascanfroni et al., 2015). AhR-driven expression of CD39 depletes eATP and allows Tr1 cell differentiation to proceed. Thus, AhR actively drives the expression of genes central in the transcriptional program of Tr1 cells, while it limits the suppressive activity of eATP-driven HIF1-α activation on Tr1-cell differentiation.
High local levels of eATP and hypoxia, which activate HIF1-α, characterize the microenvironment of inflamed tissues (Takenaka et al., 2016). Thus, these findings suggest that although IL-27 is produced at sites of inflammation (Fitzgerald et al., 2007), these sites do not support local Tr1 cell differentiation. Instead, the production of IL-27 at local sites may limit inflammation by Tr1 independent mechanisms such as direct inhibitory effects on pathogenic Th17 cells (Batten et al., 2006; Rostami and Ciric, 2013; Stumhofer et al., 2006) and also indirect effects, for example by acting on recruited dendritic cells (Mascanfroni et al., 2013).
Finally, some of the transcriptional modules controlled by AhR in Tr1 cells may be coopted by other cell types. For example, the interaction between AhR and HIF1α controls glucose metabolism in Tr1 cells (Mascanfroni et al., 2015). These effects of HIF1α and AHR on cellular metabolism may contribute to the role of these transcription factors in the differentiation of other T cell types such as Th17 cells (Dang et al., 2011; Shi et al., 2011). Moreover, AhR was implicated in the trans-differentiation of Th17 cells into IL-10 expressing Tr1-like cells (Gagliani et al., 2015), and may also control transcriptional modules coopted by tumor-infiltrating lymphocytes (Regateiro et al., 2011; Whiteside, 2014) and FoxP3+ Tregs that express IL-10 and CD39 (Deaglio et al., 2007; Maynard et al., 2007). Collectively, these findings identify AhR as a potential therapeutic target to for immune modulation.
AhR in Th17 cells
Th17 cells characterized by the production of IL-17A and the expression of the transcription factor ROR-γt participate in the immune response to extracellular bacteria and fungi, and also contribute to the pathology of several autoimmune disorders (Ivanov et al., 2006; Korn et al., 2009). Th17 cell differentiation is initiated by TGFβ in combination with IL-6 or IL-21 (Korn et al., 2007a; Nurieva et al., 2007; Veldhoen et al., 2006). AhR is highly expressed in Th17 cells and its activation by FICZ enhances Th17 cell differentiation and promotes IL-22 production (Quintana et al., 2008; Veldhoen et al., 2009; Veldhoen et al., 2008). Conversely, AhR deficiency impairs IL-22 production by Th17 cells, probably reflecting the role of AhR in facilitating the recruitment of the transcription factor RORγt to the IL-22 promoter (Qiu et al., 2012; Veldhoen et al., 2008; Yeste et al., 2014) (Figure 3).
IL-2 has been shown to inhibit Th17 differentiation (Chen et al., 2011; Laurence et al., 2007; Yang et al., 2011). AhR contributes to Th17 development by inducing the expression of Aiolos, which silences il2 expression (Quintana et al., 2012). AhR also inhibits the activation of STAT5 and STAT1, which interfere with Th17 cell differentiation (Kimura et al., 2008; Veldhoen et al., 2009). Collectively, these data identify a role for AhR during the early stages of the differentiation of Th17 cells, at a stage when they produce significant amounts of IL-10 and their pathogenic potential has not fully developed (McGeachy et al., 2007) (Lee et al., 2012). Indeed, additional factors such as IL-23 are needed for the differentiation of Th17 cells into fully pathogenic effector cells (Korn et al., 2009; Lee et al., 2012). Thus, considering reports on the AhR-dependent differentiation of Th17 cells into IL-10 producing Tr1-like cells (Gagliani et al., 2015), AhR may be associated with plastic Th17 cell populations that require additional signals to complete their differentiation into fully pathogenic Th17 cells or regulatory Tr1 cells (Figure 3).
AhR in OTHER T CELLS
Th22 cells have been identified as a population of CD4+ T cells characterized by the production of IL-22 in the absence of IL-17, differentiated in response to IL-6, IL-21 or IL-23. AhR regulates IL-22 production in these cells, as well as the expression of additional factors important for their function in mucosal immunity (Basu et al., 2012; Duhen et al., 2009; Ramirez et al., 2010; Trifari et al., 2009; Yeste et al., 2014).
AhR signaling also seems to have significant effects on CD8+ T cells. Systemic AhR activation by TCDD results in the indirect suppression of the primary CD8+ T cell response to influenza virus through a mechanism mediated by the modulation of DC function (Lawrence et al., 2006). Additionally, CD8+ T cells from mice exposed to the AhR agonist TCDD during development are less responsive to influenza virus infection later in life (Winans et al., 2015). These findings reflect epigenetic changes that cause long-term defects in CD8+ T-cell function detectable upon viral challenge (Winans et al., 2015).
AhR expression is much higher in tissue-resident CD8+ memory cells (TRMs) compared to other CD8+ T cells subsets. In fact, AhR controls the persistence TRMs in the skin, contributing to their protective role against microbial challenge (Zaid et al., 2014). Moreover, AhR activation by TCDD or Trp metabolite indole-3-lactic acid (ILA) derived from Lactobacillus reuteri has been recently associated to the induction of CD4+CD8α α+ double positive intraepithelial lymphocytes (DP IELs) which display regulatory functions associated to the induction of tolerance to dietary antigens (Cervantes-Barragan et al., 2017). Collectively these findings suggest that, similarly to previous observations on CD4+ T cells, AhR signaling may have major roles in the modulation of specific CD8+ T-cell subsets like TRMs and DP IELs.
AhR signaling also controls γδ T cells, tissue-resident lymphocytes that have important functions in regulating not only the first line immune response at epithelial sites but also tissue homeostasis (Hayday, 2009). Although AhR is expressed by all γδ T-cell subsets, AhR deficiency dramatically reduces the number of intraepithelial lymphocytes which in the skin mainly comprise Vγ3 and in the intestine Vγ5 γδ T cells as well as CD8αα αβ T cells (Kadow et al., 2011; Li et al., 2011). AhR is also essential for the production of IL-22 by IL-17 producing γδ T cells (Cibrian et al., 2016; Martin et al., 2009). These findings point to significant effects of AhR on tissue-resident T cells, supporting further investigations on the role of AhR on non-CD4+ T cells.
In summary, AhR regulates the T-cell response at multiple levels, affecting the expression of effector molecules, enzymes, transcription factors and epigenetic modifiers that control T-cell stability and metabolism. Lineage-specific responses to AhR activation are likely to result ligand-specific effect in combination with cytokine-driven effects on the genomic context that modulate the expression of AhR interaction partners as well as the accessibility of AhR direct and indirect transcriptional targets (Yosef and Regev, 2016). The comprehensive investigation of those interactions should guide the development of AhR-targeted immunomodulatory agents.
AhR IN DENDRITIC CELLS
Dendritic cells (DCs) have a central role in the control of T-cell responses and the regulation of immune tolerance (Guermonprez et al., 2002). AhR controls both the differentiation and function of DCs, with a profound impact on T-cell immunity (Quintana et al., 2015).
The AhR antagonist StemRegenin 1 promotes the expansion of human CD34+ hematopoietic stem cell progenitors (Boitano et al., 2010) and their differentiation into plasmacytoid and myeloid DCs (Thordardottir et al., 2014). Conversely, while AhR deficient murine DCs do not display impaired development in vivo or when differentiated in vitro from bone marrow (Chng et al., 2016; Nguyen et al., 2010), AhR activation by the AhR agonists VAF347, β-naphtoflavone (β-NF) or TCDD inhibits the differentiation of human monocytes into Langerhans DCs in vitro, arresting them at an early precursor cell stage (Platzer et al., 2009). VAF347 and its derivative VAG539 have been described to promote tolerance in vitro in human monocyte-derived DCs (Ettmayer et al., 2006; Lawrence et al., 2008) and in vivo in experimental models of asthma and pancreatic islets allotrasplantation by affecting the function of DCs and lymphocytes (Ettmayer et al., 2006; Hauben et al., 2008). Interestingly, the in vivo effects of VAF347 in DCs were found to be mediated by AhR when analyzed using mice expressing a dominant negative AhR and AhR knock-out mice (Lawrence et al., 2008).
AhR can also affect antigen presentation by DCs. The exposure of bone marrow derived dendritic cells (BMDCs) to TCDD decreases CD11c expression but increases MHC-II and CD86 as well as IL6 and TNFα production by BMDCs. (Bankoti et al., 2010b). Similar results were obtained in splenic DCs treated with TCDD (Bankoti et al., 2010a). Different results, however, were obtained when the AhR agonist ITE was used. AhR stimulation with ITE decreases the expression of MHC-II and co-stimulatory molecules by splenic DCs, as well as the production of Th1 and Th17 polarizing cytokines (Quintana et al., 2010). Indeed, AhR activation by ITE during EAE decreased CD86 and CD103 expression in DCs, reducing their ability to trigger proliferation and proinflammatory cytokine production in T cells, while increasing their production of TGF-β1 and IL-10. Moreover, ITE treatment increased the ability of DCs to expand Foxp3+ Tregs and IL-10+ Tr1 cells and suppress EAE (Quintana et al., 2010). Foxp3+ Tregs are thought to display limited ability to suppress local CNS inflammation (Korn et al., 2007b). Thus, the suppression of EAE by ITE administration likely involves multiple AhR-dependent mechanisms such as the suppression of effector T cells and CNS-resident cells by FoxP3+ Tregs and Tr1 cells in addition to the modulation of DC function. Taken together, however, the reports on the effects of AhR agonists on DCs point to ligand-specific effects of AhR activation in DCs.
Additional evidence for a physiologic regulatory function of AhR in DCs in vivo was recently provided by experiments performed in an ovalbumin-induced asthma model in which AhR deficient mice presented higher inflammatory response as well as Th2 differentiation and higher MHC-II and CD86 expression in DCs (Thatcher et al., 2016). Moreover it has been described during influenza virus infection that AhR signaling modulates the activity of CD103+ DCs and CD11b+ DCs, limiting the activation of protective CD8+ T cells (Jin et al., 2014a). Collectively, this evidence identifies AhR as a potential therapeutic target in DCs to modulate the T-cell response.
Several mechanisms contribute to the modulation of DC function by AhR. AhR induces the expression of the enzymes indoleamine 2,3-dioxigenase (IDO) 1 and 2 (Nguyen et al., 2010; Vogel et al., 2008). IDO1 and IDO2 catalyze Kyn production, which boosts FoxP3+ Treg differentiation (Fallarino et al., 2006). In fact AhR-deficient DCs fail to promote Treg differentiation and boost Th17 cell generation in vitro (Mezrich et al., 2010; Nguyen et al., 2010). In agreement with an immunosuppressive role for AhR in DCs, it has been recently described that in tumor infiltrating tolerogenic DCs the expression of IDO is sustained by an autocrine loop that involves AhR and Kyn (Li et al., 2016). In addition, AhR activation in DCs has been shown to upregulate the expression of the enzymatic machinery involved in the production of retinoic acid (RA) (Quintana et al., 2010), which boosts FoxP3+ Treg differentiation and suppresses effector T cells (Coombes et al., 2007; Mucida et al., 2007; Nolting et al., 2009; Pino-Lagos et al., 2011; Sun et al., 2007).
Although AhR activation in DCs seems to play mostly an anti-inflammatory role, no data are yet available on the expression of AhR by different DC subsets. This is an important point considering reports of DC populations specialized in the differentiation of effector and regulatory T cells (Heink et al., 2017; Quintana, 2016; Takenaka and Quintana, 2017). Similar to what is observed in T cells, it is likely that a combination of ligand-specific effects together with tissue-specific signals results in specific AhR-driven responses better suited to control inflammation in the local microenvironment. For example, intestinal DCs are exposed to relatively high levels of LPS and other bacterial products. Indeed, AhR expression is upregulated in DCs following LPS stimulation through a mechanism mediated by NF-κB (RelA/p50) (Vogel et al., 2014). Interestingly, AhR limits the immune response to LPS challenge through a mechanism mediated by TDO and Kyn (Bessede et al., 2014). Thus, these findings suggest a role for AhR in the tissue-specific adaptation of DCs and other immune cells.
AhR IN THE RESPONSE TO MICROORGANISMS
AhR is increasingly recognized as a regulator of the immune response to microbial pathogens and the commensal flora. AhR plays a significant role in host defense against extracellular (Streptococcus pneumonia, (Vorderstrasse and Lawrence, 2006)) and intracellular (Listeria monocytogenes, (Shi et al., 2007)) bacterial infections, as evidenced by the increased susceptibility to these microorganisms that results from AhR deficiency. In addition, Moura-Alves et al recently identified AhR as a sensor of infection by Pseudomonas aeruginosa and Mycobacterium tuberculosis (Moura-Alves et al., 2014). Indeed, AhR signaling in hematopoietic and non-hematopoietic compartments contributed to the anti-bacterial response, with a critical role for macrophages and neutrophils during P. aeruginosa infection (Moura-Alves et al., 2014). Based on these observations, AhR is likely to play a dual role during bacterial infections, driving certain anti-bacterial activities while simultaneously enforcing “disease tolerance” to minimize immunopathology to the host (Bessede et al., 2014).
AhR-deficient mice show reduced numbers of skin and gut intraepithelial lymphocytes (Li et al., 2011) and intestinal innate lymphoid cells (ILC), resulting in increased susceptibility to Citrobacter rodentium infection (Kiss et al., 2011; Qiu et al., 2012). These cell types, as well as the formation of proper intestinal lymphoid follicles, are controlled by dietary AhR ligands (Kiss et al., 2011; Li et al., 2011). Additionally, AhR activation by microbial metabolites also controls the generation of DP IELs, another important population in the control of intestinal immunity (Cervantes-Barragan et al., 2017). AhR deficiency also affects the commensal flora, probably due to the reduced production of IL-22 (Qiu et al., 2013; Zenewicz et al., 2013). Indeed, the transcription factor ID2 regulates IL-22 expression in ILCs through AhR and IL-23 dependent mechanisms, controlling gut colonization by C. rodentium (Guo et al., 2015). Moreover, IL-22 production by Th22 cells is also controlled by AhR and provides protection from enteropathogenic bacteria (Basu et al., 2012; Yeste et al., 2014). All these data point to an important role of AhR in the control of interactions with microorganisms at environmental interfaces via the regulation of the production of IL-22 and other molecules. Interestingly, the depletion of physiologic AhR ligands by overexpression of Cyp1a1 also increases susceptibility to infection by enteric bacteria (Schiering et al., 2017), highlighting the importance of AhR ligand availability and metabolism on the control of AhR-dependent immune effects.
Although it will not be thoroughly reviewed here, it is important to mention that AhR has also been studied in the context of viral and parasitic infections (Barroso et al., 2016; Sanchez et al., 2010). As we discussed, AhR activation by TCDD worsens influenza virus infection by suppressing CD8+ T cells indirectly, potentially through the modulation of antigen presenting cell function (Jin et al., 2010; Jin et al., 2014a; Lawrence et al., 2006; Warren et al., 2000). More recently, it was reported that constitutive AhR activation reduces the type I IFN (IFN-I) antiviral response (Yamada et al., 2016). AhR expression is induced in some cell types by IFN-I (Rothhammer et al., 2016), suggesting that AhR participates in a negative feedback loop that limits potential IFN-I triggered immunopathology.
AhR IN TUMOR IMMUNOLOGY
Mice with constitutively active AhR develop gastric tumors (Andersson et al., 2002). Similar findings were made in other types of cancer, highlighting tumor-intrinsic roles for AhR in multiple processes including tumor formation, proliferation and tissue invasion (Gramatzki et al., 2009; Jin et al., 2015; Lin et al., 2003; Opitz et al., 2011; Peng et al., 2009; Stanford et al., 2016; Wang et al., 2013; Yang et al., 2008). Conversely, the AhRR has been described as a tumor suppressor (Zudaire et al., 2008).
Interestingly, a positive feedback loop controlled by AhR drives IL-6 expression and promotes IDO expression and Kyn production in tumor cells (Litzenburger et al., 2014). Similarly, stem cell-like cancer cells become resistant to IFNγ-induced apoptosis through an IDO-Kyn-AhR autocrine pathway (Liu et al., 2017). Considering the effects of AhR and Kyn on the T-cell response, these findings suggest that the production of Trp-derived AhR agonists is an important mechanism of tumor immunosuppression.
Indeed, the effects of AhR on tumor development may also involve the modulation of tumor-specific immunity through the AhR-dependent depletion of Trp and the generation of Trp-derived AhR agonists. In DCs, AhR induction by TCDD promotes the expression of IDO, which degrades Trp and while it produces Kyn (Bankoti et al., 2010a; Quintana and Sherr, 2013; Vogel et al., 2008). Low Trp levels induce T-cell cycle arrest and death (Uyttenhove et al., 2003). Moreover, Trp depletion and Kyn down-regulate the TCRζ chain in CD8+ T cells (Fallarino et al., 2006; Mezrich et al., 2010; Nguyen et al., 2010). Thus, based on the role of Kyn in the modulation of T cells and APCs, these findings identify AhR activation by Kyn as an important regulator of both tumor-intrinsic functions and tumor-specific immunity.
These data also highlight the potential of the IDO/TDO-Kyn-AhR axis as a target for cancer immunotherapy. Indeed, IDO and TDO inhibitors are currently under evaluation in clinical trials (Balachandran et al., 2011; Litzenburger et al., 2014; Muller et al., 2005; Pilotte et al., 2012). AhR inhibition, however, may offer a better approach for tumor immunotherapy, likely to avoid the undesired side effects linked to IDO and TDO inhibition (Metz et al., 2012). Because of the tumor-intrinsic roles of AhR (Gabriely et al., 2017), AhR inhibitors may offer the added benefit of acting not only on tumor-specific immunity but also, directly on cancer cells.
CONCLUDING REMARKS
AhR signaling has important effects on the immune response. The development of AhR targeted therapeutics, however, should consider ligand- and cell-specific effects of AhR signaling and how these effects are modulated by the tissue microenvironment. A deep understanding of the mechanisms involved in these ligand- and cell-specific effects, their modulation by the tissue context, the role of different AhR structural conformations and interactions with protein partners, as well as the control of AhR target accessibility by epigenetic modifications should guide the design of therapeutic approaches targeting AhR.
AhR agonists and antagonists may provide new therapeutic approaches for autoimmunity and cancer immunotherapy. However, the expression of AhR in multiple tissues and cell types represents a challenge that should be addressed for the therapeutic target of this pathway. Nanoparticles, for example, may be used to deliver AhR modulators to specific cell-targets in vivo to minimize potential toxicity while maximizing therapeutic effects in specific tissues and/or cell types (Yeste et al., 2012; Yeste et al., 2016). In the context of autoimmune diseases may also control pathogenic activities associated to tissue-resident cells. AhR activation, for example, limits astrocyte-driven neurodegeneration in the CNS (Rothhammer et al., 2016) and favors the re-establishment of intestinal epithelial barrier integrity (Kiss et al., 2011; Li et al., 2011). In the context of cancer therapy, AhR antagonists may have intrinsic effects on tumor cells, affecting their metabolism and invasiveness among other beneficial effects (Gabriely et al., 2017; Opitz et al., 2011). In conclusion, AhR is not only an important regulator of the immune response in health and disease, AhR also provides an attractive target for therapeutic immunomodulation.
Acknowledgments
We would like to thank all members of the Quintana laboratory for helpful discussions. F.J.Q. acknowledges support by the NIH (NS087867, ES025530, AI126880 and AI093903), the National MS Society (RG4111A1 and JF2161-A-5), the American Cancer Society (RSG-14-198-01-LIB) and the International Progressive MS Alliance (PA-1604-08459). C G-V is supported by an Alfonso Martín Escudero Foundation postdoctoral fellowship.
Footnotes
Publisher's Disclaimer: This is a PDF file of an unedited manuscript that has been accepted for publication. As a service to our customers we are providing this early version of the manuscript. The manuscript will undergo copyediting, typesetting, and review of the resulting proof before it is published in its final citable form. Please note that during the production process errors may be discovered which could affect the content, and all legal disclaimers that apply to the journal pertain.
References
- Adachi J, Mori Y, Matsui S, Takigami H, Fujino J, Kitagawa H, Miller CA, 3rd, Kato T, Saeki K, Matsuda T. Indirubin and indigo are potent aryl hydrocarbon receptor ligands present in human urine. J Biol Chem. 2001;276:31475–31478. [Abstract] [Google Scholar]
- Andersson P, McGuire J, Rubio C, Gradin K, Whitelaw ML, Pettersson S, Hanberg A, Poellinger L. A constitutively active dioxin/aryl hydrocarbon receptor induces stomach tumors. Proceedings of the National Academy of Sciences of the United States of America. 2002;99:9990–9995. [Europe PMC free article] [Abstract] [Google Scholar]
- Apetoh L, Quintana FJ, Pot C, Joller N, Xiao S, Kumar D, Burns EJ, Sherr DH, Weiner HL, Kuchroo VK. The aryl hydrocarbon receptor interacts with c-Maf to promote the differentiation of type 1 regulatory T cells induced by IL-27. Nat Immunol. 2010;11:854–861. [Europe PMC free article] [Abstract] [Google Scholar]
- Awasthi A, Carrier Y, Peron JP, Bettelli E, Kamanaka M, Flavell RA, Kuchroo VK, Oukka M, Weiner HL. A dominant function for interleukin 27 in generating interleukin 10-producing anti-inflammatory T cells. Nat Immunol. 2007;8:1380–1389. [Abstract] [Google Scholar]
- Balachandran VP, Cavnar MJ, Zeng S, Bamboat ZM, Ocuin LM, Obaid H, Sorenson EC, Popow R, Ariyan C, Rossi F, et al. Imatinib potentiates antitumor T cell responses in gastrointestinal stromal tumor through the inhibition of Ido. Nat Med. 2011;17:1094–1100. [Europe PMC free article] [Abstract] [Google Scholar]
- Bankoti J, Burnett A, Navarro S, Miller AK, Rase B, Shepherd DM. Effects of TCDD on the fate of naive dendritic cells. Toxicological sciences : an official journal of the Society of Toxicology. 2010a;115:422–434. [Europe PMC free article] [Abstract] [Google Scholar]
- Bankoti J, Rase B, Simones T, Shepherd DM. Functional and phenotypic effects of AhR activation in inflammatory dendritic cells. Toxicology and applied pharmacology. 2010b;246:18–28. [Europe PMC free article] [Abstract] [Google Scholar]
- Barroso A, Gualdron-Lopez M, Esper L, Brant F, Araujo RR, Carneiro MB, Avila TV, Souza DG, Vieira LQ, Rachid MA, et al. The Aryl Hydrocarbon Receptor Modulates Production of Cytokines and Reactive Oxygen Species and Development of Myocarditis during Trypanosoma cruzi Infection. Infect Immun. 2016;84:3071–3082. [Europe PMC free article] [Abstract] [Google Scholar]
- Basu R, O'Quinn DB, Silberger DJ, Schoeb TR, Fouser L, Ouyang W, Hatton RD, Weaver CT. Th22 cells are an important source of IL-22 for host protection against enteropathogenic bacteria. Immunity. 2012;37:1061–1075. [Europe PMC free article] [Abstract] [Google Scholar]
- Batten M, Li J, Yi S, Kljavin NM, Danilenko DM, Lucas S, Lee J, de Sauvage FJ, Ghilardi N. Interleukin 27 limits autoimmune encephalomyelitis by suppressing the development of interleukin 17-producing T cells. Nat Immunol. 2006;7:929–936. [Abstract] [Google Scholar]
- Beischlag TV, Wang S, Rose DW, Torchia J, Reisz-Porszasz S, Muhammad K, Nelson WE, Probst MR, Rosenfeld MG, Hankinson O. Recruitment of the NCoA/SRC-1/p160 family of transcriptional coactivators by the aryl hydrocarbon receptor/aryl hydrocarbon receptor nuclear translocator complex. Mol Cell Biol. 2002;22:4319–4333. [Europe PMC free article] [Abstract] [Google Scholar]
- Bessede A, Gargaro M, Pallotta MT, Matino D, Servillo G, Brunacci C, Bicciato S, Mazza EM, Macchiarulo A, Vacca C, et al. Aryl hydrocarbon receptor control of a disease tolerance defence pathway. Nature. 2014;511:184–190. [Europe PMC free article] [Abstract] [Google Scholar]
- Bittinger MA, Nguyen LP, Bradfield CA. Aspartate aminotransferase generates proagonists of the aryl hydrocarbon receptor. Molecular pharmacology. 2003;64:550–556. [Abstract] [Google Scholar]
- Bjeldanes LF, Kim JY, Grose KR, Bartholomew JC, Bradfield CA. Aromatic hydrocarbon responsiveness-receptor agonists generated from indole-3-carbinol in vitro and in vivo: comparisons with 2,3,7,8-tetrachlorodibenzo-p-dioxin. Proceedings of the National Academy of Sciences of the United States of America. 1991;88:9543–9547. [Europe PMC free article] [Abstract] [Google Scholar]
- Boitano AE, Wang J, Romeo R, Bouchez LC, Parker AE, Sutton SE, Walker JR, Flaveny CA, Perdew GH, Denison MS, et al. Aryl hydrocarbon receptor antagonists promote the expansion of human hematopoietic stem cells. Science. 2010;329:1345–1348. [Europe PMC free article] [Abstract] [Google Scholar]
- Campbell SJ, Henderson CJ, Anthony DC, Davidson D, Clark AJ, Wolf CR. The murine Cyp1a1 gene is expressed in a restricted spatial and temporal pattern during embryonic development. J Biol Chem. 2005;280:5828–5835. [Abstract] [Google Scholar]
- Capdevila JH, Harris RC, Falck JR. Microsomal cytochrome P450 and eicosanoid metabolism. Cell Mol Life Sci. 2002;59:780–789. [Europe PMC free article] [Abstract] [Google Scholar]
- Carver LA, Bradfield CA. Ligand-dependent interaction of the aryl hydrocarbon receptor with a novel immunophilin homolog in vivo. J Biol Chem. 1997;272:11452–11456. [Abstract] [Google Scholar]
- Cervantes-Barragan L, Chai JN, Tianero MD, Di Luccia B, Ahern PP, Merriman J, Cortez VS, Caparon MG, Donia MS, Gilfillan S, et al. Lactobacillus reuteri induces gut intraepithelial CD4+CD8alphaalpha+ T cells. Science. 2017;357:806–810. [Europe PMC free article] [Abstract] [Google Scholar]
- Chen I, Safe S, Bjeldanes L. Indole-3-carbinol and diindolylmethane as aryl hydrocarbon (Ah) receptor agonists and antagonists in T47D human breast cancer cells. Biochemical pharmacology. 1996;51:1069–1076. [Abstract] [Google Scholar]
- Chen Y, Haines CJ, Gutcher I, Hochweller K, Blumenschein WM, McClanahan T, Hammerling G, Li MO, Cua DJ, McGeachy MJ. Foxp3(+) regulatory T cells promote T helper 17 cell development in vivo through regulation of interleukin-2. Immunity. 2011;34:409–421. [Abstract] [Google Scholar]
- Chiaro CR, Patel RD, Marcus CB, Perdew GH. Evidence for an aryl hydrocarbon receptor-mediated cytochrome p450 autoregulatory pathway. Molecular pharmacology. 2007;72:1369–1379. [Abstract] [Google Scholar]
- Chinen I, Nakahama T, Kimura A, Nguyen NT, Takemori H, Kumagai A, Kayama H, Takeda K, Lee S, Hanieh H, et al. The aryl hydrocarbon receptor/microRNA-212/132 axis in T cells regulates IL-10 production to maintain intestinal homeostasis. International immunology. 2015;27:405–415. [Abstract] [Google Scholar]
- Chng SH, Kundu P, Dominguez-Brauer C, Teo WL, Kawajiri K, Fujii-Kuriyama Y, Mak TW, Pettersson S. Ablating the aryl hydrocarbon receptor (AhR) in CD11c+ cells perturbs intestinal epithelium development and intestinal immunity. Sci Rep. 2016;6:23820. [Europe PMC free article] [Abstract] [Google Scholar]
- Choudhary D, Jansson I, Schenkman JB, Sarfarazi M, Stoilov I. Comparative expression profiling of 40 mouse cytochrome P450 genes in embryonic and adult tissues. Arch Biochem Biophys. 2003;414:91–100. [Abstract] [Google Scholar]
- Cibrian D, Saiz ML, de la Fuente H, Sanchez-Diaz R, Moreno-Gonzalo O, Jorge I, Ferrarini A, Vazquez J, Punzon C, Fresno M, et al. CD69 controls the uptake of L-tryptophan through LAT1-CD98 and AhR-dependent secretion of IL-22 in psoriasis. Nat Immunol. 2016;17:985–996. [Europe PMC free article] [Abstract] [Google Scholar]
- Coombes JL, Siddiqui KR, Arancibia-Carcamo CV, Hall J, Sun CM, Belkaid Y, Powrie F. A functionally specialized population of mucosal CD103+ DCs induces Foxp3+ regulatory T cells via a TGF-beta and retinoic acid-dependent mechanism. J Exp Med. 2007;204:1757–1764. [Europe PMC free article] [Abstract] [Google Scholar]
- Dang EV, Barbi J, Yang HY, Jinasena D, Yu H, Zheng Y, Bordman Z, Fu J, Kim Y, Yen HR, et al. Control of T(H)17/T(reg) balance by hypoxia-inducible factor 1. Cell. 2011;146:772–784. [Europe PMC free article] [Abstract] [Google Scholar]
- Dant TA, Lin KL, Bruce DW, Montgomery SA, Kolupaev OV, Bommiasamy H, Bixby LM, Woosley JT, McKinnon KP, Gonzalez FJ, et al. T-cell expression of AhR inhibits the maintenance of pTreg cells in the gastrointestinal tract in acute GVHD. Blood. 2017;130:348–359. [Europe PMC free article] [Abstract] [Google Scholar]
- Davarinos NA, Pollenz RS. Aryl hydrocarbon receptor imported into the nucleus following ligand binding is rapidly degraded via the cytosplasmic proteasome following nuclear export. J Biol Chem. 1999;274:28708–28715. [Abstract] [Google Scholar]
- Deaglio S, Dwyer KM, Gao W, Friedman D, Usheva A, Erat A, Chen JF, Enjyoji K, Linden J, Oukka M, et al. Adenosine generation catalyzed by CD39 and CD73 expressed on regulatory T cells mediates immune suppression. J Exp Med. 2007;204:1257–1265. [Europe PMC free article] [Abstract] [Google Scholar]
- Degner SC, Papoutsis AJ, Selmin O, Romagnolo DF. Targeting of aryl hydrocarbon receptor-mediated activation of cyclooxygenase-2 expression by the indole-3-carbinol metabolite 3,3'-diindolylmethane in breast cancer cells. J Nutr. 2009;139:26–32. [Europe PMC free article] [Abstract] [Google Scholar]
- Denis M, Cuthill S, Wikstrom AC, Poellinger L, Gustafsson JA. Association of the dioxin receptor with the Mr 90,000 heat shock protein: a structural kinship with the glucocorticoid receptor. Biochem Biophys Res Commun. 1988;155:801–807. [Abstract] [Google Scholar]
- Diani-Moore S, Ma Y, Labitzke E, Tao H, David Warren J, Anderson J, Chen Q, Gross SS, Rifkind AB. Discovery and biological characterization of 1-(1H-indol-3-yl)-9H-pyrido[3,4-b]indole as an aryl hydrocarbon receptor activator generated by photoactivation of tryptophan by sunlight. Chem Biol Interact. 2011;193:119–128. [Europe PMC free article] [Abstract] [Google Scholar]
- Dong B, Cheng W, Li W, Zheng J, Wu D, Matsumura F, Vogel CF. FRET analysis of protein tyrosine kinase c-Src activation mediated via aryl hydrocarbon receptor. Biochim Biophys Acta. 2011;1810:427–431. [Europe PMC free article] [Abstract] [Google Scholar]
- Duhen T, Geiger R, Jarrossay D, Lanzavecchia A, Sallusto F. Production of interleukin 22 but not interleukin 17 by a subset of human skin-homing memory T cells. Nat Immunol. 2009;10:857–863. [Abstract] [Google Scholar]
- Durrin LK, Jones PB, Fisher JM, Galeazzi DR, Whitlock JP., Jr 2,3,7,8-Tetrachlorodibenzo-p-dioxin receptors regulate transcription of the cytochrome P1–450 gene. J Cell Biochem. 1987;35:153–160. [Abstract] [Google Scholar]
- Ettmayer P, Mayer P, Kalthoff F, Neruda W, Harrer N, Hartmann G, Epstein MM, Brinkmann V, Heusser C, Woisetschlager M. A novel low molecular weight inhibitor of dendritic cells and B cells blocks allergic inflammation. Am J Respir Crit Care Med. 2006;173:599–606. [Abstract] [Google Scholar]
- Fallarino F, Grohmann U, You S, McGrath BC, Cavener DR, Vacca C, Orabona C, Bianchi R, Belladonna ML, Volpi C, et al. The combined effects of tryptophan starvation and tryptophan catabolites down-regulate T cell receptor zeta-chain and induce a regulatory phenotype in naive T cells. Journal of immunology (Baltimore, Md. : 1950) 2006;176:6752–6761. [Abstract] [Google Scholar]
- Farez MF, Mascanfroni ID, Mendez-Huergo SP, Yeste A, Murugaiyan G, Garo LP, Balbuena Aguirre ME, Patel B, Ysrraelit MC, Zhu C, et al. Melatonin Contributes to the Seasonality of Multiple Sclerosis Relapses. Cell. 2015;162:1338–1352. [Europe PMC free article] [Abstract] [Google Scholar]
- Fernandez-Salguero P, Pineau T, Hilbert DM, McPhail T, Lee SS, Kimura S, Nebert DW, Rudikoff S, Ward JM, Gonzalez FJ. Immune system impairment and hepatic fibrosis in mice lacking the dioxin-binding Ah receptor. Science. 1995;268:722–726. [Abstract] [Google Scholar]
- Fitzgerald DC, Ciric B, Touil T, Harle H, Grammatikopolou J, Das Sarma J, Gran B, Zhang G-X, Rostami A. Suppressive effect of IL-27 on encephalitogenic Th17 cells and the effector phase of experimental autoimmune encephalomyelitis. Journal of immunology (Baltimore, Md. : 1950) 2007;179:3268–3275. [Abstract] [Google Scholar]
- Fontenot JD, Gavin MA, Rudensky AY. Foxp3 programs the development and function of CD4+CD25+ regulatory T cells. Nat Immunol. 2003;4:330–336. [Abstract] [Google Scholar]
- Funatake CJ, Marshall NB, Steppan LB, Mourich DV, Kerkvliet NI. Cutting edge: activation of the aryl hydrocarbon receptor by 2,3,7,8-tetrachlorodibenzop-dioxin generates a population of CD4+ CD25+ cells with characteristics of regulatory T cells. Journal of immunology (Baltimore, Md. : 1950) 2005;175:4184–4188. [Abstract] [Google Scholar]
- Furman DP, Oshchepkova EA, Oshchepkov DY, Shamanina MY, Mordvinov VA. Promoters of the genes encoding the transcription factors regulating the cytokine gene expression in macrophages contain putative binding sites for aryl hydrocarbon receptor. Comput Biol Chem. 2009;33:465–468. [Abstract] [Google Scholar]
- Gabriely G, Wheeler MA, Takenaka MC, Quintana FJ. Role of AHR and HIF-1alpha in Glioblastoma Metabolism. Trends Endocrinol Metab. 2017;28:428–436. [Europe PMC free article] [Abstract] [Google Scholar]
- Gagliani N, Vesely MC, Iseppon A, Brockmann L, Xu H, Palm NW, de Zoete MR, Licona-Limon P, Paiva RS, Ching T, et al. Th17 cells transdifferentiate into regulatory T cells during resolution of inflammation. Nature. 2015;523:221–225. [Europe PMC free article] [Abstract] [Google Scholar]
- Gandhi R, Kumar D, Burns EJ, Nadeau M, Dake B, Laroni A, Kozoriz D, Weiner HL, Quintana FJ. Activation of the aryl hydrocarbon receptor induces human type 1 regulatory T cell-like and Foxp3(+) regulatory T cells. Nat Immunol. 2010;11:846–853. [Europe PMC free article] [Abstract] [Google Scholar]
- Garcia GR, Goodale BC, Wiley MW, La Du JK, Hendrix DA, Tanguay RL. In Vivo Characterization of an AHR-Dependent Long Noncoding RNA Required for Proper Sox9b Expression. Molecular pharmacology. 2017;91:609–619. [Europe PMC free article] [Abstract] [Google Scholar]
- Gillam EM, Notley LM, Cai H, De Voss JJ, Guengerich FP. Oxidation of indole by cytochrome P450 enzymes. Biochemistry. 2000;39:13817–13824. [Abstract] [Google Scholar]
- Goettel JA, Gandhi R, Kenison JE, Yeste A, Murugaiyan G, Sambanthamoorthy S, Griffith AE, Patel B, Shouval DS, Weiner HL, et al. AHR Activation Is Protective against Colitis Driven by T Cells in Humanized Mice. Cell Rep. 2016;17:1318–1329. [Europe PMC free article] [Abstract] [Google Scholar]
- Goldstone HM, Tokunaga S, Schlezinger JJ, Goldstone JV, Stegeman JJ. EZR1: a novel family of highly expressed retroelements induced by TCDD and regulated by a NF-kappaB-like factor in embryos of zebrafish (Danio rerio) Zebrafish. 2012;9:15–25. [Europe PMC free article] [Abstract] [Google Scholar]
- Gordon MW, Yan F, Zhong X, Mazumder PB, Xu-Monette ZY, Zou D, Young KH, Ramos KS, Li Y. Regulation of p53-targeting microRNAs by polycyclic aromatic hydrocarbons: Implications in the etiology of multiple myeloma. Molecular carcinogenesis. 2015;54:1060–1069. [Europe PMC free article] [Abstract] [Google Scholar]
- Gramatzki D, Pantazis G, Schittenhelm J, Tabatabai G, Kohle C, Wick W, Schwarz M, Weller M, Tritschler I. Aryl hydrocarbon receptor inhibition downregulates the TGF-beta/Smad pathway in human glioblastoma cells. Oncogene. 2009;28:2593–2605. [Abstract] [Google Scholar]
- Grenert JP, Sullivan WP, Fadden P, Haystead TA, Clark J, Mimnaugh E, Krutzsch H, Ochel HJ, Schulte TW, Sausville E, et al. The amino-terminal domain of heat shock protein 90 (hsp90) that binds geldanamycin is an ATP/ADP switch domain that regulates hsp90 conformation. J Biol Chem. 1997;272:23843–23850. [Abstract] [Google Scholar]
- Groux H, O'Garra A, Bigler M, Rouleau M, Antonenko S, de Vries JE, Roncarolo MG. A CD4+ T-cell subset inhibits antigen-specific T-cell responses and prevents colitis. Nature. 1997;389:737–742. [Abstract] [Google Scholar]
- Guermonprez P, Valladeau J, Zitvogel L, Thery C, Amigorena S. Antigen presentation and T cell stimulation by dendritic cells. Annu Rev Immunol. 2002;20:621–667. [Abstract] [Google Scholar]
- Guo X, Liang Y, Zhang Y, Lasorella A, Kee BL, Fu YX. Innate Lymphoid Cells Control Early Colonization Resistance against Intestinal Pathogens through ID2-Dependent Regulation of the Microbiota. Immunity. 2015;42:731–743. [Europe PMC free article] [Abstract] [Google Scholar]
- Han Z, Miwa Y, Obikane H, Mitsumata M, Takahashi-Yanaga F, Morimoto S, Sasaguri T. Aryl hydrocarbon receptor mediates laminar fluid shear stress-induced CYP1A1 activation and cell cycle arrest in vascular endothelial cells. Cardiovasc Res. 2008;77:809–818. [Abstract] [Google Scholar]
- Hanieh H, Alzahrani A. MicroRNA-132 suppresses autoimmune encephalomyelitis by inducing cholinergic anti-inflammation: a new Ahr-based exploration. European journal of immunology. 2013;43:2771–2782. [Abstract] [Google Scholar]
- Hankinson O. Role of coactivators in transcriptional activation by the aryl hydrocarbon receptor. Arch Biochem Biophys. 2005;433:379–386. [Abstract] [Google Scholar]
- Hauben E, Gregori S, Draghici E, Migliavacca B, Olivieri S, Woisetschlager M, Roncarolo MG. Activation of the aryl hydrocarbon receptor promotes allograft-specific tolerance through direct and dendritic cell-mediated effects on regulatory T cells. Blood. 2008;112:1214–1222. [Abstract] [Google Scholar]
- Hayday AC. Gammadelta T cells and the lymphoid stress-surveillance response. Immunity. 2009;31:184–196. [Abstract] [Google Scholar]
- Heath-Pagliuso S, Rogers WJ, Tullis K, Seidel SD, Cenijn PH, Brouwer A, Denison MS. Activation of the Ah receptor by tryptophan and tryptophan metabolites. Biochemistry. 1998;37:11508–11515. [Abstract] [Google Scholar]
- Hecht E, Zago M, Sarill M, Rico de Souza A, Gomez A, Matthews J, Hamid Q, Eidelman DH, Baglole CJ. Aryl hydrocarbon receptor-dependent regulation of miR-196a expression controls lung fibroblast apoptosis but not proliferation. Toxicology and applied pharmacology. 2014;280:511–525. [Abstract] [Google Scholar]
- Heink S, Yogev N, Garbers C, Herwerth M, Aly L, Gasperi C, Husterer V, Croxford AL, Moller-Hackbarth K, Bartsch HS, et al. Trans-presentation of IL-6 by dendritic cells is required for the priming of pathogenic TH17 cells. Nat Immunol. 2017;18:74–85. [Europe PMC free article] [Abstract] [Google Scholar]
- Hori S, Nomura T, Sakaguchi S. Control of regulatory T cell development by the transcription factor Foxp3. Science. 2003;299:1057–1061. [Abstract] [Google Scholar]
- Huang Z, Jiang Y, Yang Y, Shao J, Sun X, Chen J, Dong L, Zhang J. 3,3'-Diindolylmethane alleviates oxazolone-induced colitis through Th2/Th17 suppression and Treg induction. Mol Immunol. 2013;53:335–344. [Abstract] [Google Scholar]
- Hubbard TD, Murray IA, Bisson WH, Lahoti TS, Gowda K, Amin SG, Patterson AD, Perdew GH. Adaptation of the human aryl hydrocarbon receptor to sense microbiota-derived indoles. Sci Rep. 2015;5:12689. [Europe PMC free article] [Abstract] [Google Scholar]
- Ikuta T, Eguchi H, Tachibana T, Yoneda Y, Kawajiri K. Nuclear localization and export signals of the human aryl hydrocarbon receptor. J Biol Chem. 1998;273:2895–2904. [Abstract] [Google Scholar]
- Ikuta T, Kobayashi Y, Kawajiri K. Phosphorylation of nuclear localization signal inhibits the ligand-dependent nuclear import of aryl hydrocarbon receptor. Biochem Biophys Res Commun. 2004;317:545–550. [Abstract] [Google Scholar]
- Ikuta T, Tachibana T, Watanabe J, Yoshida M, Yoneda Y, Kawajiri K. Nucleocytoplasmic shuttling of the aryl hydrocarbon receptor. J Biochem. 2000;127:503–509. [Abstract] [Google Scholar]
- Ivanov II, McKenzie BS, Zhou L, Tadokoro CE, Lepelley A, Lafaille JJ, Cua DJ, Littman DR. The orphan nuclear receptor RORgammat directs the differentiation program of proinflammatory IL-17+ T helper cells. Cell. 2006;126:1121–1133. [Abstract] [Google Scholar]
- Jin GB, Moore AJ, Head JL, Neumiller JJ, Lawrence BP. Aryl hydrocarbon receptor activation reduces dendritic cell function during influenza virus infection. Toxicological sciences : an official journal of the Society of Toxicology. 2010;116:514–522. [Europe PMC free article] [Abstract] [Google Scholar]
- Jin GB, Winans B, Martin KC, Lawrence BP. New insights into the role of the aryl hydrocarbon receptor in the function of CD11c(+) cells during respiratory viral infection. European journal of immunology. 2014a;44:1685–1698. [Europe PMC free article] [Abstract] [Google Scholar]
- Jin UH, Kim SB, Safe S. Omeprazole Inhibits Pancreatic Cancer Cell Invasion through a Nongenomic Aryl Hydrocarbon Receptor Pathway. Chem Res Toxicol 2015 [Europe PMC free article] [Abstract] [Google Scholar]
- Jin UH, Lee SO, Sridharan G, Lee K, Davidson LA, Jayaraman A, Chapkin RS, Alaniz R, Safe S. Microbiome-derived tryptophan metabolites and their aryl hydrocarbon receptor-dependent agonist and antagonist activities. Molecular pharmacology. 2014b;85:777–788. [Europe PMC free article] [Abstract] [Google Scholar]
- Joller N, Lozano E, Burkett PR, Patel B, Xiao S, Zhu C, Xia J, Tan TG, Sefik E, Yajnik V, et al. Treg cells expressing the coinhibitory molecule TIGIT selectively inhibit proinflammatory Th1 and Th17 cell responses. Immunity. 2014;40:569–581. [Europe PMC free article] [Abstract] [Google Scholar]
- Kadow S, Jux B, Zahner SP, Wingerath B, Chmill S, Clausen BE, Hengstler J, Esser C. Aryl hydrocarbon receptor is critical for homeostasis of invariant gammadelta T cells in the murine epidermis. Journal of immunology (Baltimore, Md. : 1950) 2011;187:3104–3110. [Abstract] [Google Scholar]
- Kapitulnik J, Gonzalez FJ. Marked endogenous activation of the CYP1A1 and CYP1A2 genes in the congenitally jaundiced Gunn rat. Molecular pharmacology. 1993;43:722–725. [Abstract] [Google Scholar]
- Kaye J, Piryatinsky V, Birnberg T, Hingaly T, Raymond E, Kashi R, Amit-Romach E, Caballero IS, Towfic F, Ator MA, et al. Laquinimod arrests experimental autoimmune encephalomyelitis by activating the aryl hydrocarbon receptor. Proceedings of the National Academy of Sciences of the United States of America. 2016;113:E6145–e6152. [Europe PMC free article] [Abstract] [Google Scholar]
- Kerkvliet NI, Baecher-Steppan L, Smith BB, Youngberg JA, Henderson MC, Buhler DR. Role of the Ah locus in suppression of cytotoxic T lymphocyte activity by halogenated aromatic hydrocarbons (PCBs and TCDD): structure-activity relationships and effects in C57Bl/6 mice congenic at the Ah locus. Fundamental and applied toxicology : official journal of the Society of Toxicology. 1990;14:532–541. [Abstract] [Google Scholar]
- Kerkvliet NI, Shepherd DM, Baecher-Steppan L. T lymphocytes are direct, aryl hydrocarbon receptor (AhR)-dependent targets of 2,3,7,8-tetrachlorodibenzo-p-dioxin (TCDD): AhR expression in both CD4+ and CD8+ T cells is necessary for full suppression of a cytotoxic T lymphocyte response by TCDD. Toxicology and applied pharmacology. 2002;185:146–152. [Abstract] [Google Scholar]
- Kerkvliet NI, Steppan LB, Vorachek W, Oda S, Farrer D, Wong CP, Pham D, Mourich DV. Activation of aryl hydrocarbon receptor by TCDD prevents diabetes in NOD mice and increases Foxp3+ T cells in pancreatic lymph nodes. Immunotherapy. 2009;1:539–547. [Europe PMC free article] [Abstract] [Google Scholar]
- Kewley RJ, Whitelaw ML, Chapman-Smith A. The mammalian basic helix-loop-helix/PAS family of transcriptional regulators. Int J Biochem Cell Biol. 2004;36:189–204. [Abstract] [Google Scholar]
- Kim DW, Gazourian L, Quadri SA, Romieu-Mourez R, Sherr DH, Sonenshein GE. The RelA NF-kappaB subunit and the aryl hydrocarbon receptor (AhR) cooperate to transactivate the c-myc promoter in mammary cells. Oncogene. 2000;19:5498–5506. [Abstract] [Google Scholar]
- Kimura A, Naka T, Nakahama T, Chinen I, Masuda K, Nohara K, Fujii-Kuriyama Y, Kishimoto T. Aryl hydrocarbon receptor in combination with Stat1 regulates LPS-induced inflammatory responses. J Exp Med. 2009;206:2027–2035. [Europe PMC free article] [Abstract] [Google Scholar]
- Kimura A, Naka T, Nohara K, Fujii-Kuriyama Y, Kishimoto T. Aryl hydrocarbon receptor regulates Stat1 activation and participates in the development of Th17 cells. Proceedings of the National Academy of Sciences of the United States of America. 2008;105:9721–9726. [Europe PMC free article] [Abstract] [Google Scholar]
- Kiss EA, Vonarbourg C, Kopfmann S, Hobeika E, Finke D, Esser C, Diefenbach A. Natural aryl hydrocarbon receptor ligands control organogenesis of intestinal lymphoid follicles. Science. 2011;334:1561–1565. [Abstract] [Google Scholar]
- Korn T, Bettelli E, Gao W, Awasthi A, Jager A, Strom TB, Oukka M, Kuchroo VK. IL-21 initiates an alternative pathway to induce proinflammatory T(H)17 cells. Nature. 2007a;448:484–487. [Europe PMC free article] [Abstract] [Google Scholar]
- Korn T, Bettelli E, Oukka M, Kuchroo VK. IL-17 and Th17 Cells. Annu Rev Immunol. 2009;27:485–517. [Abstract] [Google Scholar]
- Korn T, Reddy J, Gao W, Bettelli E, Awasthi A, Petersen TR, Backstrom BT, Sobel RA, Wucherpfennig KW, Strom TB, et al. Myelin-specific regulatory T cells accumulate in the CNS but fail to control autoimmune inflammation. Nat Med. 2007b;13:423–431. [Europe PMC free article] [Abstract] [Google Scholar]
- Lahvis GP, Pyzalski RW, Glover E, Pitot HC, McElwee MK, Bradfield CA. The aryl hydrocarbon receptor is required for developmental closure of the ductus venosus in the neonatal mouse. Molecular pharmacology. 2005;67:714–720. [Abstract] [Google Scholar]
- Lamas B, Richard ML, Leducq V, Pham HP, Michel ML, Da Costa G, Bridonneau C, Jegou S, Hoffmann TW, Natividad JM, et al. CARD9 impacts colitis by altering gut microbiota metabolism of tryptophan into aryl hydrocarbon receptor ligands. Nat Med. 2016;22:598–605. [Europe PMC free article] [Abstract] [Google Scholar]
- Laurence A, Tato CM, Davidson TS, Kanno Y, Chen Z, Yao Z, Blank RB, Meylan F, Siegel R, Hennighausen L, et al. Interleukin-2 signaling via STAT5 constrains T helper 17 cell generation. Immunity. 2007;26:371–381. [Abstract] [Google Scholar]
- Lawrence BP, Denison MS, Novak H, Vorderstrasse BA, Harrer N, Neruda W, Reichel C, Woisetschlager M. Activation of the aryl hydrocarbon receptor is essential for mediating the anti-inflammatory effects of a novel low-molecular-weight compound. Blood. 2008;112:1158–1165. [Europe PMC free article] [Abstract] [Google Scholar]
- Lawrence BP, Roberts AD, Neumiller JJ, Cundiff JA, Woodland DL. Aryl hydrocarbon receptor activation impairs the priming but not the recall of influenza virus-specific CD8+ T cells in the lung. Journal of immunology (Baltimore, Md. : 1950) 2006;177:5819–5828. [Abstract] [Google Scholar]
- Lee Y, Awasthi A, Yosef N, Quintana FJ, Xiao S, Peters A, Wu C, Kleinewietfeld M, Kunder S, Hafler DA, et al. Induction and molecular signature of pathogenic TH17 cells. Nat Immunol. 2012;13:991–999. [Europe PMC free article] [Abstract] [Google Scholar]
- Lees MJ, Peet DJ, Whitelaw ML. Defining the role for XAP2 in stabilization of the dioxin receptor. J Biol Chem. 2003;278:35878–35888. [Abstract] [Google Scholar]
- Li Q, Harden JL, Anderson CD, Egilmez NK. Tolerogenic Phenotype of IFN-gamma-Induced IDO+ Dendritic Cells Is Maintained via an Autocrine IDO−Kynurenine/AhR-IDO Loop. Journal of immunology (Baltimore, Md. : 1950) 2016;197:962–970. [Abstract] [Google Scholar]
- Li Y, Innocentin S, Withers DR, Roberts NA, Gallagher AR, Grigorieva EF, Wilhelm C, Veldhoen M. Exogenous stimuli maintain intraepithelial lymphocytes via aryl hydrocarbon receptor activation. Cell. 2011;147:629–640. [Abstract] [Google Scholar]
- Lin P, Chang H, Ho WL, Wu MH, Su JM. Association of aryl hydrocarbon receptor and cytochrome P4501B1 expressions in human non-small cell lung cancers. Lung Cancer. 2003;42:255–261. [Abstract] [Google Scholar]
- Litzenburger UM, Opitz CA, Sahm F, Rauschenbach KJ, Trump S, Winter M, Ott M, Ochs K, Lutz C, Liu X, et al. Constitutive IDO expression in human cancer is sustained by an autocrine signaling loop involving IL-6, STAT3 and the AHR. Oncotarget. 2014;5:1038–1051. [Europe PMC free article] [Abstract] [Google Scholar]
- Liu Y, Liang X, Yin X, Lv J, Tang K, Ma J, Ji T, Zhang H, Dong W, Jin X, et al. Blockade of IDO-kynurenine-AhR metabolic circuitry abrogates IFN-gamma-induced immunologic dormancy of tumor-repopulating cells. Nature communications. 2017;8:15207. [Europe PMC free article] [Abstract] [Google Scholar]
- Longhi MS, Vuerich M, Kalbasi A, Kenison JE, Yeste A, Csizmadia E, Vaughn B, Feldbrugge L, Mitsuhashi S, Wegiel B, et al. Bilirubin suppresses Th17 immunity in colitis by upregulating CD39. JCI Insight. 2017;2 [Europe PMC free article] [Abstract] [Google Scholar]
- Loub WD, Wattenberg LW, Davis DW. Aryl hydrocarbon hydroxylase induction in rat tissues by naturally occurring indoles of cruciferous plants. J Natl Cancer Inst. 1975;54:985–988. [Abstract] [Google Scholar]
- Ma Q, Baldwin KT. 2,3,7,8-tetrachlorodibenzo-p-dioxin-induced degradation of aryl hydrocarbon receptor (AhR) by the ubiquitin-proteasome pathway. Role of the transcription activaton and DNA binding of AhR. J Biol Chem. 2000;275:8432–8438. [Abstract] [Google Scholar]
- Marshall NB, Vorachek WR, Steppan LB, Mourich DV, Kerkvliet NI. Functional characterization and gene expression analysis of CD4+ CD25+ regulatory T cells generated in mice treated with 2,3,7,8-tetrachlorodibenzo-p-dioxin. Journal of immunology (Baltimore, Md. : 1950) 2008;181:2382–2391. [Europe PMC free article] [Abstract] [Google Scholar]
- Martin B, Hirota K, Cua DJ, Stockinger B, Veldhoen M. Interleukin- 17-producing gammadelta T cells selectively expand in response to pathogen products and environmental signals. Immunity. 2009;31:321–330. [Abstract] [Google Scholar]
- Mascanfroni ID, Takenaka MC, Yeste A, Patel B, Wu Y, Kenison JE, Siddiqui S, Basso AS, Otterbein LE, Pardoll DM, et al. Metabolic control of type 1 regulatory T cell differentiation by AHR and HIF1-alpha. Nat Med. 2015;21:638–646. [Europe PMC free article] [Abstract] [Google Scholar]
- Mascanfroni ID, Yeste A, Vieira SM, Burns EJ, Patel B, Sloma I, Wu Y, Mayo L, Ben-Hamo R, Efroni S, et al. IL-27 acts on DCs to suppress the T cell response and autoimmunity by inducing expression of the immunoregulatory molecule CD39. Nat Immunol. 2013;14:1054–1063. [Europe PMC free article] [Abstract] [Google Scholar]
- Matsumura F. The significance of the nongenomic pathway in mediating inflammatory signaling of the dioxin-activated Ah receptor to cause toxic effects. Biochemical pharmacology. 2009;77:608–626. [Abstract] [Google Scholar]
- Maynard CL, Harrington LE, Janowski KM, Oliver JR, Zindl CL, Rudensky AY, Weaver CT. Regulatory T cells expressing interleukin 10 develop from Foxp3+ and Foxp3− precursor cells in the absence of interleukin 10. Nat Immunol. 2007;8:931–941. [Abstract] [Google Scholar]
- McGeachy MJ, Bak-Jensen KS, Chen Y, Tato CM, Blumenschein W, McClanahan T, Cua DJ. TGF-beta and IL-6 drive the production of IL-17 and IL-10 by T cells and restrain T(H)-17 cell-mediated pathology. Nat Immunol. 2007;8:1390–1397. [Abstract] [Google Scholar]
- Metz R, Rust S, Duhadaway JB, Mautino MR, Munn DH, Vahanian NN, Link CJ, Prendergast GC. IDO inhibits a tryptophan sufficiency signal that stimulates mTOR: A novel IDO effector pathway targeted by D-1-methyl-tryptophan. Oncoimmunology. 2012;1:1460–1468. [Europe PMC free article] [Abstract] [Google Scholar]
- Meyer BK, Perdew GH. Characterization of the AhR-hsp90-XAP2 core complex and the role of the immunophilin-related protein XAP2 in AhR stabilization. Biochemistry. 1999;38:8907–8917. [Abstract] [Google Scholar]
- Mezrich JD, Fechner JH, Zhang X, Johnson BP, Burlingham WJ, Bradfield CA. An interaction between kynurenine and the aryl hydrocarbon receptor can generate regulatory T cells. Journal of immunology (Baltimore, Md. : 1950) 2010;185:3190–3198. [Europe PMC free article] [Abstract] [Google Scholar]
- Miller CA., 3rd Expression of the human aryl hydrocarbon receptor complex in yeast. Activation of transcription by indole compounds. J Biol Chem. 1997;272:32824–32829. [Abstract] [Google Scholar]
- Mimura J, Ema M, Sogawa K, Fujii-Kuriyama Y. Identification of a novel mechanism of regulation of Ah (dioxin) receptor function. Genes Dev. 1999;13:20–25. [Europe PMC free article] [Abstract] [Google Scholar]
- Morales-Hernandez A, Gonzalez-Rico FJ, Roman AC, Rico-Leo E, Alvarez-Barrientos A, Sanchez L, Macia A, Heras SR, Garcia-Perez JL, Merino JM, Fernandez-Salguero PM. Alu retrotransposons promote differentiation of human carcinoma cells through the aryl hydrocarbon receptor. Nucleic acids research. 2016;44:4665–4683. [Europe PMC free article] [Abstract] [Google Scholar]
- Moura-Alves P, Fae K, Houthuys E, Dorhoi A, Kreuchwig A, Furkert J, Barison N, Diehl A, Munder A, Constant P, et al. AhR sensing of bacterial pigments regulates antibacterial defence. Nature. 2014;512:387–392. [Abstract] [Google Scholar]
- Mucida D, Park Y, Kim G, Turovskaya O, Scott I, Kronenberg M, Cheroutre H. Reciprocal TH17 and regulatory T cell differentiation mediated by retinoic acid. Science. 2007;317:256–260. [Abstract] [Google Scholar]
- Muller AJ, DuHadaway JB, Donover PS, Sutanto-Ward E, Prendergast GC. Inhibition of indoleamine 2,3-dioxygenase, an immunoregulatory target of the cancer suppression gene Bin1, potentiates cancer chemotherapy. Nat Med. 2005;11:312–319. [Abstract] [Google Scholar]
- Murray IA, Morales JL, Flaveny CA, Dinatale BC, Chiaro C, Gowdahalli K, Amin S, Perdew GH. Evidence for ligand-mediated selective modulation of aryl hydrocarbon receptor activity. Molecular pharmacology. 2010;77:247–254. [Europe PMC free article] [Abstract] [Google Scholar]
- Murray IA, Reen RK, Leathery N, Ramadoss P, Bonati L, Gonzalez FJ, Peters JM, Perdew GH. Evidence that ligand binding is a key determinant of Ah receptor-mediated transcriptional activity. Arch Biochem Biophys. 2005;442:59–71. [Abstract] [Google Scholar]
- Nair SC, Toran EJ, Rimerman RA, Hjermstad S, Smithgall TE, Smith DF. A pathway of multi-chaperone interactions common to diverse regulatory proteins: estrogen receptor, Fes tyrosine kinase, heat shock transcription factor Hsf1, and the aryl hydrocarbon receptor. Cell Stress Chaperones. 1996;1:237–250. [Europe PMC free article] [Abstract] [Google Scholar]
- Nakahama T, Hanieh H, Nguyen NT, Chinen I, Ripley B, Millrine D, Lee S, Nyati KK, Dubey PK, Chowdhury K, et al. Aryl hydrocarbon receptor-mediated induction of the microRNA-132/212 cluster promotes interleukin-17-producing T-helper cell differentiation. Proceedings of the National Academy of Sciences of the United States of America. 2013;110:11964–11969. [Europe PMC free article] [Abstract] [Google Scholar]
- Nguyen LP, Bradfield CA. The search for endogenous activators of the aryl hydrocarbon receptor. Chem Res Toxicol. 2008;21:102–116. [Europe PMC free article] [Abstract] [Google Scholar]
- Nguyen NT, Kimura A, Nakahama T, Chinen I, Masuda K, Nohara K, Fujii-Kuriyama Y, Kishimoto T. Aryl hydrocarbon receptor negatively regulates dendritic cell immunogenicity via a kynurenine-dependent mechanism. Proceedings of the National Academy of Sciences of the United States of America. 2010;107:19961–19966. [Europe PMC free article] [Abstract] [Google Scholar]
- Nolting J, Daniel C, Reuter S, Stuelten C, Li P, Sucov H, Kim BG, Letterio JJ, Kretschmer K, Kim HJ, von Boehmer H. Retinoic acid can enhance conversion of naive into regulatory T cells independently of secreted cytokines. J Exp Med. 2009;206:2131–2139. [Europe PMC free article] [Abstract] [Google Scholar]
- Nugent LF, Shi G, Vistica BP, Ogbeifun O, Hinshaw SJ, Gery I. ITE, a novel endogenous nontoxic aryl hydrocarbon receptor ligand, efficiently suppresses EAU and T-cell-mediated immunity. Invest Ophthalmol Vis Sci. 2013;54:7463–7469. [Europe PMC free article] [Abstract] [Google Scholar]
- Nurieva R, Yang XO, Martinez G, Zhang Y, Panopoulos AD, Ma L, Schluns K, Tian Q, Watowich SS, Jetten AM, Dong C. Essential autocrine regulation by IL-21 in the generation of inflammatory T cells. Nature. 2007;448:480–483. [Abstract] [Google Scholar]
- Ociepa-Zawal M, Rubis B, Lacinski M, Trzeciak WH. The effect of indole-3-carbinol on the expression of CYP1A1, CYP1B1 and AhR genes and proliferation of MCF-7 cells. Acta Biochim Pol. 2007;54:113–117. [Abstract] [Google Scholar]
- Ohtake F, Baba A, Takada I, Okada M, Iwasaki K, Miki H, Takahashi S, Kouzmenko A, Nohara K, Chiba T, et al. Dioxin receptor is a ligand-dependent E3 ubiquitin ligase. Nature. 2007;446:562–566. [Abstract] [Google Scholar]
- Okudaira N, Iijima K, Koyama T, Minemoto Y, Kano S, Mimori A, Ishizaka Y. Induction of long interspersed nucleotide element-1 (L1) retrotransposition by 6-formylindolo[3,2-b]carbazole (FICZ), a tryptophan photoproduct. Proceedings of the National Academy of Sciences of the United States of America. 2010;107:18487–18492. [Europe PMC free article] [Abstract] [Google Scholar]
- Opitz CA, Litzenburger UM, Sahm F, Ott M, Tritschler I, Trump S, Schumacher T, Jestaedt L, Schrenk D, Weller M, et al. An endogenous tumour-promoting ligand of the human aryl hydrocarbon receptor. Nature. 2011;478:197–203. [Abstract] [Google Scholar]
- Peng TL, Chen J, Mao W, Song X, Chen MH. Aryl hydrocarbon receptor pathway activation enhances gastric cancer cell invasiveness likely through a c-Jun-dependent induction of matrix metalloproteinase-9. BMC Cell Biol. 2009;10:27. [Europe PMC free article] [Abstract] [Google Scholar]
- Perdew GH. Association of the Ah receptor with the 90-kDa heat shock protein. J Biol Chem. 1988;263:13802–13805. [Abstract] [Google Scholar]
- Phelan D, Winter GM, Rogers WJ, Lam JC, Denison MS. Activation of the Ah receptor signal transduction pathway by bilirubin and biliverdin. Arch Biochem Biophys. 1998;357:155–163. [Abstract] [Google Scholar]
- Pilotte L, Larrieu P, Stroobant V, Colau D, Dolusic E, Frederick R, De Plaen E, Uyttenhove C, Wouters J, Masereel B, Van den Eynde BJ. Reversal of tumoral immune resistance by inhibition of tryptophan 2,3-dioxygenase. Proceedings of the National Academy of Sciences of the United States of America. 2012;109:2497–2502. [Europe PMC free article] [Abstract] [Google Scholar]
- Pino-Lagos K, Guo Y, Brown C, Alexander MP, Elgueta R, Bennett KA, De Vries V, Nowak E, Blomhoff R, Sockanathan S, et al. A retinoic acid-dependent checkpoint in the development of CD4+ T cell-mediated immunity. J Exp Med. 2011;208:1767–1775. [Europe PMC free article] [Abstract] [Google Scholar]
- Platzer B, Richter S, Kneidinger D, Waltenberger D, Woisetschlager M, Strobl H. Aryl hydrocarbon receptor activation inhibits in vitro differentiation of human monocytes and Langerhans dendritic cells. Journal of immunology (Baltimore, Md. : 1950) 2009;183:66–74. [Abstract] [Google Scholar]
- Poland A, Knutson JC. 2,3,7,8-tetrachlorodibenzo-p-dioxin and related halogenated aromatic hydrocarbons: examination of the mechanism of toxicity. Annu Rev Pharmacol Toxicol. 1982;22:517–554. [Abstract] [Google Scholar]
- Pongratz I, Mason GG, Poellinger L. Dual roles of the 90-kDa heat shock protein hsp90 in modulating functional activities of the dioxin receptor. Evidence that the dioxin receptor functionally belongs to a subclass of nuclear receptors which require hsp90 both for ligand binding activity and repression of intrinsic DNA binding activity. J Biol Chem. 1992;267:13728–13734. [Abstract] [Google Scholar]
- Pot C, Jin H, Awasthi A, Liu SM, Lai CY, Madan R, Sharpe AH, Karp CL, Miaw SC, Ho IC, Kuchroo VK. Cutting edge: IL-27 induces the transcription factor c-Maf, cytokine IL-21, and the costimulatory receptor ICOS that coordinately act together to promote differentiation of IL-10-producing Tr1 cells. Journal of immunology (Baltimore, Md. : 1950) 2009;183:797–801. [Europe PMC free article] [Abstract] [Google Scholar]
- Qiu J, Guo X, Chen ZM, He L, Sonnenberg GF, Artis D, Fu YX, Zhou L. Group 3 innate lymphoid cells inhibit T-cell-mediated intestinal inflammation through aryl hydrocarbon receptor signaling and regulation of microflora. Immunity. 2013;39:386–399. [Europe PMC free article] [Abstract] [Google Scholar]
- Qiu J, Heller JJ, Guo X, Chen ZM, Fish K, Fu YX, Zhou L. The aryl hydrocarbon receptor regulates gut immunity through modulation of innate lymphoid cells. Immunity. 2012;36:92–104. [Europe PMC free article] [Abstract] [Google Scholar]
- Quintana FJ. The aryl hydrocarbon receptor: a molecular pathway for the environmental control of the immune response. Immunology. 2013;138:183–189. [Abstract] [Google Scholar]
- Quintana FJ. LeA(H)Rning self-control. Cell Res. 2014;24:1155–1156. [Europe PMC free article] [Abstract] [Google Scholar]
- Quintana FJ. Old dog, new tricks: IL-6 cluster signaling promotes pathogenic TH17 cell differentiation. Nat Immunol. 2016;18:8–10. [Abstract] [Google Scholar]
- Quintana FJ, Basso AS, Iglesias AH, Korn T, Farez MF, Bettelli E, Caccamo M, Oukka M, Weiner HL. Control of T(reg) and T(H)17 cell differentiation by the aryl hydrocarbon receptor. Nature. 2008;453:65–71. [Abstract] [Google Scholar]
- Quintana FJ, Jin H, Burns EJ, Nadeau M, Yeste A, Kumar D, Rangachari M, Zhu C, Xiao S, Seavitt J, et al. Aiolos promotes T(H)17 differentiation by directly silencing Il2 expression. Nat Immunol. 2012;13:770–777. [Europe PMC free article] [Abstract] [Google Scholar]
- Quintana FJ, Murugaiyan G, Farez MF, Mitsdoerffer M, Tukpah AM, Burns EJ, Weiner HL. An endogenous aryl hydrocarbon receptor ligand acts on dendritic cells and T cells to suppress experimental autoimmune encephalomyelitis. Proceedings of the National Academy of Sciences of the United States of America. 2010;107:20768–20773. [Europe PMC free article] [Abstract] [Google Scholar]
- Quintana FJ, Sherr DH. Aryl hydrocarbon receptor control of adaptive immunity. Pharmacological reviews. 2013;65:1148–1161. [Europe PMC free article] [Abstract] [Google Scholar]
- Quintana FJ, Yeste A, Mascanfroni ID. Role and therapeutic value of dendritic cells in central nervous system autoimmunity. Cell death and differentiation. 2015;22:215–224. [Europe PMC free article] [Abstract] [Google Scholar]
- Ramirez JM, Brembilla NC, Sorg O, Chicheportiche R, Matthes T, Dayer JM, Saurat JH, Roosnek E, Chizzolini C. Activation of the aryl hydrocarbon receptor reveals distinct requirements for IL-22 and IL-17 production by human T helper cells. European journal of immunology. 2010;40:2450–2459. [Abstract] [Google Scholar]
- Rannug A, Rannug U, Rosenkranz HS, Winqvist L, Westerholm R, Agurell E, Grafstrom AK. Certain photooxidized derivatives of tryptophan bind with very high affinity to the Ah receptor and are likely to be endogenous signal substances. J Biol Chem. 1987;262:15422–15427. [Abstract] [Google Scholar]
- Regateiro FS, Howie D, Nolan KF, Agorogiannis EI, Greaves DR, Cobbold SP, Waldmann H. Generation of anti-inflammatory adenosine by leukocytes is regulated by TGF-beta. European journal of immunology. 2011;41:2955–2965. [Abstract] [Google Scholar]
- Reyes H, Reisz-Porszasz S, Hankinson O. Identification of the Ah receptor nuclear translocator protein (Arnt) as a component of the DNA binding form of the Ah receptor. Science. 1992;256:1193–1195. [Abstract] [Google Scholar]
- Roman AC, Benitez DA, Carvajal-Gonzalez JM, Fernandez-Salguero PM. Genome-wide B1 retrotransposon binds the transcription factors dioxin receptor and Slug and regulates gene expression in vivo. Proceedings of the National Academy of Sciences of the United States of America. 2008;105:1632–1637. [Europe PMC free article] [Abstract] [Google Scholar]
- Rostami A, Ciric B. Role of Th17 cells in the pathogenesis of CNS inflammatory demyelination. J Neurol Sci. 2013;333:76–87. [Europe PMC free article] [Abstract] [Google Scholar]
- Rothhammer V, Mascanfroni ID, Bunse L, Takenaka MC, Kenison JE, Mayo L, Chao CC, Patel B, Yan R, Blain M, et al. Type I interferons and microbial metabolites of tryptophan modulate astrocyte activity and central nervous system inflammation via the aryl hydrocarbon receptor. Nat Med. 2016;22:586–597. [Europe PMC free article] [Abstract] [Google Scholar]
- Rouse M, Singh NP, Nagarkatti PS, Nagarkatti M. Indoles mitigate the development of experimental autoimmune encephalomyelitis by induction of reciprocal differentiation of regulatory T cells and Th17 cells. Br J Pharmacol. 2013;169:1305–1321. [Europe PMC free article] [Abstract] [Google Scholar]
- Rutz S, Noubade R, Eidenschenk C, Ota N, Zeng W, Zheng Y, Hackney J, Ding J, Singh H, Ouyang W. Transcription factor c-Maf mediates the TGF-beta-dependent suppression of IL-22 production in T(H)17 cells. Nat Immunol. 2011;12:1238–1245. [Abstract] [Google Scholar]
- Sanchez Y, Rosado Jde D, Vega L, Elizondo G, Estrada-Muniz E, Saavedra R, Juarez I, Rodriguez-Sosa M. The unexpected role for the aryl hydrocarbon receptor on susceptibility to experimental toxoplasmosis. J Biomed Biotechnol. 2010;2010:505694. [Europe PMC free article] [Abstract] [Google Scholar]
- Schiering C, Wincent E, Metidji A, Iseppon A, Li Y, Potocnik AJ, Omenetti S, Henderson CJ, Wolf CR, Nebert DW, Stockinger B. Feedback control of AHR signalling regulates intestinal immunity. Nature. 2017;542:242–245. [Europe PMC free article] [Abstract] [Google Scholar]
- Schmidt JV, Su GH, Reddy JK, Simon MC, Bradfield CA. Characterization of a murine Ahr null allele: involvement of the Ah receptor in hepatic growth and development. Proceedings of the National Academy of Sciences of the United States of America. 1996;93:6731–6736. [Europe PMC free article] [Abstract] [Google Scholar]
- Schnekenburger M, Peng L, Puga A. HDAC1 bound to the Cyp1a1 promoter blocks histone acetylation associated with Ah receptor-mediated trans-activation. Biochim Biophys Acta. 2007;1769:569–578. [Europe PMC free article] [Abstract] [Google Scholar]
- Schroeder JC, Dinatale BC, Murray IA, Flaveny CA, Liu Q, Laurenzana EM, Lin JM, Strom SC, Omiecinski CJ, Amin S, Perdew GH. The uremic toxin 3-indoxyl sulfate is a potent endogenous agonist for the human aryl hydrocarbon receptor. Biochemistry. 2010;49:393–400. [Europe PMC free article] [Abstract] [Google Scholar]
- Seidel SD, Winters GM, Rogers WJ, Ziccardi MH, Li V, Keser B, Denison MS. Activation of the Ah receptor signaling pathway by prostaglandins. J Biochem Mol Toxicol. 2001;15:187–196. [Abstract] [Google Scholar]
- Shi LZ, Faith NG, Nakayama Y, Suresh M, Steinberg H, Czuprynski CJ. The aryl hydrocarbon receptor is required for optimal resistance to Listeria monocytogenes infection in mice. Journal of immunology (Baltimore, Md. : 1950) 2007;179:6952–6962. [Europe PMC free article] [Abstract] [Google Scholar]
- Shi LZ, Wang R, Huang G, Vogel P, Neale G, Green DR, Chi H. HIF1alpha-dependent glycolytic pathway orchestrates a metabolic checkpoint for the differentiation of TH17 and Treg cells. J Exp Med. 2011;208:1367–1376. [Europe PMC free article] [Abstract] [Google Scholar]
- Sinal CJ, Bend JR. Aryl hydrocarbon receptor-dependent induction of cyp1a1 by bilirubin in mouse hepatoma hepa 1c1c7 cells. Molecular pharmacology. 1997;52:590–599. [Abstract] [Google Scholar]
- Singh NP, Singh UP, Rouse M, Zhang J, Chatterjee S, Nagarkatti PS, Nagarkatti M. Dietary Indoles Suppress Delayed-Type Hypersensitivity by Inducing a Switch from Proinflammatory Th17 Cells to Anti-Inflammatory Regulatory T Cells through Regulation of MicroRNA. Journal of immunology (Baltimore, Md. : 1950) 2016;196:1108–1122. [Europe PMC free article] [Abstract] [Google Scholar]
- Singh NP, Singh UP, Singh B, Price RL, Nagarkatti M, Nagarkatti PS. Activation of aryl hydrocarbon receptor (AhR) leads to reciprocal epigenetic regulation of FoxP3 and IL-17 expression and amelioration of experimental colitis. PloS one. 2011;6:e23522. [Europe PMC free article] [Abstract] [Google Scholar]
- Smirnova A, Wincent E, Vikstrom Bergander L, Alsberg T, Bergman J, Rannug A, Rannug U. Evidence for New Light-Independent Pathways for Generation of the Endogenous Aryl Hydrocarbon Receptor Agonist FICZ. Chem Res Toxicol. 2016;29:75–86. [Abstract] [Google Scholar]
- Song J, Clagett-Dame M, Peterson RE, Hahn ME, Westler WM, Sicinski RR, DeLuca HF. A ligand for the aryl hydrocarbon receptor isolated from lung. Proc Natl Acad Sci U S A. 2002;99:14694–14699. [Europe PMC free article] [Abstract] [Google Scholar]
- Spolski R, Kim HP, Zhu W, Levy DE, Leonard WJ. IL-21 mediates suppressive effects via its induction of IL-10. Journal of immunology (Baltimore, Md. : 1950) 2009;182:2859–2867. [Europe PMC free article] [Abstract] [Google Scholar]
- Stanford EA, Ramirez-Cardenas A, Wang Z, Novikov O, Alamoud K, Koutrakis P, Mizgerd JP, Genco CA, Kukuruzinska M, Monti S, et al. Role for the aryl hydrocarbon receptor and diverse ligands in oral squamous cell carcinoma migration and tumorigenesis. Mol Cancer Res. 2016;14:696–706. [Europe PMC free article] [Abstract] [Google Scholar]
- Stockinger B, Veldhoen M, Hirota K. Modulation of Th17 development and function by activation of the aryl hydrocarbon receptor--the role of endogenous ligands. European journal of immunology. 2009;39:652–654. [Abstract] [Google Scholar]
- Stumhofer JS, Laurence A, Wilson EH, Huang E, Tato CM, Johnson LM, Villarino AV, Huang Q, Yoshimura A, Sehy D, et al. Interleukin 27 negatively regulates the development of interleukin 17-producing T helper cells during chronic inflammation of the central nervous system. Nat Immunol. 2006;7:937–945. [Abstract] [Google Scholar]
- Sun CM, Hall JA, Blank RB, Bouladoux N, Oukka M, Mora JR, Belkaid Y. Small intestine lamina propria dendritic cells promote de novo generation of Foxp3 T reg cells via retinoic acid. J Exp Med. 2007;204:1775–1785. [Europe PMC free article] [Abstract] [Google Scholar]
- Takenaka MC, Quintana FJ. Tolerogenic dendritic cells. Semin Immunopathol. 2017;39:113–120. [Europe PMC free article] [Abstract] [Google Scholar]
- Takenaka MC, Robson S, Quintana FJ. Regulation of the T Cell Response by CD39. Trends Immunol. 2016;37:427–439. [Europe PMC free article] [Abstract] [Google Scholar]
- Thatcher TH, Williams MA, Pollock SJ, McCarthy CE, Lacy SH, Phipps RP, Sime PJ. Endogenous ligands of the aryl hydrocarbon receptor regulate lung dendritic cell function. Immunology. 2016;147:41–54. [Abstract] [Google Scholar]
- Thordardottir S, Hangalapura BN, Hutten T, Cossu M, Spanholtz J, Schaap N, Radstake TR, van der Voort R, Dolstra H. The aryl hydrocarbon receptor antagonist StemRegenin 1 promotes human plasmacytoid and myeloid dendritic cell development from CD34+ hematopoietic progenitor cells. Stem Cells Dev. 2014;23:955–967. [Abstract] [Google Scholar]
- Trifari S, Kaplan CD, Tran EH, Crellin NK, Spits H. Identification of a human helper T cell population that has abundant production of interleukin 22 and is distinct from T(H)-17, T(H)1 and T(H)2 cells. Nat Immunol. 2009;10:864–871. [Abstract] [Google Scholar]
- Tsuji N, Fukuda K, Nagata Y, Okada H, Haga A, Hatakeyama S, Yoshida S, Okamoto T, Hosaka M, Sekine K, et al. The activation mechanism of the aryl hydrocarbon receptor (AhR) by molecular chaperone HSP90. FEBS Open Bio. 2014;4:796–803. [Europe PMC free article] [Abstract] [Google Scholar]
- Uyttenhove C, Pilotte L, Theate I, Stroobant V, Colau D, Parmentier N, Boon T, Van den Eynde BJ. Evidence for a tumoral immune resistance mechanism based on tryptophan degradation by indoleamine 2,3-dioxygenase. Nat Med. 2003;9:1269–1274. [Abstract] [Google Scholar]
- Veldhoen M, Hirota K, Christensen J, O'Garra A, Stockinger B. Natural agonists for aryl hydrocarbon receptor in culture medium are essential for optimal differentiation of Th17 T cells. J Exp Med. 2009;206:43–49. [Europe PMC free article] [Abstract] [Google Scholar]
- Veldhoen M, Hirota K, Westendorf AM, Buer J, Dumoutier L, Renauld JC, Stockinger B. The aryl hydrocarbon receptor links TH17-cell-mediated autoimmunity to environmental toxins. Nature. 2008;453:106–109. [Abstract] [Google Scholar]
- Veldhoen M, Hocking RJ, Atkins CJ, Locksley RM, Stockinger B. TGFbeta in the context of an inflammatory cytokine milieu supports de novo differentiation of IL-17-producing T cells. Immunity. 2006;24:179–189. [Abstract] [Google Scholar]
- Vikstrom Bergander L, Cai W, Klocke B, Seifert M, Pongratz I. Tryptamine serves as a proligand of the AhR transcriptional pathway whose activation is dependent of monoamine oxidases. Mol Endocrinol. 2012;26:1542–1551. [Europe PMC free article] [Abstract] [Google Scholar]
- Vogel CF, Goth SR, Dong B, Pessah IN, Matsumura F. Aryl hydrocarbon receptor signaling mediates expression of indoleamine 2,3-dioxygenase. Biochem Biophys Res Commun. 2008;375:331–335. [Europe PMC free article] [Abstract] [Google Scholar]
- Vogel CF, Khan EM, Leung PS, Gershwin ME, Chang WL, Wu D, Haarmann-Stemmann T, Hoffmann A, Denison MS. Cross-talk between aryl hydrocarbon receptor and the inflammatory response: a role for nuclear factor-kappaB. J Biol Chem. 2014;289:1866–1875. [Europe PMC free article] [Abstract] [Google Scholar]
- Vogel CF, Li W, Sciullo E, Newman J, Hammock B, Reader JR, Tuscano J, Matsumura F. Pathogenesis of aryl hydrocarbon receptor-mediated development of lymphoma is associated with increased cyclooxygenase-2 expression. Am J Pathol. 2007a;171:1538–1548. [Europe PMC free article] [Abstract] [Google Scholar]
- Vogel CF, Matsumura F. A new cross-talk between the aryl hydrocarbon receptor and RelB, a member of the NF-kappaB family. Biochemical pharmacology. 2009;77:734–745. [Europe PMC free article] [Abstract] [Google Scholar]
- Vogel CF, Sciullo E, Li W, Wong P, Lazennec G, Matsumura F. RelB, a new partner of aryl hydrocarbon receptor-mediated transcription. Mol Endocrinol. 2007b;21:2941–2955. [Europe PMC free article] [Abstract] [Google Scholar]
- Vorderstrasse BA, Lawrence BP. Protection against lethal challenge with Streptococcus pneumoniae is conferred by aryl hydrocarbon receptor activation but is not associated with an enhanced inflammatory response. Infect Immun. 2006;74:5679–5686. [Europe PMC free article] [Abstract] [Google Scholar]
- Wang K, Li Y, Jiang YZ, Dai CF, Patankar MS, Song JS, Zheng J. An endogenous aryl hydrocarbon receptor ligand inhibits proliferation and migration of human ovarian cancer cells. Cancer Lett. 2013;340:63–71. [Europe PMC free article] [Abstract] [Google Scholar]
- Wang S, Hankinson O. Functional involvement of the Brahma/SWI2-related gene 1 protein in cytochrome P4501A1 transcription mediated by the aryl hydrocarbon receptor complex. J Biol Chem. 2002;277:11821–11827. [Abstract] [Google Scholar]
- Warren TK, Mitchell KA, Lawrence BP. Exposure to 2,3,7,8-tetrachlorodibenzo-p-dioxin (TCDD) suppresses the humoral and cell-mediated immune responses to influenza A virus without affecting cytolytic activity in the lung. Toxicological sciences : an official journal of the Society of Toxicology. 2000;56:114–123. [Abstract] [Google Scholar]
- Weems JM, Yost GS. 3-Methylindole metabolites induce lung CYP1A1 and CYP2F1 enzymes by AhR and non-AhR mechanisms, respectively. Chem Res Toxicol. 2010;23:696–704. [Europe PMC free article] [Abstract] [Google Scholar]
- Whiteside TL. Induced regulatory T cells in inhibitory microenvironments created by cancer. Expert Opin Biol Ther. 2014;14:1411–1425. [Europe PMC free article] [Abstract] [Google Scholar]
- Wikoff WR, Anfora AT, Liu J, Schultz PG, Lesley SA, Peters EC, Siuzdak G. Metabolomics analysis reveals large effects of gut microflora on mammalian blood metabolites. Proceedings of the National Academy of Sciences of the United States of America. 2009;106:3698–3703. [Europe PMC free article] [Abstract] [Google Scholar]
- Wilson SR, Joshi AD, Elferink CJ. The tumor suppressor Kruppel-like factor 6 is a novel aryl hydrocarbon receptor DNA binding partner. J Pharmacol Exp Ther. 2013;345:419–429. [Europe PMC free article] [Abstract] [Google Scholar]
- Winans B, Nagari A, Chae M, Post CM, Ko CI, Puga A, Kraus WL, Lawrence BP. Linking the aryl hydrocarbon receptor with altered DNA methylation patterns and developmentally induced aberrant antiviral CD8+ T cell responses. Journal of immunology (Baltimore, Md. : 1950) 2015;194:4446–4457. [Europe PMC free article] [Abstract] [Google Scholar]
- Wincent E, Amini N, Luecke S, Glatt H, Bergman J, Crescenzi C, Rannug A, Rannug U. The suggested physiologic aryl hydrocarbon receptor activator and cytochrome P4501 substrate 6-formylindolo[3,2-b]carbazole is present in humans. J Biol Chem. 2009;284:2690–2696. [Abstract] [Google Scholar]
- Wincent E, Bengtsson J, Mohammadi Bardbori A, Alsberg T, Luecke S, Rannug U, Rannug A. Inhibition of cytochrome P4501-dependent clearance of the endogenous agonist FICZ as a mechanism for activation of the aryl hydrocarbon receptor. Proceedings of the National Academy of Sciences of the United States of America. 2012;109:4479–4484. [Europe PMC free article] [Abstract] [Google Scholar]
- Wragg D, Mwacharo JM, Alcalde JA, Wang C, Han JL, Gongora J, Gourichon D, Tixier-Boichard M, Hanotte O. Endogenous retrovirus EAV-HP linked to blue egg phenotype in Mapuche fowl. PloS one. 2013;8:e71393. [Europe PMC free article] [Abstract] [Google Scholar]
- Wu HY, Quintana FJ, da Cunha AP, Dake BT, Koeglsperger T, Starossom SC, Weiner HL. In Vivo Induction of Tr1 Cells via Mucosal Dendritic Cells and AHR Signaling. PloS one. 2011;6:e23618. [Europe PMC free article] [Abstract] [Google Scholar]
- Yamada T, Horimoto H, Kameyama T, Hayakawa S, Yamato H, Dazai M, Takada A, Kida H, Bott D, Zhou AC, et al. Constitutive aryl hydrocarbon receptor signaling constrains type I interferon-mediated antiviral innate defense. Nat Immunol. 2016;17:687–694. [Abstract] [Google Scholar]
- Yang X, Solomon S, Fraser LR, Trombino AF, Liu D, Sonenshein GE, Hestermann EV, Sherr DH. Constitutive regulation of CYP1B1 by the aryl hydrocarbon receptor (AhR) in pre-malignant and malignant mammary tissue. J Cell Biochem. 2008;104:402–417. [Abstract] [Google Scholar]
- Yang XP, Ghoreschi K, Steward-Tharp SM, Rodriguez-Canales J, Zhu J, Grainger JR, Hirahara K, Sun HW, Wei L, Vahedi G, et al. Opposing regulation of the locus encoding IL-17 through direct, reciprocal actions of STAT3 and STAT5. Nat Immunol. 2011;12:247–254. [Europe PMC free article] [Abstract] [Google Scholar]
- Yeste A, Mascanfroni ID, Nadeau M, Burns EJ, Tukpah AM, Santiago A, Wu C, Patel B, Kumar D, Quintana FJ. IL-21 induces IL-22 production in CD4+ T cells. Nature communications. 2014;5:3753. [Europe PMC free article] [Abstract] [Google Scholar]
- Yeste A, Nadeau M, Burns EJ, Weiner HL, Quintana FJ. Nanoparticle-mediated codelivery of myelin antigen and a tolerogenic small molecule suppresses experimental autoimmune encephalomyelitis. Proceedings of the National Academy of Sciences of the United States of America. 2012;109:11270–11275. [Europe PMC free article] [Abstract] [Google Scholar]
- Yeste A, Takenaka MC, Mascanfroni ID, Nadeau M, Kenison JE, Patel B, Tukpah AM, Babon JA, DeNicola M, Kent SC, et al. Tolerogenic nanoparticles inhibit T cell-mediated autoimmunity through SOCS2. Sci Signal. 2016;9:ra61. [Abstract] [Google Scholar]
- Yosef N, Regev A. Writ large: Genomic dissection of the effect of cellular environment on immune response. Science. 2016;354:64–68. [Europe PMC free article] [Abstract] [Google Scholar]
- Zaid A, Mackay LK, Rahimpour A, Braun A, Veldhoen M, Carbone FR, Manton JH, Heath WR, Mueller SN. Persistence of skin-resident memory T cells within an epidermal niche. Proceedings of the National Academy of Sciences of the United States of America. 2014;111:5307–5312. [Europe PMC free article] [Abstract] [Google Scholar]
- Zelante T, Iannitti RG, Cunha C, De Luca A, Giovannini G, Pieraccini G, Zecchi R, D'Angelo C, Massi-Benedetti C, Fallarino F, et al. Tryptophan catabolites from microbiota engage aryl hydrocarbon receptor and balance mucosal reactivity via interleukin-22. Immunity. 2013;39:372–385. [Abstract] [Google Scholar]
- Zenewicz LA, Yin X, Wang G, Elinav E, Hao L, Zhao L, Flavell RA. IL-22 deficiency alters colonic microbiota to be transmissible and colitogenic. Journal of immunology (Baltimore, Md. : 1950) 2013;190:5306–5312. [Europe PMC free article] [Abstract] [Google Scholar]
- Zhang S, Kim K, Jin UH, Pfent C, Cao H, Amendt B, Liu X, Wilson-Robles H, Safe S. Aryl hydrocarbon receptor agonists induce microRNA-335 expression and inhibit lung metastasis of estrogen receptor negative breast cancer cells. Molecular cancer therapeutics. 2012;11:108–118. [Europe PMC free article] [Abstract] [Google Scholar]
- Zudaire E, Cuesta N, Murty V, Woodson K, Adams L, Gonzalez N, Martinez A, Narayan G, Kirsch I, Franklin W, et al. The aryl hydrocarbon receptor repressor is a putative tumor suppressor gene in multiple human cancers. J Clin Invest. 2008;118:640–650. [Europe PMC free article] [Abstract] [Google Scholar]
Citations & impact
Impact metrics
Article citations
Kynurenine-AhR reduces T-cell infiltration and induces a delayed T-cell immune response by suppressing the STAT1-CXCL9/CXCL10 axis in tuberculosis.
Cell Mol Immunol, 22 Oct 2024
Cited by: 0 articles | PMID: 39438693
Combination screen in multi-cell type tumor spheroids reveals interaction between aryl hydrocarbon receptor antagonists and E1 ubiquitin-activating enzyme inhibitor.
SLAS Discov, 29(7):100186, 01 Oct 2024
Cited by: 0 articles | PMID: 39362362
Human and gut microbiota synergy in a metabolically active superorganism: a cardiovascular perspective.
Front Cardiovasc Med, 11:1411306, 11 Oct 2024
Cited by: 0 articles | PMID: 39465131 | PMCID: PMC11502352
Review Free full text in Europe PMC
The aryl hydrocarbon receptor and FOS mediate cytotoxicity induced by Acinetobacter baumannii.
Nat Commun, 15(1):7939, 11 Sep 2024
Cited by: 0 articles | PMID: 39261458 | PMCID: PMC11390868
Identification of a Compound Inhibiting Both the Enzymatic and Nonenzymatic Functions of Indoleamine 2,3-Dioxygenase 1.
ACS Pharmacol Transl Sci, 7(10):3056-3070, 12 Sep 2024
Cited by: 0 articles | PMID: 39421661
Go to all (423) article citations
Other citations
Similar Articles
To arrive at the top five similar articles we use a word-weighted algorithm to compare words from the Title and Abstract of each citation.
The Aryl Hydrocarbon Receptor in Immunity: Tools and Potential.
Methods Mol Biol, 1371:239-257, 01 Jan 2016
Cited by: 22 articles | PMID: 26530806
Review
Aryl hydrocarbon receptor control of adaptive immunity.
Pharmacol Rev, 65(4):1148-1161, 01 Aug 2013
Cited by: 195 articles | PMID: 23908379 | PMCID: PMC3799235
Review Free full text in Europe PMC
Therapeutic potential of aryl hydrocarbon receptor in autoimmunity.
Inflammopharmacology, 28(1):63-81, 15 Oct 2019
Cited by: 13 articles | PMID: 31617124
Review
The aryl hydrocarbon receptor in immune regulation and autoimmune pathogenesis.
J Autoimmun, 138:103049, 23 May 2023
Cited by: 5 articles | PMID: 37229809
Review
Funding
Funders who supported this work.
Alfonso Martín Escudero Foundation
American Cancer Society (1)
Grant ID: RSG-14-198-01-LIB
Multiple Sclerosis Society (4)
Grant ID: 01
Grant ID: PA-1604-08459
Grant ID: 14
Grant ID: 198
NIAID NIH HHS (5)
Grant ID: R01 AI093903
Grant ID: R01 AI126880
Grant ID: R56 AI093903
Grant ID: K99 AI075285
Grant ID: R00 AI075285
NIEHS NIH HHS (1)
Grant ID: R01 ES025530
NIH (4)
Grant ID: AI126880
Grant ID: NS087867
Grant ID: AI093903
Grant ID: ES025530
NINDS NIH HHS (1)
Grant ID: R21 NS087867
National MS Society (2)
Grant ID: RG4111A1
Grant ID: JF2161-A-5