Abstract
Free full text
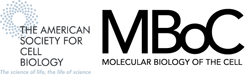
RhoA Inactivation by p190RhoGAP Regulates Cell Spreading and Migration by Promoting Membrane Protrusion and Polarity
Abstract
The binding of extracellular matrix proteins to integrins triggers rearrangements in the actin cytoskeleton by regulating the Rho family of small GTPases. The signaling events that mediate changes in the activity of Rho proteins in response to the extracellular matrix remain largely unknown. We have demonstrated in previous studies that integrin signaling transiently suppresses RhoA activity through stimulation of p190RhoGAP. Here, we investigated the biological significance of adhesion-dependent RhoA inactivation by manipulating p190RhoGAP signaling in Rat1 fibroblasts. The inhibition of RhoA activity that is induced transiently by adhesion was antagonized by expression of dominant negative p190RhoGAP. This resulted in impaired cell spreading on a fibronectin substrate, reduced cell protrusion, and premature assembly of stress fibers. Conversely, overexpression of p190RhoGAP augmented cell spreading. Dominant negative p190RhoGAP elevated RhoA activity in cells on fibronectin and inhibited migration, whereas overexpression of the wild-type GAP decreased RhoA activity, promoted the formation of membrane protrusions, and enhanced motility. Cells expressing dominant negative p190RhoGAP, but not control cells or cells overexpressing the wild-type GAP, were unable to establish polarity in the direction of migration. Taken together, these data demonstrate that integrin-triggered RhoA inhibition by p190RhoGAP enhances spreading and migration by regulating cell protrusion and polarity.
INTRODUCTION
Rho family GTPases serve as molecular switches, transducing signals from the extracellular environment to elicit cellular responses such as changes in gene expression, morphology, and migration (Hall, 1998; Sastry and Burridge, 2000). Of the 14 known Rho proteins, Cdc42, Rac1, and RhoA are the most thoroughly characterized (Takai et al., 2001). Each of these GTPases contributes to cell motility by stimulating rearrangements in the actin cytoskeleton. Cdc42 and Rac1 stimulate the formation of filopodia and lamellipodia, respectively (Ridley et al., 1992; Kozma et al., 1995; Nobes and Hall, 1995). Both of these protrusive structures allow cells to extend into new areas. Accordingly, filopodia and lamellipodia are commonly found along the circumference of spreading cells and at the leading edge of migrating cells (Hall, 1998). In addition, Cdc42 is necessary for the establishment of polarity (Nobes and Hall, 1999; Erickson and Cerione, 2001).
Active RhoA stimulates the formation of focal adhesions and stress fibers (Ridley and Hall, 1992). Induction of these structures is thought to occur via the concerted activities of the RhoA effectors Dia and Rho kinase/ROCK/ROK but may involve other targets (Kimura et al., 1996; Watanabe et al., 1999). Rho kinase stimulates myosin-based contractility by directly and indirectly elevating phosphorylation of the regulatory myosin light chain (Kaibuchi et al., 1999). The resulting activation of myosin triggers myosin filament formation and bundling of filamentous actin into stress fibers. The tension exerted by stress fibers is responsible for the maturation of focal complexes into focal adhesions by aggregating integrins and their associated proteins (Chrzanowska-Wodnicka and Burridge, 1996). Precise regulation of RhoA is crucial for efficient cell migration. Although some RhoA activity is required for migration, possibly to maintain sufficient adhesion to the substrate, high RhoA activity inhibits movement (Takaishi et al., 1994; Ridley et al., 1995; Nobes and Hall, 1999).
Because Rho proteins are involved in dynamic cellular processes, such as migration, their activity is tightly controlled by both positive and negative regulators (Van Aelst and D'Souza-Schorey, 1997). These GTPases are activated by guanine nucleotide exchange factors (GEFs), which catalyze the release of GDP, allowing GTP to bind and thereby activate the proteins. Rho proteins return to an inactive state upon hydrolysis of GTP to GDP, a reaction that is potentiated by GTPase-activating proteins (GAPs). Rho GTPases are also regulated by guanine nucleotide disassociation inhibitors, which extract Rho proteins from the plasma membrane (Olofsson, 1999). Deciphering how extracellular signals alter the activity of GEFs, GAPs, and guanine nucleotide disassociation inhibitors is critical to understanding the regulation of Rho family GTPases. Originally it was postulated that adhesion was necessary, but not sufficient, for activation of RhoA (Hotchin and Hall, 1995). Subsequent studies demonstrated that adhesion to fibronectin or integrin engagement with arginine, glycine, and aspartic acid (RGD)-containing peptides is sufficient for stimulating RhoA-dependent stress fibers (Barry et al., 1997). Ren et al. (1999) directly measured RhoA activity, revealing that adhesion to fibronectin regulates RhoA activity in a triphasic manner. RhoA is rapidly and transiently inhibited (10–30 min) when cells first bind to fibronectin. This initial inactivation is followed by an activation phase that peaks between 60 and 90 min. In the final phase, RhoA activity gradually decreases, by 2–3 h, to a level slightly higher than that of the initial inhibition.
We demonstrated in earlier studies that the initial integrin-triggered inhibition of RhoA is mediated by c-Src–dependent activation of p190RhoGAP (Arthur et al., 2000). Here we have examined the biological significance of this RhoA inactivation in cell spreading and migration. Our results suggest that localized RhoA inactivation by p190RhoGAP in response to integrin-mediated adhesion contributes to efficient cell spreading and migration by enhancing both membrane protrusion and cell polarity.
MATERIALS AND METHODS
Generation of Cell Lines
Rat1 fibroblasts were transfected with the use of LipofectAMINE PLUS (Life Technologies, Rockville, MD) with 1.05 μg of pPUR (Clontech, Palo Alto, CA) only or cotransfected with 0.05 μg of pPUR and 1.0 μg of pKH3-p190RhoGAP or pKH3-p190RhoGAPR1283A (Tatsis et al., 1998) and then selected in 10 μg/ml puromycin (Sigma, St. Louis, MO). Clonal lines were established and screened for expression by immunoblotting with monoclonal antibodies against hemagglutinin epitope (HA; Covance, Richmond, CA) and p190RhoGAP (BD Transduction Laboratories, Lexington, KY). Ten pPUR clones (mock), five HA-p190RhoGAPR1283A clones (p190-RA), and three HA-p190RhoGAP clones (wt-p190) of similar expression levels were pooled. Cells were maintained in DMEM supplemented with 10% fetal bovine serum (Life Technologies), antibiotics, and 10 μg/ml puromycin.
For all experiments, cells were replated in the absence of puromycin the day before experiments, trypsinized, washed twice in DMEM, and then suspended before plating for 1 h in DMEM and 0.5% fatty acid-free bovine serum albumin (Sigma). Plating surfaces were coated with 20 μg/ml fibronectin (Life Technologies) overnight at 4°C, followed by blocking for 1 h with 0.5% fatty acid-free bovine serum albumin. RhoA inhibition was achieved by introduction of C3 transferase as previously described (Renshaw et al., 1996). Briefly, 12 μg (spreading assays) or 34 μg (RhoA assays) of C3 transferase or glutathione S-transferase (GST; as a negative control) were mixed with 25 μl of LipofectAMINE and 200 μl of DMEM for 15 min and then added to a 10-cm dish of subconfluent cells in 5 ml of DMEM for 90 min. RhoA was activated by transfecting cells with 0.5 μg of PAX142-LscD4-HA (Whitehead et al., 1996), an expression vector encoding a constitutively active mutant of the RhoA exchange factor, Lsc. As a negative control for transfection, cells were transfected with pEGFP-C1 (Clontech).
RhoA Activity Assay
RhoA activity was measured with the use of a technique similar to the method described by Ren et al. (1999). Briefly, cells were lysed in 300 μl of 50 mM Tris, pH 7.4, 10 mM MgCl2, 500 mM NaCl, 1% Triton X-100, 0.1% SDS, 0.5% deoxycholate, 10 μg/ml each of aprotinin and leupeptin, 1 mM phenylmethylsulfonyl fluoride, and 200 μM vanadate. Lysates (500–750 μg) were cleared at 16,000 × g for 5 min, and the supernatant was rotated for 30 min with 30 μg of GST-RBD (GST fusion protein containing the RhoA-binding domain [amino acids 7–89] of Rhotekin) bound to glutathione-Sepharose beads (Amersham Pharmacia Biotech, Uppsala, Sweden). Samples were washed three times in 50 mM Tris, pH 7.4, 10 mM MgCl2, 150 mM NaCl, 1% Triton X-100, and inhibitors and then immunoblotted with RhoA monoclonal antibodies (BD Transduction Laboratories). Whole cell lysates were also immunoblotted for RhoA as loading controls.
Immunofluorescence
Cells on coverslips were fixed for 15 min in 3.7% formaldehyde and then permeabilized for 5 min in 0.5% Triton X-100. Texas Red-phalloidin and Hoechst 33342 from Molecular Probes (Eugene, OR) were used to label F-actin and nuclei, respectively. Monoclonal antibodies against the HA (Covance) or GM130 (BD Transduction Laboratories) were used, followed by incubation with fluorescein isothiocyanate-donkey anti-mouse antibody (Jackson ImmunoResearch Laboratories, West Grove, PA). Images were obtained on an Axiophot microscope (Zeiss, Thornwood, NY) with the use of a MicroMAX 5-MHz cooled charge-coupled device camera (Princeton Instrument, Trenton, NJ) and Metamorph Image software (Universal Imaging, West Chester, PA).
Spreading Assay
Suspended cells were plated on fibronectin-coated coverslips for 10, 20, 30, 45, 60, and 180 min. Coverslips were fixed and stained with Coomassie blue (2% Brilliant Blue [Sigma], 45% methanol, and 10% acetic acid) for 10 min and then washed with water and mounted. The relative areas of individual cells from Metamorph images were quantified with the use of National Institutes of Health Image software. Data from these and all other experiments were considered significantly different if the p values, as determined by two-tailed t-tests, were <0.05.
Migration Assay
The top and bottom surfaces of 8-μm pore, 6.5-mm polycarbonate Transwell filters (Corning Costar, Corning, NY) were coated with fibronectin. Cells (2.0 × 104) were seeded on the upper surface of the filters and migration was allowed to proceed for 3 h. Cells were fixed, stained with Coomassie blue, and the number of cells per 25× field on the lower surface of the filters was counted. For some experiments Rho kinase was inhibited with 3 μM Y-27632 (Uehata et al., 1997b; Welfide, Osaka, Japan).
Monolayer Wound Healing and Polarity Assay
Cells (4.0 × 105) in serum-free medium were seeded on fibronectin-coated coverslips in 24-well plates and allowed to adhere for 1 h. The monolayer was then wounded with a rubber policeman. Cells were washed once, fresh serum-free media was added, and the cells were allowed to invade the wound for 2 h (polarity assay) or 3 h (morphology assay) before fixation. Nuclei, F-actin, and the Golgi were labeled as described above with Hoechst 33342, phalloidin, and GM130 antibodies, respectively. Cells bordering the wound were considered polarized if the Golgi was orientated toward the wound-side of the nucleus (Kupfer et al., 1982).
RESULTS
Adhesion-dependent Regulation of RhoA activity by p190RhoGAP
To investigate the biological function of p190RhoGAP in integrin-mediated signaling, we generated pooled populations of Rat1 fibroblasts stably expressing dominant negative, GAP-deficient HA-p190RhoGAPR1283A (p190-RA cells), empty vector (mock cells), or overexpressing wild-type HA-p190RhoGAP (wt-p190 cells). Expression of GAP-deficient p190RhoGAP has previously been shown to have a dominant negative effect on the endogenous protein (Arthur et al., 2000). By generating stable lines, we selected for cells expressing levels of p190RhoGAP below what is required to induce the previously described severe phenotype characterized by cell retraction and beaded extensions (Tatsis et al., 1998). Expression levels of the exogenous p190RhoGAP variants were approximately four to five times higher than endogenous p190RhoGAP and had no effect on RhoA expression (Figure (Figure1A). 1A).
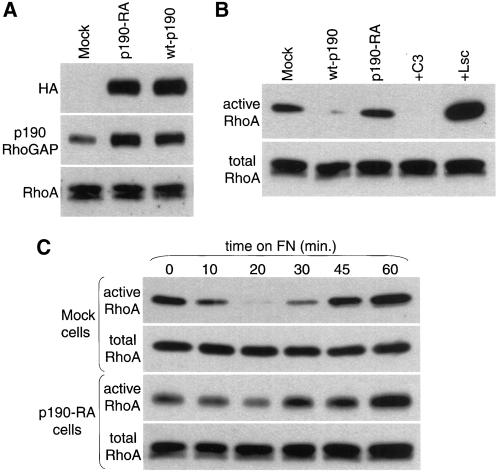
p190RhoGAP inhibits RhoA in an adhesion-dependent manner. (A) Expression levels of pooled populations of Rat1 cells stably transfected with dominant negative and wild-type p190RhoGAP. Whole cell lysates from Rat1 cell lines expressing dominant negative HA-p190RhoGAPR1283A (p190-RA), wild-type HA-p190RhoGAP (wt-p190), or empty vector (mock) were subjected to immunoblotting with monoclonal antibodies against HA or p190RhoGAP, to assess the expression level of the exogenous p190RhoGAP variants, or RhoA as a loading control. (B) Similar levels of RhoA activity in control cells and cells expressing dominant negative p190RhoGAP in the absence of adhesion. mock, wt-p190, and p190-RA cells, as well as mock cells transduced with C3 transferase or transfected with constitutively active RhoA GEF (Lsc), as negative and positive controls, were placed in suspension for 1 h and then RhoA activity was measured. Note: longer film exposures revealed detectable levels of active RhoA in wt-p190 cells but not in cells treated with C3 transferase. (C) p190RhoGAP is required for RhoA inactivation in response to adhesion on fibronectin (FN). Mock or p190-RA cells were plated on fibronectin for 0, 10, 20, 30, 45, and 60 min. Cells were then lysed and subjected to RhoA activity assays.
To examine the effect of exogenous wild-type or dominant negative p190RhoGAP, we measured RhoA activity by precipitating active GTP-bound RhoA with GST-RBD (Ren et al., 1999). In suspension, mock and p190-RA cells had similar levels of RhoA activity, whereas RhoA activity levels were reduced in wt-p190 cells (Figure (Figure1B).1B). RhoA activity was also assessed in mock cells treated with the RhoA inhibitor C3 transferase or transfected with the exchange factor Lsc as negative and positive controls, respectively, for the assay. C3 transferase decreased RhoA activity to undetectable levels, whereas RhoA activity was elevated in cells expressing Lsc (Figure (Figure1B). 1B). Adhesion of mock cells to fibronectin stimulated a rapid and transient decrease in RhoA activity (Figure (Figure1C).1C). However, RhoA inactivation in response to fibronectin was antagonized in p190-RA cells. Cells expressing dominant negative p190RhoGAP continued to show a decrease in RhoA activity in response to fibronectin adhesion, but this inactivation was greatly attenuated. These data are consistent with our previous studies (Arthur et al., 2000) and suggest that RhoA inhibition by fibronectin-stimulated signaling is mediated by p190RhoGAP. Furthermore, these data suggest that the activity of endogenous p190RhoGAP requires adhesion, given that dominant negative mutants of this GAP modify RhoA activity in adherent cells but not in suspended cells.
Regulation of the Actin Cytoskeleton by p190RhoGAP during Cell Spreading
Because RhoA regulates the organization of filamentous actin, we examined the actin cytoskeleton as cells initially adhered to fibronectin. We found that mock and wt-p190 cells spreading on fibronectin were nearly devoid of stress fibers at early time points but had extensive F-actin–rich ruffles and lamellipodia around the periphery of the cell (Figure (Figure2A).2A). In contrast, p190-RA fibroblasts had few ruffles or other protrusions but exhibited robust stress fibers. This phenotype was exaggerated in Rat1 cells transiently transfected with a vector encoding dominant negative HA-p190RhoGAPR1283A (Figure (Figure2B)2B) and found to be sensitive to the RhoA-specific inhibitor C3 transferase (data not shown). The precocious development of stress fibers in cells expressing dominant negative p190RhoGAP at early time points is in accord with the elevated RhoA activity observed in p190-RA cells (Figure (Figure1C).1C). Stress fibers developed in mock cells after 60 min on fibronectin (data not shown), consistent with the elevation in RhoA activity in these cells at this time point (Figure (Figure1C).1C). These data suggest that RhoA inhibition by p190RhoGAP is necessary for the prevention of premature stress fiber formation as cells spread on fibronectin.
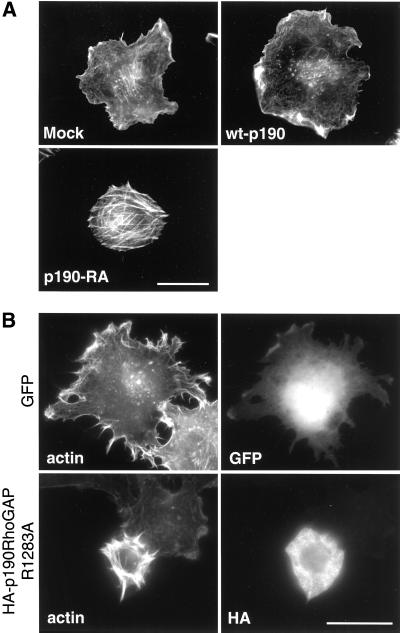
p190RhoGAP is necessary for the prevention of premature stress fiber formation in cells spreading on fibronectin. Mock, p190-RA, and wt-p190 cells (A) or Rat1 cells transiently transfected with pEGFP-C1 (GFP) or the dominant negative pKH3-HA-p190RhoGAPR1283A vector (B) were plated on fibronectin for 20 min, and then F-actin and the HA epitope (transient transfections only) were labeled. Note: the exposure time for the image of actin morphology in the cell transiently expressing HA-p190RhoGAPR1283A was shortened to compensate for intense phalloidin labeling. Bar, 20 μm.
Regulation of Cell Spreading by p190RhoGAP
Given that RhoA-mediated contractility inhibits membrane protrusion (Rottner et al., 1999; Cox et al., 2001) and that RhoA inactivation by fibronectin is antagonized in cells expressing dominant negative p190RhoGAP (Figure (Figure1C),1C), p190RhoGAP may regulate cell spreading. To test this hypothesis, we measured the relative area of individual cells during adhesion (Figure (Figure3A).3A). At early spreading time points (20 min), the area of p190-RA cells was significantly smaller than that of mock cells. Furthermore, the area of wt-p190 cells was significantly greater than the area of mock cells. After 3 h on fibronectin, we observed no significant difference in the areas of mock, p190-RA, and wt-p190 cells (data not shown). To determine whether the decreased spreading by p190-RA cells was a result of high RhoA activity (Figure (Figure1C),1C), the RhoA-specific inhibitor C3 transferase was introduced into cells. We found that RhoA inhibition by a low dose of C3 transferase partially rescued the decrease in spreading by p190-RA cells (Figure (Figure3B).3B). These data are consistent with the described role of RhoA inhibition in enhancing membrane protrusion (Rottner et al., 1999) and suggest that RhoA inactivation by p190RhoGAP contributes to efficient cell spreading on fibronectin.
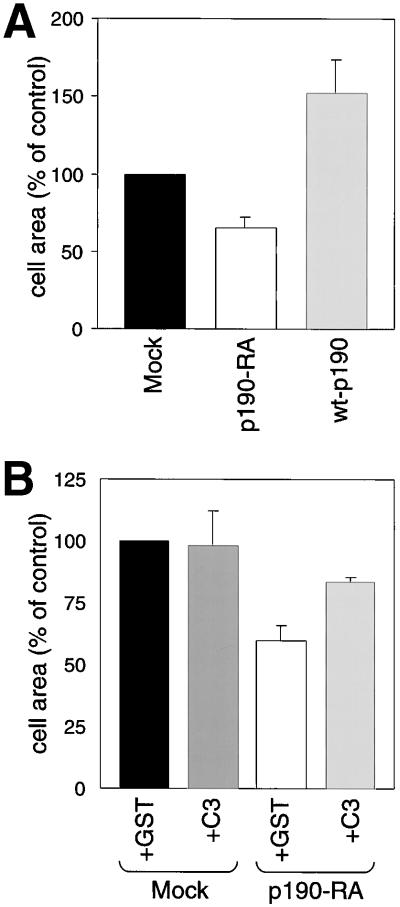
RhoA inhibition by p190RhoGAP is necessary for efficient cell spreading on fibronectin. Suspended mock, p190-RA, and wt-p190 cells (A) or mock and p190-RA transduced with GST or C3 transferase (B) were plated on fibronectin for 20 min, fixed, and then stained with Coomassie blue. The relative areas of individual cells in digital images were measured. The mean area of the control (mock cells) was set at 100%. The relative areas ± SEM were calculated from the mean areas of four separate experiments.
Regulation of Cell Migration by p190RhoGAP
The signaling and cytoskeletal dynamics that occur in spreading cells are generally thought to mimic events at the leading edge of migrating cells. Previous studies have demonstrated that p190RhoGAP localizes to membrane protrusions found at both the periphery of spreading cells and the leading edge of migrating cells (Nakahara et al., 1997; Brouns et al., 2000). We found similar subcellular localization patterns by endogenous and stably expressed HA-tagged wild-type or GAP-deficient p190RhoGAP in Rat1 cells (data not shown). These findings suggest that p190RhoGAP may be active at the leading edge of motile cells where they are actively engaging the extracellular matrix. Consistent with this hypothesis, we found that the RhoA activity in subconfluent p190-RA cells plated on fibronectin for 3 h was elevated relative to mock cells (Figure (Figure4).4). These data suggest that p190RhoGAP signaling participates in the final decrease in RhoA activity observed when cells are plated on fibronectin for 2–3 h or longer (Ren et al., 1999). As expected, RhoA activity was low in wt-p190 cells. The activity of Cdc42 and Rac1 were also examined with the use of a previously described technique (Bagrodia et al., 1998); however, no differences were detected among the mock, wt-p190, and p190-RA cell lines (data not shown).
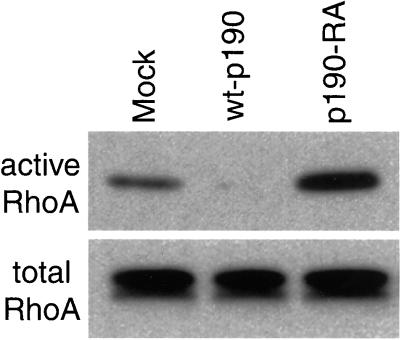
p190RhoGAP is necessary for maintenance of low basal RhoA activity in cells on fibronectin. Mock, wt-p190, and p190-RA cells were plated on fibronectin for 3 h and RhoA activity was measured.
To further explore the role of p190RhoGAP in motility, we examined the morphology of cells as they migrated into a monolayer wound (Figure (Figure5).5). We found that the p190-RA cells were less able to extend membrane protrusions into the wound area, relative to either mock or wt-p190 cells. In contrast, wt-p190 cells often extended elaborate growth cone-like protrusive structures (Figure (Figure5, 5, arrowhead). Furthermore, both mock and wt-p190 cells, but not p190-RA cells, elongated into the wound. These observations suggest that RhoA inhibition by p190RhoGAP participates in cell elongation and the formation or maintenance of membrane protrusions in migrating cells.
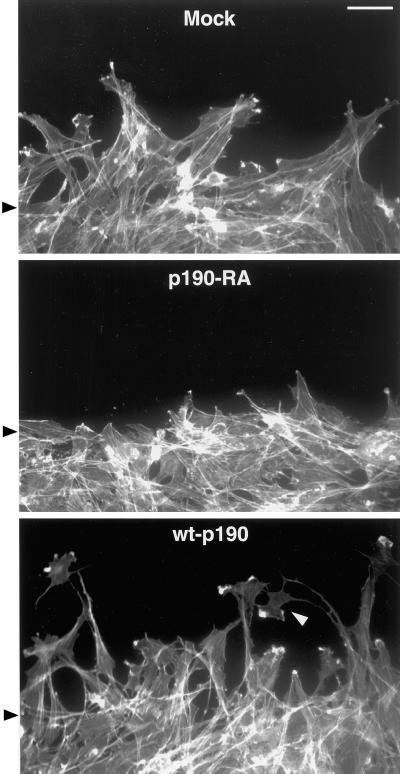
p190RhoGAP promotes membrane protrusion and cell elongation. Mock, p190-RA, and wt-p190 cells allowed to migrate into monolayer wounds for 3 h were labeled with Texas Red-phalloidin to reveal the morphology of the actin cytoskeleton. The position of the wound edges at the beginning of the assay are indicated by black arrowheads. Both mock and wt-p190 cells, but not p190-RA cells, elongated into the wound. In addition, wt-p190 cells often extended elaborate growth cone-like protrusions (white arrowhead). Bar, 20 μm.
To quantitate migration, assays were performed with the use of Transwell filters. The top and bottom surfaces of the filters were coated with fibronectin, cells were added to the upper chamber, and migration was allowed to proceed for 3 h (Figure (Figure6A).6A). We found that migration was enhanced in wt-p190 cells. Conversely, significantly fewer p190-RA cells were able to migrate through the filters compared with mock cells. To determine whether the decrease in migration by p190-RA cells was due to excessive RhoA signaling, the RhoA effector Rho kinase was inhibited with Y-27632 (Uehata et al., 1997a). Although Y-27632 did not significantly alter the motility of mock cells, treatment with this inhibitor partially rescued the migration defect exhibited by p190-RA cells (Figure (Figure6B).6B). These data, together with our finding that dominant negative p190RhoGAP elevated basal RhoA activity in adherent cells (Figure (Figure4),4), support our hypothesis that localized RhoA inhibition at the leading edge by p190RhoGAP enhances cell migration.
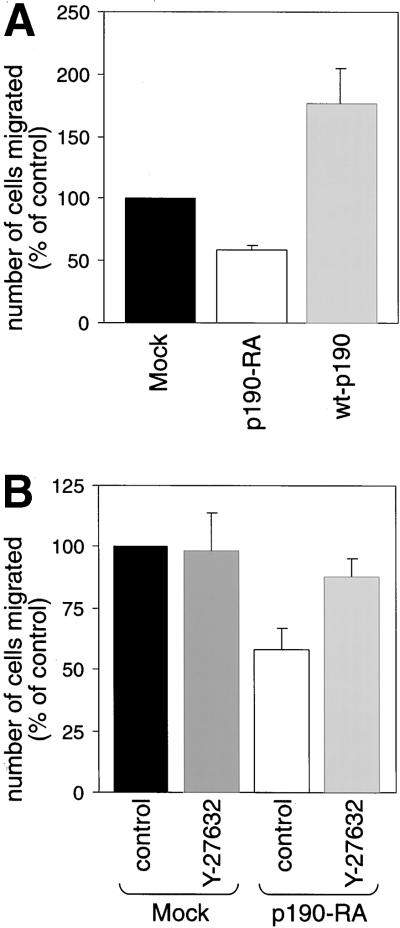
p190RhoGAP is necessary for efficient migration. (A) Transwell filters coated with fibronectin were used to measure migration by mock, p190-RA, and wt-p190 cells. Cells that had migrated to the lower surface of the filters were counted. (B) Similar assays were performed with mock and p190-RA cells treated with 3 μM Rho kinase-specific inhibitor Y-27632. The mean number of control cells (mock cells) that migrated through the filter was set at 100%. The relative number of cells per field ± SEM was calculated from the means of four separate experiments.
Requirement of p190RhoGAP for the Establishment of Polarity
Although our spreading and wound healing data implicate p190RhoGAP in the regulation of membrane protrusion, we investigated additional factors that might contribute to the migration defect exhibited by p190-RA cells. We initially observed that the nuclei in p190-RA cells were often positioned centrally within the cell, unlike mock and wt-p190 cells (not shown). Accordingly, we sought to determine whether p190RhoGAP is necessary for the establishment of cell polarity, i.e., the ability to orient in the direction of migration. To address this issue, the nuclei and Golgi were labeled in wounded monolayers (Figure (Figure7).7). Cells along the edge of monolayer wounds were considered polarized if the Golgi was localized to the wound-side of the nucleus, as previously described (Kupfer et al., 1982; Nobes and Hall, 1999). Approximately two-thirds of the Golgi in the mock and wt-p190 cells were facing the wound. However, we found that significantly fewer p190-RA cells were polarized relative to either the mock or wt-p190 cells. In fact, polarization of p190-RA cells on the edge of wounds was not significantly different from the random distribution observed in a confluent monolayer. These findings suggest that localized RhoA inhibition by p190RhoGAP is necessary for cells to establish polarity in the direction of migration, in addition to enhancing membrane protrusion.
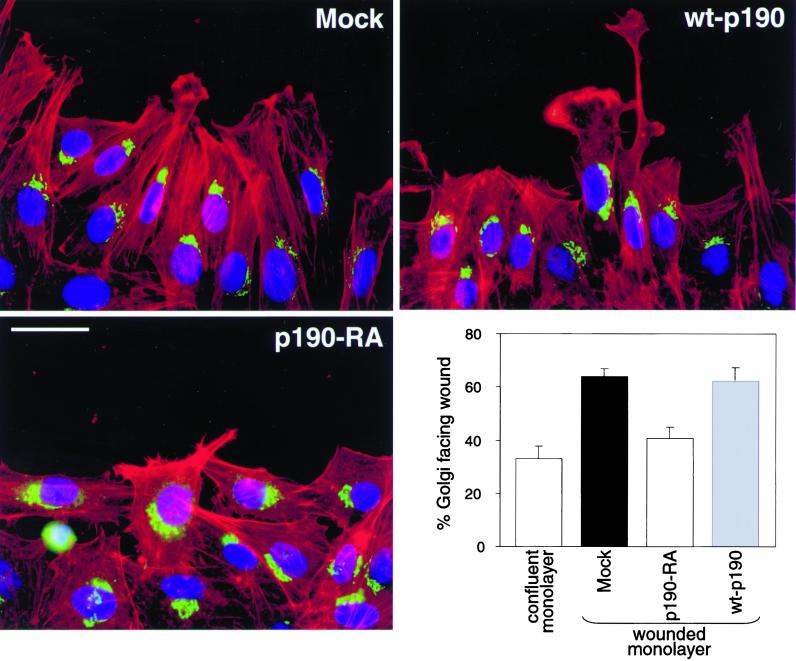
p190RhoGAP is necessary for the establishment of polarity. Mock, p190-RA, and wt-p190 cells were allowed to migrate into monolayer wounds for 2 h. The cells were triple-labeled with Hoechst 33342, GM130 (Golgi marker) antibodies, and Texas Red-phalloidin to reveal the nuclei (blue), Golgi (green), and F-actin (red), respectively. Bar, 20 μm. Cells along the edge of the wounds were scored as polarized if the Golgi was orientated to the wound-side of the nucleus, as previously described (Kupfer et al., 1982). The number of cells with Golgi facing an arbitrary point in a confluent monolayer was also counted as a measure of random orientation. The percentage of polarized cells ± SEM was calculated from the means of four separate experiments.
DISCUSSION
In this study, we examined the biological consequence of adhesion-mediated RhoA inactivation by p190RhoGAP. The impetus for this work came from several earlier studies that implicated p190RhoGAP signaling in response to adhesion. p190RhoGAP is tyrosine phosphorylated by the binding of antibodies to β1 integrins (Nakahara et al., 1997), translocates to a detergent-insoluble fraction upon adhesion to fibronectin (Sharma, 1998), and colocalizes with F-actin in lamellipodial protrusions (Nakahara et al., 1997; Brouns et al., 2000). Furthermore, we have previously described a signaling mechanism in which integrin ligation triggers RhoA inhibition via c-Src–dependent activation of p190RhoGAP (Arthur et al., 2000).
Here we show that adhesion-dependent RhoA inhibition by p190RhoGAP is necessary for efficient cell spreading and migration. Cells expressing dominant negative p190RhoGAP failed to decrease RhoA activity from high basal levels, resulting in premature stress fiber formation and impaired spreading. In contrast, overexpression of wild-type p190RhoGAP promoted cell spreading. The observation that p190RhoGAP localizes to membrane protrusions at the leading edge of migrating cells suggests that this GAP is active during migration. Consistent with this hypothesis, RhoA activity was elevated in subconfluent adherent cells expressing dominant negative p190RhoGAP. In migrating cells, expression of dominant negative p190RhoGAP inhibited both cell elongation and membrane protrusion, whereas these behaviors were enhanced in cells overexpressing wild-type p190RhoGAP. Accordingly, expression of dominant negative p190RhoGAP inhibited migration, whereas overexpression of p190RhoGAP enhanced motility. Interestingly, dominant negative p190RhoGAP also blocked the ability of cells to establish polarity in the direction of migration. We propose that RhoA inhibition by p190RhoGAP in response to adhesion to fibronectin contributes to both spreading and migration by enhancing cell protrusion, elongation, and polarity.
Cell migration is a complex process involving extension of membrane protrusions at the leading edge, the formation of adhesions, detachment, and retraction at the rear of the cell (Lauffenburger and Horwitz, 1996). Although it is well established that Cdc42 and Rac1 activity are crucial for protrusion (Van Aelst and D'Souza-Schorey, 1997), other studies have shown that RhoA inhibition is also involved. Introduction of the RhoA inhibitor C3 transferase into cells stimulates membrane ruffling (Rottner et al., 1999). Furthermore, expression of wild-type RhoA (Cox et al., 2001) or constitutively active RhoA (E.A. Cox and A. Huttenlocher, personal communication) inhibits the extension of membrane protrusions. These studies support our hypothesis that localized RhoA inactivation by p190RhoGAP is a permissive step that allows membrane extension. Without this inhibition, RhoA activity would functionally antagonize the formation of protrusions through excessive contractility. Consequently, RhoA inhibition by p190RhoGAP activity contributes to the protrusive activity required for both spreading and migration.
Recent studies have shown that the nonreceptor tyrosine kinase FAK (Ren
et al., 2000), in addition to c-Src and p190RhoGAP (Arthur
et al., 2000), is necessary for the initial
fibronectin-stimulated RhoA inactivation. Interestingly, the phenotype
we observed in spreading Rat1 cells expressing dominant negative
p190RhoGAP resembles that of spreading cells that lack FAK (Sieg
et al., 1999). It is presently unclear whether FAK
participates in RhoA inhibition by the
integrin→
c-Src
→
p190RhoGAP-signaling mechanism or if
it lies in a parallel pathway. Consistent with the idea that FAK
participates in c-Src–mediated p190RhoGAP activation, in FAK null
cells adhesion activates c-Src and yet fails to inhibit RhoA (Sieg
et al., 1998; Ren et al., 2000). In addition, a
scaffolding role has been assigned to the FAK-related kinase
Pyk2/CAKβ/CADTK/RAFTK because it binds both c-Src and p190RhoGAP and
mediates p190RhoGAP tyrosine phosphorylation by c-Src in response to
heregulin (Zrihan-Licht et al., 2000). Thus, it seems likely
that FAK mediates tyrosine phosphorylation of p190RhoGAP by c-Src in
response to adhesion, just as Pyk2 does downstream of heregulin
stimulation. Intriguingly, Masiero et al. (1999) recently
showed that FAK coimmunoprecipitates with p190RhoGAP in adherent cells.
An unexpected finding in this work was that inhibition of p190RhoGAP resulted in a failure of cells to develop the polarity that is associated with migration into a wounded monolayer. Polarity of migration is associated with selective protrusion of lamellipodia and filopodia at the leading edge, with persistence of a leading edge, and suppression of lateral protrusions (Nabi, 1999). It is also associated with orientation of the Golgi and centrosome to a region in front of the nucleus in the direction of migration (Kupfer et al., 1982; Euteneuer and Schliwa, 1992). Little is also known about the signaling pathways that are initiated by wounding a monolayer that give rise to polarized cytoskeletal organization and directed migration, although Cdc42 activity is necessary (Nobes and Hall, 1999; Erickson and Cerione, 2001). We first suspected that the effect of the dominant negative form of p190RhoGAP on polarity might be mediated directly or indirectly by diminished Cdc42 activity. However, we did not detect any change in activity of this Rho family protein. One possibility is that the elevated RhoA activity inhibits signaling downstream from Cdc42. Alternatively, it could be that RhoA-mediated contractility exerts its inhibitory effect on polarity by opposing the formation or maintenance of protrusive structures (Rottner et al., 1999; Cox et al., 2001). In this latter scheme of functional antagonism, an implication is that the protrusive structures contribute themselves to the development of polarity and are upstream of the polarized orientation of the Golgi. In future work it will be interesting to explore the hierarchy of these structures with respect to their role in governing polarity of cell migration.
Further evidence that p190RhoGAP is important for mediating cell polarity has arisen from studies of the p190RhoGAP-binding partner, p120RasGAP. The observation that recruitment of p120RasGAP to p190RhoGAP correlates with stress fiber disassembly in a Src-dependent manner has led to the conclusion that p120RasGAP is important for the activation of p190RhoGAP (Ellis et al., 1990; Settleman et al., 1992; Chang et al., 1995; van der Geer et al., 1997; Roof et al., 1998; Fincham et al., 1999). If p120RasGAP contributes to the activation of p190RhoGAP, then it would be predicted that neutralization of p120RasGAP or p190RhoGAP would produce similar phenotypes. Strikingly, p120RasGAP null cells have defects in cell elongation and polarity, which result in impaired migration (Kulkarni et al., 2000), similar to our observations of Rat1 cells expressing dominant negative p190RhoGAP. The importance of the p190RhoGAP-p120RasGAP association is further underscored by the demonstration that disruption of this complex with inhibitory peptides impairs migration and polarization (Kulkarni et al., 2000).
Together, our results and those of previous studies lead us to propose the following signaling mechanism by which adhesion to fibronectin controls cell behavior via regulation of RhoA (Figure (Figure8).8). The binding of the fibronectin RGD motif to integrins initially triggers RhoA inactivation by c-Src–dependent activation of p190RhoGAP (Arthur et al., 2000), a mechanism that likely includes FAK (Ren et al., 2000). RhoA inhibition by p190RhoGAP alleviates contractile forces that would otherwise collapse protrusions required for spreading. Next, RhoA is activated (Ren et al., 1999), resulting in the formation of stress fibers and strong adhesion through clustering of integrins to form focal adhesions (Chrzanowska-Wodnicka and Burridge, 1996). The delayed activation of RhoA by adhesion to fibronectin may be triggered by the activation of a GEF in response to binding of the RGD site to integrins (Barry et al., 1997) or by the heparin-binding domain of fibronectin triggering syndecan-4 signaling (Woods and Couchman, 1992; Bloom et al., 1999; Saoncella et al., 1999), or both. Subsequently, RhoA activity decreases to a lower basal level (Ren et al., 1999). The mechanism by which RhoA activity declines with time has not been determined, but our observation that dominant negative p190RhoGAP elevates RhoA activity at longer time points suggests that p190RhoGAP is involved in this decrease. Localized RhoA inhibition by p190RhoGAP at the leading edge allows cells to polarize and extend the membrane protrusions that are essential for cell migration. In ongoing studies, we are investigating the signaling components responsible for activation of RhoA by fibronectin binding to syndecans and integrins.
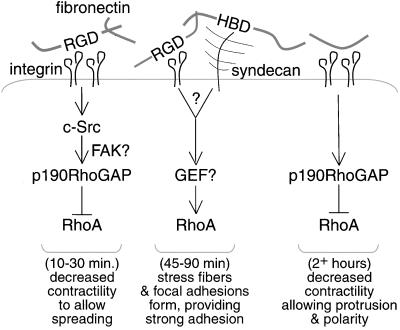
Proposed model for the regulation of RhoA by fibronectin. Adhesion to fibronectin regulates RhoA in a triphasic manner. RhoA is initially inhibited by integrin-triggered c-Src–dependent activation of p190RhoGAP. This inactivation of RhoA is necessary for efficient cell spreading by preventing Rho-mediated contractility from developing in nascent protrusions. In the second phase, integrin or syndecan signaling triggers stress fiber and focal adhesion formation via activation of RhoA. Finally, RhoA activity is reduced to a low basal activity. Low basal RhoA activity in adherent cells is maintained, at least in part, by localized p190RhoGAP activity and is necessary for the establishment of polarity and contributes to both membrane protrusion and cell elongation.
ACKNOWLEDGMENTS
The authors are grateful to Dr. Ian Macara for providing the wild-type and GAP-deficient p190RhoGAP expression vectors. We also wish to thank Christy Cloonan for excellent technical assistance, Dr. Channing Der for the Lsc construct, Geneva Arthur for preparation of the manuscript, and the members of the Burridge laboratory and Dr. Leslie Petch for thoughtful discussions. This work was supported by National Institutes of Health grant GM29860.
Abbreviations used:
GEF | guanine nucleotide exchange factor |
GAP | GTPase-activating protein |
GST | glutathione S-transferase |
HA | hemagglutinin epitope |
p190-RA | Rat1 fibroblasts stably expressing dominant negative HA-p190RhoGAP |
RBD | the RhoA-binding domain (amino acids 7–89) of Rhotekin |
RGD | single letter amino acid code for arginine, glycine, and aspartic acid |
wt-p190 | Rat1 fibroblasts stably expressing wild-type HA-p190RhoGAP |
REFERENCES
- Arthur WT, Petch LA, Burridge K. Integrin engagement suppresses RhoA activity via a c-Src-dependent mechanism. Curr Biol. 2000;10:719–722. [Abstract] [Google Scholar]
- Bagrodia S, Taylor SJ, Jordon KA, Van Aelst L, Cerione RA. A novel regulator of p21-activated kinases. J Biol Chem. 1998;273:23633–23636. [Abstract] [Google Scholar]
- Barry ST, Flinn HM, Humphries MJ, Critchley DR, Ridley AJ. Requirement for Rho in integrin signaling. Cell Adhes Commun. 1997;4:387–398. [Abstract] [Google Scholar]
- Bloom L, Ingham KC, Hynes RO. Fibronectin regulates assembly of actin filaments and focal contacts in cultured cells via the heparin-binding site in repeat III13. Mol Biol Cell. 1999;10:1521–1536. [Europe PMC free article] [Abstract] [Google Scholar]
- Brouns MR, Matheson SF, Hu K, Delalle I, Caviness VS, Silver J, Bronson RT, Settleman J. The adhesion signaling molecule p190 RhoGAP is required for morphogenetic processes in neural development. Development. 2000;127:4891–4903. [Abstract] [Google Scholar]
- Chang JH, Gill S, Settleman J, Parsons SJ. c-Src regulates the simultaneous rearrangement of actin cytoskeleton, p190RhoGAP, and p120RasGAP following epidermal growth factor stimulation. J Cell Biol. 1995;130:355–368. [Europe PMC free article] [Abstract] [Google Scholar]
- Chrzanowska-Wodnicka M, Burridge K. Rho-stimulated contractility drives the formation of stress fibers and focal adhesions. J Cell Biol. 1996;133:1403–1415. [Europe PMC free article] [Abstract] [Google Scholar]
- Cox EA, Sastry SK, Huttenlocher A. Integrin-mediated adhesion regulates cell polarity and membrane protrusion through the Rho family of GTPases. Mol Biol Cell. 2001;12:265–277. [Europe PMC free article] [Abstract] [Google Scholar]
- Ellis C, Moran M, McCormick F, Pawson T. Phosphorylation of GAP and GAP-associated proteins by transforming and mitogenic tyrosine kinases. Nature. 1990;343:377–381. [Abstract] [Google Scholar]
- Erickson JW, Cerione RA. Multiple roles for Cdc42 in cell regulation. Curr Opin Cell Biol. 2001;13:153–157. [Abstract] [Google Scholar]
- Euteneuer U, Schliwa M. Mechanism of centrosome positioning during the wound response in BSC-1 cells. J Cell Biol. 1992;116:1157–1166. [Europe PMC free article] [Abstract] [Google Scholar]
- Fincham VJ, Chudleigh A, Frame MC. Regulation of p190 Rho-GAP by v-Src is linked to cytoskeletal disruption during transformation. J Cell Sci. 1999;112:947–956. [Abstract] [Google Scholar]
- Hall A. Rho GTPases and the actin cytoskeleton. Science. 1998;279:509–514. [Abstract] [Google Scholar]
- Hotchin NA, Hall A. The assembly of integrin adhesion complexes requires both extracellular matrix and intracellular rho/rac GTPases. J Cell Biol. 1995;131:1857–1865. [Europe PMC free article] [Abstract] [Google Scholar]
- Kaibuchi K, Kuroda S, Amano M. Regulation of the cytoskeleton and cell adhesion by the Rho family GTPases in mammalian cells. Annu Rev Biochem. 1999;68:459–486. [Abstract] [Google Scholar]
- Kimura K, Ito M, Amano M, Chihara K, Fukata Y, Nakafuku M, Yamamori B, Feng J, Nakano T, Okawa K, Iwamatsu A, Kaibuchi K. Regulation of myosin phosphatase by Rho and Rho-associated kinase (Rho-kinase) Science. 1996;273:245–248. [Abstract] [Google Scholar]
- Kozma R, Ahmed S, Best A, Lim L. The Ras-related protein Cdc42Hs and bradykinin promote formation of peripheral actin microspikes and filopodia in Swiss 3T3 fibroblasts. Mol Cell Biol. 1995;15:1942–1952. [Europe PMC free article] [Abstract] [Google Scholar]
- Kulkarni SV, Gish G, van der Geer P, Henkemeyer M, Pawson T. Role of p120 Ras-GAP in directed cell movement. J Cell Biol. 2000;149:457–470. [Europe PMC free article] [Abstract] [Google Scholar]
- Kupfer A, Louvard D, Singer SJ. Polarization of the Golgi apparatus and the microtubule-organizing center in cultured fibroblasts at the edge of an experimental wound. Proc Natl Acad Sci USA. 1982;79:2603–2607. [Europe PMC free article] [Abstract] [Google Scholar]
- Lauffenburger DA, Horwitz AF. Cell migration: a physically integrated molecular process. Cell. 1996;84:359–369. [Abstract] [Google Scholar]
- Masiero L, Lapidos KA, Ambudkar I, Kohn EC. Regulation of the RhoA pathway in human endothelial cell spreading on type IV collagen: role of calcium influx. J Cell Sci. 1999;112:3205–3213. [Abstract] [Google Scholar]
- Nabi IR. The polarization of the motile cell. J Cell Sci. 1999;112:1803–1811. [Abstract] [Google Scholar]
- Nakahara H, Mueller SC, Nomizu M, Yamada Y, Yeh Y, Chen W. Activation of beta1 integrin signaling stimulates tyrosine phosphorylation of p190RhoGAP and membrane-protrusive activities at invadopodia. J Biol Chem. 1997;273:9–12. [Abstract] [Google Scholar]
- Nobes CD, Hall A. Rho, rac, and cdc42 GTPases regulate the assembly of multimolecular focal complexes associated with actin stress fibers, lamellipodia, and filopodia. Cell. 1995;81:53–62. [Abstract] [Google Scholar]
- Nobes CD, Hall A. Rho GTPases control polarity, protrusion, and adhesion during cell movement. J Cell Biol. 1999;144:1235–1244. [Europe PMC free article] [Abstract] [Google Scholar]
- Olofsson B. Rho guanine dissociation inhibitors: pivotal molecules in cellular signaling. Cell Signal. 1999;11:545–554. [Abstract] [Google Scholar]
- Ren X, Kiosses WB, Sieg DJ, Otey CA, Schlaepfer DD, Schwartz MA. Focal adhesion kinase suppresses Rho activity to promote focal adhesion turnover. J Cell Sci. 2000;113:3673–3678. [Abstract] [Google Scholar]
- Ren XD, Kiosses WB, Schwartz MA. Regulation of the small GTP-binding protein Rho by cell adhesion and the cytoskeleton. EMBO J. 1999;18:578–585. [Europe PMC free article] [Abstract] [Google Scholar]
- Renshaw MW, Toksoz D, Schwartz MA. Involvement of the small GTPase rho in integrin-mediated activation of mitogen-activated protein kinase. J Biol Chem. 1996;271:21691–21694. [Abstract] [Google Scholar]
- Ridley AJ, Comoglio PM, Hall A. Regulation of scatter factor/hepatocyte growth factor responses by Ras, Rac, and Rho in MDCK cells. Mol Cell Biol. 1995;15:1110–1122. [Europe PMC free article] [Abstract] [Google Scholar]
- Ridley AJ, Hall A. The small GTP-binding protein rho regulates the assembly of focal adhesions and actin stress fibers in response to growth factors. Cell. 1992;70:389–399. [Abstract] [Google Scholar]
- Ridley AJ, Paterson HF, Johnston CL, Diekmann D, Hall A. The small GTP-binding protein rac regulates growth factor-induced membrane ruffling. Cell. 1992;70:401–410. [Abstract] [Google Scholar]
- Roof RW, Haskell MD, Dukes BD, Sherman N, Kinter M, Parsons SJ. Phosphotyrosine (p-Tyr)-dependent and -independent mechanisms of p190 RhoGAP-p120 RasGAP interaction: Tyr 1105 of p190, a substrate for c-Src, is the sole p-Tyr mediator of complex formation. Mol Cell Biol. 1998;18:7052–7063. [Europe PMC free article] [Abstract] [Google Scholar]
- Rottner K, Hall A, Small JV. Interplay between Rac and Rho in the control of substrate contact dynamics. Curr Biol. 1999;9:640–648. [Abstract] [Google Scholar]
- Saoncella S, Echtermeyer F, Denhez F, Nowlen JK, Mosher DF, Robinson SD, Hynes RO, Goetinck PF. Syndecan-4 signals cooperatively with integrins in a Rho-dependent manner in the assembly of focal adhesions and actin stress fibers. Proc Natl Acad Sci USA. 1999;96:2805–2810. [Europe PMC free article] [Abstract] [Google Scholar]
- Sastry SK, Burridge K. Focal adhesions: a nexus for intracellular signaling and cytoskeletal dynamics. Exp Cell Res. 2000;261:25–36. [Abstract] [Google Scholar]
- Settleman J, Albright CF, Foster LC, Weinberg RA. Association between GTPase activators for Rho and Ras families. Nature. 1992;359:153–154. [Abstract] [Google Scholar]
- Sharma SV. Rapid recruitment of p120RasGAP and its associated protein, p190RhoGAP, to the cytoskeleton during integrin mediated cell-substrate interaction. Oncogene. 1998;17:271–281. [Abstract] [Google Scholar]
- Sieg DJ, Hauck CR, Schlaepfer DD. Required role of focal adhesion kinase (FAK) for integrin-stimulated cell migration. J Cell Sci. 1999;112:2677–2691. [Abstract] [Google Scholar]
- Sieg DJ, Ilic D, Jones KC, Damsky CH, Hunter T, Schlaepfer DD. Pyk2 and Src-family protein-tyrosine kinases compensate for the loss of FAK in fibronectin-stimulated signaling events but Pyk2 does not fully function to enhance FAK- cell migration. EMBO J. 1998;17:5933–5947. [Europe PMC free article] [Abstract] [Google Scholar]
- Takai Y, Sasaki T, Matozaki T. Small GTP-binding proteins. Physiol Rev. 2001;81:153–208. [Abstract] [Google Scholar]
- Takaishi K, Sasaki T, Kato M, Yamochi W, Kuroda S, Nakamura T, Takeichi M, Takai Y. Involvement of Rho p21 small GTP-binding protein and its regulator in the HGF-induced cell motility. Oncogene. 1994;9:273–279. [Abstract] [Google Scholar]
- Tatsis N, Lannigan DA, Macara IG. The function of the p190 Rho GTPase-activating protein is controlled by its N-terminal GTP binding domain. J Biol Chem. 1998;273:34631–34638. [Abstract] [Google Scholar]
- Uehata M, Ishizaki T, Satoh H, Ono T, Kawahara T, Morishita T, Tamakawa H, Yamagami K, Inui J, Maekawa M, Narumiya S. Calcium sensitization of smooth muscle mediated by a Rho-associated protein kinase in hypertension. Nature. 1997a;389:990–994. [Abstract] [Google Scholar]
- Uehata M, Ishizaki T, Satoh H, Ono T, Kawahara T, Morishita T, Tamakawa H, Yamagami K, Inui J, Maekawa M, Narumiya S. Calcium sensitization of smooth muscle mediated by a Rho-associated protein kinase in hypertension [see comments] Nature. 1997b;389:990–994. [Abstract] [Google Scholar]
- Van Aelst L, D'Souza-Schorey C. Rho GTPases and signaling networks. Genes Dev. 1997;11:2295–2322. [Abstract] [Google Scholar]
- van der Geer P, Henkemeyer M, Jacks T, Pawson T. Aberrant Ras regulation and reduced p190 tyrosine phosphorylation in cells lacking p120-Gap. Mol Cell Biol. 1997;17:1840–1847. [Europe PMC free article] [Abstract] [Google Scholar]
- Watanabe N, Kato T, Fujita A, Ishizaki T, Narumiya S. Cooperation between mDia1 and ROCK in Rho-induced actin reorganization. Nat Cell Biol. 1999;1:136–143. [Abstract] [Google Scholar]
- Whitehead IP, Khosravi-Far R, Kirk H, Trigo-Gonzalez G, Der CJ, Kay R. Expression cloning of lsc, a novel oncogene with structural similarities to the Dbl family of guanine nucleotide exchange factors. J Biol Chem. 1996;271:18643–18650. [Abstract] [Google Scholar]
- Woods A, Couchman JR. Protein kinase C involvement in focal adhesion formation. J Cell Sci. 1992;101:277–290. [Abstract] [Google Scholar]
- Zrihan-Licht S, Fu Y, Settleman J, Schinkmann K, Shaw L, Keydar I, Avraham S, Avraham H. RAFTK/Pyk2 tyrosine kinase mediates the association of p190 RhoGAP with RasGAP and is involved in breast cancer cell invasion. Oncogene. 2000;19:1318–1328. [Abstract] [Google Scholar]
Articles from Molecular Biology of the Cell are provided here courtesy of American Society for Cell Biology
Full text links
Read article at publisher's site: https://doi.org/10.1091/mbc.12.9.2711
Read article for free, from open access legal sources, via Unpaywall:
https://europepmc.org/articles/pmc59706?pdf=render
Citations & impact
Impact metrics
Citations of article over time
Alternative metrics

Discover the attention surrounding your research
https://www.altmetric.com/details/101970218
Article citations
Substrate stress relaxation regulates neural stem cell fate commitment.
Proc Natl Acad Sci U S A, 121(28):e2317711121, 05 Jul 2024
Cited by: 1 article | PMID: 38968101
Dietary xylo-oligosaccharides and arabinoxylans improved growth efficiency by reducing gut epithelial cell turnover in broiler chickens.
J Anim Sci Biotechnol, 15(1):35, 04 Mar 2024
Cited by: 0 articles | PMID: 38433214 | PMCID: PMC10910751
Integrin β3 directly inhibits the Gα13-p115RhoGEF interaction to regulate G protein signaling and platelet exocytosis.
Nat Commun, 14(1):4966, 16 Aug 2023
Cited by: 1 article | PMID: 37587112 | PMCID: PMC10432399
Diverse p120RasGAP interactions with doubly phosphorylated partners EphB4, p190RhoGAP, and Dok1.
J Biol Chem, 299(9):105098, 27 Jul 2023
Cited by: 2 articles | PMID: 37507023 | PMCID: PMC10470053
CSK-mediated signalling by integrins in cancer.
Front Cell Dev Biol, 11:1214787, 07 Jul 2023
Cited by: 4 articles | PMID: 37519303 | PMCID: PMC10382208
Review Free full text in Europe PMC
Go to all (297) article citations
Similar Articles
To arrive at the top five similar articles we use a word-weighted algorithm to compare words from the Title and Abstract of each citation.
Cadherin engagement inhibits RhoA via p190RhoGAP.
J Biol Chem, 278(16):13615-13618, 26 Feb 2003
Cited by: 109 articles | PMID: 12606561
Localized suppression of RhoA activity by Tyr31/118-phosphorylated paxillin in cell adhesion and migration.
J Cell Biol, 159(4):673-683, 01 Nov 2002
Cited by: 111 articles | PMID: 12446743 | PMCID: PMC2173105
Rapid recruitment of p120RasGAP and its associated protein, p190RhoGAP, to the cytoskeleton during integrin mediated cell-substrate interaction.
Oncogene, 17(3):271-281, 01 Jul 1998
Cited by: 28 articles | PMID: 9690509
Regulation of Rho family GTPases by cell-cell and cell-matrix adhesion.
Biol Res, 35(2):239-246, 01 Jan 2002
Cited by: 83 articles | PMID: 12415742
Review
Funding
Funders who supported this work.
NIGMS NIH HHS (2)
Grant ID: GM29860
Grant ID: R01 GM029860