Abstract
Free full text

LTBPs in Biology and Medicine; LTBP Diseases
Abstract
The latent transforming growth factor (TGF) β binding proteins (LTBP) are crucial mediators of TGFβ function, as they control growth factor secretion, matrix deposition, presentation and activation. Deficiencies in specific LTBP isoforms yield discrete phenotypes representing defects in bone, lung and cardiovascular development mediated by loss of TGFβ signaling. Additional phenotypes represent loss of unique TGFβ-independent features of LTBP effects on elastogenesis and microfibril assembly. Thus, the LTBPs act as sensors for the regulation of both growth factor activity and matrix function.
1. Introduction
The latent TGFβ binding proteins LTBPs act at the nexus of two vital matrix functions; they are crucial organizing elements for several important proteins and they are part of a mechanosensing hub that regulates signaling by TGFβ, a molecule that is a critical regulator of the expression of extracellular matrix (ECM) proteins [1]. This review will briefly describe the history and biochemistry of the LTBPs and then focus on the functions of each of these four proteins with respect to disease contributions. It is interesting that the functions of LTBPs in humans were largely derived by searching human populations for phenotypes initially described in mice with null mutations in individual LTBP genes. Special emphasis will be placed on the involvement of the LTBPs in human homeostasis and pathology, as well as unresolved questions within this field of research. Several earlier reviews provide more extensive background on biochemistry and function [2–5]. Additional descriptions of LTBP interactions with specific EMC proteins can be found within several of the other reviews in this issue of Matrix Biology.
2. History and Biochemistry
Latent TGFβ binding protein-1 (LTBP-1) was initially described as a component of a high molecular weight complex of TGFβ released from human platelets. Cloning of the LTBP-1 gene permitted the identification of three additional LTBP (LTBP-2, -3, and -4) genes [6–11].
The LTBPs are matrix proteins and have multiple calcium–binding EGF-like domains. In addition, the LTBPs contain a domain unique to the LTBPs and the fibrillins; the eight cysteine (8-Cys) or TGFβ-binding (TB) domain, which has four intra domain cystine residues [12–14]. LTBP-1 and -4 are produced as both long and short forms via the use of separate promoters that generate proteins with unique sequences at their amino termini but with identical sequences in the central and carboxy-terminal regions [13, 15]. As with other matrix proteins, multiple splice variants have been described for each of the LTBPs [16, 17].
The high molecular weight form of TGFβ associated with LTBP-1 consists of a tripartite complex composed of the mature TGFβ dimer, the TGFβ propeptide dimer (also referred to as LAP) and a single molecule of LTBP, forming the large latent complex (LLC) [7, 8]. This complex is formed intracellularly, and even after secretion the TGFβ propeptide dimer remains associated with the mature TGFβ by non-covalent bonds. This interaction with its propeptide prevents TGFβ from binding to its high affinity receptor. Thus, all TGFβ is secreted in a latent form bound to its propeptide. In order to signal, TGFβ must first dissociate from its propeptide in a process called activation, which is mediated by specific integrins, proteases, and several other molecules [18, 19].
Biochemical studies by Saharinen et al. showed binding of all three TGFβ isoforms (1, 2, and 3) to LTBP-1 and -3, binding of only TGFβ1 to LTBP-4, and no covalent interaction between any of the three TGFβ isoforms and LTBP-2 [20]. (TGFβ does not bind to any of the 8-Cys domains in fibrillin). 8-Cys domain 3 is the site of LAP binding to LTBPs [21, 22]. Structural studies of the 8-Cys domains of fibrillin and LTBP revealed that 8-Cys domains that bind TGFβ all contain a dipeptide insertion that is absent in the unreactive 8-Cys domains. The presence of this dipeptide forces cysteine residues 2 and 6 of 8-Cys domain 3 to the surface of the structure where they can pair with each other or bond with the free cysteines in LAP. The weaker binding of LTBP-4 to LAP may be explained by the replacement of three negatively charged amino acid residues in the 8-Cys domains of LTBP-1 and-3 with either uncharged or negatively charged amino acids surrounding the vital surface cysteines. Thus, docking of LAP with the 8-Cys 3 is a crucial first step in disulfide exchange. However, an important unanswered question is whether there is differential binding of to specific LTBPs. The initial experiments by Saharinen and Keski-Oja indicated a lack of TGFβ isoform binding specificity for LTBPs −1 and −3 [20]. However, as these data were derived from over-expression experiments, specificity amongst TGFβ isoform binding might exist at limiting reactant concentrations. Such selectivity might explain the specificity of certain LTBP-driven phenotypes. The reported binding of TGFβ isoforms and a variety of other molecules to the LTBPs is depicted graphically in figure 1. In this figure the binding region on the LTBP of the protein illustrated is given in green, i.e. the green dash indicates the sequence within the LTBP where binding occurs.
Beside TGFβ isoforms a number of other matrix constituents bind to the LTBPs (Fig. 1) These include fibronectin [23, 24] [25], fibrillin-1 [25–27], heparin sulfate and heparin [28], fibulin 4 and 5 [29, 30], and AdamTSL2 [26, 31]. Both myostatin and FGF-2 associate non-covalently with LTBP-3 and LTBP-2, respectively [32, 33]. However, the in vivo relevance of these interactions remains unknown.
LTBP Function
Early studies showed that LTBP-1 facilitates the secretion of latent TGFβ. This is due to the fact that, if not bound to an LTBP, the two free SH groups in the TGFβ propeptide form improper disulfide bonds resulting in intracellular protein degradation [34]. Once secreted, the LLC associates with the ECM via LTBP [27]. This matrix interaction potentially provides a reservoir of latent TGFβ that can be rapidly mobilized. Binding initially occurs to fibronectin and over time LTBP-1 molecules, as visualized by immunofluorescence, realign with fibrillin fibers [35, 36].
Binding of the LTBP-1 TGFβ complex to the ECM is necessary for latent TGFβ activation by integrins (Figure 1A, Left). Work by the Munger and the Sheppard labs clearly demonstrated that for TGFβ1 and 3, each of which has an integrin binding arginylglycylaspartic acid (RGD) sequence in the propeptide, the RGD is required for integrin-mediated activation of the latent complex [37, 38]. Mice in which TGFβ1 RGD sequence was replaced by RGE had a phenotype identical to TGFβ1 null animals. In addition mice with a TGFβ1 gene mutation in which the propeptide cysteine that binds to LTBP was mutated to serine (precluding LLC formation) had a TGFβ1 null-like phenotype [39]. This indicates that not only is integrin binding to the propeptide necessary for activation, but the latent TGFβ also must be bound to an LTBP, presumably so that the force applied by the integrin is countered by the tension from the LTBP being affixed to the ECM [18, 40, 41]. These data are in agreement with earlier work indicating that LTBP is cross-linked into the ECM by transglutaminase [42]. Analysis of the region of LTBP-1 required for ECM association and integrin-mediated activation revealed that a 55 amino acid stretch in an unstructured region is required and that this sequence binds to fibronectin [43]. LLC can also be activated by proteases, thrombospondin, and high pH. The requirements for activation of latent TGFβ isoforms 1 and 3 when bound to LTBP-3 have not been examined nor have the mechanisms for activation of TGFβ 2, which does not have an RGD sequence. Proteases can also activate latent TGFβ bound to an LTBP (Figure 2A, Right) [4].
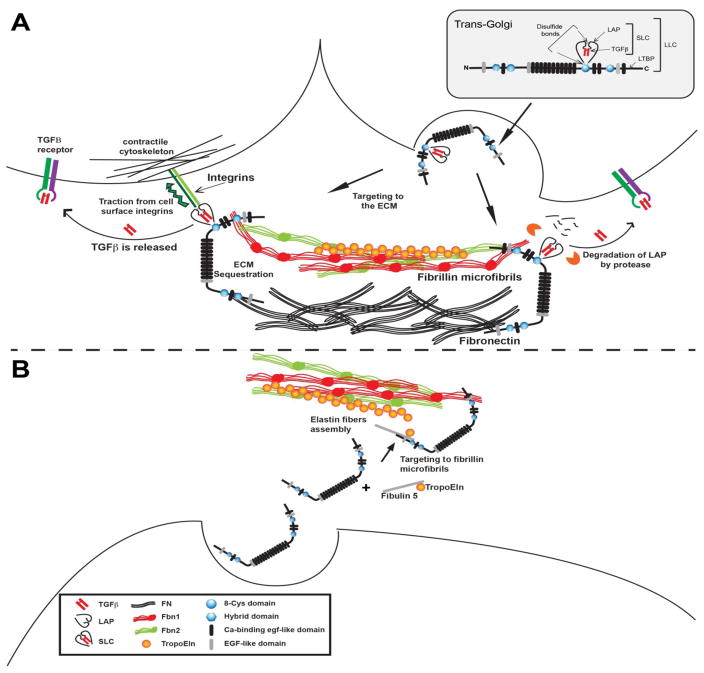
Illustration of synthesis, secretion, and activation of LTBP – TGF beta complexes.
A. Synthesis, secretion and activation by integrins (left) or proteases (right) of latent TGF beta bound to an LTBP.
B. Secretion of an LTBP interacting with matrix components in the assembly of elastin containing microfibrils.
The requirement for latent TGFβ to be bound to an LTBP and for that LTBP to be incorporated into the ECM has profound implications for the role of LTBP-TGFβ complexes in matrix biology. If the TGFβ LLC is not bound to the ECM, lower levels of active TGFβ will be generated. Thus, in conditions, such as Marfan syndrome (MFS), in which decreased amounts of LTBP are found incorporated in the ECM as a result of defective fibrillin fibers, the initial result should be a decrease, not an increase, in active TGFβ because activation of latent TGFβ is impaired. The increased levels of TGFβ reported in some situations are probably a compensatory response by the cells to the initial decrease in TGFβ. This implies that in the matrix there is a constitutive level of TGFβ signaling that maintains homeostasis. Mutations that affect the ability to maintain the basal level of active TGFβ will result in temporary decreases followed by attempts by the cells to readjust the TGFβ level to normal.
LTBP Physiology and Pathology
LTBP-1 Physiology
The use of LTBP-1 neutralizing antibodies or siRNA knock down has revealed multiple in vitro LTBP-1 functions. The first described was the ability of antibodies to LTBP-1 to block the activation of latent TGFβ in cocultures of endothelial cells and smooth muscle cells. LTBP-1 blocking antibody also inhibited the differentiation of embryonic stem (ES) cells to endothelial cells, an effect coincident with decreased levels of TGFβ in the treated cultures[44]. Nakajima et al. performed an elegant study in which antibody to LTBP-1 inhibited the endothelial-mesenchymal transformation (ETM) of mouse atrio-ventricular endocardial cells [45]. The normal process is TGFβ dependent and the addition of TGFβ in the presence of the antibody overcame the EMT blockage. These data are of particular relevance in light of the aortic phenotype of LTBP-1 knockout in mice.
Pathology
The initial mouse LTBP-1 null mutation targeted only the long form of the protein; thus synthesis of the short form, Ltbp-1S was undisturbed. Ltbp-1L−/− mice die at birth from persistent truncus arteriosis and interrupted aortic arch [46]. A similar phenotype presents when the TGF beta receptor is knocked out in cardiac neural crest cells, precursors of aortic smooth muscle cells, consistent with loss of LTBP-1L yielding decreased levels of TGF beta signaling [47]. More recent experiments revealed that the complete loss of LTBP-1 (L and S) produces a phenotype similar to loss of LTBP-1L [48]. The limited phenotypes observed in LTBP-1 null animals is surprising given the ubiquity of LTBP-1 expression and the phenotypic modifications observed with in vitro LTBP-1 suppression. The recent availability of a conditional null allele for LTBP-1 should clarify this question with the timed loss of the protein after birth and in specific tissues. Humans with loss of LTBP-1 have not been described, but this is likely an issue of appropriate screening, as the phenotypes of the other LTBP mouse mutants are replicated in human counterparts (See below).
LTBP-2 Physiology
As with the other LTBPs, LTBP-2 binds to the matrix in a fibrillin-dependent manner [49, 50]. In addition to binding to fibrillin, LTBP-2 binds to fibulin-5 and this interaction provides an important elastin fiber assembly regulatory function for LTBP-2 [29]. Although LTBP-2 was thought to be required for early mouse development [51], recent work indicates that LTBP-2 is not required for development but is necessary for the formation of microfibril bundles in the ciliary zonules [52]. In this sense LTBP-2 functions similarly to LTBP-4 (See below).
Pathology
In humans, loss of LTBP-2 results in primary congenital glaucoma with defects in the trabecular network [53, 54]. It is interesting that phenotypes in LTBP-2 null individuals and mice appears to be confined to the eye even though the protein is ubiquitous in the vascular system and multiple other tissues. One possible explanation is that LTBP-4 compensates for the loss in these other sites.
LTBP-3 Physiology
LTBP-3 has several unique features. LTBP-3 interacts with the ECM much more slowly than does LTBP-1 and LTBP-3 is secreted very poorly if not bound to latent TGF beta [55, 56]. The reason for this latter property is unknown, but unlike other LTBPs, the 2:6 cysteines in LTBP-3 that bind to LAP may be more reactive than those in the other isoforms. Without binding to LAP, these cysteines may form abnormal disulfide bonds that induce the unfolded protein response and the subsequent degradation of the misfolded LTBP-3. Therefore, both LAP and LTBP-3 may act as chaperones for each other by inducing proper disulfide bond pairing in their companion protein.
Pathology
LTBP-3 null mice and humans share similar phenotypes with short stature, spinal curvature, cranio-facial malformations, increased bone mass, and dental abnormalities, including reduced enamel production and alterations in both the mandible and maxilla [57–59]. The craniofacial dysmorphism is caused by premature ossification of the cranial synchondroses resulting in decreased anterior elongation of the face, as well as swelling of the cranial vault to accommodate the increasing size of the brain. The precocious ossification is mediated by decreased TGF beta resulting in insufficient PTHrP expression [58, 60]. The increased bone mass in LTBP-3 null mice is caused by a defect in bone resorption [59]. In some patients LTBP-3 mutations also present with acromicric and geleophysic dysplasia consistent with a role of LTBP-3 in long bone growth and lung septation [61]. LTBP-3 mice have a mild defect in the development of pulmonary alveoli during the septation phase, in addition to spleen and thymus involution [62, 63].
Further studies with LTBP-3 null mice have revealed a role of LTBP-3 in the aortic dilation and dissection observed in Marfan syndrome (MFS). Increased levels of TGFβ are found in the aortas of MFS patients and mice and antagonism of TGF beta reduces the degree of aortic dilation in MFS [64]. Consistent with a causative role of TGF beta in vessel dilation is the observation that deletion of LTBP-3 in mice with a rapidly progressing form of MFS yielded animals with normal-appearing aortas, as well as mRNA expression profiles [65]. The role of LTBP-3 in the aorta is probably more complex than simply regulating TGFβ levels, however, as a recent study from the Ramirez group indicated that inhibition of the cytokine with a pan specific antibody had little effect on vessel dilation in mice with a rapidly progressing from of MFS[66]. The apparent discrepancy between these two studies may indicate the complex nature of TGFβ, which is mediated by growth factor isoform, LTBP binding iosoform, cell of origin, and probably the availability of other signaling molecules. LTBP-3 also may have a role in mesenchymal stem cell (MSC) differentiation, as MSC differentiation to bone is inhibited when LTBP-3 is knocked down with small interfering (si) RNA [67]. This may reflect a role of TGF beta in the process, but it is also possible that LTBP-3 has a function independent of binding to LAP. Interestingly, the authors found that LTBP-1 expression is high, even as TGF beta levels decrease, indicating that the enhanced production of LTBP-1 does not supply TGF beta. These observations on a potential role of LTBPs and TGFβ in MSC differentiation is consistant with the observations of Smaldome et al. on the effects of fibrillin-1 loss and the modulation of stem cell niche associated changes in TGFβ [68].
Another provocative study implicated LTBP-3 in TGFβ action in a mouse model of lupus. The authors found that a CD4+ T cell subset expressed the early growth response gene Erg2, and that Erg2 controlled the production of TGFβ 3 that is essential for the lupus phenotype. Interestingly lack of Egr2 did not affect the transcription of the TGF beta 3 gene; rather lack of Erg2 prevented the expression of LTBP-3. Therefore, in this system, LTBP-3 levels govern extracellular TGF beta 3 activity [69]. LTBP-3 may also be important in cancer cell metastasis. The Hynes group evaluated the ECM protein content of several human tumors and found that LTBP-3 expression was increased in most of the tumors analyzed. LTBP-3 knock down in human breast cancer cells resulted in a loss of metastatic ability when injected into immunocompromised mice [70]. A recent report claims that knock down of LTBP-2 in thyroid carcinoma cells suppresses invasion [71]. Curiously the authors indicate that this effect is mediated by TGFβ even though LTBP-2 is not a carrier of TGFβ. Additional work is necessary to establish the generality of LTBP-3 support of metastasis as well as potential mechanisms, i. e. is metastasis regulated by LTBP-3 or by the TGFβ carried by LTBP-3. Although there is no direct evidence for a non-TGFβ-mediated function of LTBP-3, the fact that LTBP-2 and 4 have non-TGFβ activities indicates the potential for such a mechanism.
LTBP-4 Physiology
The biology of LTBP-4 is interesting, as both TGFβ-dependent and independent activities have been described. The initial LTBP-4 deficient mouse, which was a severe hypomorph of the short form of LTBP-4 (LTBP-4S), displayed defective elastogenesis in multiple organs including the lungs [72]. The elastin appears as globules adjacent to or on fibrillin fibrils and the ability of the elastin to envelope the microfibrils is defective. More recent studies using a complete LTBP-4 knock out replicated the findings from the LTBP-4S mouse but also indicated that LTBP-4L promotes the incorporation of fibulin 4 into the ECM [73] (Figure 2B). Experiments by Fujikawa et al. demonstrated that LTBP-2 and LTBP-4 have similar functions in the eye and that the ciliary zonules microfiber defect observed in LTBP-2 null mice can be prevented by ectopic expression of LTBP-4 [74].
Pathology
LTBP-4S null mice also develop cardiomyopathy and rectal-anal tumors, as well as increased cell proliferation in the affected tissues, which correlates with decreased TGFβ [72]. The tumor phenotype, however, appears to have been lost with successive breeding, as other labs been unable to reproduce this finding. Based upon the mouse phenotype, humans deficient in LTBP-4 were identified and displayed essentially the same features as the mouse LTBP-4 knockout [75]. The human mutant fibroblasts produce less TGF beta than normal control cells consistent with loss of TGF beta-related phenotypes in other LTBP knock out mice and people. The mouse lung developmental abnormality occurs at the saccular phase, rather than at the developmentally later septation phase as observed with LTBP-3 loss. These results are consistent with the earlier expression of LTBP-4 in the lung when compared to that of LTBP-3 [76]. The defect is dependent on elevated TGF beta 2 signaling as LTBP-4; TGFβ 2 double null embryos display normal septation as measured by Mean Linear Intercept analysis [77]. However, the septation defect is not normalized with the loss of TGFβ 1 or 3, nor does the lung abnormality occur when the LTBP-4 is mutated such that latent TGF beta cannot bind nor be activated implying that it is the loss of LTBP-4 not TGFβ that is responsible for the initial defect [78]. LTBP-4; TGFβ 2 double null mice, however, continue to display abnormal elastogenesis implying that the TGFβ 2 defect is either secondary or parallel to the elastin abnormality. The lack of association of elastogenesis and TGFβ 2 signaling in LTBP-4 deficient mice is consistent with earlier observations that LTBP-4 binds to TGFβ 1 but not to TGFβ 2 [20]. Further genetic studies with LTBP-4S mice have shown that the elastin defect arises because LTBP-4 is essential for the binding of fibulin-5-elastin complexes to fibrillin microfibrils. Knockdown of LTBP-4 using siRNA resulted in decreased deposition of fibulin 5 onto microfibrils in cultures of dermal fibroblasts. However, the addition of purified LTBP-4 stripped of TGFβ restored and enhanced fibulin 5 deposition, as well as elastic fiber deposition further supporting the TGFβ independent role of LTBP-4 in elastogenesis [30]. Thus, LTBP-4 can be thought of as an extracellular chaperone for elastin.
Although LTBP-4 null mice do not appear to display aortic dilation or dissection, a report has appeared linking an association of LTBP-4 haplotypes and increased dilation rates in Abdominal Aortic Aneurysms (AAA) [79]. The association of this increased growth of AAA and LTBP-4 haplotypes is not understood but may be related to abnormalities in the elastin organization.
An interesting set of findings involving LTBP-4 was reported regarding its potential involvement in muscular dystrophy [80–82]. The authors initially found that an LTBP-4 allele with a 12 amino acid insertion in the hinge region correlated with more severe muscular dystrophy. The LTBP-4 hinge lacking the insertion was more sensitive to proteases than the extended form of LTBP-4 and both animals and humans with the shorter form of LTBP-4 presented with less severe forms of muscular dystrophy. The severity of the phenotype also appears to depend upon the level of extracellular TGFβ and inhibition of TGFβ reduces the severity of muscle wasting. Thus, the authors have suggested that in animals and people the shorter form of LTBP-4 allows more proteolytic release of TGFβ from LTBP and this TGFβ promotes the pathological phenotype.
These later results are exciting, but contradict earlier results indicating that the lung phenotype in LTBP-4 null mice is not dependent upon TGFβ binding to the LTBP. Rather, the heightened TGFβ 2 observed in these mice is secondary and related in an unknown way to the elastogenesis defect. Indeed, the question of whether LAP can bind to LTBP-4 is controversial. As mentioned, the initial reports described LTBP-4 as binding exclusively and only to TGFβ 1 LAP. Our attempts to show binding failed (unpublished data). However, the long form of LTBP-4 is reported to bind LAP very effectively, although the site of binding was not described nor was the binding of LAP shown to produce a true LLC. The work of Chen et al. seemed to indicate that binding was very inefficient because of the charge change in the amino acids surrounding the LTBP-4 LAP binding 8-Cys domain [83]. Perhaps a way to resolve this conundrum would be to utilize the mutant LTBP-4 mice described by Dabovic et al. (2015) in which the cysteine pair that binds to LAP was mutated to serine thus precluding LTBP-4-LAP complex formation and to determine whether this affects the muscular phenotype.
Summary and Perspectives
Although the basic functions of the LTBPs have been elucidated and multiple phenotypes have been characterized (Table 1), much is not understood. As with other null mutations, it is likely that only the most obvious effects of loss of function have been described. The fact that LTBP-2 and 4 have TGFβ-independent activities suggests that LTBP-1 and 3 may also exhibit functions independent of the cytokine (Table 2). Interestingly, the idea that LTBP-1 and 3 bind all three TGFβ isoforms equivalently comes from in vitro over-expression experiments, which leaves open the possibility that there may be discrimination in TGFβ isoform binding to individual LTBPs at lower in vivo concentrations of both ligands and LTBPs. This would yield specific LTBPs regulating individual TGFβ isoform activities. The multiple splice variants of the LTBPs have not been explored in any meaningful way in vivo, but it is likely that these variants will modulate both LTBP and TGFβ activities [16]. It also is possible that LTBP degradation products, like a degradation product of fibrillin-1, are biologically active [84]. Finally, although there have been several in vitro studies on the biomechanical properties of LTBP-1, the significance to these results has not been translated to a physiological setting. It is likely that certain pathologies will reflect defects in the mechanosensing properties of LTBPs.
Table 1
LTBP-Dependent Phonotypes
Name | Mouse | Human | ||
---|---|---|---|---|
LTBP-1 | In Vivo | Persistent Truncus Arteriosis Interrupted aortic arch | Non reported | |
In Vitro | Anti-LTBP-1 antibody blocks ES cell differentiation to endothelial cells | |||
LTBP-2 | Lens Luxation | In Vivo | Primary Congenital glaucoma, Microspherophakia. | |
LTBP-3 | In Vivo | Cranial facial and dental malformations. Premature cranial synchondroses ossification | In Vivo | Acromeric and geleophysic dysplasia. Enamel abnormalities |
LTBP-4 | In Vivo | Defective elastogenesis in lung, cardiomyopathy, impaired lung development at the secular phase Modulation of muscular dystrophy phenotype. | In Vivo | Loss associated with enhances AAA growth. Decrease alveolar septation in the alveolar phase. Certain alleles enhance or diminish muscular dystrophy Phenotype. |
Table 2
Non-TGFβ Mediated Functions of LTBPs
LTBP-1 | Non-reported. |
LTBP-2 | Support of microfibril formation in the eye. |
LTBP-3 | Non-reported, possible contribution to metastasis. |
LTBP-4 | Support of elastogenesis in many tissues. Support of microfibril formation in the eye. |
Acknowledgments
Supported by a grant from the National Marfan Foundation to DBR.
Abbreviations used
AAA | Aortic abdominal aneurysm |
RGD | Arginylglycylaspartic Acid |
8-Cys | Eight cysteine |
EMT | Endothelial-mesenchymal transformation |
ECM | Extracellular Matrix |
ES | embryonic stem |
FGF | Fibroblast growth factor |
LLC | Large latent complex |
LTBP | Latent TGFβ binding protein |
MFS | Marfan syndrome |
MSC | Mesenchymal stem cell |
Si | Small interfering |
SH | Sulfhydryl |
TB | TGFβ binding |
LAP | TGFβ propeptide |
TGF | Transforming growth factor |
Footnotes
Publisher's Disclaimer: This is a PDF file of an unedited manuscript that has been accepted for publication. As a service to our customers we are providing this early version of the manuscript. The manuscript will undergo copyediting, typesetting, and review of the resulting proof before it is published in its final citable form. Please note that during the production process errors may be discovered which could affect the content, and all legal disclaimers that apply to the journal pertain.
References
Full text links
Read article at publisher's site: https://doi.org/10.1016/j.matbio.2017.11.014
Read article for free, from open access legal sources, via Unpaywall:
https://europepmc.org/articles/pmc5988920?pdf=render
Citations & impact
Impact metrics
Citations of article over time
Alternative metrics
Smart citations by scite.ai
Explore citation contexts and check if this article has been
supported or disputed.
https://scite.ai/reports/10.1016/j.matbio.2017.11.014
Article citations
Integrated bioinformatics analysis and experimental validation on malignant progression and immune cell infiltration of LTBP2 in gliomas.
BMC Cancer, 24(1):1252, 10 Oct 2024
Cited by: 0 articles | PMID: 39390437 | PMCID: PMC11466037
Mechanisms of assembly and remodelling of the extracellular matrix.
Nat Rev Mol Cell Biol, 25(11):865-885, 02 Sep 2024
Cited by: 2 articles | PMID: 39223427
Review
Poglut2/3 double knockout in mice results in neonatal lethality with reduced levels of fibrillin in lung tissues.
J Biol Chem, 300(7):107445, 04 Jun 2024
Cited by: 0 articles | PMID: 38844137 | PMCID: PMC11261140
Fibroblast and myofibroblast activation in normal tissue repair and fibrosis.
Nat Rev Mol Cell Biol, 25(8):617-638, 08 Apr 2024
Cited by: 15 articles | PMID: 38589640
Review
Mitochondrial reactive oxygen species impact human fibroblast responses to protracted γ-ray exposures.
Int J Radiat Biol, 100(6):890-902, 17 Apr 2024
Cited by: 0 articles | PMID: 38631047 | PMCID: PMC11471570
Go to all (48) article citations
Similar Articles
To arrive at the top five similar articles we use a word-weighted algorithm to compare words from the Title and Abstract of each citation.
Latent TGF-β-binding proteins.
Matrix Biol, 47:44-53, 08 May 2015
Cited by: 247 articles | PMID: 25960419 | PMCID: PMC4844006
Review Free full text in Europe PMC
Genetic analysis of the contribution of LTBP-3 to thoracic aneurysm in Marfan syndrome.
Proc Natl Acad Sci U S A, 112(45):14012-14017, 22 Oct 2015
Cited by: 35 articles | PMID: 26494287 | PMCID: PMC4653215
Assembly of fibrillin microfibrils governs extracellular deposition of latent TGF beta.
J Cell Sci, 123(pt 17):3006-3018, 10 Aug 2010
Cited by: 109 articles | PMID: 20699357 | PMCID: PMC2923573
Latent transforming growth factor-beta binding proteins (LTBPs)--structural extracellular matrix proteins for targeting TGF-beta action.
Cytokine Growth Factor Rev, 10(2):99-117, 01 Jun 1999
Cited by: 177 articles | PMID: 10743502
Review
Funding
Funders who supported this work.
NCI NIH HHS (1)
Grant ID: R01 CA034282
NHLBI NIH HHS (1)
Grant ID: P01 HL134605
National Marfan Foundation (1)
Grant ID: 15-A0-00-004779