Abstract
Free full text

Butyrophilin3A proteins and Vγ9Vδ2 T cell activation
Abstract
Despite playing critical roles in the immune response and having significant potential in immunotherapy, γδ T cells have garnered little of the limelight. One major reason for this paradox is that their antigen recognition mechanisms are largely unknown, limiting our understanding of their biology and our potential to modulate their activity. One of the best-studied γδ subsets is the human Vγ9Vδ2T cell population, which predominates in peripheral blood and can combat both microbial infections and cancers. Although it has been known for decades that Vγ9Vδ2T cells respond to the presence of small pyrophosphate-based metabolites, collectively named phosphoantigens (pAgs), derived from microbial sources or malignant cells, the molecular basis for this response has been unclear. A major breakthrough in this area came with the identification of the Butyrophilin 3A (BTN3A) proteins, members of the Butyrophilin/Butyrophilin- like protein family, as mediators between pAgs and Vγ9Vδ2T cells. In this article, we review the most recent studies regarding pAg activation of human Vγ9Vδ2T cells, mainly focusing on the roleofBTN3Aas the pAg sensing molecule, as well as its potential impact on downstream events of the activation process.
1. Introduction
As an essential component of the adaptive immune system, T cells use cell-surface bound T cell receptors (TCRs) to recognize antigens that signal the presence of non-self cells, pathogens or cellular stress. While most T cells bear a TCR composed of a heterodimer of an α and β chain, there exists a unique lineage of T cells that express heterodimeric TCRs consisting of a γ and δ chain, encoded by distinct gene loci from those used to generate the αβ TCR. Although much less abundant than αβ T cells in peripheral blood, γδ T cells predominate in tissues such as the skin, intestine and reproductive tract [1] and have been shown to play a critical, nonredundant role in various kinds of immune responses to infections, tissue damage, and cancers, indicating their potential for many clinical applications [2,3].
To understand the role of γδ T cells in an immune response, it is critical to elucidate the mechanism of TCR-dependent antigen recognition. In terms of overall structure, the γδ TCR is similar to its conventional αβ counterpart; in some cases, the TCR variable gene segments can even be used interchangeably by the TCRα and TCRδ chains [2,4]. Despite this homology, the antigen recognition mechanisms deployed by γδ T cells seem to deviate from the paradigm established in αβ T cells. Instead of recognizing antigenic peptides presented by classical major histocompatibility complex (MHC) molecules, some γδ T cells recognize lipid molecules presented by the MHC-like proteins CD1d and CD1c, while others can directly detect distinct antigens including whole proteins and non-peptidic small molecules, some without presentation via a canonical antigen-presenting MHC molecule [5–9]. Moreover, there is a poor overlap between antigens identified in mice and human systems, posing challenges for translatable mechanistic studies of γδ T cell antigen recognition [5].
Nevertheless, in recent years the field has made remarkable progress due to combined efforts from cellular and molecular immunology. In this review, we focus on one of the most investigated γδ subsets, human Vγ9Vδ2T cells. This subset represents the majority of γδ T cells in human peripheral blood with potent reactivity towards certain microbial infections and cancers [10,11]. Vγ9Vδ2T cells can specifically respond to a set of pyrophosphate metabolites derived from microbial sources or malignant cells, collectively called phosphoantigens (pAgs), in a TCR-dependent manner. In contrast to conventional αβ T cells, Vγ9Vδ2T cells do not appear to require MHC molecules for pAg-dependent activation. Furthermore, there is no evidence showing that the Vγ9Vδ2TCR directly interacts with pAgs [5,12–14]. As a result, the link that connects pAgs to Vγ9Vδ2T cell activation has been unclear.
A major breakthrough came when a key mediator, butyrophilin3A (BTN3A) from the butyrophilin (BTN) protein family, was identified as a necessary component in the pAg-dependent activation ofVγ9Vδ2T cells [15]. As their name indicates (“butter-loving”), the BTN molecules were originally identified in lactation and milk production [16]. Though related to the B7 family of co-stimulatory molecules, only in recent years have their immunomodulatory functions been defined in activating or inhibiting immune cells [16,17]. As our understanding of the BTN family expands, it is clear there exists substantial functional diversity. The role of BTN3A in pAg-dependent Vγ9Vδ2T cell activation is one such example.
In this review, we first introduce how Vγ9Vδ2T cells sense cellular stress in general and then focus on the early effort of elucidating the role of pAgs in the activation process. We next discuss the identification of BTN3A as the sensor for pAg detection and two models that have been put forth to explain how BTN3A functions in pAg-mediated activation. Lastly, we review the latest efforts towards connecting BTN3A-mediated pAg-sensing and Vγ9Vδ2TCR recognition. This review seeks to highlight the paradigm-shifting antigen-recognition mechanisms utilized by Vγ9Vδ2T cells.
2. The sensing of cellular stress by Vγ9Vδ2T cells; a novel antigen recognition mechanism is revealed
Human Vγ9Vδ2T cells play critical immunological roles in infectious diseases, tumors and homeostasis [2]. They can initiate responses against bacterial infections like tuberculosis and meningitis, as well as infection caused by protozoal parasites such as malaria [10]. They can also infiltrate several types of tumors, such as melanoma, breast, ovarian, colon, lung, pancreatic and prostate, and exhibit potent antitumor activity [18–22]. Upon stimulation, Vγ9Vδ2T cells can release cytotoxic (granzymes, granulysin, perforin, and death-inducing receptors), pro-inflammatory (TNF-α, IFN-γ, IL-17, and IL-8) or anti-inflammatory (TGF-β, IL-4, and IL-10) molecules [23,24]. In addition they can act as professional antigen-presenting cells via cross presentation to modulate other immune cells, such as Dendritic cells, T and B cells, to control infections [25,26]. The broad reactivity ofVγ9Vδ2T cells towards various kinds of cellular stress is of particular interest, not only because it has significant therapeutic potential, but it suggests a fundamentally different antigen detection mechanism than that of conventional T cell recognition whereby antigens are recognized in the context of MHC molecules.
2.1. Vγ9Vδ2T cells are activated by a diverse repertoire of molecules
Activation of Vγ9Vδ2T cells is dependent on expression of the Vγ9Vδ2TCR and this reactivity is transferable with the TCR [27,28]. Early studies excluded the requirement for peptide-MHC complexes for activation [12,13], providing the first evidence of MHC-independent yet TCR dependent T cell reactivity. More intriguing is the nature of the stimulatory molecules identified that activate Vγ9Vδ2T cells; these are discussed below and are shown in Table 1.
Table 1
Summary of reported Vγ9Vδ2T cell activators.
Activator name | Origin | Direct TCR contact | References |
---|---|---|---|
Phosphoantigens | Exogenous (from most eubacteria and apicomplexan protozoa); Endogenous | No | Morita et al. [10] |
6-O-methylglucose-containing lipopolysaccharides | Exogenous (Mycobacterium tuberculosis) | N.A. | Xia et al. [43] |
Staphylococcal enterotoxin A | Endogenous | N.A. | Rust et al. [42] |
GroEL homolog | Endogenous | N.A. | Fisch et al. [44] |
Hsp60 | Endogenous | Yes | Kaur et al. [45], Chen et al. [47] |
ULBP4 | Endogenous | Yes | Kong et al. [46] |
Human mutS homolog2 | Endogenous | Yes | Chen et al. [47] |
F1ATPase/Apolipoprotein A-I | Endogenous | Yes | Scotet et al. [48], Mookerjee-Basu et al. [50] |
Found in both infected and tumor cells, phosphoantigens (pAgs) are the best-characterized activator, capable of triggering a response from the most abundant subtype of Vγ9Vδ2T cells in the blood that has the signature Vγ9JγPVδ2TCR rearrangement [29–31]. pAgs are characterized as small molecules containing a pyrophosphate moiety with a variable organic group. pAgs can be classified as “exogenous” (from microbial origin) or “endogenous” (of mammalian origin). Exogenous pAgs were first found in extracts from Mycobacterium tuberculosis (Mtb) [12]. Most common was 4-hydroxy-3-methyl-but-2-enyl pyrophosphate (HMBPP), produced through the 2-C-methyl-D-erythritol 4-phosphate (MEP) pathway found in most eubacteria and apicomplexan protozoa [10,32,33]. Endogenous pAgs are represented by isopentenyl pyrophosphate (IPP), which is one of the first fully described Vγ9Vδ2-stimulating antigens [34]. In humans, it is one of the intermediates produced in the mevalonate (MVA) pathway and is similar to the exogenous pAg HMBPP in terms of chemical structure. Yet, it stimulates with 1000-fold less efficiency than its exogenous counterpart [32,33], preventing autoreactivity in normal cells where the concentration would be below threshold for stimulation. In tumor cells, not too surprisingly, it has been found that IPP is morbidly accumulated, likely due to hyperactivity of HMG-coA reductase, the rate-limiting enzyme in the MVA pathway [11,35]. The abnormally high intracellular concentration of IPP in these states compensates for its low potency enough to lead to the activation of Vγ9Vδ2T cells. Consistent with this idea, it has been shown that the stimulatory effect of aminobisphosphonates (NBPs) and alkylamines (both originally thought to be Vγ9Vδ2-stimulating “antigens”) comes from their ability to inhibit farnesyl pyrophosphate synthase, another enzyme in the MVA pathway that is directly downstream of IPP production [10,36]. Other notable pAg species include synthetic pAgs, such as BrHPP, most of which have chemically reactive substitutes added to the alkyl chain that confers greater antigenicity; and nucleotidic pAgs, which are generally co-produced with IPP or HMBPP and thought to be a source of cellular storage for these pAgs [33,37–41].
Other stimulants reported so far are either specifically found in microbial/protozoal-infected cells or tumor cells. In the case of infected cells, super antigen staphylococcal enterotoxin A and glycolipid components from Mtb have been found to specifically activate Vγ9Vδ2T cells [42,43]. In tumor cells, putative antigens include GroEL homolog, Hsp60, NKG2D ligand ULBP4 (in this particular case, it is also present in Epstein-Barr viral infected cells), human mutS homolog2 and mitochondrial F1-ATPase that expressed on the tumor cell surface [44–48]. Whether these activators stimulate independently or coordinate with pAgs is unclear, although it is very likely that both modes exist. For example, one study found that although the signature hydrophobic residue 97 in the CDR3δ loop of tumor-infiltrating Vγ9Vδ2TCRs is shown to be critical for pAg response, mutagenesis of this residue does not abolish the anti-tumor activity of Vγ9Vδ2T cells [49]. This suggests that a mechanism other than pAg-induced stimulation might mediate Vγ9Vδ2T cell recognition of tumor protein antigens. In favor of the latter case, one study demonstrated that an adenylated derivative of IPP could stably bind to F1 ATPase coated beads and promote TCR aggregation, cytokine secretion, and activation of the cytolytic process ofVγ9Vδ2T cells [50]. This implicated the involvement of F1-ATPase in pAg-induced T cell activation. Very recently, another study found that 6-O-methylglucose-containing lipopolysaccharides (mGLP), a mycobacterial glycolipid component, could stimulate a subset of pAg-responsive Vγ9Vδ2T cells that effectively inhibit intracellular mycobacterial growth [43]. Intriguingly, as demonstrated by this study, the pAg mediator protein BTN3A1, discussed further below, is also required for mGLP- mediated stimulation [43]. It is thus tempting to speculate that mGLP and pAgs might share a similar mechanism, even though their chemical properties are distinct.
Since pAgs can stimulate the vast majority ofVγ9Vδ2T cells and target them to both infected and tumor cells, they have received much more attention than the other stimulants discussed above. Even though the molecular mechanism behind their stimulation of Vγ9Vδ2T cells is not entirely clear, many attempts have already been made to utilize them in the development of Vγ9Vδ2T cells as an immunotherapeutic tool, especially for cancer treatment. Since NBP drugs such as zoledronic acid and pamidronate already exist on the market for treating osteoporosis and bone cancer, most of the existent clinical manipulations utilize them (as well as synthetic BrHPP) in combination with IL2 to activate and expand autologous Vγ9Vδ2T cells either in vivo or ex vivo [51]. Although these strategies are effective for Vγ9Vδ2T cell proliferation and generally considered to be safe, their clinical performance varies among different trials and has not been consistent [3,51]. Further more, Vγ9Vδ2T cells generated through these protocols can easily become anergic, posing limitation to the broader use of these cells in cancer therapy [3].
Due to these challenges, determining the molecular mechanisms behind Vγ9Vδ2T cell activation has become an essential goal for downstream medical applications. For the rest of this review, we solely focus on reviewing the literature on pAg-mediated activation ofVγ9Vδ2T cells.
2.2. How are Vγ9Vδ2T cells activated by pAgs? Early studies suggest that a pAg-mediator protein is required for Vγ9Vδ2T cell activation
As eluded to above, perhaps the only common feature of antigen recognition shared between Vγ9Vδ2T cells and conventional αβ T cells is the absolute requirement of expression of a TCR. For Vγ9Vδ2T cells, this was firmly established early on through antibody blocking and gene transfer approaches [27,28]. The crystal structure of the pAg-responsive Vγ9Vδ2TCR G115 (also the first structure of a γδ TCR) revealed important features that hinted a distinct recognition mode from αβ T cells [52]. The CDR-containing surface of G115 exhibits more protrusions and clefts compared to the flatter surfaces of conventional TCRs that engage peptide-MHC complexes (Fig. 1). Mainly contributed by the increased CDR length and alternate CDR2 position, this surface is instead reminiscent of the antigen-binding surface from an antibody [53]. Since pAgs contain a negatively charged pyrophosphate moiety, a putative binding site of pAgs within Vγ9Vδ2TCR was originally proposed to be a positively-charged, basic pocket formed by Arginine59 of CDR2γ, Lysine109 of CDR3γ and Arginine51 of CDR2δ (Fig. 1) [53]. Another important residue, position 97, is located in the CDR3δ loop and is always a hydrophobic residue in pAg-reactive Vγ9Vδ2TCRs. This position was originally proposed to interact with the hydrophobic alkyl chain from pAgs (Fig. 1) [53]. However, a more recent mutagenesis study found that besides the aforementioned residues, there is a much broader area within the TCR that is critical for pAg reactivity, essentially including all CDR loops (Fig. 1) [54]. This strongly indicated that Vγ9Vδ2TCRs engage an epitope much larger than a single pAg molecule. Indeed, all attempts to demonstrate direct interaction between pAgs and soluble, recombinant Vγ9Vδ2TCRs have thus far failed. In addition, cell-to-cell contact has been shown to be required for pAg-induced T cell activation [55]. Based on these findings, the field has speculated that analogous to peptide-MHC complex, pAgs are “presented” by a self-protein to Vγ9Vδ2TCRs.
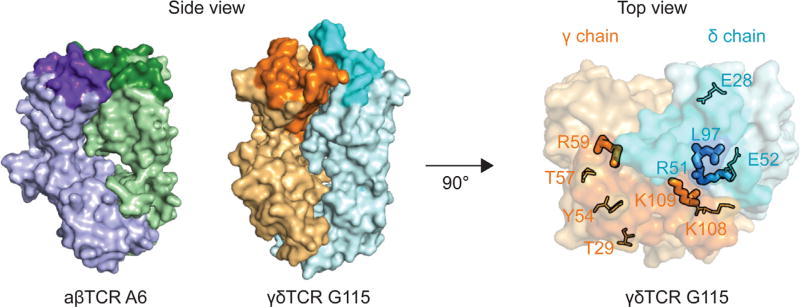
On the left, the crystal structure of a αβ TCR A6 (green and purple) is compared with the structure of a γδ TCR G115 (orange and cyan) in surface representation. The complementarity-determining regions (CDRs) are colored in darker shades in both TCRs. While the αβ TCR has a flatter CDR surface, the TCR surface has more clefts and protrusions that resembles an antigen-binding surface of an antibody. On the right, the γδ TCR G115 is rotated 90° to show the top view of the CDR surface. The residues in thick stick representation are the ones originally proposed to contact pAg. The residues in thin stick representation are the ones determined to be critical for pAg reactivity as well, extending the area in the CDR surface that is important for T cell activation.
The identity of this “presenter” protein has been of keen interest to the Vγ9Vδ2 field. Structurally speaking, the jagged surface of Vγ9Vδ2TCRs does not seem to complement a classical MHC presentation surface well [52]. Furthermore, according to the aforementioned mutagenesis study, the Vγ9Vδ2TCR footprint for a putative pAg-protein complex differs from conventional TCRs for peptide-MHC complexes as well as other unconventional TCRs for their cognate ligands [54]. Consistent with this, functional studies using an antibody blocking approach have ruled out almost all conventional candidates such as MHC class I and class II molecules, β2M and CD1 molecules [12,55]. Research groups then set out to identify a bona fide mediator, yet the nature of the pAg-induced activation process indicates swift and transient engagement of Vγ9Vδ2TCRs, presumably due to formation of very labile pAg-protein complexes with a low-affinity to Vγ9Vδ2TCR [56]. Therefore, this posed a challenge to using traditional biochemical methods to identify the pAg mediator. After almost two decades of directed research, a serendipitous discovery revealed members of the butyrophilin family, butryophilin 3A (BTN3A) as the mediators of pAg-induced activation; this group of proteins is discussed extensively in the following sections.
3. How does BTN3A1 mediate pAg induced activation of Vγ9Vδ2T cells? BTN3A1 functions as a pAg-sensing molecule
As a subfamily of butyrophilins, human BTN3A proteins are type I membrane receptors expressed on the surface of a broad variety of cell types including immune cells and some malignant cells such as ovarian cancer cells [16]. The expression level of BTN3A on some target cells such as endothelial cells and dendritic cells can be up-regulated by inflammatory cytokines like TNF-α, IFN-γ and IL6, as well as hypoxia-associated mediators VEGF and P1GF, which are also commonly up-regulated in most solid tumors [57,58]. Interestingly, it has also been shown that on activated lymphocytes such as T, NK, NKT and B cells, the level of BTN3A proteins is reduced [59]. Regulation on BTN3A expression hints their potential roles in inflammatory and tumor microenvironment.
There are three related proteins in the BTN3A family, each encoded by a separate gene, named BTN3A1, A2 and A3. All three proteins have two immunoglobulin (Ig)-like extracellular domains: a membrane proximal IgC and distal IgV domains, with over 95% sequence identity among them [60]. Indeed, antibodies specific for the extracellular domains of BTN3A (also known as CD277) cannot distinguish between the three family members [16]. However, the three BTN3A proteins are highly variable in their intracellular domains; while BTN3A1 and A3 both have a protein-protein interacting B30.2 domain with 87% sequence identity between them, A3 has an additional C-terminal tail and A2 completely lacks this domain [61]. Differences in the intracellular domain hint at a potential functional distinction among these family members.
Prior to the discovery of their role in pAg recognition, BTN3A proteins have been reported to play a part in regulating T cell proliferation and cytokine production in multiple in vitro studies using BTN3A specific antibodies [16,62]. Interestingly, their involvement in pAg recognition was a serendipitous discovery also made through antibody studies [15]. In 2012, Harly et al. found that the addition of 20.1, a weak agonist antibody specific for BTN3A, to non-susceptible target cells can trigger Vγ9Vδ2T cell activation similar to pAg treatment. In contrast, a different BTN3A specific antibody, 103.2, blocked pAg-induced T cell activation. Knock down experiments from the same study further confirmed the critical role of BTN3A in pAg mediated activation, as suppressed BTN3A expression in target cells led to a significant reduction in the pAg-induced Vγ9Vδ2T cell response. The study demonstrated a novel role for BTN3A proteins as the pAg sensor on target cells and served as a foundation for further studies dedicated to elucidate the molecular basis of BTN3A-mediated pAg activation of Vγ9Vδ2T cells.
3.1. Extracellular presentation or intracellular sensing? pAg sensing presents a paradigm-shifting model of T cell antigen recognition
The identification of BTN3A1 provided the first insight into the components required for pAg activation of Vγ9Vδ2T cells, however, the mechanism of pAg-induced activation remains unclear. The simplest model, consistent with pAg being a “presented” antigen, proposed that pAg associates with the extracellular domain of BTN3A, creating a composite epitope for recognition by the Vγ9Vδ2TCR. Biophysical and structural support for this model was soon provided [63]. However, other reports followed that demonstrated that pAgs instead interact directly with the intracellular B30.2 domain of BTN3A1, with no detectable association between pAg and the extracellular domains of BTN3A1 nor with the Vγ9Vδ2TCR, even in the presence of pAg or the agonist antibody 20.1 [64]. These contrasting studies are the basis of a heated debate over exactly how BTN3A proteins interact with pAgs [61,65–67].
The canonical view of antigen presentation and T cell activation dictates that antigens are presented extracellularly and directly recognized by the TCR, therefore, intracellular sensing of pAg by BTN3A is seemingly counterintuitive. Yet, generally speaking, many of the physiologically relevant pAgs are intracellular in target cells; accumulation of endogenous IPP is thought to be the trigger for Vγ9Vδ2 recognition of tumor cells and many (but not all) of the microbial pathogens that trigger a Vγ9Vδ2 response are intracellular pathogens [10,11,68,69]. Thus, an intracellular sensor would be an effective and sensitive means to monitor for the presence of exogenous pAgs or changes in endogenous concentration. The data to support this model is discussed below.
The first hint of pAg intracellular sensing came from the seminal study by Harly et al. [15], where the key role of BTN3A in Vγ9Vδ2T cell activation was originally described. The contribution of each BTN3A family member to pAg-induced T cell activation was carefully examined through knockdown and domain-shuffling approaches [15]. This revealed that the BTN3A1 protein, with a unique intracellular B30.2 domain, is the only indispensable family member of the three to mediate pAg-induced T cell activation; moreover, while the wild type A3 protein itself could not rescue the ability of BTN3A knockdown cells to stimulate T cells, a chimeric A3 with A1 intracellular domain could elicit even a stronger T cell response compared to wild type A1. These data strongly indicated a key role of the A1 intracellular B30.2 domain in the activation process.
Our group [64] then combined biophysical, structural and functional approaches to demonstrate a direct interaction between the A1 intracellular B30.2 domain and various kinds of pAgs including microbial source pAg HMBPP, endogenous pAg IPP, as well as synthetic pAgs such as BrHPP [64]. The affinities of these pAgs to the B30.2 domain were consistent with their potency, where the lower potency IPP dissociation constant (KD) was determined to be ~1mM whereas the KD of HMBPP was an order of magnitude stronger, ~1 uM. Through structural determination of the B30.2 domain, molecular docking and a series of mutational studies, a positively charged pocket composed of arginine 412, arginine 418, arginine 469 and histidine 351 was determined to be the binding pocket for pAgs (Fig. 2A). Other groups published findings consistent with intracellular B30.2 domain association of pAg, using similar or different approaches [70–72]. Furthermore, thorough mutagenesis of the extracellular domain of BTN3A found no effect on the pAg-induced T cell response while mutagenesis of the B30.2 intracellular domain found several sites that disrupted T cell activation [71]. Altogether, these data support the model whereby pAgs directly interact with the intracellular B30.2 domain of BTN3A1.
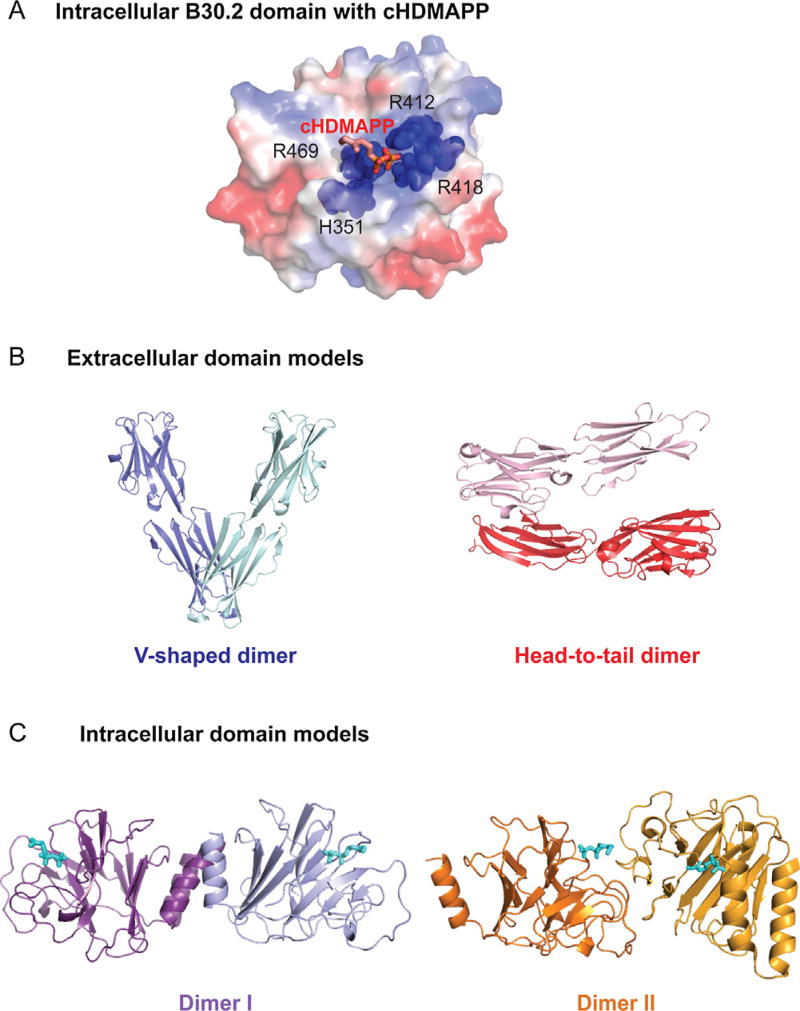
A) pAg binds to BTN3A1 intracellular B30.2 domain through a positively charged pocket. The crystal structural model of B30.2 domain is shown in surface representation with synthetic phosphoantigen cHDMAPP bound. Positively charged areas in B30.2 domain are colored in blue whereas negatively charged areas are colored in red. The residues that are key to pAg binding are shown in sphere representation with dark blue color. B) Cartoon representation of two putative dimer configurations of BTN3A1 extracellular domains. The “V-shaped” dimer is shown in two different shades of blue whereas the “Head-to-tail”dimer is shown in two different shades of red. C) Cartoon representation of two putative dimer configurations of BTN3A1 intracellular domains. The Dimer I is shown in two different shades of purple, whereas the Dimer II is shown in two different shades of orange. The position of pAg binding pocket is signified by a stick model of pAg in both dimer models.
While there is strong biochemical support for intracellular sensing of pAgs through the B30.2 domain of BTN3A1, there are some factors that have yet to be reconciled. Some pAgs do come from extracellular sources; extracellular pathogens such as E.coli as well as some immune cells such as neutrophils can secrete pAgs [73]. In addition, administration of pAgs extracellularly in in vitro cellular assays is routine. For the intracellular sensing to occur, there must be a mechanism for pAgs to cross the plasma membrane and enter the cell. Recently a study reported that ATP-binding cassette transporter A1 (ABCA1) could mediate IPP extracellular release from dendritic cells in cooperation with apolipoprotein A1 and BTN3A1 [74]. However, no intake transport mechanisms for pAgs have been identified, so this topic remains unresolved.
3.2. Consequences of pAg binding: possible transmission of an “inside-out” signal through pAg-induced conformational change of the BTN3A1 molecular sensor
Strong evidence supports the detection of pAgs through binding to the intracellular B30.2 domain on target cells. This detection appears to be the first in a series of molecular events that ultimately results in activation of Vγ9Vδ2T cells. The extracellular domains of BTN3A appear to be involved in activation as administration of the agonist antibody 20.1, which binds to the extracellular IgV domain of BTN3A, can induce pAg-independent T cell activation [15]. Since both intracellular and extracellular domains of BTN3A1 are indispensable, it is then natural to envision an “inside-out”, BTN3A1-mediated, pAg signaling mechanism that communicates an activating signal to the Vγ9Vδ2T cell via its TCR. Investigation into the proximal events that occur after pAg binding to the B30.2 domain have provided the first clues into how signals could be translated to the extracellular environment. Conformational changes to the B30.2 domain upon pAg binding were proposedearly on during the initial characterization of this interaction; attempts at crystallization of the pAg/B30.2 complex were complicated by the rapid disintegration of B30.2 crystals when pAg was “soaked in” [64], presumably due to conformational changes that occurred after pAg binding that disrupted the protein contacts in the crystal packing. More conclusive evidence came later through multiple NMR studies from our group and others probing the interaction between pAgs and BTN3A1 intracellular domain [72,75,76]. Hsiao et al. first reported chemical shift perturbations (CSPs) observed in BTN3A1 intracellular domain upon addition of pAgs, which are indicative of chemical environment change of residues involved [72]. Our group and Salim et al. then both further characterized these CSPs and found that these changes locate not only near the pAg-binding pocket, but also in places away from the pocket, demonstrating a global conformational change occurred within the B30.2 domain upon pAg binding [75,76]. As shown in the study by Salim et al., the conformational change induced by pAgs is specific, since other negatively charged, non-antigenic small molecules like citrate and malonate fail to induce a similar change within the B30.2 domain [76].
In addition to the chemical shift perturbations in the B30.2 domain, the Wiemer group from the University of Connecticut found that residues in the juxtamembrane (JTM) region, which locates at the N-terminus of the B30.2 domain and are distinct from the pAg binding pocket, also experienced significant change of chemical environment [72,77]. A recent study by Peigne et al. demonstrated that changes in this particular region of the BTN3A1 induce a markedly enhanced or reduced T cell reactivity, strongly suggesting a functional role played by the JTM region [78]. Since the JTM region connects the intracellular B30.2 domain to the transmembrane domain of the BTN3A1 molecule, it could be key for transmitting the intracellular signal extracellularly.
Crystallographic studies of BTN3A1 also provided potential models to explore in regards to alternative conformations that may be relevant to activating versus resting states on the cell surface. The BTN3A1 extracellular domains exist as dimers in solution [79], providing strong evidence that these proteins are functional dimers on the cell surface. In the crystal lattice of the full length BTN3A1 extracellular domain, two potential dimer conformations were identified [79]. One has a symmetric interface formed between the membrane-proximal IgC domains from each monomer producing a “V-shaped” homodimer (Fig. 2B). The other dimer conformation associates through an asymmetric interface between the membrane-distal IgV domain of one monomer in contact with the IgC domain of the other. The resulting homodimer would require each monomer to lay parallel to the cell surface in what has been called a “head-to-tail” dimer (Fig. 2B). Intriguingly, we recently published a structural study showing that there are two potential dimer conformations in the crystal lattice of the full length BTN3A1 intracellular domain as well [75]. One has a semisymmetric interface where part of the JTM region is sandwiched between the N-terminus of the B30.2 domain from each monomer (Fig. 2C, Dimer I). The other conformation has an even more interesting asymmetric interface that harbors the pAg-binding pocket (Fig. 2C, Dimer II). Moreover, the study showed that CSPs observed in the B30.2 domain upon pAg binding concentrated in the dimer interfaces mentioned above, suggesting that pAg-induced conformational change of the BTN3A1 intracellular domain might involve dimer conversion [75]. Since the extracellular domains of BTN3A1 also has two alternative conformations, the conformational change in the intracellular domain through sensing of pAg could translate through the membrane to change the extracellular domains from a resting to an active state.
Additional approaches have been employed to test these crystallographic-based models. We employed a Fluorescence Resonance Energy Transfer (FRET)-based solution measurement of the BTN3A1 extracellular domains to provide strong evidence for the “V-shaped” form [79] and through negative stain electron microscopy and cellular assays that the full-length BTN3A1 spontaneously adopts the ‘V-shaped’ form both in reconstituted lipid-like environment and in native cellular membrane [75]. Moreover, trapped in this ‘V-shaped’ form, a mutant BTN3A1 was defective in pAg-induced T cell activation, strongly supporting the functional importance of BTN3A1 conformations [75]. Results from another flow cytometric FRET study on BTN3A1 over-expressed cells revealed that the 20.1 antibody, which binds at the ‘head’ IgV domain of BTN3A1, was close to the cell membrane while at rest (high FRET), but became distant from the membrane (low FRET) when intracellular pAg level was elevated [80], consistent with a conformational switch of the BTN3A extracellular domains upon pAg-induced activation. Despite these results, exact molecular details on the pAg-induced conformational change of the BTN3A1 molecule still await clarification.
3.3. Does BTN3A1 work alone? Evidence for a network of proteins coordinating with BTN3A1 for activation
Using fluorescence recovery after photobleaching (FRAP), Harly et al. found that in the presence of pAgs or the 20.1 antibody, BTN3A1 molecules were immobilized on the cell surface [15], suggesting there are additional factors that are involved in transition from a resting state to activated. In the case of the 20.1 antibody, we proposed that a simple antibody-mediated cross-linking of BTN3A dimers on the surface of the cell could be the reason behind the reduced mobility of BTN3A [79], however, it is unclear as to what controls the mobility of BTN3A in the presence of pAg. Furthermore, from experiments performed in murine target cells where BTN3A1 molecules were introduced, it is clear that BTN3A1, while necessary for pAg-induced Vγ9Vδ2T cell stimulation, is not sufficient [64,81], strongly supporting the requirement of other molecular players absent in the murine lineage in this activation process.
Recent reports have identified two cytoskeletal mediators that are involved with BTN3A in pAg-induced T cell activation, providing new leads toward identifying additional players involved in this network. Periplakin, a cytoskeletal adaptor protein, and RhoB GTPase, a regulator for multiple actin-associated proteins have been shown to directly interact with BTN3A1 [70,80]. While the role of periplakin is unclear and characterized as “regulatory”, RhoB GTPase, was shown to play a positive role in pAg-triggered T cell activation, specifically in facilitating immobilization of BTN3A1 after pAg sensing [80]. Its role in pAg-dependent T cell activation strictly depends on its GTPase activity and cellular distribution, the latter of which is also regulated by the mevalonate pathway that generates the endogenous pAg IPP, revealing an intricate regulation of the pAg recognition system [80]. Both periplakin and RhoB GTPase were shown to bind to the membrane proximal region of BTN3A1 intracellular domain, which locates between the pAg-sensing B30.2 domain and the transmembrane region [70,80]. Intriguingly, while RhoB GTPase binds to the apo form of the BTN3A1 full-length intracellular domain, in the presence of pAg the interaction is mostly inhibited [80]. As mentioned earlier, the membrane proximal region undergoes a pAg-induced conformational change, and due to its strategic position in the BTN3A1 molecule, may be key for transitioning the full-length protein to the active state [72]. This suggests that the pAg-induced conformational change and membrane reorganization of BTN3A1 are coupled.
3.4. What is the role of the BTN3A2 and BTN3A3 family members?
Most of the focus on the role of BTN3A in pAg-induced T cell activation has been on BTN3A1, as it has been shown to be the indispensable BTN3A family member in mediating activation and the only one to sense pAg via its intracellular domain. However, the BTN3A family has two other members, BTN3A2 and BTN3A3, that have highly homologous extracellular domains to BTN3A1 but differ intracellularly. BTN3A2 and A3 have been shown to have immunological roles in other systems [82], yet their function in pAg-induced Vγ9Vδ2T cell stimulation has not been carefully explored until recently. While the original characterization of BTN3A1 noted that pAg-induced activation of Vγ9Vδ2T cells only occurred in cells with reintroduction of BTN3A1 (with shRNA knockdown of all three BTN3A family members), the elimination of A2 and A3 was not complete due to the limitation of the shRNA interference technique [15]. In the same study identifying a role for periplakin in pAg-induced T cell activation, contribution of the A2 and A3 family members to T cell activation was noted [70]. In addition to BTN3A knockdown target cells, A1, A2 and A3 single knockdown cells lines were also generated in this study; A2 knockdown target cells had a defect in mediating both endogenous IPP and microbial HMBPP-induced T cell activation as severe as the A1 knockdown, whereas the A3 knockdown also exhibited a significant albeit milder defect. This strongly suggests a non-redundant role of both the A2 and A3 family members.
Other supporting evidence came from a search for the minimal genetic requirements of pAg-induced T cell activation. As mentioned earlier, murine cells transduced with BTN3A1 are not sufficient to activate Vγ9Vδ2T cells in the presence of pAg. A study tested Chinese hamster ovarian (CHO) cells transduced with BTN3A1 alone or with BTN3A1 in conjunction with different human chromosomes for the ability to activate Vγ9Vδ2T cells [81]. In addition to the requirement for BTN3A1, CHO cells transduced with human chromosome 6 were able to mediate pAg-induced Vγ9Vδ2T cell activation. While many candidate genes reside on chromosome 6, the BTN3A locus containing all three genes are located within the MHC region on Chromosome 6, supporting an important role for all three family members in Vγ9Vδ2 activation.
Precedent exists for the requirement for multiple types of butyrophilin molecules in mediating their function. Recently, two independent research groups both investigated the intraepithelial lymphocytes in murine intestine and found that the resident γδ T cells are shaped and regulated in a TCR dependent manner by BTN-like 1 and 6 (BTNL1/6) molecules expressed on enterocytes [83–85]. BTNL molecules are structurally similar to BTN molecules, and in the case of BTNL1 and 6, they also harbor a similar intracellular B30.2 domain like BTN3A1. Importantly, both groups discovered that co-expression of BTNL1 and 6 is the key to eliciting a cognate γδ T cell response. Moreover, one group showed that the surface expression of BTNL6 was facilitated by BTNL1 and the two proteins formed high-molecular weight intrafamily complexes on the cell surface [85]. The interplay between BTNL proteins and their relationship with tissue-resident γδ T cells is potentially generalizable, as a parallel system in human intestine exists where BTNL3 and 8, both having a intracellular B30.2 domain, specifically coordinate to regulate intestinal Vγ4+T cells [83]. Interestingly, the authors found that BTNL3 and 8 could not be expressed effectively on cells transfected with their respective genes, unless both were co-expressed, which is reminiscent of the BTNL1/6 system [83].
It is thus tempting to speculate that BTN3A1 may also form complexes with the A2 and A3 family members; their extracellular domains are extremely similar and the residues within the potential dimer interfaces are conserved. Analogous to BTNL1/6, the formation of heteromultimers might help the surface expression of these molecules, especially BTN3A1 that is responsible for pAg sensing. This hypothesis may explain the apparent discrepancy between the original A1 rescue experiment in BTN3A knockdown cells [15] and the demonstration of the requirement for A2 and A3 in activation [70]. In the knockdown experiments, reintroduced BTN3A1 was under the cytomegalovirus (CMV) promoter and therefore overexpressed, this could potentially compensate for a defect of its surface expression due to the decreased levels of A2 and A3. In the A2 and A3 knockout studies, the overall expression of A1 was still at the endogenous level and was likely more susceptible to expression or activation defects due to the loss of endogenous A2 and A3. The importance and complexity regarding the interplay among BTN3A family members is becoming clear; the study of BTN3A2 and A3 will be critical to establishing a more comprehensive view of how BTN3A molecules mediate pAg-induced T cell activation.
4. What is the connection between BTN3A and Vγ9Vδ2T cells in detection of cellular stress?
BTN3A serves as the initial sensor of changes in pAg concentration through its intracellular B30.2 domain. Vγ9Vδ2T cells require the expression of the Vγ9Vδ2TCR to detect this signal, indeed reactivity to pAg can be transferred with the Vγ9Vδ2TCR. A recent genome database analysis of mammalian species showed a strong concomitant conservation of functional Vγ9 chains, Vδ2 chains, and BTN3 genes [86]. In this study, all three genes were identified in species of both placental magnorders, but were lost in lagomorphs and most rodents. In alpaca, the Vγ9Vδ2TCR showed characteristic Vγ9JP rearrangements and in-frame Vδ2 rearrangements, and was shown to be functional in vivo. Intriguingly, the alpaca BTN3 not only contains a B30.2 intracellular domain, but also harbors a complete conservation of the proposed pAg-interacting residues. These observations suggest co-evolution of the BTN3A genes and assembly of the Vγ9Vδ2TCR.
While BTN3A and the Vγ9Vδ2TCR are the two known required mediators in this process, the exact interplay between BTN3A and the Vγ9Vδ2TCR remains unclear. While evidence for a direct association between them is controversial [63,64] and has not been supported by other groups, the interaction between individual BTN3A1 and Vγ9Vδ2TCR molecules might be too weak to be detected by conventional methods such as surface plasma resonance (SPR). However, in a cellular setting, these molecules are both confined in two dimensions on the plasma membrane and, in the case of BTN3A, are likely to be multimerized. This enhanced effector concentration and higher avidity thus could support a direct interaction. Alternatively, the Vγ9Vδ2TCR may recognize a conformation of BTN3A1 that is induced through binding of pAg to the intracellular domain, mimicked by binding of the 20.1 antibody to the extracellular domains. This conformation may not be well represented in recombinant BTN3A extracellular domains. Lastly, there may be a requirement for heterodimerization of the BTN3A family members. While the extracellular domains of all BTN3A family members are highly homologous, there might be subtle structural differences between the BTN3A1 homodimer and BTN3A heterodimers that are discernable to the Vγ9Vδ2TCR.
The interplay between BTN3A and Vγ9Vδ2TCR might be further complicated by different stimulatory signals. A recent study by Starick et al. found that a murine reporter cell line transducted with different Vγ9Vδ2TCR clonotypes reacted differentially towards the 20.1 antibody stimulation, but not the pAgs [87]. This distinction is less evident when tested in the human Jurkat T cell system, hinting other accessory molecules of human origin may be at play. In addition, in this study, both the 20.1 and 103.2 full-length antibody and single chain version of the 20.1 antibody inhibited the pAg response of the murine transductants [87]. These observations suggest that different stimulators might utilize slightly different strategies to trigger Vγ9Vδ2T cell activation through BTN3A, with 20.1 stimulation relying more heavily on CDR3 sequences of the TCR and potentially other accessory proteins of human origin.
While it is compelling to speculate that the Vγ9Vδ2TCR directly engages BTN3A molecules on the surface of target cells, it is also possible that other molecules may be recruited during sensing of pAg that are the cognate ligands for the Vγ9Vδ2TCR. Evidence to support this includes the inability of BTN3A alone to support activation when introduced into murine cells (necessary but not sufficient). The characterization of periplakin and RhoB GTPase as intracellular BTN3A1 binders positions BTN3A1 as a hub in protein-protein interaction networks relevant to the activation process. It would be interesting to revisit the previous identified activators (Table 1), especially the ones that reported to have direct interactions with the Vγ9Vδ2TCR, and investigate their roles in the new framework of BTN3A-mediated pAg activation of Vγ9Vδ2T cells. Moreover, further genomic or proteomic screens will likely reveal candidates that will connect the pAg-sensing BTN3A1 from target cells to the TCR of Vγ9Vδ2T cells.
4.1. Conclusions and perspectives
There has been intensifying interest in γδ T cells, motivated by findings such as the identification of a tumor-associated γδ T cell gene signature as the best correlate of overall survival across 25 cancers and 14 solid non-brain tumor cases [88]. Human Vγ9Vδ2T cell, a major peripheral blood subset, has long been shown to have potent antitumor activity as well as the ability to fend off microbial infections. However, recent computational research has shown that Vγ9Vδ2 tumor-infiltrating cells did not correlate with hallmarks such as inflammation, infiltration of other innate immune cells, antigen processing and presentation, and cytolytic activity, and was variably associated with a favorable outcome, suggesting that distinct contexts underlie Vγ9Vδ2 T cell mobilization in cancers [89]. Moreover, clinical efficacy targeting Vγ9Vδ2T cells as effectors has not yet been convincing [3]. Thus, to fully realize the potential of these cells in medical applications, the need to precisely define the antigen recognition mechanism is paramount.
Great progress has been made since the discovery of BTN3A’s critical role in Vγ9Vδ2T cell recognition of pAgs. We now know that pAgs are detected through binding to the intracellular B30.2 domain of BTN3A1 and biophysical and structural data support a pAg induced conformational change of the membrane proximal region within the B30.2 intracellular domain of BTN3A. This initial sensing may be key to transmitting an activating signal to the outside of the target cell. Moreover, BTN3A molecules likely immobilize and multimerize upon pAg sensing, potentially with the help of the cytoskeletal network. Consistent with this idea, two protein partners of BTN3A1 that function in cytoskeletal regulation have been identified, hinting at a protein network orchestrating the activation process. In addition to these regulators, the non-redundant roles of the BTN3A2 and A3 family members are becoming recognized.
More recently, the importance of BTN3A in clinical settings has also been demonstrated. For example, a recent study examined the role of BTN3A molecules in a human acute myeloid leukemia (AML) xenotransplantation model and showed that treatment with the agonist antibody 20.1 against BTN3A could enhance Vγ9Vδ2-mediated cell lysis and sensitize poor monocytic differentiated blasts to Vγ9Vδ2T cell killing [90]. In another study, the importance for BTN3A1 in tumor cell recognition in zelodranate-treated colorectal cancer (CRC) cells has been recognized [91]. The study also showed that subcellular distribution rather than expression level of BTN3A1 might contribute to its sensitizing effect on Vγ9Vδ2-mediated cytotoxicity towards CRC cells [91].
Despite the major breakthrough in identification of the BTN3A proteins, it is still unclear what exactly is recognized by the Vγ9Vδ2TCR on target cells. Perhaps the TCR recognizes a certain conformation of BTN3A1 that is induced by pAg, or BTN3A molecules in an oligomeric state, or a different molecule that is recruited either extracellularly or intracellularly by pAg-bound BTN3A1. Given the potential complexity of the system, genomewide screens or large-scale proteomics may be key to identifying the additional factors at play in this process. Combined with the wealth of information on Vγ9Vδ2T cells that exists from decades of study, these breakthroughs on BTN3A are contributing to a more complete view of a T cell antigen recognition mechanism that is unprecedented in its nature (Fig. 3).
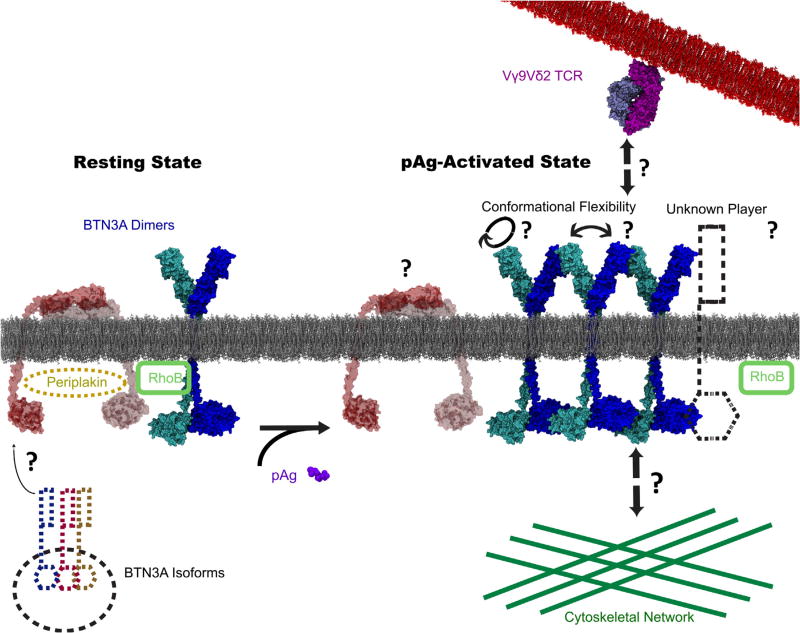
In this model, BTN3A isoforms help BTN3A1, the pAg-sensing molecule expresses on the cell surface of target cells. BTN3A1, at resting state, most likely adopts a “V-shaped” form. However, when pAg reaches a detectable level during infection or tumorigenesis, binding of pAg to the intracellular domain of BTN3A1 causes a conformational change that facilitate the whole molecule to adopt an activating state that is necessary for T cell activation. The activating state could either be a slightly rotated V-shaped, or head- to-tail, or other unknown form. The transition is either accompanied or followed by the membrane reorganization of BTN3A1, including immobilization and multimerization events, with the help of cytoskeletal system. The Vγ9Vδ2TCR either recognizes the altered conformation BTN3A1 in its oligomeric form, or recognizes another unknown TCR ligand that is recruited by the active form of BTN3A1. The whole process is likely regulated by many other proteins. The two known regulators, RhoB GTPase and periplakin, are depicted in this model.
Acknowledgments
This work is financially supported by National Institutes of Health (NIH) R01 grant No. AI115471.
References
Full text links
Read article at publisher's site: https://doi.org/10.1016/j.semcdb.2018.02.007
Read article for free, from open access legal sources, via Unpaywall:
https://europepmc.org/articles/pmc6129423?pdf=render
Citations & impact
Impact metrics
Citations of article over time
Alternative metrics
Smart citations by scite.ai
Explore citation contexts and check if this article has been
supported or disputed.
https://scite.ai/reports/10.1016/j.semcdb.2018.02.007
Article citations
Overcoming limitations for antibody-based therapies targeting γδ T (Vg9Vd2) cells.
Front Immunol, 15:1432015, 31 Jul 2024
Cited by: 0 articles | PMID: 39144149 | PMCID: PMC11321970
Review Free full text in Europe PMC
Human platelet lysate enhances in vivo activity of CAR-Vδ2 T cells by reducing cellular senescence and apoptosis.
Cytotherapy, 26(8):858-868, 12 Mar 2024
Cited by: 0 articles | PMID: 38506769 | PMCID: PMC11269029
The B7:CD28 family and friends: Unraveling coinhibitory interactions.
Immunity, 57(2):223-244, 01 Feb 2024
Cited by: 5 articles | PMID: 38354702
Review
Diester Prodrugs of a Phosphonate Butyrophilin Ligand Display Improved Cell Potency, Plasma Stability, and Payload Internalization.
J Med Chem, 66(22):15309-15325, 07 Nov 2023
Cited by: 1 article | PMID: 37934915 | PMCID: PMC10683022
A distinct topology of BTN3A IgV and B30.2 domains controlled by juxtamembrane regions favors optimal human γδ T cell phosphoantigen sensing.
Nat Commun, 14(1):7617, 22 Nov 2023
Cited by: 8 articles | PMID: 37993425 | PMCID: PMC10665462
Go to all (40) article citations
Similar Articles
To arrive at the top five similar articles we use a word-weighted algorithm to compare words from the Title and Abstract of each citation.
Butyrophilin 3A (BTN3A, CD277)-specific antibody 20.1 differentially activates Vγ9Vδ2 TCR clonotypes and interferes with phosphoantigen activation.
Eur J Immunol, 47(6):982-992, 27 Apr 2017
Cited by: 34 articles | PMID: 28386905
Activation of human γδ T cells by cytosolic interactions of BTN3A1 with soluble phosphoantigens and the cytoskeletal adaptor periplakin.
J Immunol, 194(5):2390-2398, 30 Jan 2015
Cited by: 101 articles | PMID: 25637025 | PMCID: PMC4337483
Alpaca (Vicugna pacos), the first nonprimate species with a phosphoantigen-reactive Vγ9Vδ2 T cell subset.
Proc Natl Acad Sci U S A, 117(12):6697-6707, 05 Mar 2020
Cited by: 19 articles | PMID: 32139608 | PMCID: PMC7104304
Immune recognition of phosphoantigen-butyrophilin molecular complexes by γδ T cells.
Immunol Rev, 298(1):74-83, 05 Oct 2020
Cited by: 18 articles | PMID: 33017054
Review
Funding
Funders who supported this work.
NIAID NIH HHS (1)
Grant ID: R01 AI115471
NIBIB NIH HHS (1)
Grant ID: T32 EB009412
National Institutes of HealthNational Institutes of Health (NIH) (1)
Grant ID: AI115471