Abstract
Free full text

Acute loss of Apolipoprotein E triggers an autoimmune response that accelerates atherosclerosis
Abstract
Objective
Dyslipidemia is a component of the metabolic syndrome, an established risk factor for atherosclerotic cardiovascular disease, and is also observed in various autoimmune and chronic inflammatory conditions. However, there are limited opportunities to study the impact of acquired dyslipidemia on cardiovascular and immune pathology.
Approach and results
We designed a model system that allows for the conversion to a state of acute hyperlipidemia in adult life, so that the consequences of such a transition could be observed, through conditionally deleting apolipoprotein E (APOE) in the adult mouse. The transition to hypercholesterolemia was accompanied by adaptive immune responses, including the expansion of TH1, TFH and T regulatory subsets and the formation of germinal centers. Unlike steady-state Apoe-/- mice, abrupt loss of APOE induced rapid production of antibodies recognising rheumatoid disease autoantigens. Genetic ablation of the germinal center reduced both autoimmunity and atherosclerosis, indicating that the immune response that follows loss of APOE is independent of atherosclerosis, but nevertheless promotes plaque development.
Conclusions
Our findings suggest that immune activation in response to hyperlipidemia could contribute to a wide range of inflammatory autoimmune diseases including atherosclerosis.
Introduction
Hyperlipidemia is a major risk factor for atherosclerotic cardiovascular disease. Accumulation of low-density lipoprotein (LDL) particles in the arterial intima elicits both innate and adaptive immune responses, the outcome of which is a chronic inflammatory process with build-up of atherosclerotic plaques 1,2. Retention and modification of LDL in the arterial wall leads to scavenging by macrophages, resulting in foam cell production, cholesterol microcrystal formation, and production of interleukin-1beta 3. LDL itself is also a major antigenic factor driving an adaptive immune response 4,5. Components of LDL are presented as autoantigens to T cells, which can contribute to macrophage activation and also instigate a B cell response leading to the production of antibodies to modified LDL epitopes 6. A recently published clinical trial shows that blockade of inflammatory signal by intravenous administration of an anti-IL-1b antibody significantly reduces clinical events in patients with atherosclerosis 7.
Studies of monogenic disorders of lipid metabolism, such as familial hypercholesterolemia, have yielded important information about its mechanisms and regulation. Most cases of cardiovascular disease in humans are, however, not due to monogenic disorders but complex conditions that involve exogenous factors including nutrition and infections as well as genetically controlled, intrinsic ones. In recent years, it has become evident that the metabolic syndrome characterized by hyperlipidemia, hypertension, abdominal obesity, and insulin resistance is an important contributor to cardiovascular morbidity. It is an acquired condition with signs of chronic inflammation and with a hyperlipidemic profile that involves elevated very low density lipoprotein (VLDL) as well as LDL particles. Unfortunately, our understanding of the relationship between acquired conditions such as metabolic syndrome, chronic inflammation, and atherosclerosis are limited, in part due to limited opportunities to study these conditions by experimental approaches.
Genetic mouse models that have provided important insights into the pathogenesis of atherosclerosis are based on gene targeting in the germline, leading to congenital defects in lipoprotein metabolism. One of the most widely used mouse models is the apolipoprotein E–deficient (Apoe−/−) mouse, in which targeted deletion of the Apoe gene 8,9 leads to severe hyperlipidemia and spontaneous atherosclerosis together with inflammation. The Apoe−/− model shows several similarities to the human metabolic syndrome, including chronic inflammation and insulin resistance 10. However, it is difficult to dissect the relationship between hyperlipidemia, inflammation, and atherosclerosis due to the congenital nature of disease in this model.
The other commonly used mouse model is based on targeting of the LDL receptor. Due to differences in lipid metabolism between rodents and man, the Ldlr-/- mouse is dyslipidemic but not hypercholesterolemic at birth. Instead, cholesterol rises upon feeding of a high-fat, "Western" diet, making it possible to induce the disease process postnatally. For obvious reasons, the metabolic profile of the Ldlr-/- mouse is more similar to familial hypercholesterolemia than it resembles the metabolic syndrome. Furthermore, the change of diet introduces a possible confounding factor in the experiment. Thus, it is not currently possible to separate the immune response to hyperlipidemia vis à vis the inflammatory response to atherosclerotic lesion formation by using currently available genetic mouse models.
Dyslipidemia is also associated with a number of other autoimmune conditions, particularly rheumatoid arthritis, systemic lupus erythematosus (SLE) 11 and Sjögrens disease. It is well-established that a pre-existing rheumatological autoimmune disorder increases the probability of developing cardiovascular disease. One possibility is that dyslipidemia could be a common initiating and contributing factor for both atherosclerosis development and these more orthodox autoimmune conditions. There is mounting evidence that cholesterol loading of hematopoietic cells can lead to immune abnormalities 12. Dendritic cell-specific loss of the ATP binding cassette transporters A1 and G1 (ABCA1/G1) results in SLE-like symptoms 13, as does maintaining Apoe-/- mice on a western diet 14. Alternatively, a recent report suggested loss of Apoe must be combined with loss of liver X receptor beta (Lxrβ) to induce autoimmunity and kidney pathology 15. Nevertheless, the consequences of these dysregulated immune responses on atherosclerosis development remain unknown. Is this autoimmunity actually a defence response that helps prevent atherosclerotic plaque formation, or is the autoimmune response to dyslipidemia a broad contributor to multiple disease states?
We reasoned that if the Apoe gene were deleted in the adult mouse, this would lead to rapid hypercholesterolemia and potentially reveal any inflammatory responses that accompany this disruption in homeostasis. Upon conditional deletion of the Apoe gene in the adult mouse we observed a rapid T and B cell response culminating in the formation of germinal centers and systemic autoimmunity. Genetic loss of either mature B cells or germinal center responses revealed that this autoimmune response promotes atherosclerotic lesion development. Our findings demonstrate that dyslipidemia initiates broad adaptive immune responses that could have consequences for multiple chronic autoimmune diseases.
Material and Methods
The data that support the findings of this study are available from the corresponding author upon reasonable request.
Mice
Mice containing a floxed allele of Apoe have been described previously 16 and backcrossed to the C57/Bl6 strain at 10 times. The ROSA26CreERt2 strain 17 was imported from Jackson lab and backcrossed to the C57/Bl6 strain 9 times. The Apoe-/- mouse line 18 was originally obtained from Taconic. The Pax5-/- 19 Pax5fl/fl 20, Aicda-Cre and Cd23-Cre 21 lines have been previously described22 and were crossed onto the Apoe-/- background. The Pax5fl/fl Apoe-/-, Pax5+/- Apoe-/- Aicda-Cre and Pax5+/- Apoe-/- Cd23-Cre mouse lines were bred and maintained independently and intercrossed together to generate experimental and control littermates, and backcrossed to the C57/Bl6 strain at least 6 times. Mice were maintained under specific pathogen-free conditions. Breeding mice were fed standard low fat diet R36 (1260kJ/100g, 18% protein, 4% fat; Lantmännen, Sweden) and adult experimental mice received chow diet R70 (1254kJ/100g, 14% protein, 4,5% fat; Lantmännen, Sweden). Western diet consisted of R638 (15,6MJ/kg, 17,2% protein, 21% fat; Lantmännen, Sweden). All animal experiments were performed according to valid ethical permits and regularly controlled by the Swedish Veterinary authorities and the Stockholm Board for Animal Ethics.
Tamoxifen induction
A 60mg/ml solution of tamoxifen was prepared by dissolving first in ethanol and then peanut oil and heating to 50°C until the tamoxifen is dissolved so that the final solution contained 10% ethanol and 90% peanut oil by volume. Approximately 150μl of this solution (9mg total tamoxifen) was administered as a single dose by oral gavage using a bulb-tipped feeding needle into mice aged 10 to 12 weeks of both genders, unless otherwise stated. Mice were routinely bled several days after tamoxifen induction to monitor plasma cholesterol levels. Occasional experimental mice that did not show the expected increase in plasma cholesterol were excluded from the analysis.
Splenectomy
Mice were anaesthetized with isofluorane and a 1cm incision was made on the left side of the abdomen. The spleen was extracted from the peritoneal cavity and vessels and nerves slowly cauterized with a burner. The peritoneum and skin were finally sewed with reabsorbing vicryl sutures. Sham-operated mice were opened and re-sutured thereafter. After approximately 2 weeks of recovery, mice received tamoxifen as described above and were fed western diet.
Bone marrow transplantation
Donor mice were sacrificed with carbon dioxide and the tibia and femur from both hind legs were removed. Bones were crushed using a pestle and mortar. The cell suspension was then filtered through a 100 μm nylon mesh and the erythrocytes were lysed on ice using in-house made Ammonium-Chloride-Potassium buffer. Cells were then counted, washed in Hanks’ Balanced Salt solution (1X) (HBSS), and the pellet was re-suspended to the final concentration of 4 million cells per 100 μl in HBSS. Each recipient mouse received two doses of 4,8 Gy irradiation (X-RAD 320 from PXi Precision X-Ray) with 3 hours between the doses, and was then injected by tail vein injection with 100 μl of cell suspension. Recipient mice were then maintained in a specific pathogen-free environment and fed daily for 3 weeks with wet food. Three months after bone marrow transplantation tamoxifen was administered.
Western blot
Plasma was mixed with 1:40 in PBS and thereafter diluted 1:1 with Laemmli sample buffer (62.5 mM Tris–HCl pH 6.8, 10% v/v glycerol, 1% v/v SDS, 0.005% w/v bromophenol blue, 355 mM 2-mercaptoethanol). The samples were loaded onto SDS-PAGE gels and then transferred to Immobilon-P polyvinylidene difluoride (PVDF) membranes (Millipore, MA, USA) Membranes were stained with Ponceau and cut into two pieces at 50 kDa. The membranes were washed and thereafter blocked in 10% w/v skim milk (SM) in Tris-buffered saline containing 0.1% v/v Tween-20 (TBST) for 60 mins at RT. After washes in TBST, the the membrane was cut in two and incubated with anti-mouse transferrin antibody (ab82411 Abcam) diluted 1:500 as a loading control or with anti-mouse ApoE (ab20874 Abcam) diluted 1:500 both in 0.5% w/v BSA/TBST overnight at 4°C. Membranes were washed three times in TBST for 10 min and incubated with fluorescently labelled secondary antibody 1:10000 in 5% v/v SM/TBST for 1h at RT. The membranes were washed three times for 10 min in TBST and proteins were visualized using Li-Cor Odyssey imaging system.
Cholesterol and lipid profiling
The levels of cholesterol and triglycerides in mouse plasma were quantified using an enzymatic colorimetric method (Randox Laboratories) following the manufacturer’s protocol. Cholesterol lipoprotein profiles in plasma were determined by size-exclusion chromatography using HR10/30 Superose 6 column (GE Healthcare) and a Discovery BIO GFC-500 precolumn (5 cm × 7.8 i.d.; Supelco; Sigma-Aldrich) coupled to Prominence UFLC system (Shimadzu). The system was equilibrated with Tris-buffered saline, pH 7.4, and fractions were collected using Foxy Jr. fraction collector (Teledyne Isco, Inc.). Total cholesterol in each fraction was determined as described above.
Atherosclerosis analysis
Mice of a defined sex were analysed23, as indicated in the figure legends. Aortas for en face staining were rinsed in 70% ethanol for 5 min, and stained in Sudan IV (5 g Sudan IV Sigma, 500 ml 70% ethanol, 500 ml 100% acetone) for 7-8 min. Following a washing step in 80% ethanol for 30 sec, aortas were rinsed in PBS and photographed using a light microscope. Lesion quantification was performed with ImageJ software. Oil Red O working solution was prepared from a stock solution (1g oil red O, O0625 Sigma, in 100 ml isopropanol) by diluting 150 ml of stock in 100 ml H2O. Aortic root sections were incubated in filtered Oil Red O working solution for 20 min and rinsed in H2O for 5 min. Hematoxylin (Mayers HTX, Histolab) staining was carried out and rinsed in tepid H2O. Lesion quantification was performed with ImageJ software in 8 consecutive sections, each 100 µm apart from each other24.
Flow cytometry
Spleen, lymph nodes, bone marrow, aorta and peritoneal cavity lavage were fractioned to single cell suspension and filtered with 100 µm sterile cell strainers. Erythrocytes, when present, were lysed for 3 min with 1 ml of ACK buffer on ice. Cells were seeded at 100x106 cells/ml and Fc blocking was carried out for 30 min. Staining was performed on ice for 40 min against mouse CD3ε (PB 500A2 or PerCP 145-2C11), CD4 (APCH7 GK1.5), CD5 (PE 53-7.3), CD8 (FITC 53-6.7), CD25 (PE-Cy7 PC61.5), c-kit (PE 2B8), CD44 (PE IM7), CD62L (APC MEL-14), CXCR5 (biotin, 2G8), PD-1 (AF647 29F.1A12), B220 (APC-Cy7 RA3-6B2), CD19 (APC 1D3), GL7 (APC GL-7), CD95 (PE-Cy7 Jo2), IgD (PerCP 11-26c.2a), IgM (Pacific Blue Il/41), CD21 (FITC 7G6), CD23 (PECy7 B3B4), CD11b (PerCP M1/70), CD11c (APC HL3), Ly6C (FITC AL-21), Ly6G (PB 1A8), F4/80 (PECy7 BM8), Sca1 (APC D7), CD48 (FITC HM48-1) and CD150 (PE-Cy7 TC15-12F12.2). For intracellular staining, following staining for the extracellular markers, splenocytes were treated with the Foxp3/Transcription Factor Staining Buffer Set kit (eBioscence) according to the manufacturer´s protocol and stained with the intracellular antibodies FOXP3 (PE FJK-16s) and Tbet (Brilliant Violet 421 4B10) for 30 min on ice. Immune cell populations were defined as follows: B1 (CD19+B220lo), B1a (CD19+B220loCD5+), B1b (CD19+B220loCD5-), B-2 (CD19+B220+), immature B (CD19+B220+IgM+IgD-), recirculating (CD19+B220+IgM+IgD+), pro-B (CD19+B220+ IgM−IgD-c-Kit+CD25−), pre-B (CD19+B220+ IgM−IgD-c-Kit-CD25+), germinal center B (CD19+B220+IgD-GL7+CD95+), transitional subset 1 (B220+IgMhiIgDlo), transitional subset 2 (B220+IgMhiIgDhi), follicular B (B220+IgMloIgDhi), marginal zone (B220+IgMhiIgDloCD21+CD23lo), DN (CD4-CD8-), DP (CD4+CD8+), CD4SP (CD4+CD8-), CD8SP (CD4-CD8+), naïve CD4 T-cell (CD3+CD4+CD8-CD44-CD62L+), effector/memory CD4 T-cell (CD3+CD4+CD8-CD44+CD62L-), TFH (CD3+CD4+CD44+PD-1+CXCR5+), B-1 (CD19+B220lo), B-2 (CD19+B220+), (CD19+B220hiIgM+), neutrophils (lin-CD11b+Ly6G+), CD11b+CD11c+ cells, eosinophils (Lin-CD11b+CD11c-Ly6G-SSChi), inflammatory monocytes (Lin-CD11b+CD11c-Ly6G- SSClo F4/80-Ly6Chi), macrophages (Lin-CD11b+CD11c-Ly6G- SSClo F4/80+Ly6C+), patrolling macrophages (Lin-CD11b+CD11c-Ly6G- SSClo F4/80+Ly6C-), HSC (Lin-Sca1+c-Kit+ CD150+ CD48-), MPP (Lin-Sca1+c-Kit+ CD150- CD48-), HPC1 (Lin-Sca1+c-Kit+ CD150- CD48+) and HPC2 (Lin-Sca1+c-Kit+ CD150+ CD48+). Samples were acquired with a Dako CyAn (Beckman Coulter) flow cytometer and analysed with FlowJo software.
Immunofluorescence
Spleen sections (10 μm in thickness) were fixed in ice-cold acetone and blocked with Fc-Block (CD16/CD32) in PBS 0.1% BSA prior to incubation with Alexa Fluor 488-conjugated antibody to mouse GL7 (GL7, Biolegend), APC-conjugated IgD (11-26c.2a, eBioscience) and counterstained with DAPI (Sigma D8417). For detection of immune complex deposition in the kidneys, kidneys were mounted in OCT and 6 µm thick frozen sections were blocked with normal horse serum (Vector S-2000) then stained against IgM and IgG2c whilst nuclei were DAPI stained
Aortic arches were harvested, removed surrounding fat and adventitia and fixed in 4% PFA. After they were cut open longitudinally the arch containing lesser and greater curvature was pinned on a parafilm bed. Spleen sections (10 µm in thickness) were fixed in 4% PFA. Permeabilisation was performed with 0.2% Triton X-100 in PBS. Blocking was carried out with normal horse serum followed by primary antibody (CD11c N418, biotin, Pharmingen), and secondary antibody (Streptavidin Dylight 488 conjugated (Vector SA-5488)) staining. Subsequently tissue was stained with Nile red (Sigma N3013)and finally DAPI. Confocal images were taken using a Leica TCS inverted microscope.
In vitro stimulation of T-cells
Cell culture was performed in IMDM supplemented with penicillin/streptomycin, glutamine, 2-mercaptoethanol (all from Invitrogen), and 8% heat-inactivated FCS (Sigma). To analyze cytokine production, PMA (50 ng/ml) and 5 μM ionomycin (both from Sigma) were added to cultures of total splenocytes (4x106/ml) for 3 hours in the presence of Brefeldin A (5μM) Sigma). The eBioscience Fixation/Permeabilization Kit was used for intracellular staining of cells for cytokines using the antibodies Ifn-γ (XMG1.2), Il-10 (JES5-16E3) and Il-4 (11B11).
Cell isolation from aorta
Mice were sacrificed using carbon dioxide and the vasculature was perfused by left ventricular puncture using PBS. All abdominal organs were removed leaving aorta with adventitia and heart intact. Spleens were collected as digestion controls. Peri-aortic adipose tissue and para-aortic lymph nodes were carefully removed under a dissection microscope. Whole aortas were then micro-dissected and weighed. Spleens were cut in pieces with the same weight as the aortas for digestion control. Tissues were then incubated for 60 min in 37°C in an enzymatic solution (1ml/10mg tissue) of PBS and 20mM HEPES with 488 U/ml Collagenase I, 230 U/ml Collagenase XI, 125 U/ml Hyaluronidase and 60 U/ml DNase I. After digestion, tissues were mashed through 70-μm cell strainers and analysed by FACS.
Enzyme-linked immunosorbent assay (ELISA)
ELISA for total immunoglobulin isotypes in mouse plasma were performed by coating plates with isotype-specific antibodies for capture. Detection was carried out using alkaline phosphatase coupled goat anti-mouse Ig isotype (Southern Biotechnology Associates) and alkaline phosphatase yellow (pNPP) Liquid Substrate (Sigma-Aldrich). For autoantigens, ELISA plates were coated with, PC-BSA (Biosearch Technologies), Cardiolipin (Sigma-Aldrich), LPS (Invivogen) and recombinant Insulin (Sigma-Aldrich). Serum was added after blocking and antigen-reactive IgG was measured with alkaline phosphatase – conjugated anti – mouse IgG antibody (SouthernBiotech). Antibodies against Ro-52, Ro-60, SmD1 and SS-B were measured using commercial kits (Signosis, Inc) following manufacturer’s instructions. All samples were corrected for background binding.
CuOx-LDL and MDA-LDL were prepared as described previously 25. AP-labelled goat anti-mouse IgM (μ-chain specific; Sigma-Aldrich) and goat anti-mouse IgG (γ-chain specific; Sigma-Aldrich) was used for detection. T15-clonospecific antibodies (e.g. E06) in plasma were quantified using a chemiluminescent-based capture assay. In brief, the monoclonal anti-idiotypic antibody AB1-2, a mouse IgG1 which identifies a determinant requiring co-expression of the canonical T15 VH/T15 VL regions 26 was used as capture antibody. T15-clonospecific antibodies were detected using an AP-labelled goat anti-mouse IgM (μ-chain specific; Sigma-Aldrich). Purified E06, a clonospecific IgM monoclonal antibody 27, was used as a positive control. ApoE protein levels in mouse plasma were measured with a Mabtech ELISA kit (product code 3752-1HP-2) according to manufacturer´s instructions.
Statistics
Quantitative data are presented as mean±standard error of the mean (SEM). Outliers were identified using ROUT analysis. Normal distribution was examined using either the D’Agostino-Pearson or K-S test. Differences between two groups were tested by Student’s t test for unpaired means or MannWhitney test, if normal distribution was not confirmed. One-Way or Two-way of Variance (ANOVA) was used for analysis of more than two groups followed by Bonferroni’s Multiple Comparison test or uncorrected fisher’s LSD. Statistical significance was assumed if P reached a value of 0.05 or lower. All data was analysed in GraphPad Prism.
Results
Deletion of the Apoe gene in the adult mouse creates an inducible model of hyperlipidemia and atherosclerosis
We hypothesized that somatic deletion of the Apoe gene in the adult mouse would produce a similar phenotype as germline deletion of Apoe 8,9. This experimental approach is dependent on achieving a very high deletion frequency of the Apoe allele, as re-introduction of the APOE protein into Apoe−/− mice to only 4% of wild type levels (achieved by bone marrow transplantation) fully rescues blood lipid profiles and prevents atherosclerosis 28. We crossed a floxed allele of Apoe 16 with the ROSA26CreERt2 tamoxifen-inducible Cre line 17. The floxed allele was further crossed with the germline deleted Apoe (Apoe-/- strain) allele 18, to create Apoefl/- ROSA26 CreERt2/+ experimental mice. Hence, in the experimental mice, only one floxed allele needs to be deleted. For control mice, the wild type allele was crossed with the floxed allele to produce Apoefl/+ ROSA26 CreERt2/+.
We administered a single dose of tamoxifen by oral gavage and monitored deletion of the APOE protein in plasma by Western blot. The Apoefl/+ ROSA26 CreERt2/+ strain (henceforth referred to as control mice) showed unchanged levels of APOE protein following tamoxifen ingestion. However Apoefl/- ROSA26 CreERt2/+ mice (henceforth referred to as experimental mice) manifested a noticeable reduction in plasma APOE levels already 48 hours after Cre induction. One week after the start of the deletion time course, the APOE protein was barely detectable and remained so 21 days after tamoxifen administration (Fig1A and FigSIA).
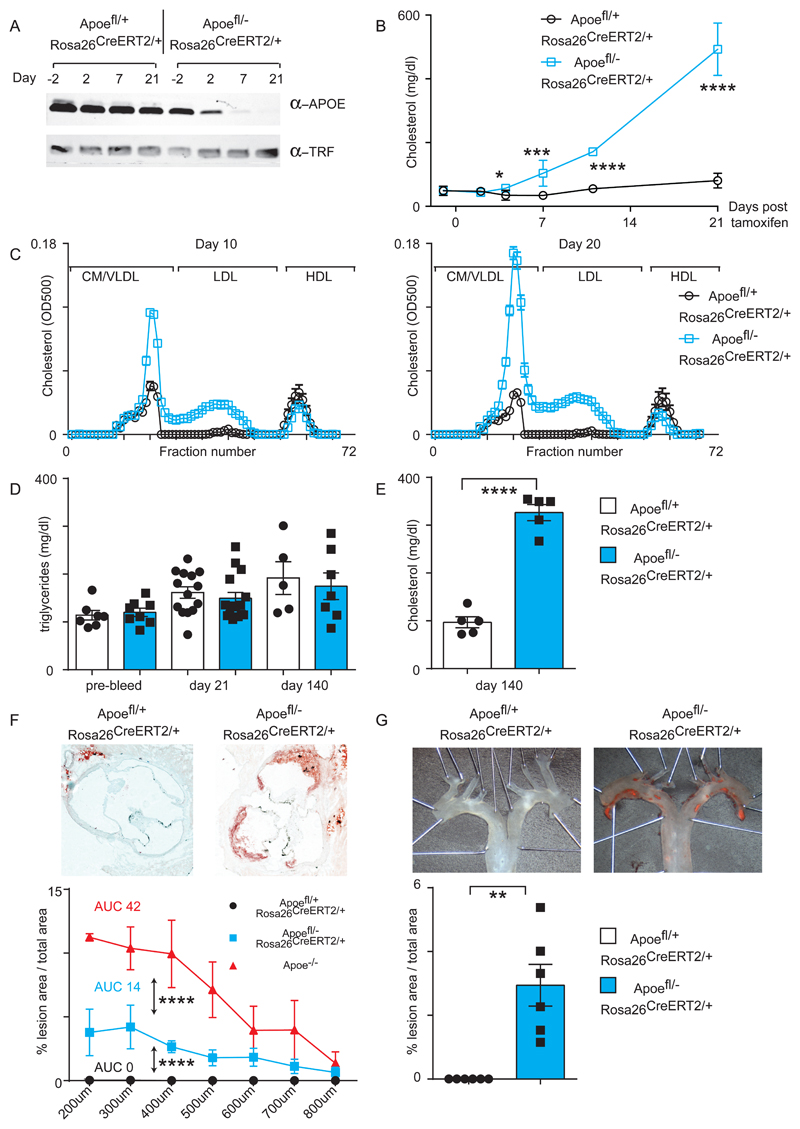
(A) Control and experimental mice (10 to 12 weeks of age) were administered an oral dose of tamoxifen and plasma levels of APOE protein were determined by Western blot. Transferrin (TRF) protein was used as a loading control.
(B) Measurement of plasma cholesterol at indicated time points. Control Apoefl/+ ROSA26 CreERt2/+ mice are shown in black (n= 4-8) and experimental Apoefl/- ROSA26 CreERt2/+ mice are in blue (n= 5-8). Significance determined by student t-test for individual groups of mice sacrificed at the indicated time point.
(C) Lipoprotein profiling for cholesterol content of plasma at day 10 (left panel) and day 20 (right panel) following tamoxifen administration. n= 5 mice for each group.
(D) Plasma triglycerides measurements at day -2, day 21 and day 140. Control n=5-14 and experimental n=7-15 mice per group.
(E) Plasma cholesterol measurement at day 140 after initiation of Apoe deletion. n= 5 mice per group. Significance determined by student t-test.
(F) Atherosclerosis in Oil Red O stained aortic root at 20 weeks post-tamoxifen administration. Representative pictures from female mice are shown in the top panel and quantification in the bottom panel for male mice. Significance determined by 2-way ANOVA. Control n=6 and experimental n=5 mice per group.
(G) Atherosclerosis in Sudan IV-stained en face aorta arch. Representative pictures from female mice are shown in the top panel and quantification for male mice in the bottom panel. Significance determined with a Mann-Whitney test. Control n=6 and experimental n=6 mice per group.
All univariate scatter plots are ± SEM. *p < 0.05, ***p < 0.001, ****p < 0.0001 compared to control mice.
We next measured plasma cholesterol levels at various time points after the administration of tamoxifen. Four days post-deletion we noticed a significant increase in cholesterol levels that continued upwards at later time points, reaching over 500mg/dl 21 days post induction, a similar cholesterol level to what we observe in the Apoe-/- strain 29 (Fig1B). Additionally, control mice showed enrichment of cholesterol in the HDL fraction as expected, whereas experimental mice incorporated cholesterol into their chylomicron/VLDL fraction already 10 days post-tamoxifen and this was further increased at day 20 (Fig1C). Non-fasted plasma triglyceride levels were unaltered between control and experimental mice even 140 days after APOE deletion (Fig1D).
Next, we investigated if loss of APOE in the adult is sufficient to induce atherosclerosis similar to the conventional Apoe-/- strain. We examined mice 140 days after a single tamoxifen treatment, maintained on a chow diet throughout, and confirmed that plasma cholesterol remained elevated (Fig1E), and APOE protein was largely absent at this time point (FigSIB). We isolated hearts and aortas from both control and experimental mice, and quantified atherosclerotic lesions in Oil Red O stained cryosections of the aortic root (Fig1F) or en face within the aortic arch and thoracic aorta following Sudan IV staining (Fig1G). As expected, control mice were negative for plaque formation. However, experimental mice maintained on a normal chow diet readily demonstrated atherosclerotic lesion formation that is characteristic of the Apoe-/- strain, although the atherosclerotic burden was lower than the Apoe-/- strain (Fig1F). In summary, by deleting the Apoe gene in the adult mouse, we created an inducible model of atherosclerosis and hyperlipidemia.
Hyperlipidemia can induce inflammation independently of atherosclerosis plaque formation
We hypothesized that an acute increase in plasma lipid levels may activate the immune system in a manner that is not evident in the conventional Apoe-/- strain. When analysing the spleen at day 10 following APOE deletion, we observed a marked increase in B cells bearing the surface activation markers CD95 and GL7, indicating that germinal centers (GCs) are formed at the very onset of hyperlipidemia (Fig2A). Immunofluorescence of spleen sections at day 10 confirmed the presence of GCs (Fig2B). These GCs could also be observed at day 21 (FigSIC,D), and were maintained up to day 140 (FigSIE). Marginal zone B cells were also expanded at this extended day 140 time point (FigSIE). This induction of GCs was not representative of a general phenomenon of splenic B cell activation or accumulation, as other splenic B cell subsets were not significantly increased at these early time points (FigSIE). Also, loss of Apoe did not induce a general phenotype of B cell activation, as lymph node B cells (FigSIIA), peritoneal B cell populations (FigSIIB), and bone marrow B cell progenitors were unaltered (FigSIIC) at day 10 post-tamoxifen.
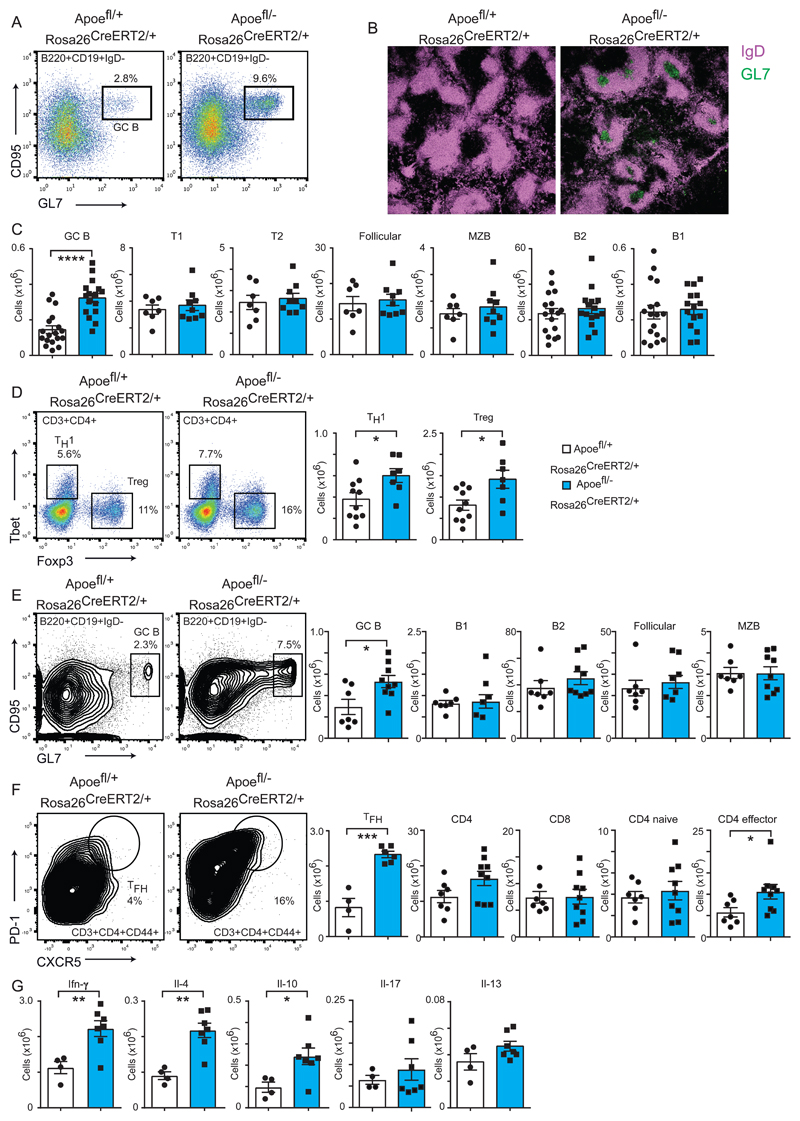
(A) Representative FACS analysis depicting percentages of germinal center B cells formation in the spleen of mice on chow diet for 10 days after administration of tamoxifen.
(B) Histological analysis of germinal centers at day 10 following tamoxifen administration by confocal microscopy. Cryosections of spleens corresponding to the indicated genotypes were stained with eFluor488-labeled GL7 antibody (green) and APC-labelled IgD antibody (purple).
(C) Quantification of the indicated B cell populations in the spleen as determined by flow cytometry 10 days after administration of tamoxifen. Absolute cell numbers of control Apoefl/+ ROSA26 CreERt2/+ mice shown as white bars (n= 7-17) and experimental Apoefl/- ROSA26 CreERt2/+ mice are in blue (n= 9-16), representing mixed genders maintained on chow diet.
(D) Induction of TH1 and Treg lineages in the spleen as determined by intracellular staining for TBET and FOXP3 respectively. Mice were analysed 10 days after administration of tamoxifen and maintained on a chow diet. Representative FACS panels are shown in the left panel and quantification of the total cell number is shown in the univariate scatter plots on the right. Control n=10 and experimental n=7 mice per group, both genders.
(E) Germinal center formation (left panel) in the spleen at day 70 following tamoxifen administration and administration of a western diet. The right panel depicts spleen B cell numbers for the indicated subsets. Control n=6-7 and experimental n=6-9 mice per group, mixed genders.
(F) Flow cytometry depicting TFH cell formation (left panel) and quantification of T cell subsets in the spleen 70 days after tamoxifen administration and administration of a western diet. Control n=4-7 and experimental n=6-9 mice per group, mixed genders.
(G) Cytokine production in activated T cells following in vitro stimulation and intracellular staining. FACS analysis of the spleen 70 days after tamoxifen administration and administration of a western diet Absolute number of cells for the indicate cytokine are shown. N=4 for controls and n=7 for experimental mice, mixed genders.
All univariate scatter plots are ± SEM. Significance determined by student t-test. *p < 0.05, **p < 0.01, ****p < 0.0001 compared to control mice.
We next examined the T cell populations, as CD4+ T cells have been shown to become activated in the context of atherosclerosis 5. We were unable to detect a significant increase in the absolute numbers of CD4+ or CD8+ T cells at any time point up to 140 days, or a transition of CD4+ T cells from a naïve (CD44-CD62L+) to an effector/memory state (CD44+CD62L-) 10 or 21 days after tamoxifen administration (FigSIIIA,B), but limited activation was present at day 140 (FigSIIIC). Similarly, splenic myeloid populations were unaffected at day 10 (FigSIVA). We further examined the splenic CD4+ T cell pool to determine if specific effector lineages were induced. The data showed significant increases in TH1 (CD4+ Tbet+) and Treg (CD4+ Foxp3+) populations in the spleen at day 10 (Fig2D), when blood cholesterol levels have approximately tripled in experimental mice.
We next wished to determine if this specific lymphoid activation present in the spleen at day 10, typified by GC formation, precedes definitive atherosclerosis formation. Unsurprisingly, visual inspection of the aorta 10 days after tamoxifen administration, corresponding to only 6 days of raised plasma cholesterol levels, did not reveal any fatty streak formation (data not shown). We further tested for intracellular neutral lipid deposition through Nile Red staining of the luminal face of the lesser curvature of the aorta as previously performed (FigSIVB). Control and experimental mice were negative for Nile Red positive cells at day 10. However, day 140 experimental mice showed extensive Nile Red positive cells within the aorta. Furthermore, investigation of T and B cell recruitment to the aorta at day 18 by flow cytometry revealed no significant differences in lymphocyte numbers (FigSIVC).
We next subjected the mice to a western diet regime to determine if immune activation in peripheral lymphoid organs beyond the spleen could be induced. Under these conditions we still observed GC formation at day 21 (FigSVA) and day 70 (Fig2E) following tamoxifen addition, while other B cell subsets were not expanded. However, additional GC formation could also be observed in peripheral aortic and inguinal lymph nodes (FigSVB). Hematopoietic stem cell populations and B cell progenitors in the bone marrow were also expanded (FigSVIA,B). Analysis of the T cell compartment at day 70 following tamoxifen administration in mice maintained on a western diet revealed that T follicular helper cells, but not T cells in general, were increased in frequency and number (Fig2F). Intracellular cytokine staining following in vitro stimulation with phorbol 12-myristate 13-acetate (PMA) and ionomycin revealed increases in interferon-γ, interleukin-4 and interleukin-10 positive cells in experimental mice compared to controls. Levels of interleukin-13 and interleukin-17 were not significantly increased (Fig2G). Finally, we compared control and experimental mice with Apoe-/- and Ldlr-/- mice, with all strains placed on a western diet for 2 weeks. As previously reported 30, Apoe-/- mice displayed GC formation in the spleen, but not the Ldlr-/- strain. T cell subsets were broadly similar across all four strains, with an exception of lower CD8+ T cell numbers in Apoe-/- mice compared to both control and experimental strains (FigSVII).
The transition to hyperlipidemia induces broad humoral responses against self antigens
We focused on investigating the immune responses of mice 70 days after the commencement of a western diet and examined whether the observed immune activation was coupled to autoimmune B cell activation. As a consequence of the GC formation, splenic plasma cell formation was increased in experimental versus control mice (Fig3A). Experimental mice presented increases in the titres of plasma immunoglobulins IgM and IgG but not IgA. Amongst the IgG isotypes, IgG2b and IgG2c were significantly increased whereas IgG1 and IgG3 were not affected compared to control mice. Noticeably, we did not observe a similar pattern in Apoe-/- mice, which displayed elevated levels of IgA and IgG3 immunoglobulin isotypes, but had reduced IgG1 compared to both control and experimental mice (Fig3B).
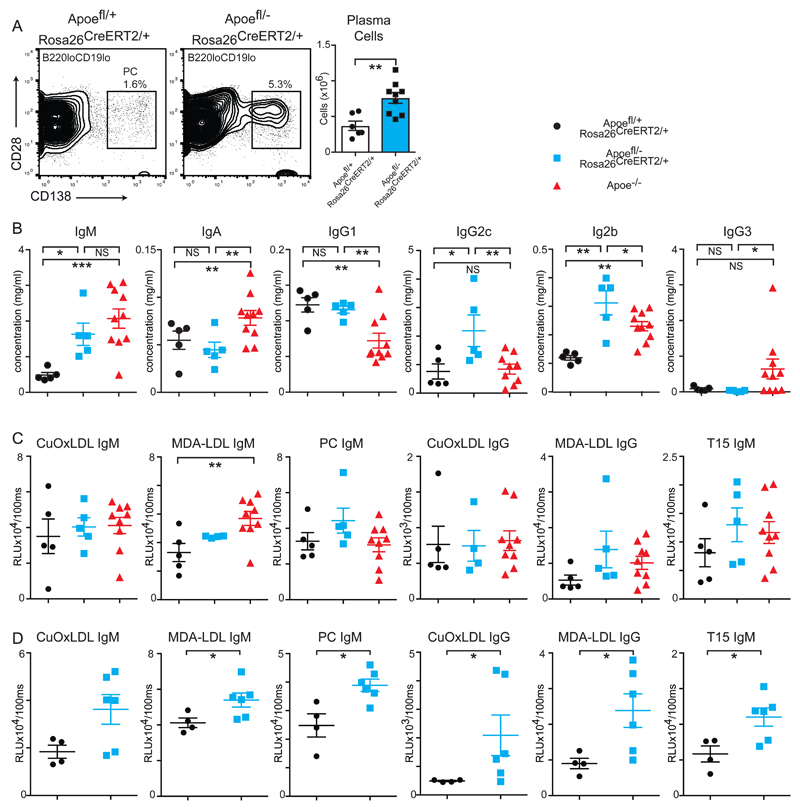
(A) Flow cytometry of splenic cells to detect plasma cells (left panel) at day 70 following tamoxifen administration and fed a western diet. Absolute cell numbers are shown on the right. Control n=6 and experimental n=8 mice per group.
(B) ELISA measurements of plasma immunoglobulin isotypes. Control Apoefl/+ ROSA26 CreERt2/+ mice are shown in black circles (n= 5), experimental Apoefl/- ROSA26 CreERt2/+ mice are in blue squares (n= 5) and Apoe-/- (n= 10) mice are red triangles. All mice were maintained on western diet for 10 weeks. Statistical significance was assessed by 1-way ANOVA.
(C) Plasma auto-antibody production as determined by ELISA. Control Apoefl/+ ROSA26 CreERt2/+ mice are shown in black circles (n=5), experimental Apoefl/- ROSA26 CreERt2/+ mice are in blue squares (n= 4-5) and Apoe-/- mice are red triangles (n= 9-10). All mice were maintained on western diet for 10 weeks. Statistical significance was assessed by 1-way ANOVA.
(D) Plasma auto-antibody production as determined by ELISA. Control Apoefl/+ ROSA26 CreERt2/+ mice are shown in black circles (n= 4), experimental Apoefl/- ROSA26 CreERt2/+ mice are in blue squares (n=6). All mice were maintained on western diet for 5 weeks. Statistical significance was assessed by Mann-Whitney test.
All univariate scatter plots are ± SEM. *p < 0.05, **p < 0.01, ****p < 0.0001 compared to control mice.
Surprisingly, these increased titres did not result in increases antibody responses against modified LDL in control, experimental or Apoe-/- mice maintained on a western diet for 10-weeks (Fig3C). However, broad increases in IgM and IgG reactivity against MDA-LDL, CuOx-LDL and anti-phosphocholine responses were significantly increased in experimental mice versus controls after 5-weeks of western diet (Fig3D).
Further humoral differences were also observed when comparing the acute response to the chronic deletion of Apoe. Experimental mice showed IgG reactivity against common autoantigens both 5 and 10 weeks after tamoxifen administration relative to controls. This included several autoantigens involved in Sjögren's syndrome and SLE, including Ro-52 and Ro-60, SmD1, cardiolipin and SSB(La). Apoe-/- mice that were also maintained on a western diet for 10 weeks did not present broad self-recognition (Fig4A&B). There were also increases in IgM and IgG antibodies against atherosclerosis-associated antigens in chow-maintained mice over time (FigSVIII). Examination of kidney sections from experimental mice, but not controls, revealed widespread IgM and IgG deposition (Fig4C). In summary, conditional loss of Apoe results in a distinct and autoimmune-biased humoral immune response compared to the homeostatic Apoe-/- strain.
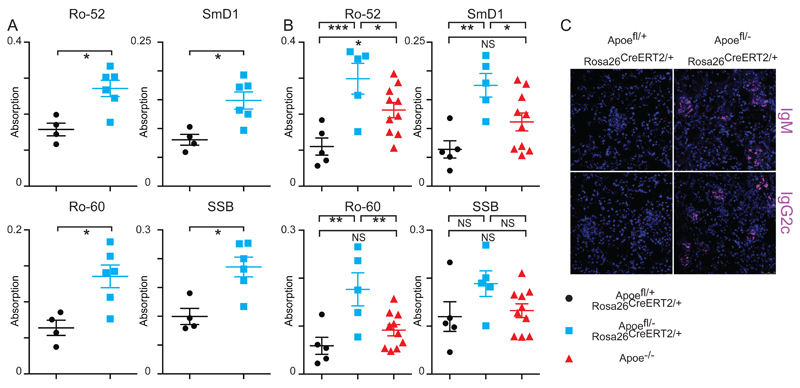
(A) Plasma autoantibody titres in controls Apoefl/+ ROSA26 CreERt2/+ shown as black circles (n=4) versus Apoefl/- ROSA26 CreERt2/+ experimental mice of mixed genders shown as blue squares (n=6) kept on western diet for 10 weeks after tamoxifen administration. All univariate scatter plots are ± SEM. Significance determined by Mann-whitney test. *p < 0.05, **p < 0.01, ****p < 0.0001.
(B) Plasma autoantibody titres in controls (black circles n=5), Apoefl/- ROSA26 CreERt2/+ mice (blue squares n=5) and Apoe-/- (red triangles n=10) kept on western diet for 10 weeks after tamoxifen administration. All univariate scatter plots are ± SEM. Significance determined by 1-way ANOVA test. *p < 0.05, **p < 0.01, ****p < 0.0001.
(C) Confocal microscopy of kidney cryosections taken from mice at day 70 following tamoxifen administration and maintained on a western diet. IgM/IgG2c is shown in purple and counterstained with DAPI (blue).
The spleen does not play an essential role in promoting inflammation in response to dyslipidemia
We next addressed the relevance of this splenic autoimmune response to atherosclerosis lesion development. The spleen has previously been shown to have a central function in regulating immune responses in atherosclerosis. We therefore performed a splenectomy or alternatively a sham operation on experimental mice maintained on a western diet for 70 days to determine the role of this organ in the response to inducible hyperlipidemia. Loss of the spleen resulted in decreases in the B2 and B1a, but not the B1b cell population in the peritoneal cavity, similar to previously reported splenectomised mice (FigSIXA). We next assessed how serum immunoglobulin titres were affected and found significant decreases in total IgM and IgG but not IgA, with IgG2b the most significantly affected of the IgG isotypes (FigSIXB). However, plasma cholesterol levels were not altered (FigSIXC). Atherosclerotic lesion development in the aortic root was slightly but significantly increased upon loss of the spleen, possibly because decreases in IgM levels can result in increased atherosclerosis (FigSIXD).
The systemic B cell response dictates the inflammatory pro-atherosclerotic response to hyperlipidemia
We next adopted a genetic approach to ablate B cell lineages systemically in response to hyperlipidemia. We engineered two strains that conditionally delete the B cell-specific transcription factor Pax5, which is required for B cell identity and function 31–33. We first used Cd23-Cre, which is expressed in transitional B cells 21 and deletes in follicular and marginal zone B cells 34, as well as memory B cells following immunisation 35. This strain was additionally crossed onto the Apoe-/- background so that they could be bone marrow transplanted into Apoefl/- ROSA26 CreERt2/+ experimental mice. Transplantation of Pax5fl/- Cd23-Cre Apoe-/- bone marrow into Apoefl/- ROSA26 CreERt2/+ hosts resulted in strong decreases in B1, B2, GC B and plasma cells compared to Pax5fl/+ Cd23-Cre Apoe-/- donors when analysed 10-weeks after tamoxifen administration and maintained on a western diet (Fig5A). Total plasma cholesterol levels were unaffected (Fig5B). However, in contrast to splenectomised mice, we observed broad decreases in immunoglobulin isotypes (Fig5C) and also reductions in autoantibody production and IgG responses against MDA-LDL (Fig5D). We next assessed atherosclerosis development and found a significant 44% decrease in aortic root lesion formation upon loss of mature B cell function and autoreactive antibody production (Fig5E).
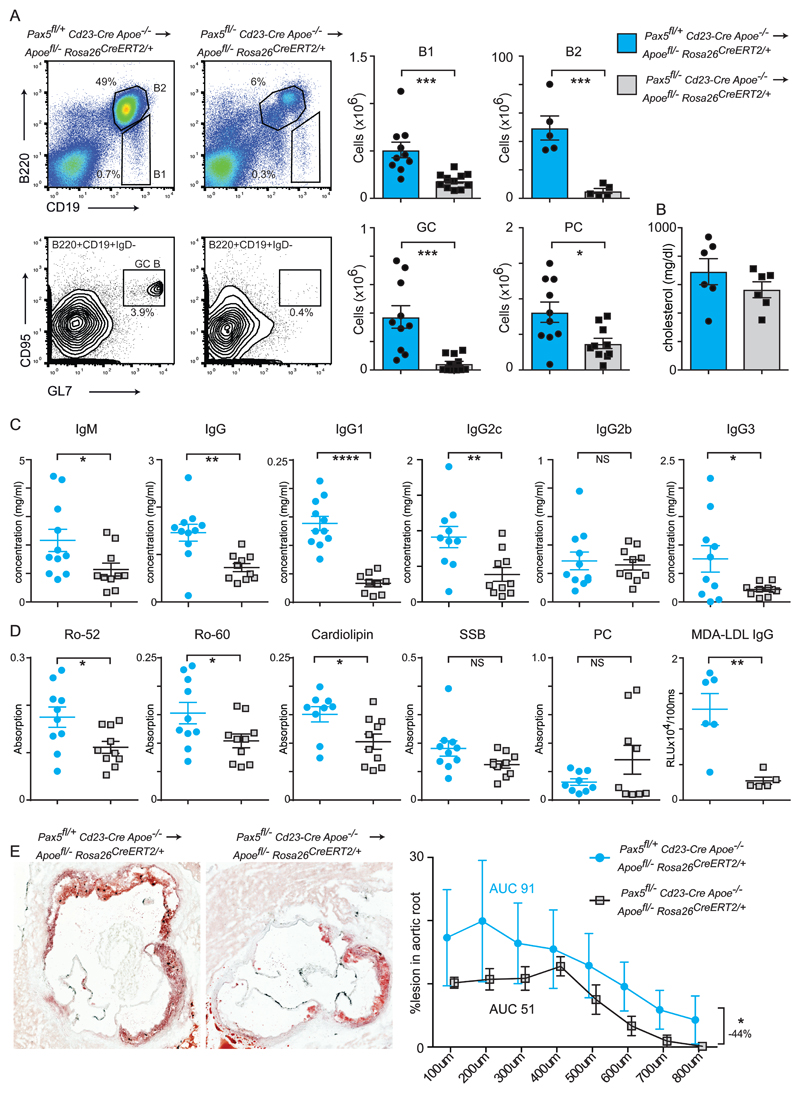
(A) Flow cytometry analysis of the spleen following bone marrow transplant of Pax5fl/+ Cd23-Cre Apoe-/- (blue bars n=10) or Pax5fl/- Cd23-Cre Apoe-/- (grey bars n=11) donors into Apoefl/- ROSA26 CreERt2/+ hosts of both genders. Analysis was performed at day 70 following tamoxifen administration and mice were maintained on a western diet. Absolute cell numbers for the indicated B cell subsets are indicated.
(B) Plasma cholesterol at sacrifice of bone marrow-transplanted hosts. N=6 for both genotypes.
(C) Antibody titres of bone marrow-transplanted hosts. N=9-11 for both genotypes.
(D) Plasma autoantibody titres in bone marrow-transplanted hosts. N=9-10 for both genotypes.
(E) Oil Red O-stained aortic root sections from female mice of the indicated genotypes. Quantification is shown in the right panel. Significance determined by 2-way ANOVA. Percentage difference between the two genotypes was calculated by area under the curve measurements.
All univariate scatter plots are ± SEM. Significance determined by student t-test unless otherwise stated. *p < 0.05, **p < 0.01, ***p < 0.001 compared to control mice.
Deletion of the germinal center response results in reduced atherosclerosis
Due to the broad B cell defects observed in Pax5fl/- Cd23-Cre Apoe-/- transplanted hosts, we next attempted to effectively ablate only the GC response. Aicda-Cre specifically deletes in GC B cells and has previously been utilised to abolish GC function 21,36. We therefore transplanted Pax5fl/- Aicda-Cre Apoe-/- bone marrow into Apoefl/- ROSA26 CreERt2/+ hosts. We observed normal B cell numbers but selective loss of GCs and plasma cells (PCs) relative to controls when analysed 10-weeks after tamoxifen administration and maintained on a western diet (Fig6A). Similar to the Pax5fl/- Cd23-Cre Apoe-/- transplanted hosts, there was no effect on plasma cholesterol levels (Fig6B). However, as expected, the loss of GC function decreased antibody titres (Fig6C) as well as autoantibody production and IgG responses against MDA-LDL (Fig6D). T cell subsets were unaffected (FigSX). Atherosclerosis was significantly decreased by 38%, directly linking the GC response to disease development (Fig6E). Similar reductions were obtained using male recipients (data not shown). These finding show that the GC dependent autoimmune response to hyperlipidemia promotes atherosclerosis development.
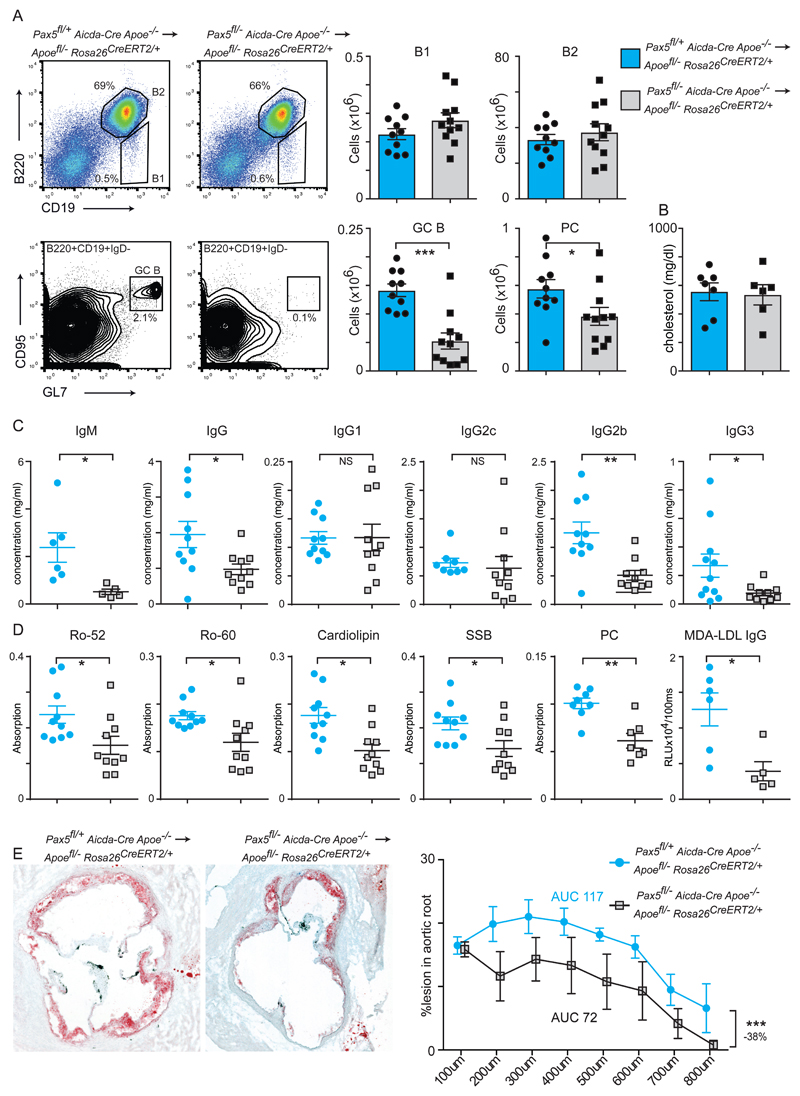
(A) Flow cytometry analysis of the spleen following bone marrow transplant of Pax5fl/+ Aicda-Cre Apoe-/- (blue bars n=10) or Pax5fl/- Aicda-Cre Apoe-/- (grey bars n=11) donors into Apoefl/- ROSA26 CreERt2/+ hosts of both genders. Analysis was performed at day 70 following tamoxifen administration and mice were maintained on a western diet. Absolute cell numbers for the indicated B cell subsets are indicated.
(B) Plasma cholesterol at sacrifice of bone marrow-transplanted hosts. N=6 for both genotypes.
(C) Antibody titres of bone marrow-transplanted hosts. N=5-11 for both genotypes.
(D) Plasma autoantibody titres in bone marrow-transplanted hosts. N=5-10 for both genotypes.
(E) Oil Red O stained aortic root sections from female mice for the indicated genotypes. Quantification is shown in the right panel. Significance determined by 2-way ANOVA. Percentage difference between the two genotypes was calculated by area under the curve measurements.
All univariate scatter plots are ± SEM. Significance determined by student t-test unless otherwise stated. *p < 0.05, ***p < 0.001 compared to control mice.
Discussion
Germline deletion of the Apoe gene results in mice that are hyperlipidemic from birth, show signs of chronic inflammation, and develop spontaneous atherosclerosis. In this study, we have discovered that transition to an APOE null state in the adult mouse leads to a sequence of events, with hyperlipidemia rapidly followed by an adaptive immune response characterised by the formation of splenic germinal centers and linked to systemic autoimmunity. Immune activation preceded the development of atherosclerotic plaques, indicating that changes in metabolism in adult life can have direct effects on immunity, independent of but impacting on atherosclerosis.
Immune activation following loss of APOE expanded TH1, TFH, Treg and GC B cell numbers. APOE has also been suggested to prevent proliferation of T cells in a non-specific manner 37. However, we did not observe a general expansion of T cells upon somatic loss of Apoe in vivo in the time windows we examined. The humoral response in conditionally deleted adult mice was noticeably different to that of germline deleted Apoe-/- mice. Although both strains increased their immunoglobulins titres compared to controls, and have ongoing germinal center activity, Apoe-/- mice can be classified as IgMhiIgAhiIgG3hiIgG1lo, whereas the Apoefl/- ROSA26 CreERt2/+ strain was IgMintIgG2bhiIgG2chi. The specificity of antibody responses was markedly different, with Apoefl/- ROSA26 CreERt2/+mice displaying broad reactivity against common autoantigens, whereas the germline deleted Apoe-/- strain showed much lower reactivity to self-antigens. It is possible that this break in tolerance is due to the abrupt shift to dyslipidemia in the conditional deletable mouse, whereas the Apoe-/- strain which is born hypercholesterolemic, has greater opportunities for immune education or longer time to anergize cells against self. Ldlr-/- mice did not develop GCs during the acute phase following a transition to hypercholesterolemia, in agreement with a recent study indicating that extended periods of a high-fat diet are necessary to induce TFH and GC formation in the Ldlr-/- strain 38. As this diet also induces atherosclerosis formation, it is not clear whether the appearance of GCs is related to the increase in plasma cholesterol or plaque formation. A strategy whereby acute hypercholesterolemia can be induced upon loss of LDLR would be required to see if the immune activation observed upon loss of APOE is a generalised response to hypercholesterolemia. Nevertheless, this strain of conditional loss of APOE will be useful for further studies whereby an acute induction of hypercholesterolemia is desired.
The spleen forms an intimate relationship with the blood by responding to circulating pathogens and autoantigens, and has a proven protective function against atherosclerosis in mice 39–42, and complications arising from atherosclerosis in humans 43,44. The spleen may also possess pro-atherosclerotic functions through the supply of monocytes 45. Noticeably, IgG1 and IgG2c were robustly produced in the absence of the spleen implicating other secondary lymphoid organs in the humoral autoimmune response. However, serum IgM titres and peritoneal B1a cells were strongly decreased following splenectomy, which are both thought to help protect against atherosclerosis lesion formation. The balance of these plaque promoting and regressing activities may explain the almost neutral effect of spleen removal with regards to atherosclerosis. The discrepancy in immune responses between the germline and inducible Apoe-/- models emphasizes the importance of further studies investigating changes in lipid metabolism in adult life as risk factors for cardiovascular and other diseases. Our inducible Apoe-/- model should be helpful in such investigations. Ingestion of a lipid-rich meal in humans can result in acute post-prandial inflammation, with short term increases in blood myeloid and lymphoid populations 46,47. In mice however, the immune response to raised blood lipid levels is thought to be of a chronic low-grade nature with monocytosis developing over time 48. This expansion in myeloid cells is believed to be a cell intrinsic function of APOE in hematopoietic progenitors 49. Here, we also observed an expansion of hematopoietic and B cell progenitors in mice maintained on a western diet. This expansion proceeds after GCs have been formed. Thus, both germline and acute loss of APOE can perturb immune homeostasis and this effect can be exacerbated by maintaining mice on a western diet.
Loss of mature B cells and also GC B cells reduced but did not entirely abolish the production of autoimmune antibodies. Nevertheless, the removal of these B cell subsets was sufficient to significantly reduce atherosclerosis burden. This shows that the adaptive immune response that occurs in response to acute hyperlipidemia upon APOE loss promotes atherosclerosis, at least with regard to humoral immunity. This does not exclude the possibility that atherosclerosis-dampening responses also occur, as we found that the Treg cell population is concomitantly expanded. Regardless, the classical autoimmune features we observe in this inducible Apoe knockout strain, such as germinal center formation and autoantibody production, underlines that atherogenesis and dyslipidemia are intimately linked to the immune system. Recent reports have demonstrated autoimmunity due to cholesterol loading of immune cells in the Apoe-/-Lxrβ-/- and Abca1−/−Abcg1−/− strains 13,15. This study adds to the weight of evidence that the use of cholesterol-lowering drugs as anti-inflammatory agents in autoimmune disorders, especially rheumatoid diseases, could have beneficial clinical outcomes.
Supplementary Material
Graphical abstract
Supplementary figures
Acknowledgments
We thank Maria Ozsvar Kozma for expert help with ELISA measurements.
Sources of funding
This research was supported the Swedish Research Council, grants K2013-65X-06816-30-4 (project grant) and 349-2007-8703 (Linnaeus support to the Center of Excellence for Research on Inflammation and Cardiovascular Disease (CERIC)), the EU FP7-Health-2013-innovation-1 programme Athero-B-Cell and the Swedish Heart-Lung foundation (Hjärtlungfonden).
Nonstandard Abbreviations and Acronyms
APOE | Apolipoprotein E |
TH1 | T lymphocyte helper cell 1 |
TFH | T follicular helper cell |
HDL | High-density lipoprotein |
LDL | Low-density lipoprotein |
VLDL | Very low density lipoprotein |
ABCA1/G1 | ATP binding cassette transporters A1 and G1 |
Lxrβ | Liver X receptor beta |
GCs | Germinal centers |
PMA | Phorbol 12-myristate 13-acetate |
MDA-LDL | Malondialdehyde-modified low density lipoprotein |
CuOx-LDL | Copper oxidized low density lipoprotein |
Footnotes
Author Contributions
MC performed atherosclerosis experiments. KEP performed Western blots. MC, KEP, MG, LH and CJB performed ELISA. MC, KEP, JS, CT, JC and KH performed FACS. KH performed bone marrow transplant and aorta analysis. JS, CT and JC performed in vitro T cell stimulation. MC and AD performed immunofluorescence. KAP and DJK performed HPLC.KEP, SM and AG performed splenectomy. DKJ performed initial characterisation of the mice
NNM provided the Apoe floxed mouse line. GKH helped with the initiation and analysis of the project and revision of the manuscript. SM designed the study, analysed the data and wrote the manuscript with input from all authors.
Disclosures
The authors have no disclosures.
References
Full text links
Read article at publisher's site: https://doi.org/10.1161/atvbaha.118.310802
Read article for free, from open access legal sources, via Unpaywall:
https://www.ahajournals.org/doi/pdf/10.1161/ATVBAHA.118.310802
Citations & impact
Impact metrics
Citations of article over time
Alternative metrics
Article citations
Autoimmune diseases and atherosclerotic cardiovascular disease.
Nat Rev Cardiol, 21(11):780-807, 27 Jun 2024
Cited by: 1 article | PMID: 38937626
Review
Adaptive immunity and atherosclerosis: aging at its crossroads.
Front Immunol, 15:1350471, 15 Apr 2024
Cited by: 3 articles | PMID: 38686373 | PMCID: PMC11056569
Review Free full text in Europe PMC
Kupffer cells dictate hepatic responses to the atherogenic dyslipidemic insult.
Nat Cardiovasc Res, 3(3):356-371, 11 Mar 2024
Cited by: 2 articles | PMID: 39196121 | PMCID: PMC11358021
Association Between Atherosclerosis-Related Cardiovascular Disease and Uveitis: A Systematic Review and Meta-Analysis.
Diagnostics (Basel), 12(12):3178, 15 Dec 2022
Cited by: 2 articles | PMID: 36553185 | PMCID: PMC9777442
Review Free full text in Europe PMC
Immunological mechanisms of exercise therapy in dyslipidemia.
Front Physiol, 13:903713, 08 Aug 2022
Cited by: 3 articles | PMID: 36003652 | PMCID: PMC9393246
Review Free full text in Europe PMC
Go to all (20) article citations
Data
Data behind the article
This data has been text mined from the article, or deposited into data resources.
BioStudies: supplemental material and supporting data
Similar Articles
To arrive at the top five similar articles we use a word-weighted algorithm to compare words from the Title and Abstract of each citation.
Germinal Center-Derived Antibodies Promote Atherosclerosis Plaque Size and Stability.
Circulation, 139(21):2466-2482, 01 May 2019
Cited by: 39 articles | PMID: 30894016
Artery Tertiary Lymphoid Organs Control Multilayered Territorialized Atherosclerosis B-Cell Responses in Aged ApoE-/- Mice.
Arterioscler Thromb Vasc Biol, 36(6):1174-1185, 21 Apr 2016
Cited by: 72 articles | PMID: 27102965 | PMCID: PMC4894775
Dyslipidemia-associated alterations in B cell subpopulation frequency and phenotype during experimental atherosclerosis.
Atherosclerosis, 247:118-126, 29 Dec 2015
Cited by: 10 articles | PMID: 26897258
Meta-Analysis of Leukocyte Diversity in Atherosclerotic Mouse Aortas.
Circ Res, 127(3):402-426, 16 Jul 2020
Cited by: 174 articles | PMID: 32673538 | PMCID: PMC7371244
Review Free full text in Europe PMC
Funding
Funders who supported this work.
Austrian Science Fund FWF (2)
Lipid-peroxidation derived epitopes and macrophages
Univ.Prof. Dr. Christoph BINDER, Medical University of Vienna
Grant ID: F 3015
Microparticles as modulators of inflammation and thrombosis
Univ.Prof. Dr. Christoph BINDER, Medical University of Vienna
Grant ID: F 5402
NHLBI NIH HHS (2)
Grant ID: R01 HL049277
Grant ID: R01 HL042630