Abstract
Background
Dormancy is one characteristic of cancer cells to make patients remain asymptomatic before metastasis and relapse, which is closely related to the survival rate of cancer patients, including head and neck squamous cell carcinoma (HNSCC). PRRX1 has previously been implicated in the invasion and metastasis of the epithelial-mesenchymal transition (EMT) process in different types of human carcinoma. However, whether PRRX1 can regulate cancer dormancy and its reactivation, leading to the migration and invasion of HNSCC cells, remains elusive. The aim of this study was to determine the role of PRRX1 in cellular phenotype plasticity and cancer dormancy of HNSCC cells and its association with miRNAs in HNSCC.Methods
The expression of PRRX1 was detected by immunohistochemical staining in primary HNSCC samples and the metastatic lymph nodes. Meanwhile, the role of PRRX1 and its relationship with miR-642b-3p and EMT in cellular phenotype plasticity and cancer dormancy of HNSCC were investigated in vitro and in vivo.Results
PRRX1 was significantly higher at the invasive front of HNSCC samples compared with the metastatic lymph nodes, and such switch process was accompanied by the cellular phenotype plasticity and cell dormancy activation. In HNSCC cell lines, PRRX1 positively promoted the expression of known EMT inducers and cooperated with activated TGF-β1 to contribute to EMT and migration and invasion of HNSCC cells. Then, we found that overexpression of miR-642b-3p, one of the most significantly downregulated miRNAs in PRRX1-overexpressed cells, significantly reduced the migration and invasion, and increased cell proliferation and apoptosis. And miR-642b-3p restoration reversed PRRX1-induced cell dormancy and EMT of HNSCC cells through TGF-β2 and p38. Finally, we demonstrated that overexpressed PRRX1 was closely correlated with miR-642b-3p downregulation and the upregulation of TGF-β2 and p38 in a xenograft model of HNSCC.Conclusions
Our findings showed that PRRX1 may be one of the main driving forces for the cellular phenotype plasticity and tumor dormancy of HNSCC. Therefore, we can raise the possibility that EMT may help to keep cancer cell in dormant state and mesenchymal-epithelial transition may resurge dormancy in HNSCC.Free full text

PRRX1 Regulates Cellular Phenotype Plasticity and Dormancy of Head and Neck Squamous Cell Carcinoma Through miR-642b-3p12
Associated Data
Abstract
BACKGROUND: Dormancy is one characteristic of cancer cells to make patients remain asymptomatic before metastasis and relapse, which is closely related to the survival rate of cancer patients, including head and neck squamous cell carcinoma (HNSCC). PRRX1 has previously been implicated in the invasion and metastasis of the epithelial-mesenchymal transition (EMT) process in different types of human carcinoma. However, whether PRRX1 can regulate cancer dormancy and its reactivation, leading to the migration and invasion of HNSCC cells, remains elusive. The aim of this study was to determine the role of PRRX1 in cellular phenotype plasticity and cancer dormancy of HNSCC cells and its association with miRNAs in HNSCC. METHODS: The expression of PRRX1 was detected by immunohistochemical staining in primary HNSCC samples and the metastatic lymph nodes. Meanwhile, the role of PRRX1 and its relationship with miR-642b-3p and EMT in cellular phenotype plasticity and cancer dormancy of HNSCC were investigated in vitro and in vivo. RESULTS: PRRX1 was significantly higher at the invasive front of HNSCC samples compared with the metastatic lymph nodes, and such switch process was accompanied by the cellular phenotype plasticity and cell dormancy activation. In HNSCC cell lines, PRRX1 positively promoted the expression of known EMT inducers and cooperated with activated TGF-β1 to contribute to EMT and migration and invasion of HNSCC cells. Then, we found that overexpression of miR-642b-3p, one of the most significantly downregulated miRNAs in PRRX1-overexpressed cells, significantly reduced the migration and invasion, and increased cell proliferation and apoptosis. And miR-642b-3p restoration reversed PRRX1-induced cell dormancy and EMT of HNSCC cells through TGF-β2 and p38. Finally, we demonstrated that overexpressed PRRX1 was closely correlated with miR-642b-3p downregulation and the upregulation of TGF-β2 and p38 in a xenograft model of HNSCC. CONCLUSIONS: Our findings showed that PRRX1 may be one of the main driving forces for the cellular phenotype plasticity and tumor dormancy of HNSCC. Therefore, we can raise the possibility that EMT may help to keep cancer cell in dormant state and mesenchymal-epithelial transition may resurge dormancy in HNSCC.
Introduction
Head and neck squamous cell carcinoma (HNSCC) with more than 600,000 newly diagnosed cases per year is the sixth most common cancer in the world [1]. In the developed countries, the average 5-year survival rate is ~50% [2]. Improvements in radio- and chemotherapy and recently introduced targeted antibody therapy and surgical procedures during the past decades have resulted in a significantly better quality of HNSCC patients. However, local recurrence and distant metastasis still remain common, and the rate of survival has been only marginally enhanced [3]. Therefore, it is necessary to clarify the molecular mechanism of the invasion and metastasis, which will greatly contribute to the prevention and treatment of HNSCC.
Cellular phenotype plasticity is considered as the cellular phenotype conversion between epithelium and mesenchyma [4]. Epithelial-mesenchymal transition (EMT) is a process of epithelial cell transferring to a mesenchymal phenotype, and mesenchymal-epithelial transition (MET) is reversible from EMT. Epithelial cells are polygons, E-cadherin is the gatekeeper to maintain the tight connections of epithelial cells, and cytokeratin makes up the largest subgroup of intermediate filament proteins. In contrast, mesenchymal cells with spindly fibroblastic morphology rarely build tight connections, with no E-cadherin, and vimentin is the most abundant protein in the cell skeleton. The cellular phenotype plasticity has been confirmed to play an important role in the process of tumor invasion and metastasis [5], [6].
Cancer dormancy has been shown as a period in cancer progression in which residual disease existed but remained indiscernible or asymptomatic clinically for a long time [7]. Cancer cell dormancy represents a stage of cell growth arrest, which may appear in the course of primary tumor formation and after dissemination of the primary tumor cells or in micrometastasis [8]. It has been shown that cancer cell dormancy is the major cause of metastasis which is featured with cells that are not divided and arrested in G0-G1 cell cycle [9], [10], [11].
Evidences have shown that EMT and MET are closely connected with cancer dormancy and its reactivation. Colorectal tumor cells with invasiveness and migration which have undergone EMT program manifested low levels of Ki67, the proliferation marker [12]. In metastatic hepatic niche, Chao and his colleagues found that hepatocyte triggered MET and the consequent re-expression of E-cadherin in the secondary site, which increased the survival and chemoresistance of metastatic tumor cells, a characteristic of dormancy [13]. These indicated that the cellular phenotype plasticity, to some extent, can influence cancer dormancy. More recently, Boix et al. [14] found that treatment of miR122-positive cells with an inhibitor of TGFβR1 activation abolished tumor dormancy program and recovered cell proliferation rate through a Smad-independent TGF-β response in human HCC-BCLC9 cells. This result proved that microRNAs (miRNAs), as master regulators of transcriptome, at least in part mediate cancer dormancy. We therefore hypothesized that the cellular phenotype plasticity can regulate cancer dormancy and its reactivation through miRNAs, leading to the migration and invasion of HNSCC cells. PRRX1, paired-related homeobox transcription factor, had previously been implicated in EMT program and the invasion and metastasis of carcinoma [15], [16]. Here, we would like to investigate the role of PRRX1 in cellular phenotype plasticity and cancer dormancy of HNSCC cells and its association with miRNAs.
Results
PRRX1 Associates with the Cellular Phenotype Plasticity and Tumor Dormancy at the Invasive Front of HNSCC Patients
To investigate the expression and significance of PRRX1 in HNSCC patients, immunohistochemical analysis of PRRX1 was performed at the invasive front of the 89 primary tumors and the 60 metastatic lymph nodes. The positive rate of PRRX1 at the invasive margin of HNSCC primary tumors was 67.42% (60/89), and the positive expression of PRRX1 was significantly associated with pathological classification, local recurrence, and metastasis of HNSCC patients (P < .05, Table 1). At the invasive margin of the primary tumors, tumor cells lost the abilities of aggregation and connection, dispersed around without the polarity and regularity, led to the detachment of small cell clusters, and formed the cell strips or trabs (Figure 1A and B). These isolated cells turned spindle cells with flat nucleus, and immunohistochemistry straining showed the positive expression of PRRX1 of many cancer cells (Figure 1E and F) and the negative expression of E-cadherin (Figure 1I and J). And these cells also showed positive expression of Slug and Sip1, EMT transcription factors (Supplementary Figure S1). These indicate the loss of epithelial characteristics and the gain of mesenchymal capabilities of tumor cells, that is, EMT occurred at the invasive front of the primary tumor. However, the metastatic lymph node of HNSCC cases showed cancer cell nest with many keratosic substances, an epithelial cell characteristic (Figure 1C and D), accompanying the positive expression of PRRX1, Slug, and Sip1 in few cancer cells (Figure 1G and H, Supplementary Figure S1) and the positive expression of E-cadherin of many cancer cells (Figure 1K and L). These changes of PRRX1 expression in the primary HNSCC and the metastatic lymph node were detected in 51 of the 60 (85%) HNSCC cases. Importantly, as shown in Figure1U, these differences between the primary tumor and metastatic lymph node were significant. These demonstrated that the loss of epithelial morphology of the tumor cells at the invasive front can reverse at the second sites. Hence, PRRX1 might be involved in the cellular phenotype plasticity of HNSCC.
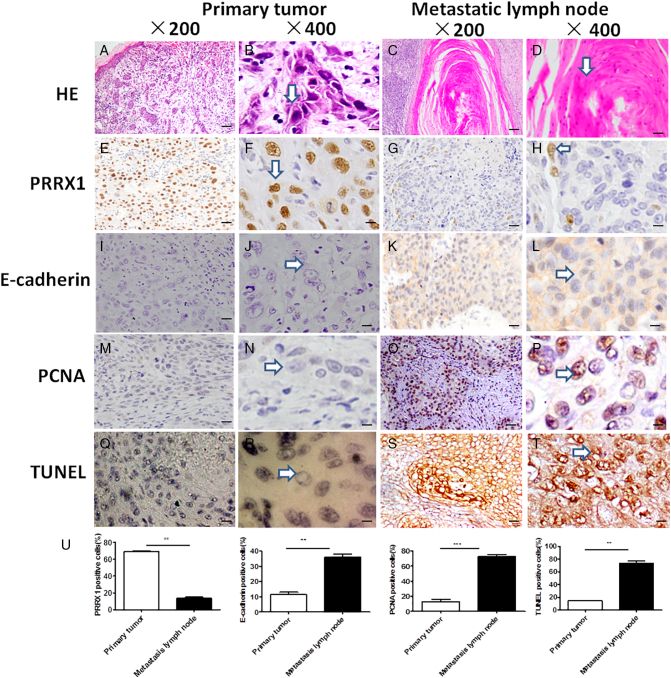
PRRX1 involved in the cellular phenotype plasticity and tumor dormancy at the invasive front of the primary tumor and the metastatic lymph node in HNSCC patients. (A-D) Cancer cells at the invasive front were flat with nucleus (arrows), and cells in the metastatic lymph node had plenty of keratosic substances (arrows). HE staining. (E-H) Immunohistochemistrical staining of PRRX1. Most of the nucleus of cancer cells at the invasive front showed positive expression of PRRX1 (arrows), while only some of the nucleus of cancer cells in the metastatic lymph node were positive (arrows). (I-L) Immunohistochemical staining of E-cadherin. The cell membrane of cancer cells in the invasive front showed negative expression of E-cadherin (arrows), while plenty of cell membrane of cancer cells in the metastatic lymph node were positive (arrows). (M-P) Immunohistochemical staining of PCNA. The nucleus of cancer cells at the invasive front showed negative expression of PCNA (arrows), while the nucleus of many cancer cells in the metastatic lymph node was positive (arrows). (Q-T) TUNEL staining of cancer cells at the invasive front showed negative expression (arrows), while cancer cells in the metastatic lymph node were positive (arrows). (U) The proportion of the positive cells of PRRX1, E-cadherin, PCNA, and TUNEL were calculated, respectively. Student's paired t test was used to analyze the differences between the primary tumor and metastatic lymph node (**P < .01, ***P < .001).
Table 1
PRRX1 Expression at the Invasive Margin of 89 HNSCC Primary Tumors and Its Association with Clinical-Pathologic Characteristics of the Patients
Clinical-Pathologic Variables | No. of Patients | PRRX1 Expression | P Value | ||
---|---|---|---|---|---|
Negative (%) | Positive (%) | ||||
Age | <50 | 31 | 11 | 20 | .465 |
≥50 | 58 | 18 | 40 | ||
Gender | Female | 35 | 10 | 25 | .275 |
Male | 54 | 19 | 35 | ||
TNM stage | I-II | 49 | 16 | 33 | .979 |
III-IV | 40 | 13 | 27 | ||
Histological classification | Grade I | 38 | 20 | 18 | .000 |
Grade II and III | 51 | 9 | 42 | ||
Local invasion | No | 66 | 24 | 42 | .037 |
Yes | 23 | 5 | 18 |
Further, we found that while the metastatic lymph node had numbers of PCNA, a proliferation marker, expressing cells (Figure 1O and P), tumor cells at the invasive front lost PCNA expression (Figure 1M and N). Importantly, TUNEL staining showed the negative expression of the disseminated tumor cells of the primary tumors (Figure 1Q and R), while the metastatic lymph node with epithelial growth pattern showed the positive expression (Figure 1S and T). The Student's paired t test confirmed that there were significant differences of PCNA and TUNEL expression between the primary tumor and metastatic lymph node (Figure1U). Hence, the acquisition of mesenchyma-like abilities of cancer cells at the invasive front was involved in tumor dormancy, a shutdown of proliferative activity. These suggest that EMT induction of tumor cells might be accompanied by tumor dormancy in HNSCC patients.
Exogenous PRRX1 Expression Induces EMT and Cell Dormancy of HNSCC Cells
To further determine the relationship between PRRX1 and EMT in HNSCC, we stably overexpressed PRRX1 in HNSCC cell lines Cal-27 by using Lentivirus infection, as confirmed by immunoblotting and real-time PCR (Figure 2A). We observed that Cal-27 cells expressing exogenous PRRX1 displayed an elongated fibroblast-like morphology with scattered distribution in culture, whereas cells transfected with vector retained their cobblestone-like morphology with tight cell-cell adhesion (Figure 2B). The PRRX1 overexpressed Cal-27 cells exhibited a significant downregulation of E-cadherin from cell-cell contacts; meanwhile, the mesenchymal markers N-cadherin and Vimentin were dramatically upregulated (Figure 2A). Further, Slug and Sip1 were elevated in response to PRRX1 overexpression in a variable extent, whereas the endogenous mRNA levels of Twist1 and Snail did not change (Figure 2C). These morphologic and molecular changes suggested an apparent transition of the PRRX1-expressing Cal-27 cells from an epithelial to mesenchymal status. Typically, the EMT phenotype is usually accompanied by the acquisition of cell traits such as greater migration and more invasive ability. As shown in Figure 2D, PRRX1 overexpressed Cal-27 cells dramatically increased their migratory and invasive behaviors. Similar results were observed in SCC-9, SCC-15, and SCC-25 cells (Figure S2, Figure S3). Together, these results show that PRRX1 is a novel inducer of EMT and promotes the migration and invasion of HNSCC cells. Importantly, we found that overexpression of PRRX1 significantly decreased cell division and apoptosis in HNSCC cells (Figure 2E and F). This showed that PRRX1 could induce EMT as well as cell dormancy in HNSCC cells.
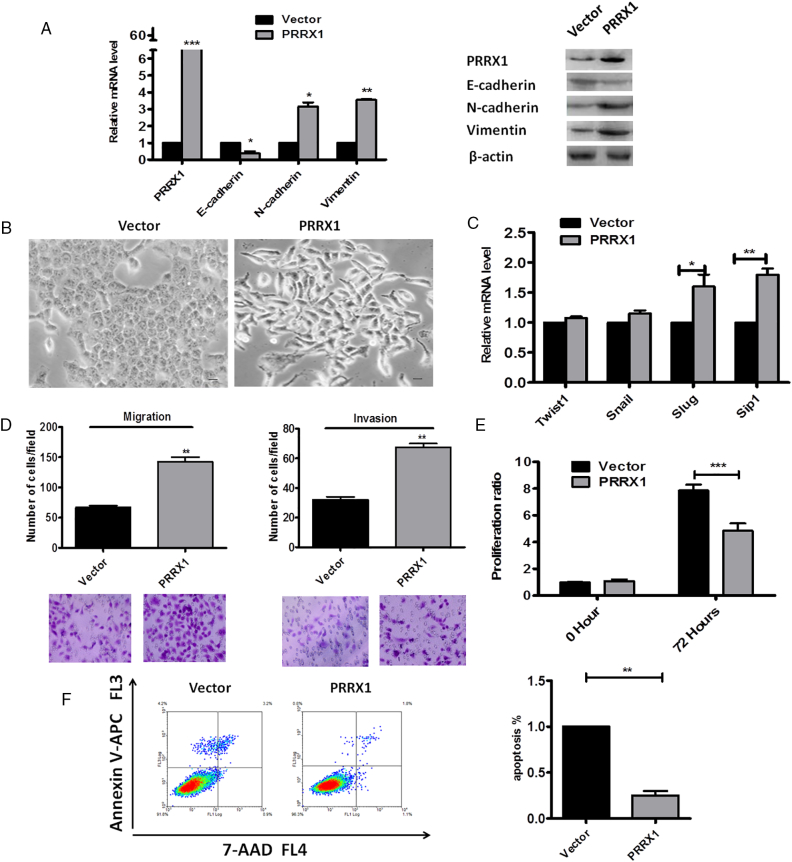
PRRX1 induces a full EMT in Cal-27 cells concomitant with the acquisition of cell dormancy. (A) Real-time RT-PCR (left panel) and Western blot (right panel) analyses of vector and PRRX1-expressing cells showed the repression of E-cadherin and the activation of N-cadherin and vimentin following PRRX1 ectopic expression. Error bars represent the mean ± SD of triplicate experiments (*P < .05, **P < .01, ***P < .001). (B) Phase-contrast images showing the phenotype of vector-transfected cells of Cal-27 cells and of those in which PRRX1 has been ectopically expressed. Scale bar, 100 mm. (C) Real-time RT-PCR assay was performed to detect the mRNA levels of Twist1, Snail, Slug, and Sip1. Error bars represent the mean ± SD of triplicate experiments (*P < .05, **P < .01). (D) Migration assay and invasion assay were conducted to measure the migratory and invasive potentials of Cal-27 cells between vector and PRRX1-expressing cells. Representative images of migrated and invaded cells were shown. Error bars represent the mean ± SD of triplicate experiments (**P < .01). (E) PRRX1 overexpression reduced Cal-27 cells proliferation based on the MTT assay. Error bars represent the mean ± SD of triplicate experiments (***P < .001). (F) FACS analysis (left panel) and quantification (right panel) of cell apoptosis in vector and PRRX1 overexpression cells. Error bars represent the mean ± SD of triplicate experiments (**P < .01).
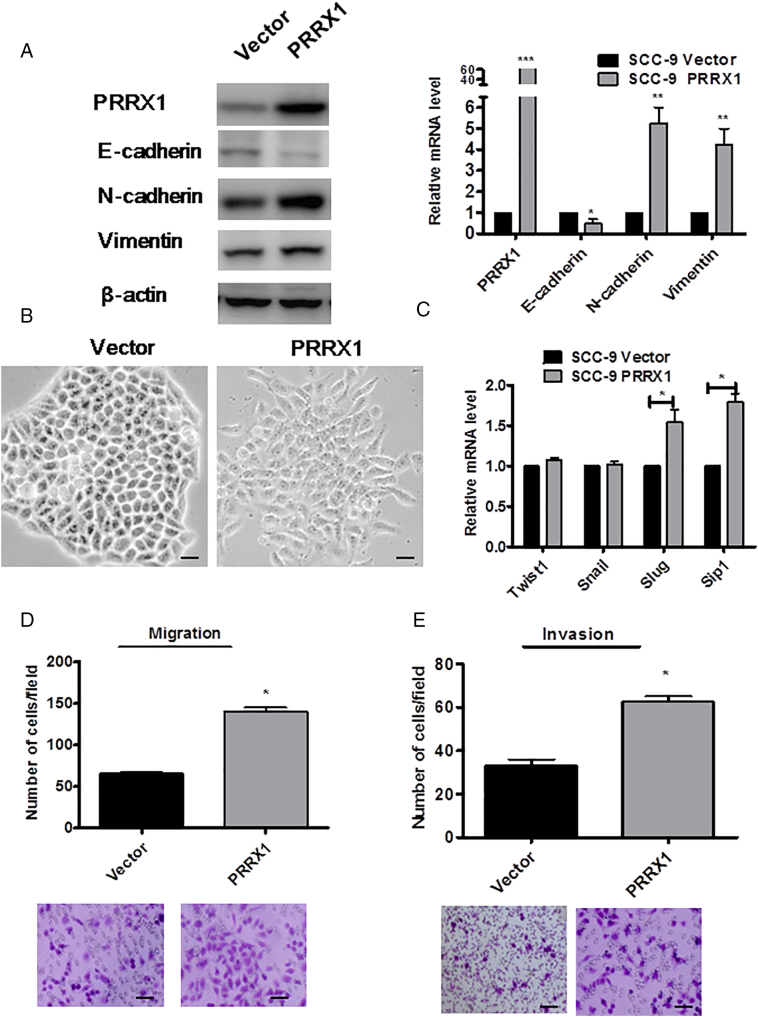
PRRX1 induces a full EMT in SCC-9 cells. (A) Western blot (left panel) and real-time RT-PCR (right panel) analyses of vector and PRRX1-expressing cells showed the repression of E-cadherin and the activation of N-cadherin and vimentin following PRRX1 ectopic expression. Error bars represent the mean ± SD of triplicate experiments (*P<.05, **P<.01, ***P<.001). (B) Phase-contrast images showing the phenotype of vector-transfected cells of SCC-9 cells and of those in which PRRX1 has been ectopically expressed. Scale bar, 100 mm. (C) Real-time RT-PCR assay was performed to detect the mRNA levels of Twist1, Snail, Slug, and Sip1. Error bars represent the mean ± SD of triplicate experiments (*P<.05). Migration assay (D) and invasion assay (E) were conducted to measure the migratory and invasive potentials of SCC-9 cells between vector and PRRX1-expressing cells. Representative images of migrated and invaded cells were shown. Error bars represent the mean ± SD of triplicate experiments (*P<.05).
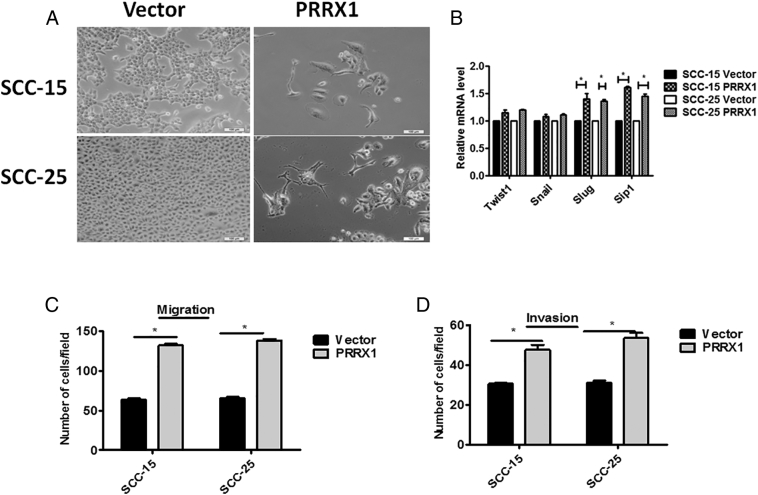
PRRX1 induces a full EMT in SCC-15 and SCC-25 cells. (A) Phase-contrast images showing the phenotype of vector-transfected cells of SCC-15 and SCC-25 cells and of those in which PRRX1 has been ectopically expressed. Scale bar, 100 μm. (B) Real-time RT-PCR assay was performed to detect the mRNA levels of Twist1, Snail, Slug, and Sip1. Error bars represent the mean ± SD of triplicate experiments (*P<.05). Migration assay (C) and invasion assay (D) were conducted to measure the migratory and invasive potentials of SCC-15 and SCC-25 cells between vector and PRRX1-expressing cells. Error bars represent the mean ± SD of triplicate experiments (*P<.05).
Loss of PRRX1 Reverts EMT to MET and Leads to Activation of Cell Dormancy
We next carried out a loss-of-function assay to further confirm the role of PRRX1 in HNSCC cells. We silenced PRRX1 using siRNA in Cal-27 cells, as confirmed by immunoblotting and real-time PCR (Figure 3A). We found that suppression of PRRX1 expression resulted in the downregulation of N-cadherin and vimentin, the upregulation of E-cadherin, and MET evidenced by the changes of morphology (Figure 3B). Slug and Sip1 were reduced in PRRX1 silenced cells, whereas the mRNA levels of Twist1 and Snail did not significantly change (Figure 3C). And silencing PRRX1 prominently impaired the migration and invasion ability of Cal-27 cells (Figure 3D). Furthermore, we found that knockdown of PRRX1 promoted cell proliferation and apoptosis in HNSCC cells (Figure 3E and F). Similar results were observed in SCC-9 cells (Supplementary Figure S4). Thus, our loss-of-function study suggested that the suppression of PRRX1 could reverse the EMT phenotype and lead to MET with resurging tumor dormancy.
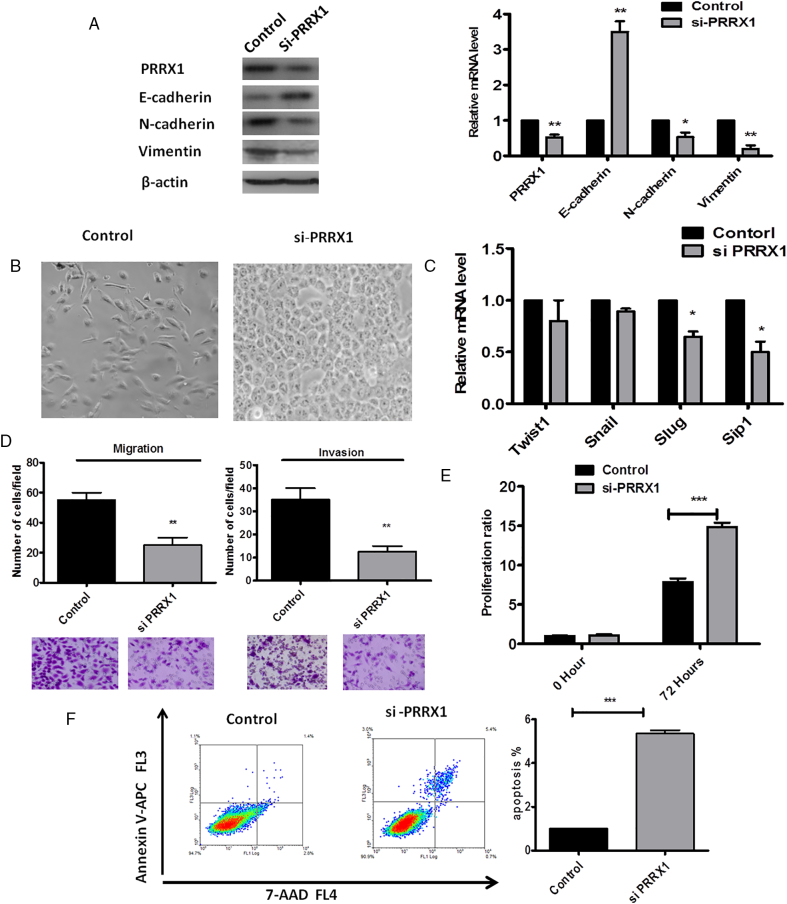
PRRX1 silencing reverts EMT in Cal-27 cells concomitant with the resurgement of cell dormancy. (A) Western blot (left panel) and real-time RT-PCR (right panel) analyses of the control and PRRX1 silencing cells showed the activation of E-cadherin and the repression of N-cadherin and vimentin following PRRX1 knockdown. Error bars represent the mean ± SD of triplicate experiments (*P < .05, **P < .01). (B) Phase-contrast images showing the phenotype of control-transfected cells of PRRX1 overexpression cells and of those in which PRRX1 has been decreased expressed. Scale bar, 100 mm. (C) Real-time RT-PCR assay was performed to detect the mRNA levels of Twist1, Snail, Slug, and Sip1. Error bars represent the mean ± SD of triplicate experiments (* P < .05). (D) Migration assay and invasion assay were conducted to measure the migratory and invasive potentials of PRRX1 knockdown cells. Representative images of migrated and invaded cells were shown. Error bars represent the mean ± SD of triplicate experiments (**P < .01). (E) PRRX1 knockdown increased Cal-27 cells proliferation based on the MTT assay. Error bars represent the mean ± SD of triplicate experiments (***P < .001). (F) FACS analysis of cell apoptosis in control cells and PRRX1 knockdown cells. The data showed that PRRX1 knockdown cells increased the ratio of cell apoptosis compared with the control cells (***P < .001).
PRRX1 Cooperated with Activated TGF-β1 to Promote EMT
Previous studies demonstrated the important function of TGF-β1 signal in EMT [17], [18]. We next investigate here whether TGF-β1 signal pathway is involved in PRRX1-induced EMT. Our results showed that the protein levels of TGF-β1, TGF-βR2, p-SMAD2, and p-SMAD3 increased in PRRX1-overexpressed Cal-27 cells and SCC-9 cells (Figure 4A). Then, we used a specific TGF-β receptor kinase inhibitor, SB431542, to block the TGF-β1 signaling in PRRX1-overexpressed Cal-27 cells and SCC-9 cells. We found that suppression of TGF-β1 signaling by the inhibitor reduced TGF-β1, TGF-βR2, p-SMAD2, and p-SMAD3 level and downregulated vimentin expression but increased E-cadherin expression (Figure 4B). Moreover, treatment of PRRX1-overexpressed Cal-27 cells and SCC-9 cells with SB431542 reduced their migration and invasive ability (Figure4C and D). These results suggest that the intensified TGF-β1 signaling induced by PRRX1 promotes cell motility and EMT.
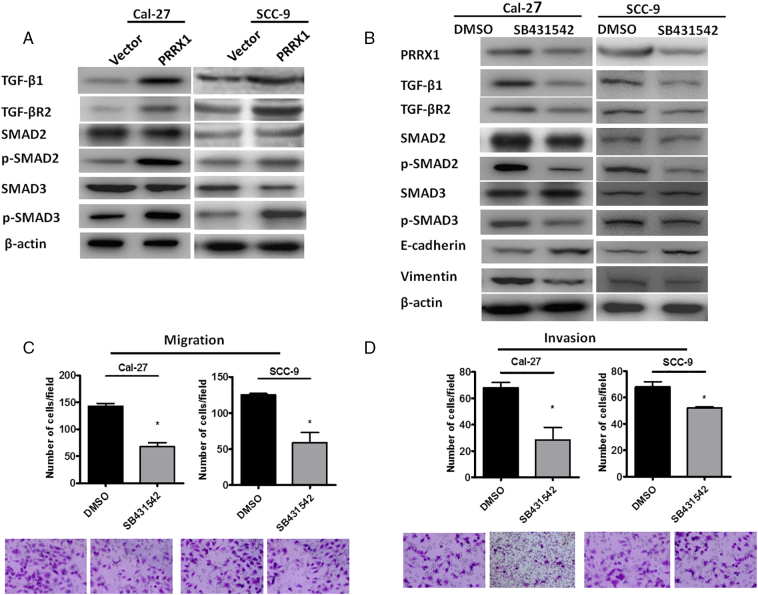
PRRX1 is required for both TGF-β1 dependent and independent EMT in HNSCC cells. (A) Western blot analyses of the vector and PRRX1 overexpression cells showed the activation of TGF-β1, TGF-βR2, p-SMAD2, and p-SMAD3 in Cal-27-PRRX1 and SCC-9-PRRX1 cells. (B) Western blot analyses of Cal-27-PRRX1 and SCC-9-PRRX1 cells showed the inhibition of TGF-β1, TGF-βR2, p-SMAD2, and p-SMAD3 after SB431542 stimulation. (C) Migration assay showed that the migratory potential of Cal-27-PRRX1 and SCC-9-PRRX1 cells decreased after SB431542 stimulation. Error bars represent the mean ± SD of triplicate experiments (* P < .05). (D) Invasion assay showed that the invasive potential of Cal-27-PRRX1 and SCC-9-PRRX1 cells decreased after SB431542 stimulation. Error bars represent the mean ± SD of triplicate experiments (*P < .05).
miR-642b-3p Was Directly Regulated by PRRX1 in HNSCC Cells
It has been reported that miRNAs, as master regulators of transcriptome, at least in part mediate cancer dormancy. Hence, we compared the miRNA expression profile of Cal-27 cells in which PRRX1 had been ectopically expressed with that of control cells by microRNA microarray (Figure 5A). Using a two-fold change and P < .05 as the threshold cutoff, we found that 97 miRNAs were upregulated and 129 were downregulated (Figure 5B; Supplementary Tables S1-S2). To validate the profile data, we chose the five upregulated miRNAs and the five downregulated miRNAs according to the fold change ranging from 10 to 20 (Table S3) and performed real-time reverse transcription polymerase chain reaction (RT-PCR) analysis in cells treated with PRRX1. As shown in Figure 5C, the expression of 10 miRNAs mentioned above was significantly regulated by PRRX1, and the expression trends of the selected miRNAs in PRRX1-overexpressed cells were consistent with the miRNA sequencing results.
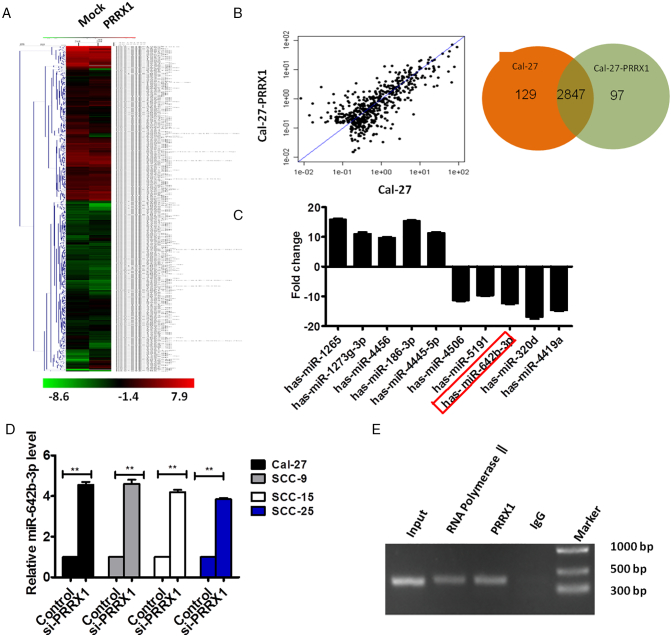
Effect of PRRX1 on the expression of miRNA in HNSCC cells. (A and B) Heatmap diagram of differential miRNA expression between with PRRX1 overexpression and mock in Cal-27 cells. Expression data were obtained using Feature Extraction software (version10.7.1.1, Agilent Technologies) and standardized by Genespring software (version 12.5; Agilent Technologies). Expression values shown were mean centered. Using a two-fold change and P < .05 as the threshold cutoff, 97 miRNAs were upregulated and 129 miRNAs were downregulated in PRRX1 overexpression cells. (C) Expression levels of miRNAs in the control and PRRX1-overexpressed cells by RT-PCR. The expression trends of the 10 miRNAs in PRRX1-overexpressed cells were consistent with the miRNA sequencing results. Error bars represent the mean ± SD of triplicate experiments. (D) RT-PCR analysis examined the expression of miR-642b-3p in Cal-27, SCC-9, SCC-15, and SCC-25 cells with PRRX1 knocked down. The data showed that miR-642b-3p levels were significantly increased by PRRX1 knockdown. Error bars represent the mean ± SD of triplicate experiments (** P < .001). (E) ChIP assay was performed with the miR-642b-3p promoter fragment containing the HRE. Input DNA that was not enriched by immunoprecipitation was amplified as a positive control. The assay demonstrated the direct binding of PRRX1 to the HRE region of miR-642b-3p in HNSCC cells.
We focused on miR-642b-3p for further study because it was significantly decreased in PRRX1-overexpressed HNSCC cells and was expressed at quite low levels. And studies have shown that miR-642b-3p is associated with tumorigenesis and cell growth [19], [20]. Nevertheless, the expression of miR-642b-3p in HNSCC tissues and cells, as well as the mechanisms underlying miR-642b-3p silencing in HNSCC, remains unknown. To study whether miR-642b-3p expression was PRRX1 dependent, PRRX1 was stably knocked down in HNSCC cells by lentiviral shRNAs. The level of miR-642b-3p was significantly increased by PRRX1 knockdown, suggesting that miR-642b-3p expression is dependent on PRRX1 in HNSCC cells (Figure 5D).
Then, we performed chromatin immunoprecipitation (ChIP) assays to verify whether PRRX1 directly binds to the predicted HRE region of miR-642b-3p. PCR products corresponding to the miR-642b-3p HRE-containing promoter region were detected in PRRX1-overexpressed HNSCC cells, showing that the combination capacity of PRRX1 and miR-642b-3p promoter was significantly increased (Figure 5E). And input DNA that was not enriched by immunoprecipitation was amplified as a positive control. These results confirmed the direct binding of PRRX1 to the HRE region of miR-642b-3p in HNSCC cells.
miR-642b-3p Mediated PRRX1-Induced Cell Dormancy and EMT of HNSCC Cells
To study the function of miR-642b-3p, we overexpressed miR-642b-3p in Cal-27 cells and SCC-9 cells using the mimics. The mimics resulted in a 3.18-fold increase of miR-642b-3p in cells by qRT-PCR. As shown in Figure 6A and B, the overexpression of miR-642b-3p significantly reduced the migration and invasion abilities of target cells compared with the control. The apoptosis and proliferation of cells treated with miR-642b-3p mimics were significantly increased compared with the control (Figure 6C and D).
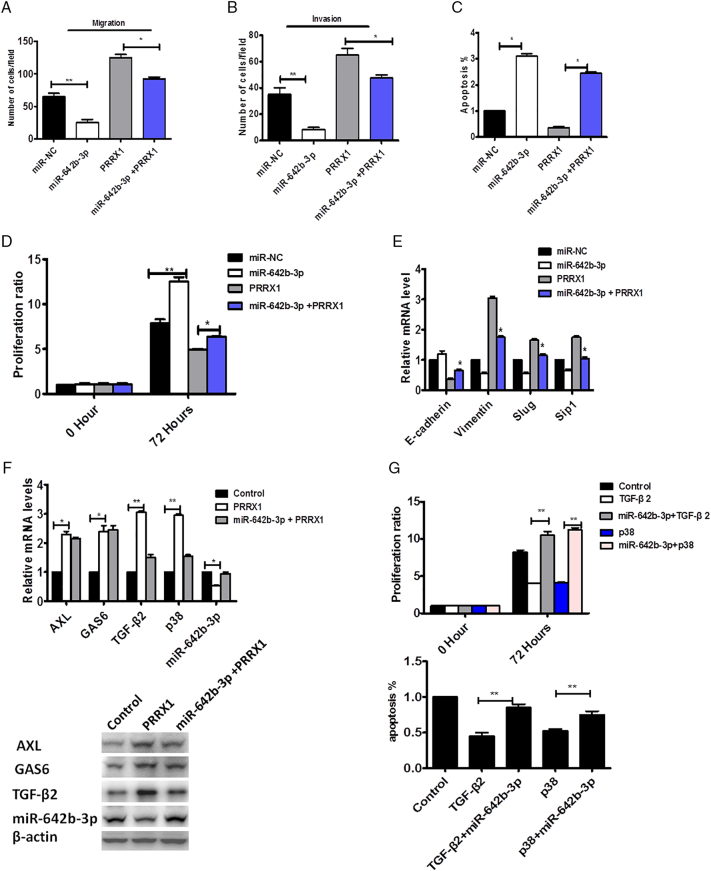
miR-642b-3p restoration inhibited PRRX1-induced proliferation and EMT of HNSCC cells. Migration (A) and invasion (B) assay of Cal-27 cells untreated or treated with miR-NC or miR-642b-3p or miR-642b-3p and PRRX1 for 48 hours. The mean was derived from cell counts of five fields, and each experiment was repeated three times (*P < .05, **P < .01). (C) The apoptosis of Cal-27 cells untreated or treated with miR-NC or miR-642b-3p or miR-642b-3p and PRRX1. Each experiment was repeated three times (*P < .05). (D) The proliferation of Cal-27 cells untreated or treated with miR-NC or miR-642b-3p or miR-642b-3p and PRRX1 for 48 hours. Each experiment was repeated three times (*P < .05, **P < .01). (E) RT-PCR analysis examined the expression of E-cadherin, vimentin, Slug, and Sip1. The data showed that miR-642b-3p markedly decreased vimentin, Slug, and Sip1 expression, while it significantly enhanced E-cadherin levels in PRRX1-overexpressed Cal-27 cells. Error bars represent the mean ± SD of triplicate experiments (*P < .05). (F) RT-PCR and Western blot examined the expression of p38, AXL, GAS6, and TGF-β2 activities in HNSCC cells with PRRX1-overexpressed group and PRRX1 + miR-642b-3p–overexpressed group. The data showed that TGF-β2, p38, AXL, and GAS6 expression was significantly boosted and miR-642b-3p was downregulated in PRRX1-overexpressed cells. And TGF-β2 and p38 were significantly reduced, while AXL and GAS6 expression had no alteration, in the PRRX1 and miR-642b-3p–overexpressed group. Each experiment was repeated three times (*P < .05, **P < .01). (G) SCC-25 cell proliferation and apoptosis in TGF-β2 overexpression, p38 overexpression, TGF-β2+ miR-642b-3p mimic, and p38+ miR-642b-3p mimic group, respectively, based on the MTT assay and FACS analysis, respectively. TGF-β2 and p38 overexpression reduced the apoptosis and proliferation of cells, and the miR-642b-3p mimic can restore the apoptosis and proliferation of cells. Error bars represent the mean ± SD of triplicate experiments (**P < .01).
To further study whether PRRX1-induced dormancy and the migration and invasion were dependent on miR-642b-3p, miR-642b-3p was overexpressed in PRRX1-overexpressed cells. The restoration of miR-642b-3p levels inhibited HNSCC cell proliferation and apoptosis (Figure 6C and D) and increased the migration and invasion of cells (Figure 6A and B). Moreover, we found that miR-642b-3p markedly decreased the expression of vimentin, Slug and Sip1 while significantly enhanced E-cadherin level in PRRX1-overexpressed cells (Figure 6E). The same data were obtained in SCC-15 and SCC-25 cells (Supplementary Figure S5). These results suggest that PRRX1-induced cell dormancy, and migration and invasion are dependent on the decrease of miR-642b-3p, which inhibited EMT of the target cells.
miR-642b-3p Inhibited Cell Dormancy Through TGF-β2 and p38 in HNSCC Cells
It was reported that upregulation of TGF-β2 can induce cancer cell dormancy and TGF-β2–induced cancer cell dormancy requires p38, AXL, and GAS6 [21], [22]. Here, we examined the role of p38, AXL, GAS6, and TGF-β2 in PRRX1-induced dormancy of HNSCC cells. We found that TGF-β2, p38, AXL, and GAS6 expression was significantly boosted and miR-642b-3p was downregulated in PRRX1-overexpressed HNSCC cells (Figure 6F). And we overexpressed miR-642b-3p in PRRX1-overexpressed HNSCC cells and found that TGF-β2 and p38 were significantly reduced, while AXL and GAS6 expression had no alteration (Figure 6F). This indicated that the effect of miR-642b-3p on the PRRX1-induced dormancy might mainly be dependent on TGF-β2 and p38. Subsequently, we analyzed the role of TGF-β2 and p38 on HNSCC cells treated by miR-642b-3p. As shown in Figure 6G, miR-642b-3p mimic can restore the apoptosis and proliferation of cells, which were significantly damped by TGF-β2 and p38 overexpression. This indicated that TGF-β2 and p38 were involved in the effect of miR-642b-3p on the PRRX1-induced dormancy.
PRRX1 Overexpression Induced Tumor Dormancy and EMT in an Xenograft Model
To further examine the role of PRRX1 in tumorigenesis, PRRX1 overexpression and vector cells were injected subcutaneously into nu/nu mice, respectively. As shown in Figure 7A, tumor volume in PRRX1-overexpressed group of the xenograft nude mice model was smaller than the vector 6 weeks after subcutaneously injecting Cal-27 cells (P < .01, Figure 7A and B). This suggests that PRRX1 might inhibit tumor growth and induce tumor dormancy of the primary HNSCC. Immunohistochemistrical staining showed the positive expression of PRRX1 in the xenograft of PRRX1-overexpression group and the negative expression in the vector (Figure 7C). Further, the data showed that there were strong positive expression of PRRX1, TGF-β2, and p38 and weak positive expression of E-cadherin and miR-642b-3p in PRRX1-overexpression group, consistent with the in vitro analysis (Figure 7D and E). Together, the data suggested that PRRX1 overexpression contributed to tumor dormancy and EMT in the xenograft model, which involved miR-642b-3p.
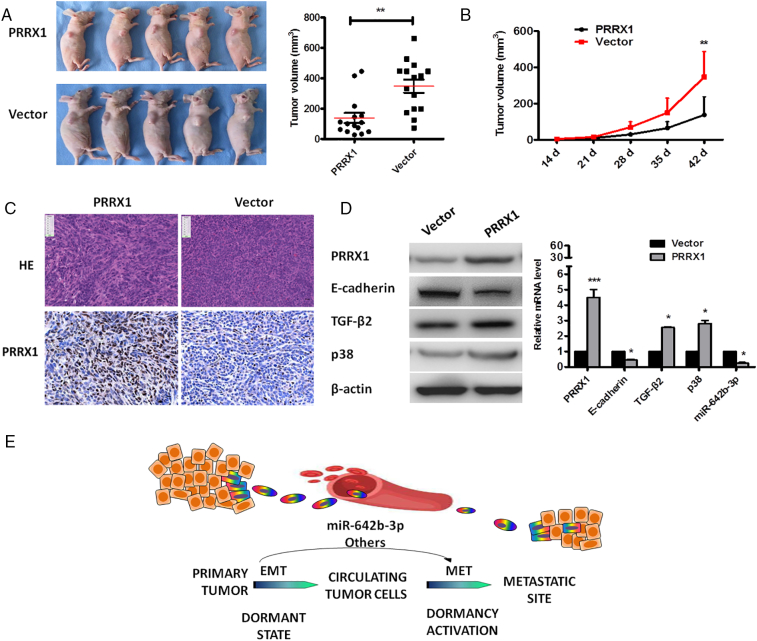
PRRX1 overexpression inhibited tumor growth and induced EMT in an xenograft model. (A) Imaging of primary tumor volume at day 42 between PRRX1-overexpression and vector cell groups injected subcutaneously into nu/nu mice. Quantitative data were on the right. The data showed that PRRX1 inhibited the growth of the tumor (**P < .01). (B) Growth curve of primary tumor for PRRX1 overexpression and vector cell groups. The data showed that tumor growth in PRRX1-overexpression group was significantly slower than the vector group (**P < .01). (C) HE and immunohistochemical staining of primary tumors showed that tumors in the vector group exhibited an epithelial morphology, whereas the tumors in PRRX1-overexpression group had a more spindle-cell morphology (×100). There was the strong expression of PRRX1 in PRRX1-overexpression group, while there was weak expression of PRRX1 in vector group. Scale bar, 100 mm. (D) Western blot examined the expression of E-cadherin, PRRX1, TGF-β2, and p38. The data showed that E-cadherin was downregulated and PRRX1, TGF-β2 and p38 were upregulated in PRRX1-overexpressed cells compared with the vector. (E) RT-PCR analysis examined the expression of E-cadherin, PRRX1, TGF-β2, p38, and miR-642b-3p. The data showed that, compared with the vector, PRRX1-overexpressed cells exhibited a significant downregulation of E-cadherin and miR-642b-3p, and upregulation of PRRX1, TGF-β2, and p38. Error bars represent the mean ± SD of triplicate experiments. (*P < .05, ***P < .001). (G) Schematic figure illustrated that EMT positive cancer cells may enter into dormant state and they need to undergo MET to activate dormancy through the control of miRNAs.
Discussion
Tumor dormancy was first defined by Willis [23] and then redefined by Hadfield [24] as a temporary mitotic and growth arrest. The mitotic arrest precisely refers to a G0-G1 arrest in certain cancer cells [11]. The growth arrest means the equilibrium between cell division and apoptosis. In this study, we initially showed that PRRX1 was involved in the cellular phenotype plasticity and tumor dormancy at the invasive front of HNSCC patients. Then, PRRX1 was proved to be an EMT inducer, and ectopic overexpression of PRRX1 in HNSCC cells was sufficient to enhance cell migration and invasion, along with the dormant property of cancer cells defined by low levels of PCNA and TUNEL negative staining. Finally, we explored the miRNA expression profiles of PRRX1-overexpressed HNSCC cells and the control cells using microRNA microarray. We found that PRRX1 reduced the level of miR-642b-3p, which mediated PRRX1-induced cell dormancy and EMT of HNSCC cells through TGF-β2 and p38. And we demonstrated that PRRX1 expression was correlated with miR-642b-3p, TGF-β2, and p38 in a xenograft model of HNSCC. We therefore suggest that PRRX1 can regulate the transition of EMT and MET, and dormant state of cancer cells through miR-642b-3p.This is the first study, to our knowledge, suggesting that EMT positive cancer cells may enter into dormant state and they need to undergo MET to activate dormancy through the control of miRNAs (Figure 7G).
PRRX1, one of the members of the homeobox protein family, is mainly expressed in the nucleus and plays a critical role in transcriptional activation and inducing downstream gene expression [25]. PRRX1 has been reported to be closely related to the tumor invasion and metastasis of EMT process in different parts or different pathological types of human carcinoma [26], [27]. In this study, HE staining in the primary tumor and lymph nodes of HNSCC samples showed that the growth and progression of HNSCC had both an initial dedifferentiation and a subsequent epithelial redifferentiation, indicating that HNSCC cells had the cellular phenotype plasticity. During this process, PRRX1 was the positive expression in the majority of cancer cells at the invasive front, and PRRX1 was the negative expression of the majority of cancer cells in the lymph node of HNSCC, suggesting that PRRX1 might be a driving force for the highly regulated morphogenetic process of HNSCC. Then, we investigated the role of PRRX1 in cancer cells in vitro and the mice tumor tissue in vivo to verify this speculation. We found that exogenous PRRX1 expression induced EMT process and increased migration and invasion of cancer cells. During the process of EMT, the levels of epithelial junction proteins such as E-cadherin decreased, and the expression of mesenchymal proteins such as N-cadherin and vimentin increased, accompanying the increase of EMT transcription factors Slug and Sip1. And the loss of PRRX1 reverted EMT to MET in HNSCC cells. These were in line with the previous reports. Ocaña et al. [16] reported that PRRX1, a newly identified EMT inducer, promoted full EMT in cancer cells. The loss of PRRX1 was required for cancer metastasis. Downregulation of PRRX1 caused the acquisition of MET. In gastric carcinoma, the high expression of PRRX1 promoted the EMT process through mediating Wnt/beta-catenin signaling [28]. These indicated that PRRX1 is a new EMT inducer of HNSCC and promotes tumor migration and invasion, and PRRX1 may be one of the main driving forces for the cellular phenotype plasticity of HNSCC.
Filippo G. put forward the hypotheses about the relationship between EMT, MET, and metastasis and thought that EMT-positive cells may enter into dormant state and they need to undergo MET process to regain proliferation [8]. These indicated that cancer cell dormancy plays an important role in EMT, MET, and metastasis. Hence, we further investigated the role of PRRX1 in tumor dormancy. First, we found that PRRX1-induced EMT reduced cell proliferation and apoptosis of cancer cells. This is supported by the data of Park et al., who found that EMT transcription factor Snail could suppress tumor cell proliferation through binding to flanking region of PCNA gene to decrease its expression [29]. Zeb facilitates a growth arrest by targeting the expression of cyclin D1. And Zeb-1 and Snail induce G1 arrest by promoting hypophosphorylation of RB and decreasing the expression of cyclin D1 [30]. The proliferation of tumor cells of squamous cell carcinoma metastastasis is suppressed by activating Twist1 [31]. These suggested that quiescence is a general characteristic of tumor cells that have undergone EMT [30].
Further, we found that the loss of PRRX1 reverted EMT to MET in HNSCC cells and knockdown of PRRX1 induced part of cancer cells to proliferate and resurge dormancy. Barkan et al. [32] reported that dormant D2.0R cells express plenty of E-cadherin, indicating that these cells have undergo MET, and the expression of Twist activated metastatic outgrowth in these dormant cells. On the other side, the hepatic nonparenchymal cells in metastatic hepatic niche can induce EMT and initiate outgrowth from metastatic dormancy by EGFR partly [33]. This indicated that the MET program might rebuild the connection of tumor cells and induce part of cancer cells, not all the cancer cells, to activate dormancy [34].
miRNAs, a class of small molecules, are involved in regulating diverse biological processes, including development, differentiation, metabolism, cancer, and viral infection. In Kaposi's sarcoma, a number of herpesviral miRNAs, expressed during the latency phase of viral lifecycle, have been shown to inhibit lytic replication of herpes simplex virus and human cytomegalovirus and promote latency by directly targeting the viral immediate-early genes [35]. In breast cancer, miRNAs can be transferred from cell to cell through gap junctional intercellular communication to regulate the function of stem cell and dormancy [36], [37]. Almog et al. [38] firstly reported on the intricate involvement of a single miRNA acting as a master regulator of human tumor dormancy and found that overexpression of miR-190 in otherwise fast-growing tumors resulted in inhibition of tumor progression and induction of dormancy. Then, miR-138 or miR-346 have been shown to efficiently induce lung colonization in the majority of mice (3/5), indicating that these microRNAs promote exit from dormancy in the lung [39]. Our results showed that miR-642b-3p had quite lower levels among the differentially expressed miRNAs in PRRX1-overexpressed HNSCC cells and the control cells. miR-642b-3p overexpression in HNSCC cells resulted in a significant inhibition of tumor progression. A possible explanation to the transient effect of miR-642b-3p expression on tumor growth patterns could be that miR-642b-3p expression in tumor cells induces cellular stress (death or quiescence). This could lead to the selection of cells that lost or repressed miR-642b-3p expression or, alternatively, upregulated compensatory mechanisms that circumvent miR-642b-3p effects. This supports the hypothesis that miR-642b-3p was lost or repressed in tumor dormant cells.
Then, we further investigated the function of miR-642b-3p in PRRX1 modulation of cancer progression. We found that the restoration of miR-642b-3p levels in PRRX1-overexpressed cells inhibited HNSCC cell dormancy, migration, and invasion through TGF-β2 and p38. Moreover, miR-642b-3p markedly decreased N-cadherin expression while significantly enhancing E-cadherin levels. The result of ChIP assay further showed that PRRX1-induced dormancy and the migration and invasion are dependent on miR-642b-3p. Knowledge on the functional roles of miR-642b-3p in cancer dormancy and EMT is currently limited. However, a recent study showed that the expression of miR-642b-3p was overall higher in breast cancer patients with stage I, II, and III compared to stage IV, with potential utilization for early detection [20]. The levels of three miRNAs (miR-22-3p, miR-642b-3p, and miR-885-5p) and CA19-9 were significantly higher in pancreatic cancer patients, even those with early-stage disease (IB and IIB), than in healthy control [19]. In addition, Yamaguchi et al. [40] generated sunitinib-resistant renal cell carcinoma cell lines, identified miRNAs contributing to sunitinib resistance, and found that expression of miR-642b-3p was significantly increased and may contribute to sunitinib resistance in human cancers. These results, along with ours, suggest that miR-642b-3p is involved in tumor dormancy and is important for tumor growth and chemotherapy resistance.
In conclusion, we demonstrated that PRRX1 is upregulated in primary tumor and downregulated in the lymph nodes and such switch process is accompanied by the cellular phenotype plasticity. The high level of PRRX1 promotes the cell dormancy, EMT, and migration and invasion of HNSCC cells, in which miRNAs, especially miR-642b-3p, play an important role. Our findings define an important function for PRRX1 in HNSCC progression by orchestrating the cellular phenotype plasticity and tumor dormancy through miRNAs and suggest that there are some dormant cancer cells with EMT character that reside in HNSCC patients, and these cells may be responsible for lymph node metastasis and prognosis. However, how do mesenchymal cancer cells diminish the expression of PRRX1 in metastatic site in order to begin MET activity? What is the switch or sensor of the plasticity? Much should be further done.
Conclusion
This study demonstrated that the high level of PRRX1 promoted the cell dormancy, EMT, and migration and invasion of HNSCC cells, in which miRNAs, especially miR-642b-3p, played an important role. So, PRRX1 might be a useful predictor to identify those HNSCC patients who are at high risk of cancer dormancy, migration, and invasion in preoperative setting to further investigate the effect of chemotherapy in patients with PRRX1-positive tumors.
Material and Methods
Clinical Samples Collection and Study
Paraffin-embedded sections of 89 HNSCC patients and 60 metastatic lymph nodes of HNSCC patients, who underwent resection of their tumors without preoperative chemotherapy, hormone therapy, or radiotherapy, were obtained from the Department of Oral and Maxillofacial Surgery, West China Hospital of Stomatology, Sichuan University, between 2006 and 2010. All the sections were histologically confirmed in a blinded manner by two pathologists. The protocol of the study was approved by the Institutional Ethics Committee of the West China Medical Center, Sichuan University, China.
Immunohistochemistry
Paraffin-embedded sections were cut and stained using a conventional immunohistochemistry procedure.
Real-Time RT-PCR
Total RNA was extracted from cells using the trizol (Invitrogen, Carlsbad, CA). The quantity and quality of total RNA were quantified with the NanoDrop ND-1000 Spectrophotometer (Thermo Scientific Inc., Waltham, MA) via measurement of the absorbance of the RNA sample at a wavelength of 260 and 280 nm. RNA (1 μg) was reverse transcribed to cDNA using the SuperScript III First-Strand Synthesis System (Invitrogen, Carlsbad, CA) with random hexamers according to the manufacturer's protocol.The resulting cDNA was diluted and used as a template for real-time PCR using LightCycler (Roche Diagnostics GmbH, Mannheim, Germany). The primers of genes were list in Supplementary Table S4.
Western Blot
Total proteins were isolated from the cultured cells with a total protein extraction kit (Keygen, Nanjing, China), and protein concentrations were detected by a bicinchoninic acid protein assay kit (Pierce, Rockford, IL). The procedure of Western blot was done according to the manufacturer's instructions.
Immunofluorescence
Cells were cultured on Lab-Tek Chambered Coverglass (Thermo Fisher Scientific, Rochester, NY) at a density of 3×104 cells per chamber. Upon reaching 70% confluency, culture medium was removed; cells were washed and fixed with 4% paraformaldehyde and permeabilized with 0.1% Triton X-100 in PBS. To avoid nonspecific binding of antibody, cells were blocked with 1% bovine serum albumin prepared in PBS. Lastly, cells were incubated with PRRX1 antibody (1:250 dilution), twist antibody (1:100 dilution), E-cad (1:100 dilution), vimentin (1:100 dilution), and Alexa Fluor 488–conjugated secondary donkey anti-rabbit antibody (1:1000 dilution). Cells were visualized using the Olympus Fluoview confocal microscope (Tokyo, Japan), and confocal fluorescence images were taken.
In Vitro Cell Migration and Invasion Assay
Migration of cells was assessed using noncoated membrane Transwells (24-well inserts; pore size, 8 μm; BD Biosciences). About 5×104 cells suspended in serum free medium were plated in the top chamber, and medium supplemented with serum was used as a chemoattractant in the lower chamber. The invasion assay was conducted as described for migration assay using 1.5×105 cells and Matrigel-coated membrane (24-well insert, pore size, 8 μm; BD Biosciences). After 24 hours of incubation, cells remaining on top of the membrane and not migrating were removed using a cotton swab. Migrated cells on the lower surface of the membrane were stained with 0.1% Crystal Violet; five fields per filter were counted.
Cell Proliferation Assays
Cell growth rates were assessed by the MTT assay. Cells were seeded onto 96-well plates in triplicate. After incubation for indicated time, 3-(4,5-dimethylthiazol-2-yl)-2,5-diphenyltetrazolium bromide solution was added to each well and incubated for 4 hours at 37°C before removal of the culture medium. DMSO was then added and shaken for 30 minutes at room temperature. Cell viability was determined by measuring the absorbance at 490 nm. The experiments were performed at least three times.
Apoptosis Detection by FCM
Apoptotic cells were differentiated from viable or necrotic ones by combined application of Annexin V–FITC and propidium iodide (BD Biosciences Clontech, USA). The samples were washed twice and adjusted to a concentration of 1 × 106 cells/ml with 4°C PBS. The Falcon tubes (12 mm × 75 mm, polystyrene round-bottom) were used in this experiment; 100 μl of suspensions was added to each labeled tube; 10 μl of Annexin V–FITC and 10 μl propidium iodide (20 μg/ml) were added into the labeled tube, which was incubated for at least 20 minutes at room temperature in the dark; and then 400 μl of PBS binding buffer was added to each tube without washing, which was analyzed using FCM analysis (BD Biosciences Clontech, USA) as soon as possible.
Cloning, Lentivirus Preparation, and Plasmids
The full-length cDNA of PRRX1 was cloned into the pLV.Des3d.P/Puro plasmid vector and transfected into cells by LipofectAMINE reagent (Invitrogen) according to the manufacturer's instructions. Stable transfected cells were selected in puromycin and further subcloned. Human PRRX1 NM_006902.3, 654 bp:ATGACCTCCAGCTACGGGCACGTTCTGGAGCGGCAACCGGCGCTGGGCGGCCGCTTGGACAGCCCGGGCAACCTCGACACCCTGCAGGCGAAAAAGAACTTCTCCGTCAGTCACCTGCTAGACCTGGAGGAAGCCGGGGACATGGTGGCGGCACAGGCGGATGAGAACGTGGGCGAGGCTGGCCGGAGCCTGCTGGAGTCGCCGGGACTCACCAGCGGCAGCGACACCCCGCAGCAGGACAATGACCAGCTGAACTCAGAAGAAAAAAAGAAGAGAAAGCAGCGAAGGAATAGGACAACCTTCAATAGCAGCCAGCTGCAGGCTTTGGAGCGTGTCTTTGAGCGGACACACTATCCTGATGCTTTTGTGCGAGAAGACCTTGCCCGCCGGGTGAACCTCACCGAGGCGAGAGTGCAGGTGTGGTTTCAGAACCGAAGAGCCAAGTTCCGCAGGAATGAGAGAGCCATGCTAGCCAATAAAAACGCTTCCCTCCTCAAATCCTACTCAGGAGACGTGACTGCTGTGGAGCAGCCCATCGTACCTCGTCCTGCTCCGAGACCCACCGATTATCTCTCCTGGGGGACAGCGTCTCCGTACAGATCCTCGTCCCTCCCAAGATGTTGTTTACACGAGGGGCTTCATAACGGATTCTAA.
miRNA Microarray and Data Analysis
Total RNA from cells was isolated using TRIzol reagent (Invitrogen, Carlsbad, CA) following the manufacturer's instructions. Two milligrams of total RNA from each sample was labeled using the miRCURYTM Hy3TM/ Hy5TM labeling kit and hybridized on the miRCURYTM LNA Array (v. 8.0) (Exiqon, Vedbaek, Denmark). Signal intensities were normalized using the global Lowess regression algorithm. For subsequent analysis, we used the log2 of the background-subtracted, normalized median spot intensities of ratios from the two channels (Hy3/Hy5). To find consistently differentially expressed genes, the data were subjected to SAM analysis.
miRNA Expression Analysis
Total RNA was isolated from cell lines using TRIzol (Invitrogen). Reverse transcription was performed using miScript Reverse Transcription Kit (Qiagen), and real-time PCR was performed in triplicate on CFX96 Real-Time PCR Detection System (Bio-Rad) using the SYBR Premix Ex Taq (Takara). The 2−ΔΔCT method was used to determine the relative gene expression, with miRNA levels normalized to U6 small nuclear RNA.
Xenografts
The tumor cells were subcutaneously injected into the flank of 6-week-old BALB/c nu/nu mice and examined every 5 to 7 days for tumor appearance. Tumor growth was measured once weekly until 42 days after inoculation by determining the tumor volumes using the formula (length × width2)/2. All animal experiments were approved by the Animal Care Committee of Sichuan University, China.
Statistical Analyses
All statistical analyses were done using the SPSS package (version 13.0). A value of P < .05 was considered statistically significant.
The following are the supplementary data related to this article.
Supplementary tables
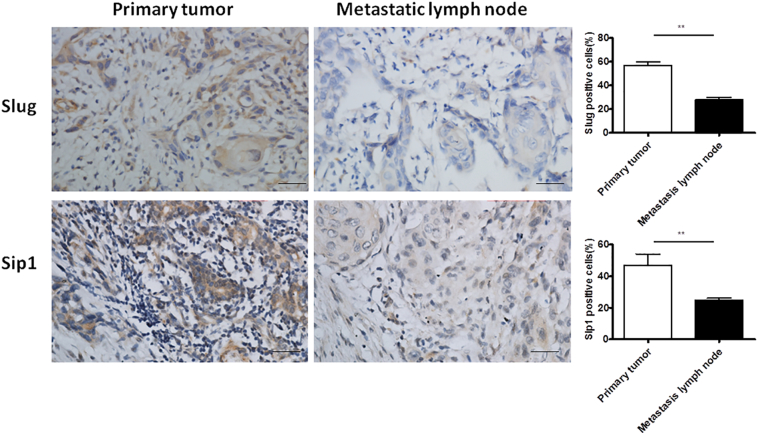
Expression of Slug and Sip1 at the invasive front of the primary tumor and the metastatic lymph node in HNSCC patients. Immunohistochemistrical staining of Slug and Sip1 of cancer cells in HNSCC patients. Most of the cytoplasm of cancer cells at the invasive front of the primary tumor showed strong positive expression of Slug and Sip1, while the cytoplasm of cancer cells in the metastatic lymph node had weak positive expression of Slug and Sip1. Scale bar, 50 μm. The proportions of the positive cells of Slug and Sip1 were calculated, respectively. Student paired t test was used to analyze the differences between the primary tumor and metastatic lymph node (**P<.01).
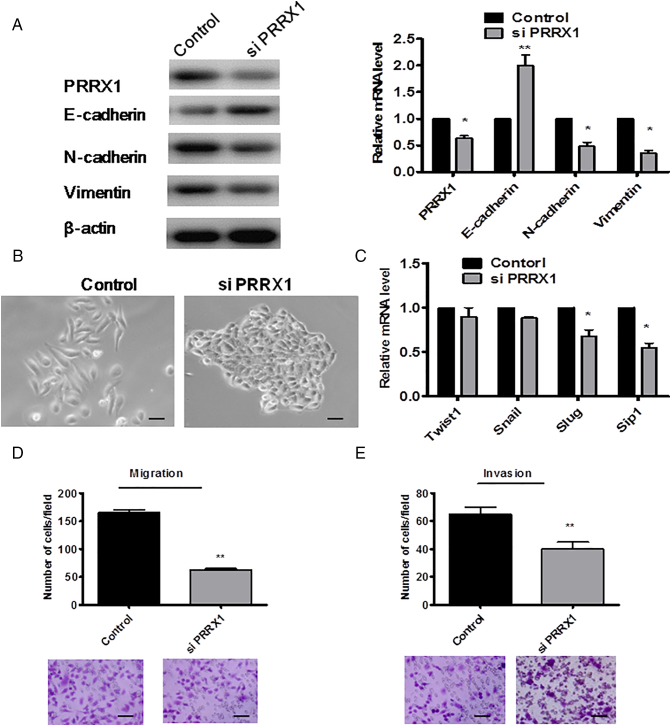
PRRX1 silencing reverts EMT in SCC-9 cells concomitant with the resurgement of cell dormancy. (A) Western blot (left panel) and real-time RT-PCR (right panel) analyses of the control and PRRX1 silencing cells showed the activation of E-cadherin and the repression of N-cadherin and Vimentin following PRRX1 knockdown. Error bars represent the mean ± SD of triplicate experiments (* P<.05, **P<.01). (B) Phase-contrast images showing the phenotype of control-transfected cells of PRRX1 overexpression cell and of those in which PRRX1 has been decreased expressed. Scale bar, 100 mm. (C) Real-time RT-PCR assay was performed to detect the mRNA levels of Twist1, Snail, Slug, and Sip1. Error bars represent the mean ± SD of triplicate experiments (*P<.05). Migration assay (D) and invasion assay (E) were conducted to measure the migratory and invasive potentials of PRRX1 knockdown cells. Representative images of migrated and invaded cells were shown. Error bars represent the mean ± SD of triplicate experiments (**P<.01).
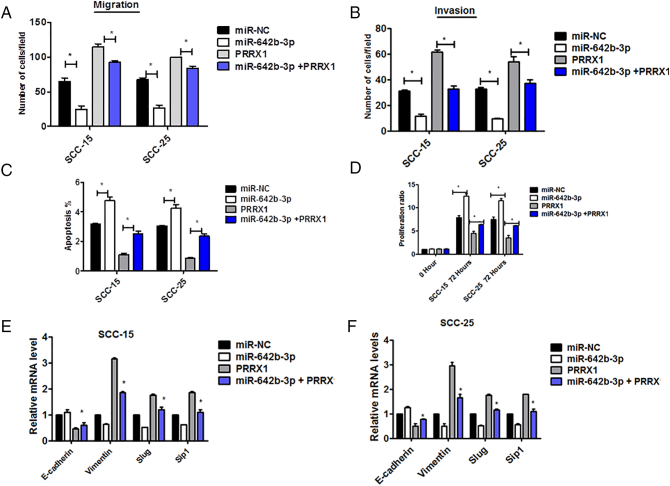
miR-642b-3p restoration inhibited PRRX1-induced proliferation and EMT of SCC-15 and SCC-25 cells. Migration (A) and invasion (B) assays of SCC-15 and SCC-25 cells untreated or treated with miR-NC or miR-642b-3p or miR-642b-3p and PRRX1 for 48 hours. The mean was derived from cell counts of five fields, and each experiment was repeated three times (*P<.05). (C) The apoptosis of SCC-15 and SCC-25 cells untreated or treated with miR-NC or miR-642b-3p or miR-642b-3p and PRRX1. Each experiment was repeated three times (*P<.05). (D) The proliferation of SCC-15 and SCC-25 cells untreated or treated with miR-NC or miR-642b-3p or miR-642b-3p and PRRX1 for 48 hours. Each experiment was repeated three times (*P<.05). (E) RT-PCR analysis examined the expression of E-cadherin, vimentin, Slug, and Sip1. The data showed that miR-642b-3p markedly decreased vimentin, Slug, and Sip1 expression, while it significantly enhanced E-cadherin levels in PRRX1-overexpressed SCC-15 and SCC-25 cells. Error bars represent the mean±SD of triplicate experiments (*P<.05).
Declarations
Ethics Statement
The human specimens in this study were reviewed and approved by the Institutional Ethics Committee of the West China Medical Center, Sichuan University, China (No. WCHSIRB-D-2013-179). Every patient signed separate informed consent forms for sampling and molecular analysis. The animal study was approved by the Animal Care and Use Committee of the West China Medical Center, Sichuan University, China (No. SKLODLL2013A184).
Consent for Publication
Not applicable.
Availability of Data and Material
The datasets generated and/or analyzed during the current study are available in the [PUBMED] repository, [PERSISTENT WEB LINK TO DATASETS].
Funding
This work was supported by National Natural Science Foundation of China grants (nos. 81672672, 81502357, 81572650, 81772891, and 81372891), by National Program on Key Research Project of China (2016YFC0902700), by Natural Science Foundation of Zhejiang Province (Q142114001), and by the Fundamental Research Funds for the Central Universities (2017).
Authors' Contributions
X. H. L. and Y. L. T. designed the study and wrote the paper. J. J., Min Z., and Mei Z. performed the experiments. X. Y., L. L., S. S. W., J. S. W., X. H. Y., J. B. W., X. P., and Y. J. T. analyzed data and drafted the manuscript. J. J. collected samples. Min Z. and Mei Z. analyzed data and revised the manuscript. X. H. L. and Y. L. T. designed and supervised the studies and wrote the paper.
Footnotes
1Conflict of Interest: The authors declare no conflicts of interest.
2Acknowledgements: This work was supported by National Natural Science Foundation of China grants (Nos. 81672672, 81502357, 81572650, 81772891 and 81372891), by National Program on Key Research Project of China (2016YFC0902700), by Natural Science Foundation of Zhejiang Province (Q142114001), and by the Fundamental Research Funds for the Central Universities (2017).
References
Articles from Neoplasia (New York, N.Y.) are provided here courtesy of Neoplasia Press
Citations & impact
Impact metrics
Citations of article over time
Alternative metrics
Smart citations by scite.ai
Explore citation contexts and check if this article has been
supported or disputed.
https://scite.ai/reports/10.1016/j.neo.2018.12.001
Article citations
PRRX1 silencing is required for metastatic outgrowth in melanoma and is an independent prognostic of reduced survival in patients.
Mol Oncol, 18(10):2471-2494, 08 Jul 2024
Cited by: 0 articles | PMID: 38978350 | PMCID: PMC11459042
Transcription Factors and Markers Related to Epithelial-Mesenchymal Transition and Their Role in Resistance to Therapies in Head and Neck Cancers.
Cancers (Basel), 16(7):1354, 29 Mar 2024
Cited by: 3 articles | PMID: 38611032 | PMCID: PMC11010970
Review Free full text in Europe PMC
Circ_MAPK9 promotes STAT3 and LDHA expression by silencing miR-642b-3p and affects the progression of hepatocellular carcinoma.
Biol Direct, 19(1):4, 02 Jan 2024
Cited by: 1 article | PMID: 38163874 | PMCID: PMC10759731
Key processes in tumor metastasis and therapeutic strategies with nanocarriers: a review.
Mol Biol Rep, 51(1):197, 25 Jan 2024
Cited by: 0 articles | PMID: 38270746
Review
Paired-related homeobox 1 induces epithelial-mesenchymal transition in oesophageal squamous cancer.
World J Gastrointest Oncol, 15(12):2185-2196, 01 Dec 2023
Cited by: 0 articles | PMID: 38173429 | PMCID: PMC10758647
Go to all (33) article citations
Data
Data behind the article
This data has been text mined from the article, or deposited into data resources.
BioStudies: supplemental material and supporting data
Similar Articles
To arrive at the top five similar articles we use a word-weighted algorithm to compare words from the Title and Abstract of each citation.
microRNA-203 suppresses invasion and epithelial-mesenchymal transition induction via targeting NUAK1 in head and neck cancer.
Oncotarget, 7(7):8223-8239, 01 Feb 2016
Cited by: 38 articles | PMID: 26882562 | PMCID: PMC4884988
Targeting STAT3/miR-21 axis inhibits epithelial-mesenchymal transition via regulating CDK5 in head and neck squamous cell carcinoma.
Mol Cancer, 14:213, 21 Dec 2015
Cited by: 48 articles | PMID: 26690371 | PMCID: PMC4687320
Antitumor miR-150-5p and miR-150-3p inhibit cancer cell aggressiveness by targeting SPOCK1 in head and neck squamous cell carcinoma.
Auris Nasus Larynx, 45(4):854-865, 09 Dec 2017
Cited by: 32 articles | PMID: 29233721
Epithelial-to-mesenchymal transition and cancer stem(-like) cells in head and neck squamous cell carcinoma.
Cancer Lett, 338(1):47-56, 04 Jul 2012
Cited by: 65 articles | PMID: 22771535
Review
Funding
Funders who supported this work.
Fundamental Research Funds for the Central Universities
National Natural Science Foundation of China (5)
Grant ID: 81502357
Grant ID: 81372891
Grant ID: 81572650
Grant ID: 81672672
Grant ID: 81772891
National Program on Key Research Project of China (1)
Grant ID: 2016YFC0902700
Natural Science Foundation of Zhejiang Province (1)
Grant ID: Q142114001