Abstract
Free full text

Alpha-tocopherol: roles in prevention and therapy of human disease
Abstract
Alpha-tocopherol, one of the eight isoforms of vitamin E, is the most potent fat-soluble antioxidant known in nature. For years, it was thought that α-tocopherol only functioned as a scavenger of lipid peroxyl radicals, specifically, oxidized low-density lipoprotein (oxLDL), thereby serving as a chief antioxidant for the prevention of atherosclerosis. In recent years, the many roles of α-tocopherol have been uncovered, and include not only antioxidant functions, but also pro-oxidant, cell signaling and gene regulatory functions. Decades of clinical and preclinical studies have broadened our understanding of the antioxidant vitamin E and its utility in a number of chronic, oxidative stress-induced pathologies. The results of these studies have shown promising, albeit mixed reviews on the efficacy of α-tocopherol in the prevention and treatment of heart disease, cancer and Alzheimer’s disease. Future studies to uncover cellular and systemic mechanisms may help guide appropriate clinical treatment strategies using vitamin E across a diverse population of aging individuals.
1. Introduction
Vitamin E is the name given to a family of eight molecules, each consisting of a chromanol ring with an aliphatic side chain. They consist of two groups, tocopherols and tocotrienols, based on the side chain being saturated or unsaturated, respectively. Within with each group, there are four isoforms, α, β, γ, and δ named for specific methyl group substitutions at positions 5, 7 and 8 of the chromanol ring [1]. Tocopherols have three chiral centers, and the α-isoform is most commonly found in the 2R,4′R,8′R configuration. Although the β-, γ-, δ- are absorbed by humans through the intestine, they are not recognized by the liver protein, α-tocopherol transfer protein (α-TTP) and replenished in circulation like the α-isoform [2]. Also, α-tocopherol functions as the most potent naturally occurring scavenger of reactive oxygen and reactive nitrogen species (ROS and RNS), surpassed only by recently developed synthetic compounds [3].
Dietary vitamin E is obtained mainly from plant sources including sunflower seeds, olive oil and almonds, which contain high amounts of α-tocopherol, while most other oils and seed oils are rich in γ-tocopherol. Although the majority of western dietary vitamin E consists of γ-tocopherol, the α-isoform is predominant in vitamin E supplements [4] in the form of RRR-α-tocopherol or molar equivalent tocopherol esters. RRR-α-tocopherol supplementation is considered safe below the tolerable upper intake level of 1000 mg daily for adults, as established by the Institute of Medicine. However, some studies have shown that vitamin E supplementation is safe in clinical trials in amounts up to 1073 mg (1600 International Units (IU) [5]. These levels are far above the recommended vitamin E intake of 400 IU/day. Absorption of this vitamin occurs in the small intestine along with other fat-soluble nutrients. Pancreatic lipases and bile salts aid in the formation of micelles. The bioavailability of vitamin E is impacted by the levels of dietary fats as well as plant sterols that share similar digestion and absorption pathways [6,7].
The α-tocopherol isoform of vitamin E inhibits the radical chain propagation within lipid domains by its own conversion into an oxidized product, α-tocopheroxyl free radical [8,9] (Fig. 1). Alpha-tocopherol reacts with lipid peroxyl radicals at a rate several orders of magnitude faster than the peroxyl radical propagation reaction [4]. The original form can be regenerated by reduction by ascorbate (vitamin C) or ubiquinol-10 [1]. This regeneration enhances the antioxidant capacity of vitamin E and has led to the clinical investigation of vitamin C use in concert with vitamin E supplementation [4].
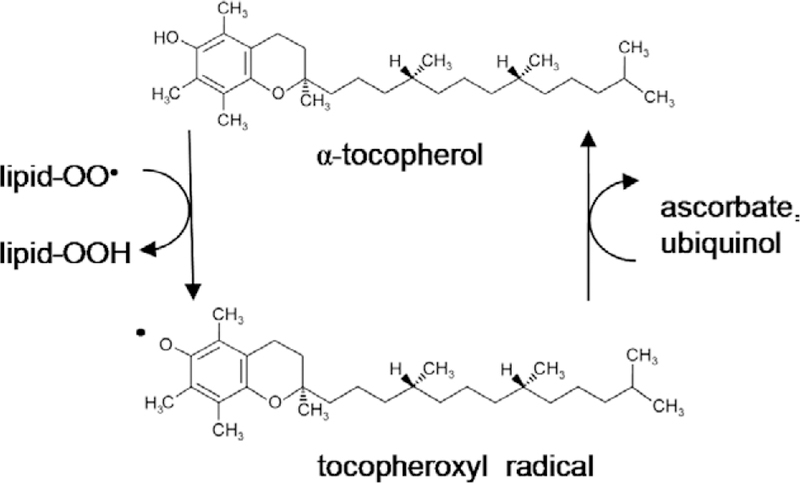
Structure of α-tocopherol form of vitamin E (top) before the 6-OH group hydrogen is donated to a lipid radical. This reaction forms a stable lipid hydroperoxide and a tocopheroxyl radical (bottom). The original state of α-tocopherol can be regenerated by a reduction reaction with hydroxyl groups from other antioxidants such as vitamin C (ascorbate) or ubiquinol.
2. Vitamin E uptake and transport
Vitamin E and other fat-soluble nutrients are emulsified by bile salts and pancreatic enzymes into micelle particles in the small intestine. All vitamin E isoforms are transported purportedly in a similar fashion as dietary cholesterol, via the selective cholesterol uptake activity of the Niemann Pick C1-like protein localized in the brush border membrane [10]. Once inside the enterocyte, cholesterol trafficking is controlled by the activity of ATP-binding cassette (ABC) transporters, a pathway potentially shared by vitamin E and its derivatives [11]. These transporters are controlled at the transcriptional level by liver X receptors, nuclear factors whose agonists include oxidized cholesterol and plant sterols [12]. Supporting the hypothesis that vitamin E is transported in a similar fashion as cholesterol is the observation that ABCA1 plays a role in the efflux of α-tocopherol in cultured macrophages and fibroblasts [13]. The transporters ABCA1 and ABCG1 enable cholesterol uptake into the lymphatic system [14,15] while ABCG5 and ABCG8 control the efflux of cholesterol into the intestinal lumen. The transcriptional activation of ABCG5 and ABCG8 by sterol-induced liver X receptors reduces the overall intestinal absorption of cholesterol [16]. Chylomicrons, containing all eight equally represented isoforms of vitamin E among other lipid soluble nutrients, are secreted from enterocytes into the lymph and are released into the circulation via the thoracic duct. Lipoprotein lipases, bound to the endothelium, hydrolyze triacylglycerols from the surface of the chylomicron particle, and subsequently, free fatty acids and monoacylglycerols are released as the particle becomes smaller from the loss of surface molecules [2]. During this process, some vitamin E is transferred to high-density lipoproteins (HDL) and the rest remain in the chylomicron until the particle is taken into hepatocytes via LDL receptor and LDL receptor-related protein-mediated endocytosis [17]. Several other factors may be involved in the uptake of vitamin E in HDL and particle remnants in the liver, including the scavenger receptor B type I (SR-BI) [18]. In hepatocytes, RRR α-tocopherol is selectively bound to the α-tocopherol transfer protein (α-TTP), a Sec14 protein family member expressed in the liver, brain and placenta [19,20], while other isoforms of vitamin E are excreted in the bile. Individuals that lack a functional α-TTP suffer from a neuro-degenerative disease called ataxia with vitamin E deficiency [21], suggesting a role for this protein in the retention and subsequent plasma dispersal of α-tocopherol. After binding to α-TTP, α-tocopherol is trafficked into late endosomes/lysosomes and then into very low-density lipoprotein (VLDL) particles at the plasma membrane; a process that is proposed to involve the Golgi apparatus and/or the ABCA1 transporter [13,22,23]. Excess α-tocopherol is secreted from the liver in the form of VLDL particles, which are broken down by lipoprotein lipases of peripheral cell endothelium and transferred into lipoprotein particles (HDL, LDL, VLDL remnants) and either taken up by the liver or delivered to cells via receptor-mediated and non-receptor mediated pathways [2].
3. Oxidative stress and human disease
Oxidative stress is a necessary phenomenon in growth, development and differentiation. Such processes as proliferation, wounding and aging incite the generation of lipid hydroperoxides from cell membrane phospholipids. These reactive lipids can function as signaling molecules and can induce apoptosis. The specific levels of ROS and RNS, respectively, within a cell can determine a number of processes including the activity of specific enzymes and proteases, changes in ion levels (such as Ca2+), the expression of genes and whether a cell lives (mitosis) or dies (apoptosis or necrosis) [24]. Several naturally occurring free radical scavengers, including beta-carotene, ascorbate and vitamin E, help maintain homeostatic levels of these reactive species by terminating a chain reaction of oxidizing events [1]. Survival enzymes such as glutathione peroxidase (GPx) and superoxide dismutase (SOD) are also responsible for the maintenance of oxidative homeostasis.
In situations of elevated oxidative stress, O2• can react with nitric oxide (NO) and produce peroxynitrite (ONOO–), a potent oxidant of proteins, lipids and DNA [25]. Chronic, unbridled oxidative damage has been implicated as the culprit behind many chronic diseases, including cancer, atherosclerosis, diabetes, Alzheimer’s [26–28] and other neurodegenerative diseases [26,29,30]. ROS, superoxide and hydrogen peroxide [31] play a key role as signaling molecules in the angiotensin II (AngII) system, a major signaling pathway for cardiovascular homeostasis. Alterations in AngII-mediated redox signaling have been observed in hypertension and atherosclerosis [32].
Modulation of enzyme transcription and/or activity by vitamin E has been shown in genes involved in oxidative stress, proliferation, inflammation and apoptosis. Such genes include, but are not limited to, SOD, NO synthase, cyclooxygenase-2, NAPDH oxidase, nuclear factor kappa B, phospholipase A2, protein phosphatase 2A, 5-lipooxygenase, activator protein-1, pregnane X receptor, and protein kinase C [4,33]. Data extracted from cellular and animal models have consistently shown that the gene regulatory effects of vitamin E reduce the levels of O2 and lipid peroxides, and diminish the number and severity of atherogenic events [33].
Excess levels of vitamin E present in the liver activate the pregnane X receptor, a transcription factor that may lead to the expression of drug resistance genes including cytochrome P450, glutathione S-transferase A2, multidrug resistance-associated protein 2 (ABCC2), and hydroxysteroid sulfotransferase (SULT2–40/41) [34–37]. During transport via plasma lipoproteins, vitamin E protects the carrier particle from free radical peroxidative damage. This is one of the major and well-characterized modes of α-tocopherol antioxidant functions. However, cellular and preclinical animal models have demonstrated the existence of several “nonantioxidant” functions of vitamin E.
The downstream effects of oxidative damage are associated with several human pathologies including atherosclerosis [peroxidation of lipids (oxLDL) in fatty streak formation in the sub-endothelial space of blood vessels], cancer (oxidative damage to DNA and mutagenesis, resistance to free radical-inducing chemotherapy or radiation), and Alzheimer’s disease (oxidative damage-induced neuronal apoptosis, β-amyloid expression-secretion plaque formation).
4. Atherosclerosis
Reactive species in the vasculature are generated predominantly by the activity of NAD(P)H oxidase [38], xanthine oxidase, endothelial NO synthase (eNOS) and the mitochondrial respiratory chain [39,40]. One of the first events in atherogenesis is the oxidative modification of LDL induced by ROS and/or RNS. Oxidized LDL (oxLDL) stimulates CD36 and scavenger receptor-A (SR-A) expression in monocytes, macrophages and smooth muscle cells. This induces foam cell formation in macrophages as oxLDL uptake and retention are enhanced by the activity of these receptors. Further, oxLDL induces hypertrophy and hyperplasia of smooth muscle cells, which, in turn, narrow the vascular lumen. OxLDL also stimulates a pro-inflammatory state, inducing the expression of endothelial adhesion molecules, chemotaxis of leukocytes and inhibiting the migration of macrophages outside the sub-endothelial space. Taken together, oxLDL enhances many factors that contribute to the formation of an atherosclerotic lesion [25]. In addition, there are growing lines of evidence for candidate oxidative stress pathway genes that may predispose the vasculature of individuals to a pro-inflammatory/atherogenic condition. These include genes encoding p22phox (an NAD(P)H oxidase), mitochondrial SOD, manganese SOD and NO synthase 3 [41–43]. Other polymorphisms associated with cardiovascular disease have previously been reviewed at length [44]. Alpha-tocopherol hampers the pro-atherogenic effects of oxidative stress not only by acting as a potent free radical scavenger, but also as a regulator of gene expression. The SR-A and CD36 scavenger receptors, mentioned above, are down-regulated in the presence of α-tocopherol [45–47]. Thus, gene regulation may be an important component of anti-atherogenic effects of vitamin E.
5. Clinical utility of alpha-tocopherol for cardiovascular disease
Although there is mounting evidence for the efficacy of α-tocopherol and other antioxidants such as vitamin C in the treatment and/or prevention of cardiovascular disease, there are conflicting reports on the use of vitamin E in clinical studies. Of five primary prevention studies, four showed no positive effect of vitamin E in the incidence of cardiovascular disease events (α-Tocopherol Beta-Carotene Cancer Prevention Study (ATBC) [48], Primary Prevention Project (PPP) [49], Vitamin E Atherosclerosis Prevention Study (VEAPS) [50], Age-Related Eye Disease Study (AREDS) [51], one showing a benefit of vitamin E (Antioxidant Supplementation in Atherosclerosis Prevention (ASAP) Study [52]). Of the secondary intervention trials for vitamin E, that is, in testing the occurrence of endpoints of cardiovascular disease in subjects who have already undergone a disease event. Of seven clinical studies, four show no concise beneficial effect (Gruppo Italiano per lo Studio della Sopravvivenza nell’Infartomiocardico (GISSI) [53], The Heart Outcomes Prevention Evaluation (HOPE) [54], Heart Protection Study (HPS), Womens’ Angiographic Vitamin and Estrogen Trial (WAVE) [55], HDL-Atherosclerosis Treatment Study (HATS) [56] while the other three demonstrated a positive outcome for individuals with higher vitamin E intake [Cambridge Heart Antioxidant Study (CHAOS) [57], Secondary prevention with antioxidants of cardiovascular disease in endstage renal disease (SPACE) [58], and the Transplant-associated Arteriosclerosis study [59].] The authors of a meta-analysis of seven randomized trials [60] concluded that there were no significant differences in individuals given vitamin E, alone or in combination with beta-carotene, on mortality or incidence of stroke. Taken together, there is no concrete evidence of vitamin E as an intervening agent to reduce the mortality caused by cardiovascular disease, particularly in high-risk individuals [60].
A number of explanations have been proposed for the lack of consistency in the results of randomized trials. Certain factors in the trial subjects were not taken into consideration namely, general lifestyle, diet and fitness (all factors that are difficult to accurately assess) that may play a role in cardio-vascular disease risk. Also, the trials vary from the use of vitamin supplements to the survey of antioxidant rich food intake. The use of the latter is more complex due to the additional nutrients such as flavonoids, lycopenes, polyphenols that are present in antioxidant rich foods. It is widely accepted in the medical community that a ‘healthy diet’ is essential in the prevention of cardiovascular disease and some dietary intervention studies have shown a benefit of antioxidant rich foods such as fruits and vegetables or a ‘Mediterranean’ diet [61,62]. This may suggest that antioxidants like vitamin E may act synergistically with other naturally occurring compounds to maintain a healthy cardiovascular system. In addition to its antioxidant function, α-tocopherol has been shown to have other effects on cellular processes, namely on the immune system, proliferation and apoptosis, which makes it an interesting candidate for the study of its anti-cancer properties.
6. Cancer
The capacity of vitamin E, particularly α-tocopherol, to quench free radical damage, induce apoptosis and impact expression of oncogenes makes it a strong candidate for chemotherapeutic strategies. Earlier studies showed that vitamin E inhibited carcinogenesis and UV-induced DNA damage in animal models [63–66]. These preclinical data also revealed that this micronutrient might stimulate an anti-tumor immune response by inducing chemotaxis of macrophages and lymphocytes to the tumor site [67]. Tumor responses seen following vitamin E treatment may also be due to activation of anti-tumor factors, including tumor necrosis factors (TNF-α and TNF-β) and wild-type p53 [68]. Furthermore, vitamin E and its derivatives have shown potent apoptotic effects in several human cancer cell lines [69,70] and also enhance the efficacy of chemotherapeutic agents in animal models [71]. In addition to its enhancement of apoptotic pathways, vitamin E can also inhibit tumor survival factors such as protein kinase C (PKC) [65]. PKC is a well-established anti-apoptotic factor expressed in several tumor types. Amazingly, vitamin E, specifically α-tocopherol succinate (α-TS), has a tumor-specific impact in prostate and breast tissue, as treatment did not cause apoptosis in normal epithelial cells [72]. Through its proven efficacy in several cancer cell studies and promising results from preliminary clinical trials, α-TS has been dubbed the most effective form of vitamin E in the adjuvant treatment of cancer [73] when used in combination with other micronutrients (such as vitamin C, retinoic acid and carotenoids) during chemotherapy or radiation [74]. However, other clinical trials have given mixed reviews on the use of vitamin E during chemotherapy. Bairati et al. [75], conducted a multi-center, randomized, double-blind placebo controlled trial of 540 patients with stage I or II head and neck cancer, being concurrently treated with radiation therapy. Patients were treated daily with 400IU α-tocopherol and or placebo during radiation and continued 3 years beyond. Unexpectedly, patients supplemented with α-tocopherol had a higher incidence of second primary cancers and a lower degree of cancer-free survival [76]. One study showed that α-tocopherol helped to prevent in the prevention of oral squamous cell carcinomas even though three prospective randomized trials using vitamin E as a chemopreventive agent failed to show positive effects (CARET, ATBC and PHS) [77]. A few studies showed that α-tocopherol was of little to no benefit in preventing lung cancer [78].
The uptake and absorption of vitamin E, being similar to dietary cholesterol, is an interesting parallel, since it may, in some cases, counteract the uptake and delivery of excess cholesterol to tissues [79]. Abnormal LDL cholesterol metabolism has shown an association with many forms of cancer including prostate, breast, pancreatic, squamus cell and small cell lung cancer [80–83]. LDL is the primary carrier of essential polyunsaturated fatty acids, such as linoleic acid and arachidonic acid (AA) [84]. The carriage of excess AA into cells may enhance proliferation through its conversion to prostaglandin E2 (by cyclooxygenase-2) and downstream regulation of immediate early genes (such as c-fos) [85,86]. Hence, the effects of vitamin E derivatives on cancer cells in vivo may begin with its effects on LDL uptake.
As mentioned in the previous section, several reasons may exist for varied clinical reports on the effects of a dietary supplement. These could include variability in the forms of vitamin E used (α-tocopherol, α-TS), type of cancer, stage of disease and method of treatment [74,75]. Studies demonstrating the anti-cancer actions of vitamin E, particularly α-TS, in human cancer cells cannot be disregarded and warrant further examination.
7. Alzheimer’s disease
Aside from heart disease and cancer, free radical damage is also involved in the etiology of several neurodegenerative diseases such as Alzheimer’s. AD is the most common neurodegenerative disease, affecting nearly 15 million people worldwide [87] and present in almost half of individuals over the age of 85 [88]. Post-mortem AD brain biopsies show an accumulation of extracellular senile plaques in neurofibrillary tangles and cerebral blood vessels. The plaques themselves are comprised of fibrillar and non-fibrillar β-amyloid (AB) peptide and the resultant loss of neuronal function in the limbic and association cortices causes the major symptom of AD, dementia [89]. At the cellular level, AB renders oxidative damage to proteins and lipids, propagates ROS formation, stimulates NOS activity and oxidizes glutamine synthetase (a key enzyme an excitatory neurotransmission pathway). Potentiation of ROS generation by oxidized proteins eventually results in neuronal death. Therefore, the antioxidant function of α-tocopherol (in supplements) and dietary tocopherol as a therapeutic strategy for Alzheimer’s disease has been studied in both the preclinical and clinical setting.
In several animal studies of oxidant-induced brain injury animal models, the use of α-tocopherol/tocopherol succinate, alone or in combination with other antioxidative nutrients (proanthocyanidin, ascorbic acid, beta-carotene), helps to reduce the levels of brain damaging ROS and/or oxidative products [90,91]. Epidemiological studies and clinical trials, similar to vitamin E supplementation and heart disease, show mixed results of modest benefit to no effect of this antioxidant in efforts to prevent AD and/or dementia. Response relies on many factors including the form of vitamin E administered (in supplemental form or from food sources), with a mixture of various tocopherols showing improved ability to protect against AD compared to α-tocopherol alone [92,93]. A separate clinical study showed that α-tocopherol supplementation did, in fact, delay or prevent the AD diagnosis of elderly individuals with signs of mild cognitive impairment [94]. The use of ascorbic acid in concert with vitamin E, as in many heart disease prevention trials, also may improve the effects of this micronutrient in patients with AD [95]. However, some clinical studies show no evidence for the utility of vitamin E in individuals affected by AD [96–98].
Cognitive benefit of vitamin E supplementation may often rely on genetic factors [92]. For example, individuals that carry an allele of the ϵ4 isoform of APOE were shown to benefit more from vitamin E compared to non-ϵ4 carrying subjects [89]. Several genes have shown association with AD predisposition, APOE ϵ4 being the most common [89,99]. APOE-associated VLDL particles are most abundant in brain tissue, which is consistent with mounting evidence that AD pathology is associated with cholesterol metabolism [87,100]. Individuals with high cholesterol were shown to be at an increased risk of developing AD [101]. To this end, patients administered cholesterol-lowering therapy (statins), demonstrated a decreased incidence of AD and lowered Aβ levels [102–105]. Several genetic factors may exist that could modulate the efficacy of vitamin E in AD. ABCA1 is a key transporter in the cellular efflux of HDL and influences the age of AD onset and cholesterol levels within cerebrospinal fluid [106]. Tangier disease patients, harboring mutations in ABCA1, are typically shown to have reduced plasma HDL levels and a concomitant increase in the risk for AD development [106,107]. Polymorphism in CYP46A1, a central cholesterol catabolic enzyme in the brain, is also associated with this disease [108]. In addition, the ABC transporter-2 gene (ABCA2), which, like its close relative ABCA1 with high expression in the brain, is genetically linked to AD, involved in cholesterol trafficking and may have an additional role in resistance to oxidative stress [109,110].
Several lines of preclinical and clinical evidence indicate a preventive and therapeutic role for vitamin E in AD. In addition to an antioxidant function, the aforementioned nonantioxidant properties of α-tocopherol in modulating gene expression may also play a role in cell signaling pathways. Most data at the present time support its antioxidant role in the brain, although the precise mechanism for the protective effects of this nutrient against oxidative damage caused and perpetuated by AD remains elusive [94].
8. Conclusions
Inconsistencies in the results of vitamin E treatment in clinical trials may be due to the variety of doses used and forms of the supplied micronutrient (natural versus synthetic, and/or mixtures of the tocopherol isoforms). Bioavailability may also vary among individuals based on a number of genetic and environmental factors. In addition, males and females may have more differences since vitamin E is carried in different lipoproteins (LDL for males and HDL for females) [111]. Inter-individual variability also plays a factor in terms of risk. For example, individuals with chronic liver disease (cholestasis) and cystic fibrosis (reduced pancreatic enzyme secretion) have a hampered intestinal absorption of lipid soluble nutrients [112]. Hormone replacement therapy clinical trials also face similar inconsistencies when risk factors are attempted to be assessed for cardiovascular disease, Alzheimer’s disease and breast cancer development [4]. Conflicting reports for the clinical efficacy of lipid soluble vitamins (E, C, beta-carotene, etc.) and exogenous hormones may be due to such variation in uptake and local concentrations for effected systems/tissues, not to mention the battery of genetic, environmental, and behavioral differences between individuals. Vitamin E, cholesterol, and other lipid soluble and sterol related compounds, may share common pathways of uptake and systemic/intracellular distribution. Each has been shown, in preclinical studies, to be effectors of gene expression and impact the local oxidative environment. Technological advances in biomedical science are moving toward the elucidation of each of these pathways in preclinical and clinical studies. As the understanding of these complex and often intersecting pathways is ever-increasing, future treatments will undoubtedly become more customized for individuals affected by cancer, heart disease and dementia, diseases that plague a majority of aging individuals.
References
Full text links
Read article at publisher's site: https://doi.org/10.1016/j.biopha.2005.06.005
Read article for free, from open access legal sources, via Unpaywall:
https://europepmc.org/articles/pmc6361124?pdf=render
Citations & impact
Impact metrics
Article citations
Antimicrobial Properties and Therapeutic Potential of Bioactive Compounds in <i>Nigella sativa</i>: A Review.
Molecules, 29(20):4914, 17 Oct 2024
Cited by: 0 articles | PMID: 39459282 | PMCID: PMC11510594
Review Free full text in Europe PMC
Chemical Profiling and Antioxidant, Anti-Inflammatory, Cytotoxic, Analgesic, and Antidiarrheal Activities from the Seeds of Commonly Available Red Grape (Vitis vinifera L.).
Nutr Metab Insights, 17:11786388241275100, 18 Sep 2024
Cited by: 0 articles | PMID: 39315363 | PMCID: PMC11418367
Targeting mitochondrial quality control: new therapeutic strategies for major diseases.
Mil Med Res, 11(1):59, 21 Aug 2024
Cited by: 2 articles | PMID: 39164792 | PMCID: PMC11337860
Review Free full text in Europe PMC
Myalgic Encephalomyelitis/Chronic Fatigue Syndrome: the biology of a neglected disease.
Front Immunol, 15:1386607, 03 Jun 2024
Cited by: 4 articles | PMID: 38887284 | PMCID: PMC11180809
Review Free full text in Europe PMC
Physiological potential of different Sorghum bicolor varieties depending on their bioactive characteristics and antioxidant potential as well as different extraction methods.
Heliyon, 10(16):e35807, 05 Aug 2024
Cited by: 0 articles | PMID: 39220962 | PMCID: PMC11365355
Go to all (94) article citations
Similar Articles
To arrive at the top five similar articles we use a word-weighted algorithm to compare words from the Title and Abstract of each citation.
Vitamin E: a dark horse at the crossroad of cancer management.
Biochem Pharmacol, 86(7):845-852, 03 Aug 2013
Cited by: 35 articles | PMID: 23919929 | PMCID: PMC4100069
Review Free full text in Europe PMC
Vitamin E forms in Alzheimer's disease: a review of controversial and clinical experiences.
Crit Rev Food Sci Nutr, 50(5):414-419, 01 May 2010
Cited by: 23 articles | PMID: 20373187
Review
Vitamin E and its function in membranes.
Prog Lipid Res, 38(4):309-336, 01 Jul 1999
Cited by: 229 articles | PMID: 10793887
Review
Role of Vitamin E in the Treatment of Alzheimer's Disease: Evidence from Animal Models.
Int J Mol Sci, 18(12):E2504, 23 Nov 2017
Cited by: 47 articles | PMID: 29168797 | PMCID: PMC5751107
Review Free full text in Europe PMC