Abstract
Rationale
Fine particulate matter air pollution of 2.5 μm or less in diameter (PM2.5) has been associated with an increased risk of respiratory disease, but assessments of specific respiratory infections in adults are lacking.Objectives
To estimate the rate of respiratory infection healthcare encounters in adults associated with acute increases in PM2.5 concentrations.Methods
Using case-crossover methods, we studied 498,118 adult New York State residents with a primary diagnosis of influenza, bacterial pneumonia, or culture-negative pneumonia upon hospitalization or emergency department (ED) visit (2005-2016). We estimated the relative rate of healthcare encounters associated with increases in PM2.5 in the previous 1-7 days and explored differences before (2005-2007), during (2008-2013), and after (2014-2016) implementation of air quality policies and economic changes.Results
Interquartile range increases in PM2.5 over the previous 7 days were associated with increased excess rates (ERs) of culture-negative pneumonia hospitalizations (2.5%; 95% confidence interval [CI], 1.7-3.2%) and ED visits (2.5%; 95% CI, 1.4-3.6%), and increased ERs of influenza ED visits (3.9%; 95% CI, 2.1-5.6%). Bacterial pneumonia hospitalizations, but not ED visits, were associated with increases in PM2.5 and, though imprecise, were of a similar magnitude to culture-negative pneumonia (Lag Day 6 ER, 2.3%; 95% CI, 0.3-4.3). Increased relative rates of influenza ED visits and culture-negative pneumonia hospitalizations were generally larger in the "after" period (P < 0.025 for both outcomes), compared with the "during" period, despite reductions in overall PM2.5 concentrations.Conclusions
Increased rates of culture-negative pneumonia and influenza were associated with increased PM2.5 concentrations during the previous week, which persisted despite reductions in PM2.5 from air quality policies and economic changes. Though unexplained, this temporal variation may reflect altered toxicity of different PM2.5 mixtures or increased pathogen virulence.Free full text

The Association between Respiratory Infection and Air Pollution in the Setting of Air Quality Policy and Economic Change
Abstract
Rationale: Fine particulate matter air pollution of 2.5 μm or less in diameter (PM2.5) has been associated with an increased risk of respiratory disease, but assessments of specific respiratory infections in adults are lacking.
Objectives: To estimate the rate of respiratory infection healthcare encounters in adults associated with acute increases in PM2.5 concentrations.
Methods: Using case–crossover methods, we studied 498,118 adult New York State residents with a primary diagnosis of influenza, bacterial pneumonia, or culture-negative pneumonia upon hospitalization or emergency department (ED) visit (2005–2016). We estimated the relative rate of healthcare encounters associated with increases in PM2.5 in the previous 1–7 days and explored differences before (2005–2007), during (2008–2013), and after (2014–2016) implementation of air quality policies and economic changes.
Results: Interquartile range increases in PM2.5 over the previous 7 days were associated with increased excess rates (ERs) of culture-negative pneumonia hospitalizations (2.5%; 95% confidence interval [CI], 1.7–3.2%) and ED visits (2.5%; 95% CI, 1.4–3.6%), and increased ERs of influenza ED visits (3.9%; 95% CI, 2.1–5.6%). Bacterial pneumonia hospitalizations, but not ED visits, were associated with increases in PM2.5 and, though imprecise, were of a similar magnitude to culture-negative pneumonia (Lag Day 6 ER, 2.3%; 95% CI, 0.3–4.3). Increased relative rates of influenza ED visits and culture-negative pneumonia hospitalizations were generally larger in the “after” period (P<
0.025 for both outcomes), compared with the “during” period, despite reductions in overall PM2.5 concentrations.
Conclusions: Increased rates of culture-negative pneumonia and influenza were associated with increased PM2.5 concentrations during the previous week, which persisted despite reductions in PM2.5 from air quality policies and economic changes. Though unexplained, this temporal variation may reflect altered toxicity of different PM2.5 mixtures or increased pathogen virulence.
Influenza and bacterial pneumonia are a leading cause of adult morbidity and mortality in the United States (1), and their risk factors are actively being studied (2, 3). Although fine particulate matter air pollution of 2.5 μm or less in diameter (PM2.5) has previously been associated with general cardiopulmonary morbidity and mortality worldwide (4), studies have also identified it as a potential contributor to respiratory infection in both adults and children (5–9), specifically, influenza (5, 6) and bacterial pneumonia (7, 9). An increased risk of respiratory infection associated with PM2.5 exposure has been reported in Utah (6, 8), and in a study of four other U.S. cities (10). Recent studies examined PM2.5 associations with respiratory viral infections in children (0–17 yr old) and adults (≥18 yr old) (6), lower respiratory infection severity in older adults (≥65) (8), and respiratory disease in general, including pneumonia in children and adults (10). Although specific respiratory infections (e.g., respiratory syncytial virus) have been studied in children, it is unclear whether specific respiratory infections (e.g., culture-negative pneumonia, influenza, and bacterial pneumonia) in adults are associated with short-term PM2.5 exposure.
Changes in the ambient concentration and composition of PM2.5 provide a unique opportunity to investigate whether these changes in ambient PM2.5 composition result in changes in the rate of respiratory infection associated with each IQR increase in PM2.5 concentration (i.e., is the same PM2.5 mass more or less toxic?). Policy initiatives to improve air quality in New York State and the Northeast Region of the United States over the past 10 years were summarized by Squizzato and colleagues (11). These initiatives included requirements for ultralow sulfur (<15 ppm S) fuel for on-road and nonroad diesel, and after July 1, 2012, for home heating. Pollution controls were required for on-road, heavy-duty, diesel vehicles. Ontario, Canada has closed its coal-fired power plants, and emission controls were installed on Ohio River Valley plants, reducing NOx and SO2 emissions upwind of New York State. The 2008 recession and the change in relative prices of natural gas and coal and oil resulted in a shift in generation to gas (11). PM2.5, NOx, and SO2 concentrations have decreased across New York State after these policies were implemented and the 2008 recession occurred (11). However, the composition of PM changed, with increased concentrations of secondary organic carbon (SOC) (12), which might modify the rate of respiratory infections associated with PM2.5.
To address the relative lack of studies of specific respiratory infections in adults, we used a large, multiyear (2005–2016) New York state–wide database to separately estimate the rates of hospital admissions and emergency department (ED) visits for influenza, bacterial pneumonia, and culture-negative pneumonia, among New York adult residents, associated with short-term increases in mean PM2.5 concentrations in the previous 1–7 days. We hypothesized that increased PM2.5 concentrations would be associated with increased rates of all outcomes. We then explored whether each relative rate differed before (2005–2007), during (2008–2013), and after (2014–2016) the changes in air quality described previously here.
Methods
Study Population
From the SPARCS (Statewide Planning and Research Cooperative System) database, respiratory infection hospital admissions and ED visits (patients treated and released home) were retained for all adult New York residents (≥18 yr of age) who lived within 15 miles of the Buffalo, Rochester, Albany, Bronx, Manhattan, or Queens, New York, PM2.5 monitoring sites from January 1, 2005 to December 31, 2016 (n=
319,570 hospitalizations and n
=
178,548 ED visits were available for analysis). We included subjects with a primary diagnosis (at time of hospitalization or ED visit) of influenza (International Classification of Disease [ICD] 9
=
487.0, 487.8, 488.0, 488.01, 488.02, 488.1, 488.11, 488.12, 488.8, 488.81, 488.82; ICD10
=
J09, J09.X1, J09.X2, J10.0, J10.00, J10.01, J10.08, J10.1, J11.0, J11.00, J11.08, J11.1), bacterial pneumonia (ICD9
=
481, 482, 483.0, 483.1; ICD10
=
J13, J14, J15, J16, A48.1), or culture-negative pneumonia (ICD9
=
485, 486; ICD10
=
J18). Culture-negative pneumonia is a common diagnosis, as modern culture techniques only identify a causative pathogen in under 50% of the patients diagnosed with pneumonia (13, 14). Culture-negative pneumonia is best viewed as an undifferentiated infection, as it can be bacterial or viral in origin. This study was reviewed and approved by the Institutional Review Board at the University at Albany, State University of New York.
Air Pollution and Weather
Hourly PM2.5 concentrations at the six urban air-monitoring stations (Buffalo, Rochester, Albany, the Bronx, Manhattan, and Queens, NY) were retrieved from the U.S. Environmental Protection Agency (https://aqs.epa.gov/api). Further details on measurement of PM2.5, temperature, and relative humidity have been described previously (12). For each subject, daily PM2.5, temperature, and relative humidity values were assigned from the monitoring station closest to their residence.
Statistical Analysis
To estimate the rate of respiratory infection hospital admissions and ED visits associated with each interquartile range (IQR) increase in PM2.5 concentration on the same day (Lag Day 0), we used a time-stratified, case–crossover design (15, 16). For all influenza hospital admissions from all six urban sites (assuming a common slope across sites), we fit a conditional logistic regression model stratified on each respiratory infection hospital admission matched set (one case and three to four control periods per subject), and regressed case–control status (i.e., case=
1, control
=
0) against the mean PM2.5 concentration on case and control days. Because case periods and their matched control periods are derived from the same person, and a conditional analysis is conducted, non–time-varying confounders, such as underlying medical conditions, long-term time trends, and season, are controlled by design. As is standard in case–crossover studies, from this statistical model, the odds ratio is a direct estimate of the rate ratio and its 95% confidence interval (CI). The excess rate (ER) is the percent increase in the rate per unit of exposure (i.e., [rate ratio
−
1.0]
×
100%).
We included natural splines for temperature and relative humidity (4 df), which were determined using Akaike’s information criterion (17). This same model was run for the PM2.5 means of Lag Days 0–1, 0–2, 0–3, 0–4, 0–5, and 0–6, and then separately for ED visits and hospitalizations for influenza, bacterial pneumonia, and culture-negative pneumonia. Because we examined seven lag times for each disease subgroup, statistical significance for slopes was defined as P less than 0.007 (0.05/7). The lag times averaged over increasing lag days rather than segmental lag days (i.e., Day 1, Day 2, Day 3) were used to more accurately view the ER pattern across lag times. In the Results section, we present the largest lagged effect, and then describe whether other lag times had similar effects. Furthermore, inference was made considering several factors, including the pattern of response across these lag averaging times, the precision of each estimate, as well as statistical significance.
Next, we explored whether the association between PM2.5 and each respiratory infection admission rate differed by period (before=
2005–2007, during
=
2008–2013, after
=
2014–2016), by adding indicator variables for period and two interaction terms of period and PM2.5 to the model. The significance of the difference of the PM2.5 effect across periods was evaluated by a 2-df test for interaction. If statistically significant, we examined whether the ER in the after period was different from the ER in the during and before periods, using a P value of 0.025 to define statistical significance. All analyses were done using R version 3.0.1 (https://www.r-project.org/).
Results
Subjects who were hospitalized or required ED evaluation for respiratory infection were predominantly older in the before period (mean age, 65 yr) compared with the during and after periods (59 yr old). The race/ethnicity of the subject population changed from the before to the after period with a decreased proportion of white subjects (54% down to 43%) and a larger proportion of black and Hispanic subjects (24% up to 27% and 15% up to 18%, respectively). The race and sex of subjects (53–54% female) remained stable across the multiple periods.
The majority of patients in the before, during, and after periods had health care encounters (hospitalizations or ED visits) for culture-negative pneumonia (89%, 77%, and 68%, respectively). The most common comorbidities for subjects included hypertension (20–38%), fluid and electrolyte disorders (15–28%), diabetes (13–22%), and heart failure (13–22%) (Table 1). The mean (±SD) length of stay for subjects decreased from the before period (5.6 d) to the after period (3.3 d) (Table 1). The overall number of yearly hospitalizations steadily decreased (from 34,458 to 21,735), whereas the number of ED visits increased (from 9,799 to 20,874) from 2005 to 2016. The distributions of PM2.5 concentrations for case and control periods are shown in Figure 1 and in Table E1 in the online supplement. Overall, case and control period concentrations were similar. We did not observe a difference in the seasonal patterns for respiratory infection hospitalizations or ED visits from 2005 to 2016 (see Table E2). For influenza, the highest proportion of admissions and ED visits were present in winter and spring, whereas the lowest proportion of admissions was present in summer and fall.
Table 1.
Characteristics of respiratory infectious hospital admissions and emergency department visits (2005–2016), by study site/city
Characteristic | Before (n![]() ![]() | During (n![]() ![]() | After (n![]() ![]() | |||
---|---|---|---|---|---|---|
n | % | n | % | n | % | |
Infection | ||||||
![]() | 111,450 | 89 | 193,713 | 77 | 83,060 | 68 |
![]() | 6,057 | 5 | 38,745 | 15 | 27,484 | 23 |
![]() | 8,310 | 7 | 18,387 | 7 | 10,912 | 9 |
Male | 59,377 | 47 | 116,344 | 46 | 55,530 | 46 |
Age, yr, mean (SD) | 65 (21) | 59 (22) | 59 (21) | |||
![]() | 18,016 | 14 | 54,975 | 22 | 26,979 | 22 |
![]() | 14,296 | 11 | 30,407 | 12 | 14,092 | 12 |
![]() | 15,689 | 12 | 36,159 | 14 | 19,416 | 16 |
![]() | 16,988 | 14 | 34,098 | 14 | 18,126 | 15 |
![]() | 23,160 | 18 | 36,110 | 14 | 17,173 | 14 |
![]() | 37,668 | 30 | 59,096 | 24 | 25,670 | 21 |
Race/ethnicity | ||||||
![]() | 67,804 | 54 | 121,693 | 49 | 52,563 | 43 |
![]() | 30,181 | 24 | 65,887 | 26 | 32,924 | 27 |
![]() | 790 | 1 | 1,267 | 1 | 335 | — |
![]() | 4,150 | 3 | 9,945 | 4 | — | — |
![]() | 47 | — | 161 | — | — | — |
![]() | 18,260 | 15 | 43,293 | 17 | 21,699 | 18 |
Year | ||||||
![]() | 44,257 | 35 | — | — | — | — |
![]() | 41,569 | 33 | — | — | — | — |
![]() | 39,991 | 32 | — | — | — | — |
![]() | — | — | 40,894 | 16 | — | — |
![]() | — | — | 51,487 | 21 | — | — |
![]() | — | — | 36,711 | 15 | — | — |
![]() | — | — | 39,736 | 16 | — | — |
![]() | — | — | 39,260 | 16 | — | — |
![]() | — | — | 42,757 | 17 | — | — |
![]() | — | — | — | — | 40,412 | 33 |
![]() | — | — | — | — | 38,438 | 32 |
![]() | — | — | — | — | 42,606 | 35 |
Season | ||||||
![]() | 27,126 | 22 | 57,659 | 23 | 22,744 | 19 |
![]() | 33,880 | 27 | 61,976 | 25 | 35,484 | 29 |
![]() | 25,263 | 20 | 49,191 | 20 | 20,553 | 17 |
![]() | 39,548 | 31 | 82,019 | 33 | 42,675 | 35 |
Length of stay, d, mean (SD) | 5.6 (9.0) | 4.3 (8.2) | 3.3 (5.6) | |||
Comorbidity | | |||||
![]() | 47,285 | 38 | 83,298 | 33 | 24,610 | 20 |
![]() | 35,774 | 28 | 64,010 | 26 | 17,990 | 15 |
![]() | 28,161 | 22 | 53,119 | 21 | 16,164 | 13 |
![]() | 25,652 | 20 | 54,113 | 22 | 15,616 | 13 |
![]() | 17,523 | 14 | 46,396 | 19 | 16,689 | 14 |
![]() | 22,705 | 18 | 39,104 | 16 | 10,642 | 9 |
![]() | 21,918 | 17 | 38,262 | 15 | 11,269 | 9 |
![]() | 15,035 | 12 | 34,835 | 14 | 12,665 | 10 |
![]() | 15,964 | 13 | 31,175 | 12 | 8,598 | 7 |
![]() | 14,260 | 11 | 31,187 | 12 | 9,856 | 8 |
![]() | 12,535 | 10 | 28,939 | 12 | 8,204 | 7 |
![]() | 9,996 | 8 | 27,455 | 11 | 9,923 | 8 |
![]() | 7,265 | 6 | 27,688 | 11 | 9,232 | 8 |
![]() | 6,158 | 5 | 24,215 | 10 | 13,482 | 11 |
![]() | 9,898 | 8 | 23,967 | 10 | 9,634 | 8 |
Definition of abbreviation: SD=
standard deviation.
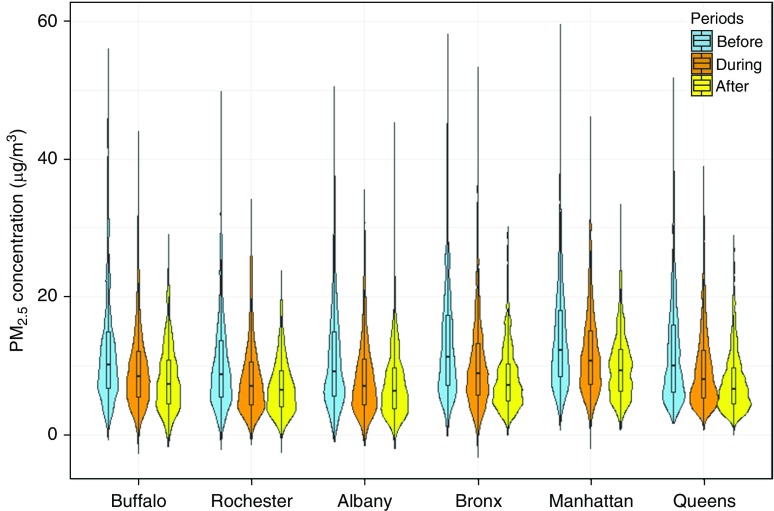
Distribution of fine particulate matter air pollution of 2.5 μm or less in diameter (PM2.5) concentrations (μg/m3) for case periods by study site and time period.
For culture-negative pneumonia, IQR increases in PM2.5 concentration in the previous 2–7 days (Lag Days 0–1, 0–2, 0–3, 0–4, 0–5, and 0–6) were associated with increased rates of hospitalizations, with the largest in the previous 5 days (ER, 2.5%; 95% CI, 1.8–3.2%) and 6 days (ER, 2.5%; 95% CI, 1.7–3.2%) (Figure 2; Table 2). Similarly, IQR increases in PM2.5 in the previous 4–7 days were associated with increased rates of culture-negative pneumonia ED visits, with the largest in the previous 6 days (ER, 2.5%; 95% CI, 1.4–3.6%). Although there were no associations between PM2.5 and influenza hospitalizations, IQR increases in the previous 5–7 days were associated with increased rates of ED visits for influenza, with the largest at 7 days (ER, 3.9%; 95% CI, 2.1–5.6%). Though imprecise due to low sample size, the ER of bacterial pneumonia hospitalizations in the previous 1–7 days were of similar magnitude to those of culture-negative pneumonia (Lag Day 6 ER, 2.3%; 95% CI, 0.3–4.3). No association was observed between PM2.5 and ED visits for bacterial pneumonia (Table 2 and Figure 2).
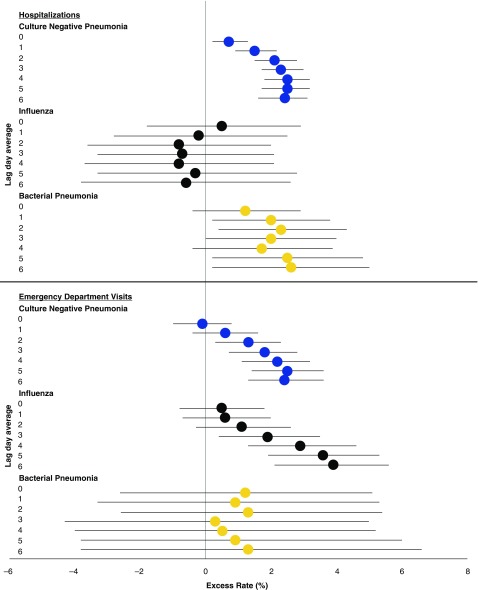
Excess rate (and 95% confidence interval [CI]) of infectious disease hospitalizations and emergency department visits associated with each interquartile range increase in concentration of fine particulate matter air pollution of 2.5 μm or less in diameter, by infection and lag day mean.
Table 2.
Excess rate of respiratory infectious hospital admissions and emergency department visits associated with interquartile range increases in fine particulate matter air pollution of 2.5 μm or less in diameter concentration, by lag time, and outcome*
Outcome | Lag Days | Hospital Admissions | Emergency Department Visits | ||||||
---|---|---|---|---|---|---|---|---|---|
IQR (μg/m3) | n Cases | Excess Rate % (95% CI) | P Value | IQR (μg/m3) | n Cases | Excess Rate % (95% CI) | P Value | ||
Culture-negative pneumonia | 0 | 7 | 267,905 | 0.7 (0.2 to 1.3) | 0.01 | 6.6 | 110,982 | −0.1 (−1.0 to 0.8) | 0.78 |
0–1 | 6.5 | 269,968 | 1.5 (0.9 to 2.2) | <0.001 | 6.1 | 112,113 | 0.6 (−0.4 to 1.6) | 0.22 | |
0–2 | 6.2 | 272,191 | 2.1 (1.5 to 2.8) | <0.001 | 5.8 | 113,187 | 1.3 (0.3 to 2.3) | 0.01 | |
0–3 | 5.7 | 272,681 | 2.3 (1.7 to 3.0) | <0.001 | 5.3 | 113,382 | 1.8 (0.7 to 2.8) | <0.001 | |
0–4 | 5.5 | 273,017 | 2.5 (1.8 to 3.2) | <0.001 | 5.1 | 113,521 | 2.2 (1.1 to 3.2) | <0.001 | |
0–5 | 5.2 | 273,266 | 2.5 (1.7 to 3.2) | <0.001 | 4.9 | 113,623 | 2.5 (1.4 to 3.6) | <0.001 | |
0–6 | 5 | 273,430 | 2.4 (1.6 to 3.1) | <0.001 | 4.8 | 113,679 | 2.4 (1.3 to 3.6) | <0.001 | |
Influenza | 0 | 6.4 | 14,072 | 0.5 (−1.8 to 2.9) | 0.67 | 6.7 | 55,819 | 0.5 (−0.8 to 1.8) | 0.47 |
0–1 | 6.5 | 14,388 | −0.2 (−2.8 to 2.5) | 0.88 | 6.4 | 56,573 | 0.6 (−0.7 to 2.0) | 0.36 | |
0–2 | 6.4 | 14,690 | −0.8 (−3.6 to 2.0) | 0.56 | 6.2 | 57,308 | 1.1 (−0.3 to 2.6) | 0.14 | |
0–3 | 5.7 | 14,729 | −0.7 (−3.3 to 2.1) | 0.63 | 6 | 57,365 | 1.9 (0.4 to 3.5) | 0.01 | |
0–4 | 5.6 | 14,738 | −0.8 (−3.7 to 2.1) | 0.58 | 5.9 | 57,399 | 2.9 (1.3 to 4.6) | <0.001 | |
0–5 | 5.5 | 14,750 | −0.3 (−3.3 to 2.8) | 0.86 | 5.7 | 57,430 | 3.6 (1.9 to 5.3) | <0.001 | |
0–6 | 5.5 | 14,758 | −0.6 (−3.8 to 2.6) | 0.71 | 5.4 | 57,455 | 3.9 (2.1 to 5.6) | <0.001 | |
Bacterial pneumonia | 0 | 6.5 | 29,774 | 1.1 (−0.4 to 2.7) | 0.16 | 6.2 | 6,862 | 1.6 (−1.9 to 5.1) | 0.37 |
0–1 | 6 | 30,066 | 1.9 (0.2 to 3.6) | 0.03 | 6 | 6,925 | 1.4 (−2.4 to 5.3) | 0.48 | |
0–2 | 5.7 | 30,378 | 2.1 (0.4 to 3.9) | 0.02 | 5.2 | 7,002 | 1.5 (−2.2 to 5.3) | 0.44 | |
0–3 | 5.3 | 30,442 | 1.8 (0.0 to 3.6) | 0.05 | 5.5 | 7,007 | 0.2 (−4.0 to 4.5) | 0.94 | |
0–4 | 5.2 | 30,484 | 1.7 (−0.2 to 3.6) | 0.07 | 5 | 7,010 | 0.0 (−4.1 to 4.3) | 0.99 | |
0–5 | 5.2 | 30,509 | 2.3 (0.3 to 4.3) | 0.03 | 5 | 7,019 | 0.4 (−4.0 to 5.0) | 0.87 | |
0–6 | 5 | 30,518 | 2.1 (0.1 to 4.2) | 0.04 | 5 | 7,020 | 0.5 (−4.2 to 5.4) | 0.83 |
Definition of abbreviations: CI=
confidence interval; IQR
=
interquartile range.
Next, we explored whether the relative rates of hospitalizations for each outcome associated with IQR increases in ambient PM2.5 concentrations in the before, during, and after periods were different to determine whether changes in PM concentration and composition may have differentially triggered these respiratory infections (Table 3). The rate of culture-negative pneumonia hospitalizations, but not ED visits, associated with each 6.2-μg/m3 increase in PM2.5 concentration in the previous 3 days (Lag Days 0–2) was different across periods (P=
0.002), with the largest ERs in the before period (2.9%; 95% CI, 2.0–3.8%) and after period (3.5%; 95% CI, 1.5–5.5%) compared with the during period (1.0%; 95% CI, 0.1–1.9%) (Figure 3). There were also generally similar patterns in the previous 2, 4, and 5 days. Similarly, the increased rate of influenza ED visits associated with each 6.4-μg/m3 increase in PM2.5 concentration in the previous 3 days was different across periods (P
<
0.001), with the largest ERs in the before period (3.0%; 95% CI, 0.5–5.6%) and after period (5.7%; 95% CI, 2.6–8.8%) compared with the during period (−0.7%; 95% CI, −2.5% to 1.1%) (Figure 3). There were similar patterns in the previous 1, 2, 4, and 5 days (Table 3).
Table 3.
Excess rate of respiratory infectious hospital admissions associated with each interquartile range increase in concentration of fine particulate matter air pollution of 2.5 μm or less in diameter, by lag time, outcome, and time period
Outcome | Lag Day | Before (n) | During (n) | After (n) | IQR (μg/m3) | Before | During | After | P Value for Interaction (2 df) | |||
---|---|---|---|---|---|---|---|---|---|---|---|---|
Excess Risk % (95% CI) | P Value | Excess Risk % (95% CI) | P Value | Excess Risk % (95% CI) | P Value | |||||||
Hospital admissions | ||||||||||||
![]() | 0 | 87,108 | 131,018 | 49,779 | 7.0 | 1.2 (0.4 to 1.9) | 0.002 | 0.2 (−0.6 to 1.0) | 0.67 | 1.0 (−0.7 to 2.7) | 0.26 | 0.16 |
0–1 | 87,347 | 132,663 | 49,958 | 6.5 | 2.2 (1.4 to 3.0) | <0.001 | 0.7 (−0.2 to 1.5) | 0.14 | 2.1 (0.2 to 3.9) | 0.03 | 0.02 | |
0–2 | 87,569 | 134,430 | 50,192 | 6.2 | 2.9 (2.0 to 3.8) | <0.001 | 1.0 (0.1 to 1.9) | 0.04 | 3.5 (1.5 to 5.5)* | <0.001 | 0.002 | |
0–3 | 87,614 | 134,839 | 50,228 | 5.7 | 3.1 (2.1 to 4.0) | <0.001 | 1.3 (0.4 to 2.3) | 0.01 | 3.7 (1.7 to 5.7)† | <0.001 | 0.01 | |
0–4 | 87,647 | 135,110 | 50,260 | 5.5 | 3.2 (2.2 to 4.2) | <0.001 | 1.5 (0.5 to 2.5) | 0.00 | 3.7 (1.6 to 5.8) | 0.001 | 0.02 | |
0–5 | 87,647 | 135,332 | 50,287 | 5.2 | 3.1 (2.1 to 4.1) | <0.001 | 1.6 (0.6 to 2.7) | 0.00 | 3.4 (1.3 to 5.6) | 0.002 | 0.06 | |
0–6 | 87,647 | 135,477 | 50,306 | 5.0 | 3.1 (2.1 to 4.2) | <0.001 | 1.5 (0.4 to 2.5) | 0.01 | 2.8 (0.6 to 5.0) | 0.01 | 0.06 | |
![]() | 0 | 1,031 | 5,883 | 7,158 | 6.4 | 7.2 (1.5 to 13.1) | 0.01 | −0.8 (−3.5 to 1.9) | 0.56 | 0.1 (−3.2 to 3.5) | 0.95 | 0.03 |
0–1 | 1,048 | 6,083 | 7,257 | 6.5 | 5.6 (−0.3 to 11.9) | 0.07 | −1.5 (−4.4 to 1.5) | 0.33 | −0.3 (−4.0 to 3.5) | 0.86 | 0.08 | |
0–2 | 1,066 | 6,265 | 7,359 | 6.4 | 4.5 (−1.7 to 11.1) | 0.16 | −2.3 (−5.3 to 0.8) | 0.14 | −0.1 (−4.0 to 4.1) | 0.98 | 0.07 | |
0–3 | 1,066 | 6,292 | 7,371 | 5.7 | 4.9 (−0.9 to 11.1) | 0.10 | −2.2 (−5.1 to 0.7) | 0.14 | 0.3 (−3.5 to 4.3) | 0.86 | 0.03 | |
0–4 | 1,066 | 6,295 | 7,377 | 5.6 | 4.8 (−1.2 to 11.1) | 0.12 | −2.4 (−5.4 to 0.7) | 0.13 | 0.3 (−3.7 to 4.4) | 0.89 | 0.03 | |
0–5 | 1,066 | 6,301 | 7,383 | 5.5 | 4.7 (−1.3 to 11.2) | 0.13 | −1.9 (−5.0 to 1.4) | 0.26 | 1.3 (−2.9 to 5.6) | 0.56 | 0.03 | |
0–6 | 1,066 | 6,306 | 7,386 | 5.5 | 4.2 (−2.0 to 10.8) | 0.19 | −2.2 (−5.5 to 1.3) | 0.22 | 0.9 (−3.3 to 5.4) | 0.67 | 0.04 | |
![]() | 0 | 7,452 | 14,424 | 7,898 | 6.5 | 1.8 (−0.1 to 3.9) | 0.07 | −0.0 (−2.1 to 2.2) | 0.99 | 2.1 (−1.6 to 6.0) | 0.27 | 0.33 |
0–1 | 7,503 | 14,629 | 7,934 | 6 | 2.3 (0.3 to 4.4) | 0.03 | 1.4 (−0.9 to 3.7) | 0.23 | 1.7 (−2.2 to 5.8) | 0.39 | 0.78 | |
0–2 | 7,564 | 14,838 | 7,976 | 5.7 | 2.3 (0.3 to 4.5) | 0.03 | 1.9 (−0.4 to 4.4) | 0.11 | 1.7 (−2.3 to 5.9) | 0.42 | 0.92 | |
0–3 | 7,573 | 14,886 | 7,983 | 5.3 | 1.8 (−0.3 to 4.0) | 0.09 | 1.9 (−0.6 to 4.4) | 0.13 | 1.6 (−2.5 to 5.9) | 0.44 | 0.99 | |
0–4 | 7,580 | 14,916 | 7,988 | 5.2 | 1.7 (−0.5 to 3.9) | 0.14 | 1.9 (−0.6 to 4.5) | 0.15 | 1.5 (−2.7 to 5.9) | 0.50 | 0.98 | |
0–5 | 7,580 | 14,938 | 7,991 | 5.2 | 2.2 (−0.1 to 4.5) | 0.06 | 2.6 (−0.1 to 5.4) | 0.06 | 1.3 (−3.1 to 5.9) | 0.56 | 0.88 | |
0–6 | 7,581 | 14,945 | 7,992 | 5 | 2.2 (−0.1 to 4.6) | 0.06 | 2.3 (−0.5 to 5.2) | 0.10 | 0.8 (−3.7 to 5.4) | 0.74 | 0.81 | |
Emergency department visits | ||||||||||||
![]() | 0 | 23,175 | 55,434 | 32,373 | 6.6 | −0.1 (−1.4 to 1.3) | 0.93 | −0.6 (−1.8 to 0.6) | 0.35 | 1.1 (−0.9 to 3.1) | 0.29 | 0.35 |
0–1 | 23,267 | 56,319 | 32,527 | 6.1 | 0.9 (−0.5 to 2.3) | 0.23 | 0.2 (−1.1 to 1.5) | 0.79 | 1.2 (−0.9 to 3.4) | 0.25 | 0.59 | |
0–2 | 23,353 | 57,167 | 32,667 | 5.8 | 1.5 (0.0 to 3.1) | 0.05 | 1.0 (−0.3 to 2.3) | 0.14 | 1.6 (−0.7 to 3.9) | 0.17 | 0.82 | |
0–3 | 23,360 | 57,329 | 32,693 | 5.3 | 2.0 (0.4 to 3.6) | 0.01 | 1.5 (0.2 to 2.9) | 0.03 | 1.9 (−0.4 to 4.2) | 0.10 | 0.88 | |
0–4 | 23,364 | 57,433 | 32,724 | 5.1 | 2.9 (1.2 to 4.6) | 0.001 | 1.7 (0.3 to 3.1) | 0.02 | 2.0 (−0.3 to 4.4) | 0.10 | 0.52 | |
0–5 | 23,364 | 57,518 | 32,741 | 4.9 | 3.2 (1.4 to 4.9) | <0.001 | 2.2 (0.7 to 3.7) | 0.004 | 2.2 (−0.2 to 4.7) | 0.08 | 0.64 | |
0–6 | 23,364 | 57,561 | 32,754 | 4.8 | 3.0 (1.2 to 4.9) | 0.001 | 2.1 (0.6 to 3.7) | 0.01 | 2.0 (−0.5 to 4.5) | 0.12 | 0.69 | |
![]() | 0 | 4,889 | 31,115 | 19,815 | 6.7 | 1.5 (−0.9 to 3.9) | 0.22 | −0.6 (−2.2 to 0.9) | 0.42 | 3.0 (0.5 to 5.6) | 0.02 | 0.04 |
0–1 | 4,931 | 31,710 | 19,932 | 6.4 | 2.0 (−0.4 to 4.5) | 0.10 | −0.7 (−2.4 to 1.0) | 0.41 | 3.8 (1.0 to 6.7) | 0.01 | 0.02 | |
0–2 | 4,962 | 32,310 | 20,036 | 6.2 | 3.0 (0.5 to 5.6) | 0.02 | −0.7 (−2.5 to 1.1) | 0.43 | 5.7 (2.6 to 8.8)* | <0.001 | <0.001 | |
0–3 | 4,965 | 32,354 | 20,046 | 6 | 4.1 (1.5 to 6.7) | 0.002 | 0.2 (−1.7 to 2.1) | 0.87 | 5.9 (2.6 to 9.3)* | <0.001 | 0.01 | |
0–4 | 4,966 | 32,373 | 20,060 | 5.9 | 4.9 (2.3 to 7.6) | <0.001 | 1.3 (−0.7 to 3.3) | 0.22 | 6.2 (2.7 to 9.8) | <0.001 | 0.03 | |
0–5 | 4,966 | 32,392 | 20,072 | 5.7 | 5.4 (2.7 to 8.1) | <0.001 | 2.1 (−0.1 to 4.2) | 0.06 | 6.4 (2.8 to 10.1) | <0.001 | 0.07 | |
0–6 | 4,966 | 32,404 | 20,085 | 5.4 | 5.4 (2.8 to 8.1) | <0.001 | 2.7 (0.5 to 4.9) | 0.02 | 5.5 (1.9 to 9.2) | 0.002 | 0.23 | |
![]() | 0 | 690 | 3,292 | 2880 | 6.2 | 0.1 (−5.6 to 6.1) | 0.98 | −0.4 (−4.7 to 4.0) | 0.85 | 7.7 (1.3 to 14.6) | 0.02 | 0.08 |
0–1 | 691 | 3,338 | 2896 | 6 | 0.1 (−5.9 to 6.5) | 0.97 | −0.6 (−5.3 to 4.2) | 0.79 | 7.1 (0.2 to 14.4) | 0.04 | 0.15 | |
0–2 | 704 | 3,384 | 2914 | 5.2 | −0.4 (−6.0 to 5.6) | 0.90 | −0.3 (−4.8 to 4.5) | 0.91 | 7.2 (0.5 to 14.3) | 0.03 | 0.12 | |
0–3 | 704 | 3,386 | 2917 | 5.5 | −2.3 (−8.4 to 4.2) | 0.48 | −1.5 (−6.5 to 3.9) | 0.59 | 6.5 (−1.1 to 14.7) | 0.10 | 0.13 | |
0–4 | 704 | 3,388 | 2918 | 5 | −2.4 (−8.3 to 4.0) | 0.46 | −1.1 (−6.3 to 4.3) | 0.68 | 5.2 (−2.2 to 13.2) | 0.17 | 0.22 | |
0–5 | 704 | 3,395 | 2920 | 5 | −1.7 (−7.9 to 4.9) | 0.60 | −0.7 (−6.2 to 5.0) | 0.80 | 5.0 (−2.7 to 13.4) | 0.21 | 0.33 | |
0–6 | 704 | 3,396 | 2920 | 5 | −1.6 (−8.1 to 5.3) | 0.64 | 0.4 (−5.5 to 6.7) | 0.90 | 3.3 (−4.8 to 12.1) | 0.44 | 0.59 |
Definition of abbreviations: CI=
confidence interval; IQR
=
interquartile range.
Note: models adjusted for temperature (4 df) and relative humidity using natural splines (4 df).




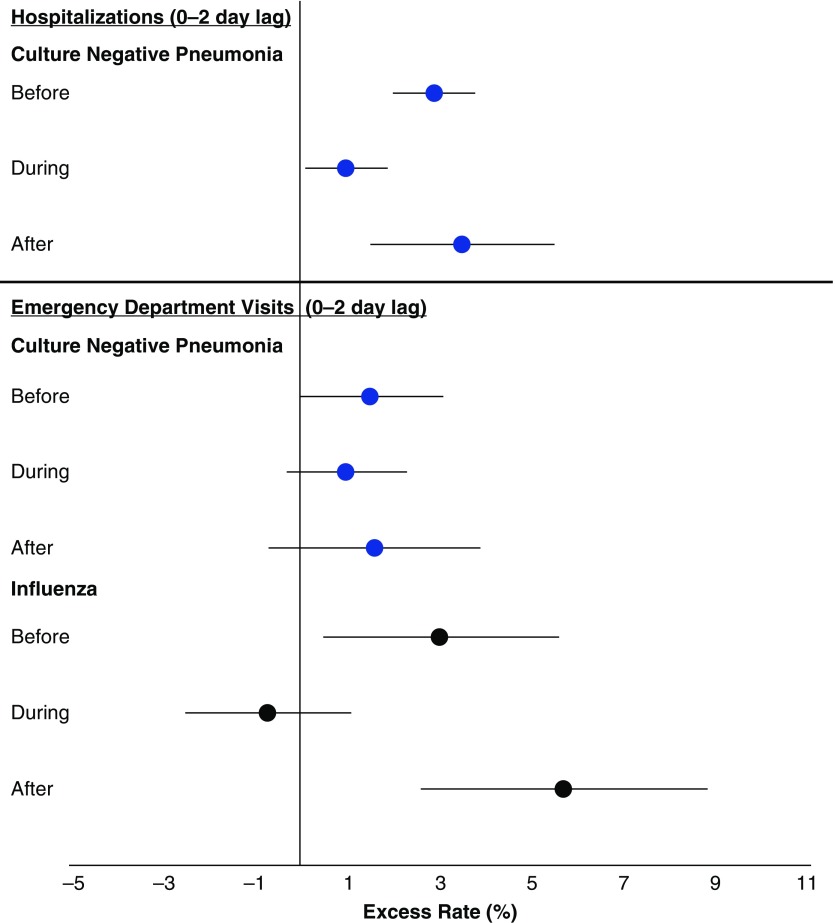
Excess rate (and 95% confidence interval [CI]) of hospitalizations and emergency department visits for culture-negative pneumonia and influenza associated with each interquartile range increase in concentration of fine particulate matter air pollution of 2.5 μm or less in diameter for the 0- to 2-day lag time by period.
Although inconsistent with the pattern of relative rates of influenza ED visits, the rate of influenza hospital admissions associated with increased PM2.5 concentrations was largest in the before period, with lower rates in the during and after periods. Although not significantly different, the rate of ED visits for bacterial pneumonia was substantially larger in the after period than in the during and before periods. There were no patterns in the relative rate of hospital admissions for bacterial pneumonia (Table 3).
Discussion
As hypothesized, we found that increased relative rates of hospital admissions and ED visits for culture-negative pneumonia (1–2%) were significantly associated with increased PM2.5 concentrations in the previous 2–7 days, whereas increased relative rates of influenza ED visits (3–4%), but not hospital admissions, were associated with increased PM2.5 concentrations in the previous 5–7 days. Increased relative rates of bacterial pneumonia hospitalizations were also associated with increased PM2.5 concentrations in the previous 1–7 days. These increased relative rates were independent of temperature and relative humidity changes, as well as subject characteristics (e.g., age, race, sex, socioeconomic status, previous health events) that were controlled by design in the case–crossover study.
A few studies have previously reported positive associations between short-term ambient air pollution exposures and hospitalizations for pneumonia (8), outpatient clinic visits for pneumonia (18), viral respiratory infections (5, 6), and general lower respiratory tract infections in subjects with asthma (19), with some associations reported in children (5, 6, 20) and others in adults (5, 8, 19). The Global Burden of Disease estimated a 50–100% increased risk of lower respiratory tract infection associated with 50- to 150-μg/m3 increases in PM2.5 concentration (9). A recent study of adults in the Wasatch Valley of Utah reported a 7% (95% CI, 4–11%) increased odds of lower respiratory tract infection associated with each 10-μg/m3 increase in PM2.5 in the previous 7 days (6). These results are consistent with our finding of an ER of hospitalization and ED visits for culture-negative pneumonia (1–2%) and ED visits for influenza (1–4%) (Table 2). The increased association of air pollution with ED visits for influenza in the previous 5–7 days is consistent with the clinical expectation that 95% of patients with influenza become symptomatic within 2 days (21) and may present for care over the next several days as symptoms worsen. A recent large epidemiologic study of respiratory disease and PM2.5 in four U.S. cities reported increased ERs (0.6–0.8%) of ED visits for respiratory disease (chronic obstructive pulmonary disease, upper respiratory infection, pneumonia, asthma and/or wheeze, and bronchiolitis) per IQR increase in PM2.5 in the previous 2 days. There were similar effect sizes for pneumonia ED visits associated with increased PM2.5 concentrations in the previous 1–4 days in each city (10). However, the lack of specificity in the type of infection makes comparisons between studies more difficult. Our inclusion of culture-negative pneumonia (in addition to bacterial pneumonia and influenza) may begin to address this issue. Culture-negative pneumonia is a common clinical diagnosis, due to the limitations in modern culture techniques to accurately establish a microbiologic diagnosis for pneumonia (14). Due to current diagnostic limitations, such as obtaining adequate samples, differentiating infection from colonization, and the difficulty of growing organisms on artificial media, making even a basic differentiation between broad categories of infection (bacterial vs. viral) is difficult (13).
We found the rate of culture-negative pneumonia hospitalizations associated with each 6.2-μg/m3 increase in PM2.5 concentration in the previous 3 days, and the rate of influenza ED visits associated with each 6.4-μg/m3 increase in PM2.5 concentration in the previous 3 days, were increased after air quality changes occurred from 2008–2013 (11) compared with the during period. Although concentrations of PM2.5 and several other pollutants subsequently decreased, changes in PM composition (e.g., proportionally more SOC) also occurred (12). Similarly, although pollutant concentrations generally decreased in a recent study in Los Angeles, increases in oxidant concentrations were also observed (22). The pattern of PM2.5/culture-negative pneumonia associations across time periods suggests that these changes in PM composition and air pollution mixture may indicate that, by some mechanism, the same mass of PM is more toxic in the after period than in earlier periods.
Mechanistically, air pollution’s negative impact on local airways leading to inflammation and disruption of the lung’s innate immune system, including mucociliary clearance, macrophage function, or epithelial barrier disruption, is well studied in cell and rodent models (23–25). The cellular signaling pathways of cytokine-mediated inflammation directed by specific signaling proteins (i.e., Toll-like receptors) appear to be a common response to both air pollution exposure and infectious pathogens, leading to the hypothesis that air pollution can alter the innate immune system’s response to infection (26). A study of diesel exhaust exposure’s effect on human respiratory epithelial cells observed an upregulation of the interferon (IFN) gene production via a Toll-like receptor pathway (27), indicating a downstream effect of particulate air pollution on the genetic response to viral infection (28). The increased IFN activity from PM2.5 exposure may lead to a priming effect on the immune system, thereby producing a more severe response to viral infections (26). Furthermore, a recent double-blind crossover study of diesel exhaust exposure in humans observed reductions in two antimicrobial peptides (α-defensin 1 and S100A7) in bronchoalveolar lavage fluid, indicating an impaired ability to resolve inflammation from infections (29). The potential for PM2.5 to increase the immune/inflammatory response to infection and decrease the ability to clear this inflammation is likely to lead to a severe course of infection requiring an ED visit or hospitalization. Further mechanistic research to elucidate the impact of specific components of PM2.5 on the innate immune response to infection is needed.
In our New York State study, SOC is one component observed in higher concentrations in the after period compared with the during period (12). SOCs are a PM2.5 component formed from the atmospheric oxidation of biogenic and anthropogenic volatile organic compounds to form reactive particles (30). In the online supplement, we describe the methods for estimating the concentrations of SOC (see Equation E1 and Figure E1) and the statistical analysis of the differences in concentrations among the periods (see Figures E2 and E3, Tables E3 and E4). Similar to overall PM2.5, the broad mechanism of SOC-mediated injury is thought to involve the creation or delivery of reactive oxygen species, leading to oxidative stress and inflammation in the lungs and other organs (31, 32). It is currently unknown to what degree the mechanism of SOC-induced injury overlaps with that of injury from other pollutants. Though speculative, the similar general pattern of relative rates of healthcare encounters for infection observed in our study to the pattern of SOC concentrations (both higher in the after period compared with the during period) may indicate that PM2.5 rich in SOC may alter the body’s immune response to infection.
Other possible explanations for the increased relative rates of health care encounters for influenza in the after period in relation to PM2.5 compared with earlier periods could be an increase in the virulence of infections, changes in the use or efficacy of the flu vaccine, or an overall change in clinical care. Despite stability in vaccination rates (33), the Influenza Vaccine Effectiveness Network has observed significant variability in the estimated efficacy of the flu vaccine each year (34), thought to be related to genetic changes (antigenic drifts and shifts), which change an individual strain’s virulence (35, 36). The nationwide trend for influenza infection over the course of the during and after periods of our study (2010–2016) was one of increasing rates of hospitalizations and total numbers of medical visits (37). Similarly, across New York State, the proportion of patients hospitalized for influenza infection increased from the before to after period (from 17% to 27%, respectively). Thus, the increasing virulence of influenza infection over time may contribute to the increased relative rate of influenza ED visits associated with PM2.5. An explanatory hypothesis is that, even if the impairment of the immune system is lessened in the setting of lower levels of PM2.5, the virulence of the organism may lead to a proportionally increased severity in course, increasing the relative rate of clinically significant infection over time. Finally, the trend of increasing numbers of ED visits and decreasing numbers of hospitalizations for culture-negative pneumonia and bacterial pneumonia may reflect an overall change in medical practice, in part due to accountable care organization–driven hospital policies facilitating treatment in the ED and discharge to outpatient care, rather than hospital admission (38).
Although this study could be generalized to most of the eastern half of the United States, where there were decreases in the burning of high sulfur bituminous coal resulting in reductions of SO2 concentrations (39), there were also several limitations to consider. First, although multiple national and New York State air quality and energy policies were implemented in the during period (2008–2013), a global recession also occurred in 2008. This economic downturn decreased demand for energy that, in turn, contributed to the reduction in NO2 levels in the United States (40). This economic downturn also added to the decreased air pollutant concentrations, due to a slowing of industrial production and reduced energy consumption (41). Thus, any change in the rate of respiratory infection associated with air pollutant concentrations cannot be attributed to individual policies or economic events. Second, all study subjects from each site were assigned the same PM2.5 concentrations for a specific day, regardless of how close they lived to a monitoring site, which likely resulted in exposure misclassification. This error is likely a combination of Berkson and classical error, resulting in a bias toward the null and underestimates of effect (42, 43). It is also possible that the observed differences in period-specific rate ratios may be due to differences in the degree of exposure misclassification and underestimation by period (i.e., the ambient PM2.5 concentration is a better proxy for individual subject’s PM2.5 exposure in the after period than the during period, resulting in a greater underestimation in the during period). Although similar patterns were not observed with all outcomes, future analyses are needed to investigate this further. Third, the diagnosis classification codes were changed mid-study from the 9th version of the ICD (ICD9) to the 10th version (ICD-10) on October 1, 2015 (in the after period). However, all ICD9 and ICD10 codes were reviewed by study physicians to ensure consistency of disease groups included and excluded from the study. Therefore, any outcome misclassification and downward bias should be minimal. To minimize the degree of overlap between case and control periods, we performed a standard case–crossover analysis limited to 7-day lag periods rather than including longer lag periods. In addition, we did not include multiple lag days of temperature and relative humidity in our models, and thus, it is possible that this, in part, could be an explanation for the smaller effect sizes observed at Lag Day 0 compared with other lag days. Finally, SOC values could only be estimated every third or sixth day, depending on the monitoring site, because of the sampling and analysis schedule and time. Thus, a comparable analysis with SOC is not possible with these data.
In summary, increased rates of culture-negative pneumonia healthcare encounters, ED visits for influenza and hospitalizations for bacterial pneumonia were associated with increased concentrations of PM2.5 over the previous few days. Changing pollutant mixtures, resulting from air quality policies and decreased energy demand and consumption during the recession, may have changed the toxicity of the PM2.5 in this study. The complex relationship between different types of respiratory infections and changing compositions of air pollution mixtures during and after periods of improved air quality requires further study.
Footnotes
Supported by New York State Energy Research and Development Authority agreements 59800, 59802, and 100412, and National Institutes of Environmental Health Sciences grant P30 ES01247; D.P.C. was supported by National Institutes of Health training grant T32-HL066988-1; D.P.C., S.W.T., P.K.H., M.M., S.S., E.v.W., M.J.U., and D.Q.R. are employed by the University of Rochester Medical Center, and W.Z. and S.L. are employed by the University at Albany, State University of New York.
The opinions expressed in this report do not necessarily reflect those of the New York State Energy Research and Development Authority or the State of New York.
Author Contributions: Study concept and design—D.P.C., W.Z., S.L., S.W.T., P.K.H., and D.Q.R.; acquisition, analysis, or interpretation of data—all authors; drafting of the manuscript—D.P.C., P.K.H., M.J.U., and D.Q.R.; critical review and revision of the manuscript—all authors; statistical analysis—W.Z., S.L., and S.W.T.; study supervision—D.P.C., S.L., P.K.H., and D.Q.R.
This article has an online supplement, which is accessible from this issue’s table of contents at www.atsjournals.org.
Author disclosures are available with the text of this article at www.atsjournals.org.
References
Articles from Annals of the American Thoracic Society are provided here courtesy of American Thoracic Society
Full text links
Read article at publisher's site: https://doi.org/10.1513/annalsats.201810-691oc
Read article for free, from open access legal sources, via Unpaywall:
https://europepmc.org/articles/pmc6394122?pdf=render
Citations & impact
Impact metrics
Article citations
A participatory study of indoor environment quality in homes of children and youth in Kanehsatake First Nation.
Sci Rep, 14(1):26022, 29 Oct 2024
Cited by: 0 articles | PMID: 39472467 | PMCID: PMC11522685
Comparison of the rate of healthcare encounters for influenza from source-specific PM<sub>2.5</sub> before and after tier 3 vehicle standards in New York state.
J Expo Sci Environ Epidemiol, 10 Aug 2024
Cited by: 0 articles | PMID: 39127830
Fine particulate matter manipulates immune response to exacerbate microbial pathogenesis in the respiratory tract.
Eur Respir Rev, 33(173):230259, 01 Jul 2024
Cited by: 0 articles | PMID: 39231594 | PMCID: PMC11372469
Review Free full text in Europe PMC
Is Pollution the Primary Driver of Infectious Syndemics?
Pathogens, 13(5):370, 30 Apr 2024
Cited by: 1 article | PMID: 38787222 | PMCID: PMC11124193
Review Free full text in Europe PMC
Seasonal extreme temperatures and short-term fine particulate matter increases pediatric respiratory healthcare encounters in a sparsely populated region of the intermountain western United States.
Environ Health, 23(1):40, 15 Apr 2024
Cited by: 1 article | PMID: 38622704 | PMCID: PMC11017546
Go to all (54) article citations
Data
Data behind the article
This data has been text mined from the article, or deposited into data resources.
BioStudies: supplemental material and supporting data
Lay summaries
Plain language description
Similar Articles
To arrive at the top five similar articles we use a word-weighted algorithm to compare words from the Title and Abstract of each citation.
PM<sub>2.5</sub> and its components and respiratory disease healthcare encounters - Unanticipated increased exposure-response relationships in recent years after environmental policies.
Environ Pollut, 360:124585, 20 Jul 2024
Cited by: 0 articles | PMID: 39038774
Associations between Source-Specific Particulate Matter and Respiratory Infections in New York State Adults.
Environ Sci Technol, 54(2):975-984, 18 Dec 2019
Cited by: 31 articles | PMID: 31755707 | PMCID: PMC6978840
Air pollution and acute respiratory infections among children 0-4 years of age: an 18-year time-series study.
Am J Epidemiol, 180(10):968-977, 16 Oct 2014
Cited by: 112 articles | PMID: 25324558 | PMCID: PMC4224364
Short-Term Elevation of Fine Particulate Matter Air Pollution and Acute Lower Respiratory Infection.
Am J Respir Crit Care Med, 198(6):759-766, 01 Sep 2018
Cited by: 152 articles | PMID: 29652174
Funding
Funders who supported this work.
NHLBI NIH HHS (1)
Grant ID: T32 HL066988
NIEHS NIH HHS (1)
Grant ID: P30 ES001247