Abstract
Importance
Few studies have assessed the effects of daily vitamin D doses at or above the tolerable upper intake level for 12 months or greater, yet 3% of US adults report vitamin D intakes of at least 4000 IU per day.Objective
To assess the dose-dependent effect of vitamin D supplementation on volumetric bone mineral density (BMD) and strength.Design, setting, and participants
Three-year, double-blind, randomized clinical trial conducted in a single center in Calgary, Canada, from August 2013 to December 2017, including 311 community-dwelling healthy adults without osteoporosis, aged 55 to 70 years, with baseline levels of 25-hydroxyvitamin D (25[OH]D) of 30 to 125 nmol/L.Interventions
Daily doses of vitamin D3 for 3 years at 400 IU (n = 109), 4000 IU (n = 100), or 10 000 IU (n = 102). Calcium supplementation was provided to participants with dietary intake of less than 1200 mg per day.Main outcomes and measures
Co-primary outcomes were total volumetric BMD at radius and tibia, assessed with high resolution peripheral quantitative computed tomography, and bone strength (failure load) at radius and tibia estimated by finite element analysis.Results
Of 311 participants who were randomized (53% men; mean [SD] age, 62.2 [4.2] years), 287 (92%) completed the study. Baseline, 3-month, and 3-year levels of 25(OH)D were 76.3, 76.7, and 77.4 nmol/L for the 400-IU group; 81.3, 115.3, and 132.2 for the 4000-IU group; and 78.4, 188.0, and 144.4 for the 10 000-IU group. There were significant group × time interactions for volumetric BMD. At trial end, radial volumetric BMD was lower for the 4000 IU group (-3.9 mg HA/cm3 [95% CI, -6.5 to -1.3]) and 10 000 IU group (-7.5 mg HA/cm3 [95% CI, -10.1 to -5.0]) compared with the 400 IU group with mean percent change in volumetric BMD of -1.2% (400 IU group), -2.4% (4000 IU group), and -3.5% (10 000 IU group). Tibial volumetric BMD differences from the 400 IU group were -1.8 mg HA/cm3 (95% CI, -3.7 to 0.1) in the 4000 IU group and -4.1 mg HA/cm3 in the 10 000 IU group (95% CI, -6.0 to -2.2), with mean percent change values of -0.4% (400 IU), -1.0% (4000 IU), and -1.7% (10 000 IU). There were no significant differences for changes in failure load (radius, P = .06; tibia, P = .12).Conclusions and relevance
Among healthy adults, treatment with vitamin D for 3 years at a dose of 4000 IU per day or 10 000 IU per day, compared with 400 IU per day, resulted in statistically significant lower radial BMD; tibial BMD was significantly lower only with the 10 000 IU per day dose. There were no significant differences in bone strength at either the radius or tibia. These findings do not support a benefit of high-dose vitamin D supplementation for bone health; further research would be needed to determine whether it is harmful.Trial registration
ClinicalTrials.gov Identifier: NCT01900860.Free full text

Effect of High-Dose Vitamin D Supplementation on Volumetric Bone Density and Bone Strength
Key Points
Question
Does higher-dose vitamin D supplementation improve bone mineral density (BMD, measured using high-resolution peripheral quantitative computed tomography) and bone strength (measured as failure load)?
Findings
In this randomized clinical trial that included 311 healthy adults, treatment with vitamin D for 3 years at a dose of 4000 IU per day or 10000 IU per day, compared with 400 IU per day, resulted in statistically significant lower radial BMD (calcium hydroxyapatite; −3.9 mg HA/cm3 and −7.5 mg HA/cm3, respectively); tibial BMD was significantly lower only with the daily dose of 10
000 IU. There were no significant differences in bone strength at either the radius or tibia.
Meaning
Among healthy adults, supplementation with higher doses of vitamin D did not result in improved bone health; further research would be needed to determine whether it is harmful.
Abstract
Importance
Few studies have assessed the effects of daily vitamin D doses at or above the tolerable upper intake level for 12 months or greater, yet 3% of US adults report vitamin D intakes of at least 4000 IU per day.
Objective
To assess the dose-dependent effect of vitamin D supplementation on volumetric bone mineral density (BMD) and strength.
Design, Setting, and Participants
Three-year, double-blind, randomized clinical trial conducted in a single center in Calgary, Canada, from August 2013 to December 2017, including 311 community-dwelling healthy adults without osteoporosis, aged 55 to 70 years, with baseline levels of 25-hydroxyvitamin D (25[OH]D) of 30 to 125 nmol/L.
Interventions
Daily doses of vitamin D3 for 3 years at 400 IU (n=
109), 4000 IU (n
=
100), or 10
000 IU (n
=
102). Calcium supplementation was provided to participants with dietary intake of less than 1200 mg per day.
Main Outcomes and Measures
Co-primary outcomes were total volumetric BMD at radius and tibia, assessed with high resolution peripheral quantitative computed tomography, and bone strength (failure load) at radius and tibia estimated by finite element analysis.
Results
Of 311 participants who were randomized (53% men; mean [SD] age, 62.2 [4.2] years), 287 (92%) completed the study. Baseline, 3-month, and 3-year levels of 25(OH)D were 76.3, 76.7, and 77.4 nmol/L for the 400-IU group; 81.3, 115.3, and 132.2 for the 4000-IU group; and 78.4, 188.0, and 144.4 for the 10000-IU group. There were significant group
×
time interactions for volumetric BMD. At trial end, radial volumetric BMD was lower for the 4000 IU group (−3.9 mg HA/cm3 [95% CI, −6.5 to −1.3]) and 10
000 IU group (−7.5 mg HA/cm3 [95% CI, −10.1 to −5.0]) compared with the 400 IU group with mean percent change in volumetric BMD of −1.2% (400 IU group), −2.4% (4000 IU group), and −3.5% (10
000 IU group). Tibial volumetric BMD differences from the 400 IU group were −1.8 mg HA/cm3 (95% CI, −3.7 to 0.1) in the 4000 IU group and −4.1 mg HA/cm3 in the 10
000 IU group (95% CI, −6.0 to −2.2), with mean percent change values of −0.4% (400 IU), −1.0% (4000 IU), and −1.7% (10
000 IU). There were no significant differences for changes in failure load (radius, P
=
.06; tibia, P
=
.12).
Conclusions and Relevance
Among healthy adults, treatment with vitamin D for 3 years at a dose of 4000 IU per day or 10000 IU per day, compared with 400 IU per day, resulted in statistically significant lower radial BMD; tibial BMD was significantly lower only with the 10
000 IU per day dose. There were no significant differences in bone strength at either the radius or tibia. These findings do not support a benefit of high-dose vitamin D supplementation for bone health; further research would be needed to determine whether it is harmful.
Trial Registration
ClinicalTrials.gov Identifier: NCT01900860
Introduction
Vitamin D supplementation has been considered beneficial for prevention and treatment of osteoporosis. Clinical trial data support skeletal benefits of vitamin D supplementation in persons with circulating 25-hydroxyvitamin D (25[OH]D) levels of less than 30 nmol/L.1,2 However, recent meta-analyses do not support a major treatment benefit of vitamin D for osteoporosis3,4 or preventing falls4 or fractures.4,5 Most vitamin D supplementation recommendations range from 400 to 2000 IU daily6,7 with a tolerable upper intake level of 4000 IU to 10000 IU6,8. Between 2013 and 2014, three percent of US adults consumed daily doses of at least 4000 IU of vitamin D9; one study assessed the effects of daily doses of greater than 4000 IU for at least 12 months, finding 6500 IU no different than 800 IU for changes in bone mineral density (BMD).10
The clinical diagnosis of osteoporosis and prediction of fracture risk relies on dual x-ray absorptiometry (DXA) scans measuring areal BMD at different skeletal sites. However, bone strength, an important component of fracture risk, is determined by a combination of bone mass, morphology, and microarchitecture.11 High-resolution peripheral quantitative computed tomography (HR-pQCT) has higher resolution and sensitivity than DXA and assesses compartmental volumetric bone density, microarchitecture, and estimates of bone strength. In a prospective study with 7254 participants, HR-pQCT parameters were associated with fracture risk independently of DXA-measured areal BMD.11 Vitamin D effects on the skeleton have not been prospectively assessed using HR-pQCT.
Motivated by the prevalence of high-dose vitamin D supplementation among healthy adults, this study explored the dose-response effects of daily vitamin D supplementation (400, 4000, 10000 IU) on total volumetric BMD and bone strength in healthy community-dwelling adults over 3 years, while ensuring adequate calcium intake. It was hypothesized that a higher dose of vitamin D has a positive effect on HR-pQCT measures of volumetric density and strength, perhaps via suppression of parathyroid hormone (PTH)–mediated bone turnover.
Methods
Study Design and Oversight
This was a single-center, 3-year, double-blind, randomized clinical trial with 3 parallel groups (400, 4000, or 10000 IU vitamin D3 daily), undertaken between August 2013 and December 2017 (trial protocol in Supplement 1 and statistical analysis plan in Supplement 2). Participants were recruited from the general population by means of letter, posters, and public media.12 The trial was approved by the Conjoint Health Research Ethics Board at the University of Calgary and Health Canada. Each participant provided written informed consent before randomization.
Participants
Healthy men and women aged 55 to 70 years were eligible if they had a DXA lumbar spine and total hip areal BMD T score greater than −2.5 SD, serum 25(OH)D between 30 and 125 nmol/L, and normal serum calcium (2.10-2.55 mmol/L). Participants were excluded if their screening serum 25(OH)D was less than 30 nmol/L or greater than 125 nmol/L; serum calcium was greater than 2.55 mmol/L or less than 2.10 mmol/L; or vitamin D supplement use was greater than 2000 IU per day for the past 6 months. Further exclusions included use of bone active medication within the last 2 years; disorders known to affect vitamin D metabolism such as sarcoidosis, renal failure, malabsorption disorders, kidney stone within the past 2 years, or regular use of tanning salons; DXA T score compatible with osteoporosis at the lumbar spine, total hip or femoral neck; and high 10-year risk (≥20%) for osteoporotic fracture (as defined by the World Health Organization's Canadian FRAX calculator). Participants with DXA T scores between −1.0 and −2.5 (low bone density) were not excluded. Participant-reported race/ethnicity (non-Hispanic white, non-Hispanic black, Asian, Hispanic) was captured due to possible differences in vitamin D metabolism.13
Randomization and Masking
A statistician unrelated to the trial generated a randomization table, assigning participants in 1:1:1 ratio to receive cholecalciferol (vitamin D3) 400, 4000, or 10000 IU orally once daily, stratified by sex. Randomization was performed within sex using simple randomization in block sizes of 60 or 30 and were not revealed to the research team prior to allocation. Participants and the research team were blinded to allocation.
Procedures
Participants were prescribed vitamin D3 liquid drops (5 drops/d) orally. Preparations (80 IU/drop, 800 IU/drop, or 2000 IU/drop) were provided and checked for quality by Ddrops Canada. Adherence to treatment was accessed from daily diaries and calculated as number days of supplement administration vs total number of days of follow-up, expressed as a percentage.
Participants were requested to take no more than 200 IU per day of additional vitamin D (eg, a multivitamin supplement). Because the estimated median contribution of vitamin D from diet is approximately 200 IU,6 the participants randomized to 400 IU per day received the recommended dietary allowance of 600 IU for adults younger than age 70 years.6 Calcium intake was assessed by a food frequency questionnaire.14 Participants not consuming the recommended dietary allowance of calcium (1200 mg/d)15 received calcium citrate tablets (each containing 300 mg elemental calcium) as needed (≤maximum of 600 mg/d) to approximate a total daily intake of 1200 mg.
Outcomes
Primary outcome variables (Supplement 1) were based on HR-pQCT measurements of total volumetric BMD at the distal radius and tibia and bone strength (failure load) by finite element analysis at the distal radius and tibia for a total of 4 co-primary variables. Observation of a significant benefit at 1 skeletal site in either volumetric BMD or bone strength was regarded as a positive result at that site. Secondary bone outcomes included total hip areal BMD by DXA, HR-pQCT measures of cortical and trabecular volumetric BMD, cortical porosity, and trabecular number. Balance (postural sway), physical function (timed up and go; grip strength) and quality of life (SF36 questionnaire) were additional secondary outcomes. Safety outcomes, defined a priori, were as follows: all serious adverse events, hypercalcemia, hypercalciuria, renal dysfunction, nephrolithiasis, hepatic dysfunction, falls, fragility fractures, and cancer.
Total volumetric BMD and bone microarchitecture were assessed by HR-pQCT (nominal isotropic voxel of 60.7 μm, XtremeCT II, Scanco Medical) at baseline, 6, 12, 24, and 36 months. Three-dimensional image registration was applied to ensure the same region of interest was assessed longitudinally.16 Failure load was estimated by finite element analysis (FAIM, version 8.0, Numerics88 Solutions). Areal BMD was measured annually by DXA (GE Lunar iDXA, GE Healthcare, enCORE v16). Precision scores for HR-pQCT range from less than 2.4% for density to greater than 3.3% for microarchitecture parameters, with the exception of cortical porosity (<13.7%).17 DXA precision is 0.55% coefficient of variation for the total hip.
Postural sway was assessed (Biosway machine, 950-460, Biodex) on a foam surface with eyes closed.18 Grip strength was measured using the Jamar 200-lb hand dynamometer (12-0600).19 A standard assessment of timed up and go was evaluated.20 Participants completed a self-administered SF-36 v2 to assess quality of life.21 Assessments of physical function and questionnaires were completed during annual visits.
All blood and urine clinical chemistry measures were performed at Calgary Laboratory Services (CLS) as fasting morning samples and processed in real time. Serum 25(OH)D was measured by DiaSorin Liaison XL system; CLS participates in the Vitamin D External Quality Assessment Scheme (DEQAS). Serum intact PTH was measured using the DiaSorin Liaison 1–84 PTH assay. C-telopeptide of type 1 collagen (CTx) was measured by Cobas e immunoassay analyzer ECLIA. Mean immunoassay coefficient of variation at CLS was 2.5% to 8.6% for 25(OH)D, 6.0% to 7.1% for PTH, and 3.9% to 4.2% for CTx. To ensure the avoidance of vitamin D toxicity, as manifested by hypercalciuria or hypercalcemia, calcium and creatinine were assessed via blood and urine collection (24-hour urine at baseline and months 12, 24, and 36, second void urine sample at months 6, 18, and 30) and monitored by the physician investigators (E.O.B. and D.A.H.) throughout the study.
At each visit, participants reported new or ongoing medical issues and hospitalizations in an interview-style discussion with a member of the study team. Serious adverse events were defined as events that were fatal or life-threatening, or which resulted in hospitalization or prolongation of existing hospital stay, or caused persistent or significant incapacity or disability. Serious adverse events were adjudicated by the study physicians, and falls and fractures were adverse events of special interest. Fractures of the fingers and toes and traumatic fractures were not included. Fractures were reviewed by study clinicians via participant medical records and x-ray reports where available. Low-trauma (fragility) fractures were defined as resulting from low trauma, such as a fall from standing height or less.
Statistical Analysis
Sample size estimation was based on primary outcome variables of total volumetric BMD at the tibia and radius. The 4 co-primary outcome variables are highly correlated and therefore each variable was tested at the α level of .025. In a population-based prospective cohort of postmenopausal women, an annual decrease of approximately 1.8% was observed over 3 years for radial total volumetric BMD.22 Assigning the 400-IU group a more conservative annual decline of 1.2% (values greater than 1.2% would require a smaller sample size), the minimal clinically relevant benefit for the 4000-IU group would be improving the rate of decline by 50% or more (1.2%-0.6% annually) and for the 10000-IU group arresting the rate of decline to 0%, (Supplement 2). Based on sample size calculations and allowing for 20% attrition, a total of 300 participants gave 90% power at an α level of .025. All P values were 2-sided and a P value of less than .05 was set for secondary variables and safety parameters. Because of the potential for type 1 error due to multiple comparisons, findings for analyses of secondary end points should be interpreted as exploratory.23
Participants who received at least 1 dose of study drug and at least 1 follow-up visit were included in the primary analysis. A constrained linear mixed-effects model24 was used to analyze each outcome variable. Fixed effects included treatment group, a quadratic effect of time if warranted, and a treatment×
time interaction, which if statistically significant, indicated a significant treatment effect. Random effects included time in which both intercept and slope could vary. The correlation within participants was modeled as an autoregressive error of order 1. Missing data were accounted for by using the mixed-effects model.25
All participants, irrespective of number of follow-up visits, were included in the safety analysis. Safety outcomes were reported as the proportion of participants in each group who experienced serious and prespecified adverse events. Adverse events were examined formally (provided the overall prevalence of the adverse event was ≥4% and ≤96%) for between-treatment group differences for trend in proportions using logistic regression.26 The adverse event (yes or no) was the binary outcome variable and the dose (400 IU, 4000 IU, or 10000 IU) was the explanatory variable in this simple logistic regression.
Analyses were conducted using the R project for Statistical Computing (R, version 3.4). An independent data safety and monitoring board oversaw the study.
Results
The flow of participants is presented in Figure 1. A total of 542 participants were screened, 311 met inclusion criteria and were randomized, and 287 (92%) completed all aspects of the 3-year trial. The primary analyses included 303 participants. Baseline demographic information is shown in Table 1. The cohort was 53% men, and predominantly white (95%). Classified by DXA T score, participants had normal (81%) or low (19%) total hip areal BMD.
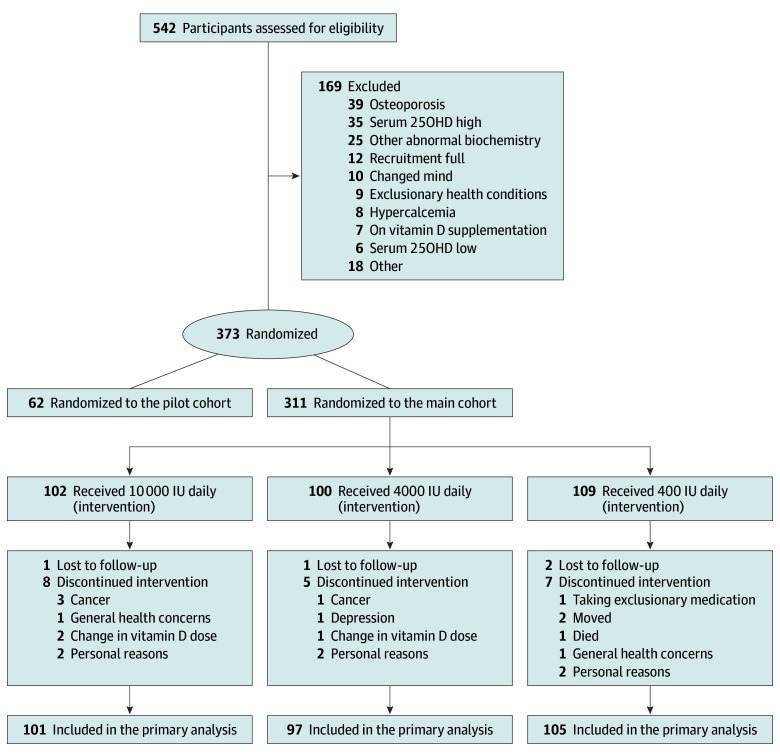
Participants who received at least 1 dose of study drug and underwent at least 1 follow-up measurement were included in the primary analyses.
Table 1.
Variable | No. (%) | ||
---|---|---|---|
10![]() | 4000 IU | 400 IU | |
No. of participants | 101 | 97 | 105 |
Demographics | |||
Sex | |||
Men | 53 (52.5) | 50 (51.5) | 60 (57.1) |
Women | 48 (47.5) | 47 (48.5) | 45 (42.9) |
Age, mean (SD). y | 61.9 (4.1) | 62.6 (4.3) | 62.2 (4.2) |
Years since menopause, mean (SD), y | 12.5 (5.6) | 11.7 (7.3) | 12.6 (5.9) |
BMI, mean (SD)a | 27.1 (4.1) | 28.1 (5.0) | 27.7 (4.3) |
Body fat, mean (SD), % | 32.2 (8.4) | 34.0 (8.9) | 33.3 (9.0) |
Race/ethnicity | |||
Non-Hispanic white | 98 (97.0) | 94 (96.9) | 98 (93.3) |
Non-Hispanic black | 0 | 1 (1.0) | 1 (1.0) |
Asian | 2 (2.0) | 2 (2.1) | 5 (4.7) |
Hispanic | 1 (1.0) | 0 | 1 (1.0) |
Health characteristics, mean (SD) | |||
Systolic blood pressure, mm Hg | 125.6 (16.0) | 131.6 (16.7) | 128.5 (15.4) |
Diastolic blood pressure, mm Hg | 80.4 (10.5) | 80.8 (8.3) | 81.1 (7.7) |
Vitamin D supplementationb | 34 (34) | 38 (39) | 32 (30) |
Medical history | |||
Skin cancerc | 15 (14.9) | 7 (7.2) | 12 (11.4) |
Other cancer | 10 (9.9) | 7 (7.2) | 5 (4.8) |
Cardiovascular-related diagnoses | 13 (12.9) | 10 (10.3) | 21 (20.0) |
Type 2 diabetes | 3(3.0) | 4 (4.1) | 2 (1.9) |
Rheumatoid arthritis | 1 (1.0) | 2 (2.1) | 1 (1.0) |
Asthma | 9 (8.9) | 6 (6.2) | 5 (4.8) |
Smoker | 4 (4.0) | 0 | 2 (1.9) |
Fallsd | 18 (17.8) | 20 (20.6) | 22 (21.0) |
Fracture after age 50 y | 17 (16.8) | 13 (13.4) | 17 (16.2) |
Lumbar spine T scoree | 0.1 (1.5) | 0.2 (1.3) | 0.0 (1.5) |
Total hip T scoref | 0.0 (1.1) | 0.2 (1.1) | 0.1 (1.1) |
Laboratory values | |||
Serum | |||
25(OH)D, nmol/L | 78.4 (18.4) | 81.3 (20.1) | 76.7 (21.0) |
PTH, ng/L | 22.1 (7.4) | 21.5 (6.6) | 22.2 (7.7) |
Calcium, mmol/L | 2.4 (0.1) | 2.4 (0.1) | 2.4 (0.1) |
Phosphate, mmol/L | 1.0 (0.1) | 1.0 (0.2) | 1.0 (0.2) |
Creatinine, μmol/L | 80.1 (14.4) | 79.7 (15.1) | 80.7 (13.9) |
Alkaline phosphatase, U/L | 70.1 (17.1) | 65.8 (15.3) | 69.1 (18.1) |
Estimated GFR, mL/min/1.73m2 | 80.2 (11.4) | 80.3 (12.0) | 80.7 (11.2) |
24-h Urine calcium, mmol/d | 4.2 (2.0) | 4.8 (2.1) | 4.2 (2.0) |
Plasma CTx (β-crosslaps), ng/Lg | 344.9 (126.6) | 339.4 (130.7) | 335.5 (118.8) |
Fasting blood glucose, mg/dL | 102.6 (12.6) | 102.6 (5.4) | 102.6 (5.4) |
Hemoglobin A1C, % | 5.2 (1.6) | 5.1 (0.6) | 5.1 (0.8) |
Abbreviations: BMI, body mass index; CTx, C-telopeptide of type 1 collagen (β-crosslaps); GFR, glomerular filtration rate; PTH, parathyroid hormone; 25(OH)D, 25-hydroxyvitamin D.
Eleven participants discontinued the study supplement but continued follow-up (400 IU, 2; 4000 IU, 3; 10000 IU, 6). Reasons for discontinuing included starting or stopping hormone therapy, concerns with kidney function or stones, decision to take other vitamin D supplementation, and possible hyperparathyroidism. The mean vitamin D supplementation adherence was 99% (range, 81%-100%) for participants who completed the trial on study supplement. There were no significant differences in adherence between treatment groups. Mean serum 25(OH)D measurements did not change for the 400-IU group from baseline through 36 months (76.3-77.4 nmol/L). A significant increase in mean serum 25(OH)D from baseline was seen in the 4000-IU group at 3 months (81.3-115.3 nmol/L) with a further increase to 132.2 nmol/L by month 36. The baseline to 3 month increase in the 10000-IU group (78.4-188.0 nmol/L), increased to 200.4 nmol/L at 18 months but fell to 144.4 nmol/L at 36 months (Figure 2; eTable 1 and eFigure 1 in Supplement 3). In addition, many study participants (76%) received calcium supplements throughout the study; more than half (65%) took a single 300 mg tablet per day.
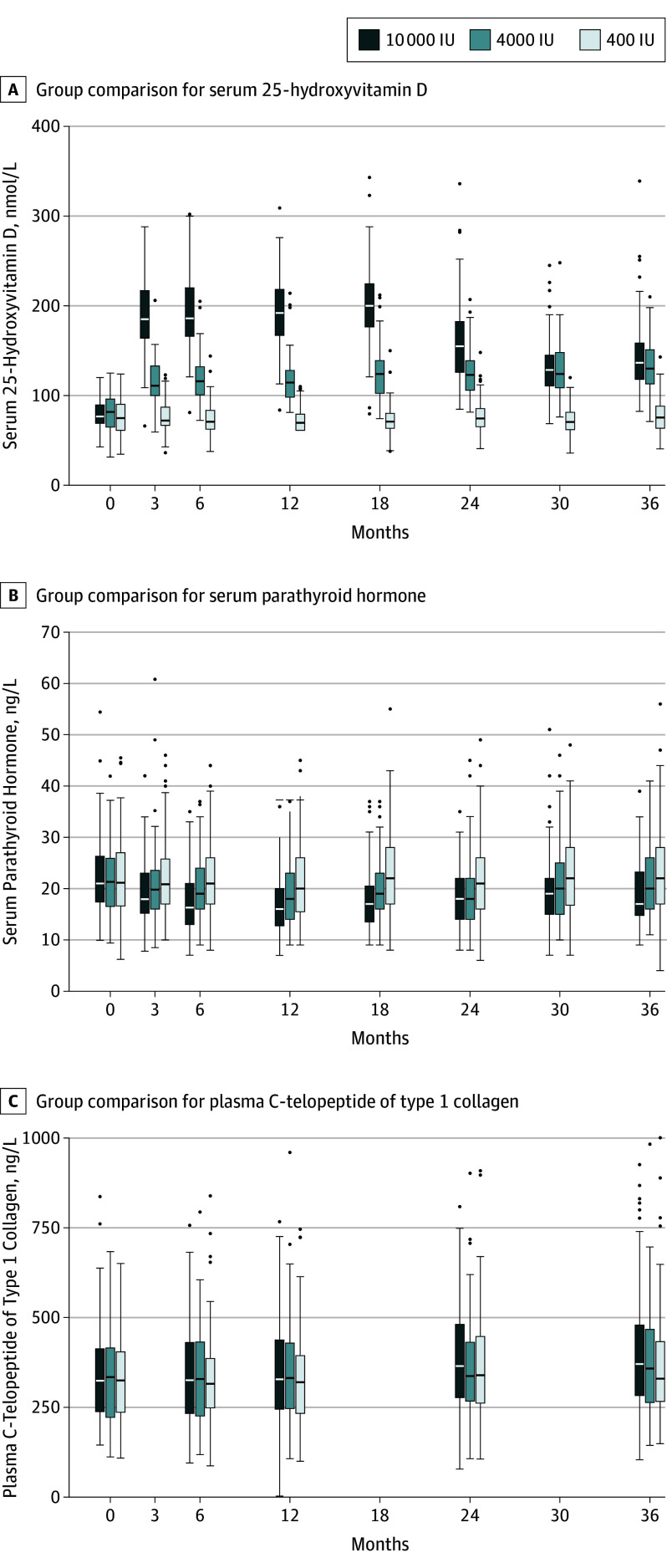
The horizontal line in the middle of each box indicates the median, the top and bottom borders of the box mark the 75th and 25th percentiles, the whiskers above and bolow the box indicate the 90th and 10th percentiles, and the points beyond the whiskers are outliers beyond the 90th or 10th percentiles.
Some participants had missing HR-pQCT data due to motion artifact (12 participants had at least 1 radius scan removed) or inadequate (<75%) overlap in scan region across all study visits (13 participants had radius scans at one time point removed). One participant had all radius scans removed due to motion and inadequate scan region overlap.
Primary Outcomes
Data distribution and changes in volumetric bone density and strength are displayed in Figure 3. Group×
time interactions were significant in total volumetric BMD at the tibia (P
<
.001) and radius (P
<
.001). At both sites, total volumetric BMD displayed a negative dose-response relationship (Figure 3; eTable 2 in Supplement 3)—greater losses associated with higher dose of vitamin D supplementation. At trial end, the difference between the 4000 and 400 IU group was −3.9 mg hydroxyapatite (HA)/cm3 (95% CI, −6.5 to −1.3) and between the 10
000 and 400 IU group was −7.5 mg HA/cm3 (95% CI, −10.1 to −5.0) for total volumetric BMD at the radius, based on mixed-model analysis. This is equivalent to changes from the mean baseline values of −1.2% (400-IU group), −2.4% (4000-IU group) and −3.5% (10
000-IU group) at the radius. For mixed-model analysis of total volumetric BMD at the tibia, the equivalent differences between groups were −1.8 mg HA/cm3 (95% CI, −3.7 to 0.1; not statistically significant) in the 4000-IU group and −4.1 mg HA/cm3 (95% CI, −6.0 to −2.2) in the 10
000-IU group; equivalent mean changes from baseline were −0.4% (400 IU-group), −1.0% (4000 IU-group) and −1.7% (10
000 IU-group). Bone strength decreased over trial duration; however, group
×
time interactions were not significant (radius, P
=
.06; tibia, P
=
.12).
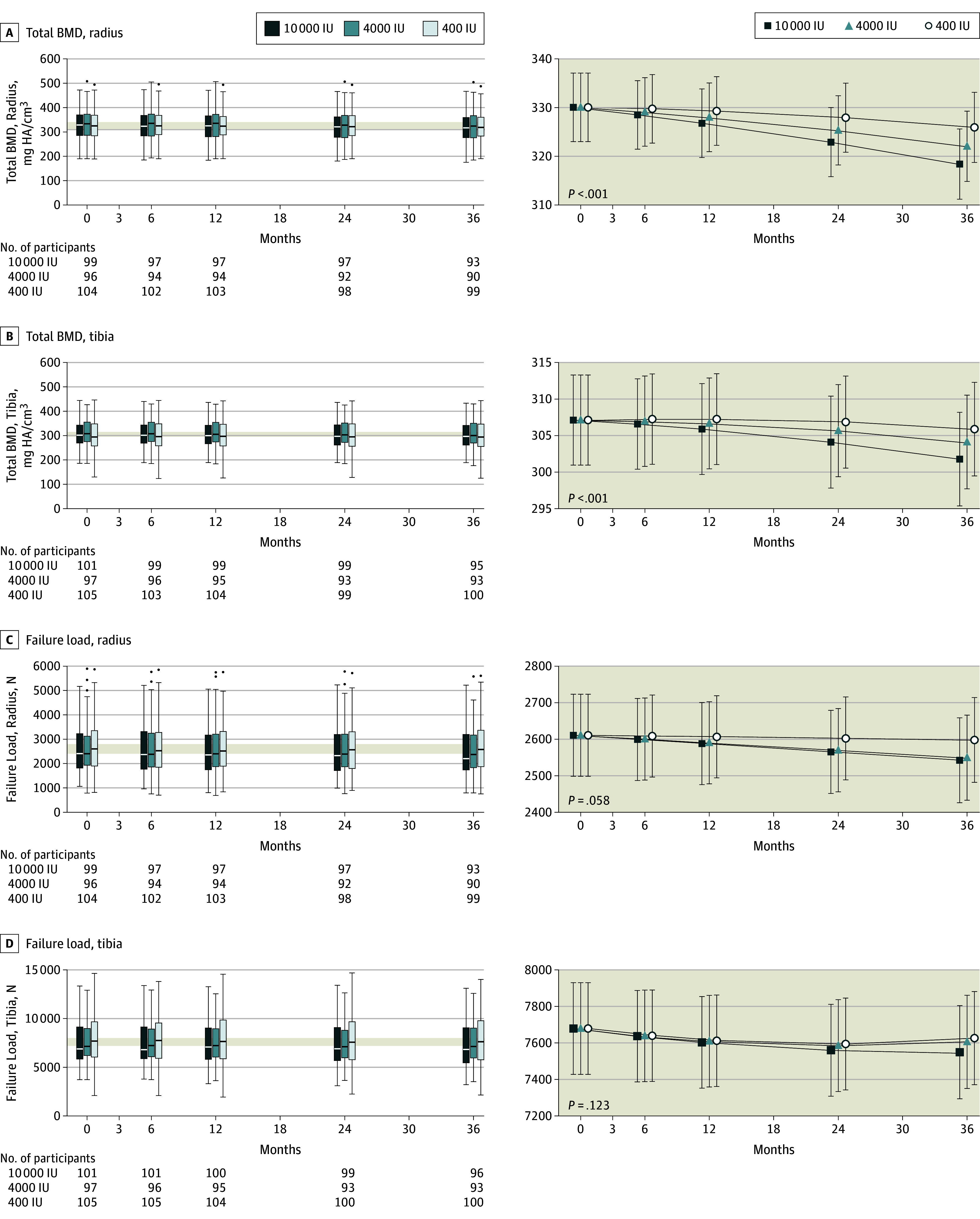
The horizontal line in the middle of each box (left column) indicates the median, the top and bottom borders of the box mark the 75th and 25th percentiles, the whiskers above and bolow the box indicate the 90th and 10th percentiles, and the points beyond the whiskers are outliers beyond the 90th or 10th percentiles. The modeled data (right column) show the mean 95% CIs for the predicted values using the random-effects model. P values indicate the group×
time interaction effect.
Secondary Outcomes
For secondary outcomes, the point estimates represent the difference for 4000 or 10000 IU compared with the 400 IU at 36 months. There were significant group
×
time interactions for cortical volumetric BMD of the radius (P
=
.001; −6.3 [95% CI, −11.1 to −1.5] for 4000 IU and −8.6 [95% CI, −13.3 to −3.8] for 10
000 IU) and tibia (P
=
.004; −4.2 [95% CI, −9.8 to 1.2] for 4000 IU and −9.8 [95% CI, −14.8 to −4.0] for 10
000 IU), for trabecular volumetric BMD of the radius (P
=
.01; −0.95 [95% CI, −2.6 to 0.7] for 4000 IU and −2.71 [95% CI, −4.3 to −1.1] for 10
000 IU; eFigure 2 and eFigure 3 in Supplement 3), and for trabecular number for the radius (P
=
.01; 0.003 [95% CI, −0.013 to 0.018] for 4000 IU and −0.019 [95% CI, −0.035 to −0.004] for 10
000 IU; eTable 3, eFigure 4, and eFigure 5 in Supplement 3). As observed with total volumetric BMD, cortical BMD results also demonstrated larger losses in bone density associated with higher dose of vitamin D at both sites. Trabecular volumetric BMD increased over trial duration with the 10
000-IU group gaining the least amount of bone density at the radius.
Group×
time interactions were not statistically significant for trabecular volumetric BMD of the tibia (P
=
.13; 0.20 [95% CI, −0.1 to 1.5] for 4000 IU and −1.1 [95% CI, −2.4 to 0.2] for 10
000 IU), cortical porosity of the radius (P
=
.37; 0.06 [95% CI, −0.025 to 0.15] for 4000 IU and 0.04 [95% CI, −0.043 to 0.13] for 10
000 IU) and tibia (P
=
.89; 0.01 [95% CI, −0.17 to 0.19] for 4000 IU and 0.04 [95% CI, −0.13 to 0.22] for 10
000 IU), and trabecular number for the tibia (P
=
.20; −0.01 [95% CI, −0.03 to 0.01] for 4000 IU and −0.02 [95% CI, −0.04 to 0.004] for 10
000 IU).
DXA total hip areal BMD remained stable over the duration of the trial with no significant differences between groups (P=
.87; 0.0007 [95% CI, −0.0064 to 0.0078] for 4000 IU and 0.0019 [95% CI, −0.0052 to 0.0090] for 10
000 IU; eTable 3 and eTable 4 in Supplement 3). Differences between all 3 treatment groups at 3 years were less than 0.2%. There were no significant changes in balance (P
=
.12; −0.005 [95% CI, −0.09 to 0.08] for 4000 IU and −0.08 [95% CI, −0.17 to 0.004] for 10
000 IU), grip strength (P
=
.92; −0.12 [95% CI, −1.4 to 1.1] for 4000 IU and −0.26 [95% CI, −1.5 to 1.0] for 10
000 IU), timed up-and-go (P
=
.27; 0.38 [95% CI, −0.11 to 0.86] for 4000 IU and 0.31 [95% CI, −0.18 to 0.79] for 10
000 IU), or quality of life (P
=
.57 for the mental component summary; 0.70 [95% CI, −0.8 to 2.2] for 4000 IU and 0.71 [95% CI, −0.8 to 2.2] for 10
000 IU).
PTH decreased between baseline and month 18 and was lowest in the 10000-IU group (eFigure 1 in Supplement 3). CTx increased throughout the study, being highest in the 10
000-IU group (eFigure 1 in Supplement 3).
After study completion and removal of blinding, a decline in 25(OH)D was detected in the 10000-IU group at the 24-month assessment. Upon investigation, it was determined that without the knowledge of investigators, Ddrops changed its manufacturing laboratory source, and the long-term stability of the 2000 IU per drop solution was reduced. This change began affecting the 10
000-IU group between 18 and 24 months (depending on participant progress through the study). With independent third-party testing and information from Ddrops, participants began receiving a dose estimated to vary between 2000 and 10
000 IU per day. Despite reduced supplementation, mean 25(OH)D levels remained higher than the 4000-IU group throughout the study.
Prespecified safety outcomes are shown in Table 2. In total, 44 serious adverse events occurred in 38 (12.2%) participants. One death (presumed myocardial infarction) occurred in a participant in the 400-IU group. Among the prespecified adverse events, only hypercalcemia (P=
.005) and hypercalciuria (P
=
.006) demonstrated a significant dose-response effect. All episodes of hypercalcemia were mild (2.56-2.64 mmol/L) and resolved on follow-up testing. One participant in the 10
000-IU group experienced 2 episodes of transient hypercalcemia (months 6 and 30). There was no significant difference in falls detected among the 3 groups.
Table 2.

10![]() ![]() ![]() | 4000 IU (n![]() ![]() | 400 IU (n![]() ![]() | ||||
---|---|---|---|---|---|---|
Total Events, No. | Participants With Events, No. (%) | Total Events, No. | Participants With Events, No. (%) | Total Events, No. | Participants With Events, No. (%) | |
Serious adverse eventsb | 14 | 14 (14) | 10 | 8 (8) | 20 | 16 (15) |
Hypercalcemiac | 10 | 9 (9) | 4 | 4 (4) | 0 | 0 |
Hypercalciuriad | 54 | 34 (33) | 34 | 22 (22) | 25 | 19 (17) |
Renal dysfunctione | 4 | 2 (2) | 2 | 2 (2) | 1 | 1 (1) |
Nephrolithiasis | 2 | 1 (1) | 1 | 1 (1) | 0 | 0 |
Hepatic dysfunctionf | 3 | 3 (3) | 3 | 3 (3) | 8 | 5 (5) |
Falls | 6 | 5 (5) | 11 | 10 (10) | 4 | 4 (4) |
Low-trauma fracturesg | 5 | 5 (5) | 3 | 3 (2) | 4 | 4 (4) |
Cancerh | 5 | 5 (5) | 3 | 3 (3) | 1 | 1 (1) |
Abbreviations: ALT, alanine aminotransferase; AST, aspartate aminotransferase; ULN, upper limit of normal.
SI units: to convert ALT and AST to μkat/L, multiply by 0.0167.


Discussion
This 3-year randomized clinical trial examined the effect of 3 daily doses of vitamin D: 400 IU, 4000 IU (the National Academy of Medicine [formerly the Institute of Medicine {IOM}] tolerable upper intake level), and 10000 IU in healthy adults aged 55 to 70 years and failed to find a positive effect of vitamin D on volumetric BMD and estimated bone strength, as measured by HR-pQCT at the radius and tibia.
Instead of the hypothesized increase, a negative dose-response relationship was observed for volumetric BMD. Using the 400-IU group as a reference point, high-dose vitamin D supplementation (10000 IU/d) was associated with a significantly greater loss of bone. Because these results are in the opposite direction of the research hypothesis, this evidence of high-dose vitamin D having a negative effect on bone should be regarded as hypothesis generating, requiring confirmation with further research. Therefore, the appropriate interpretation of this study is that for maintenance of bone quality in healthy vitamin D–sufficient adults, these results do not support a skeletal benefit of vitamin D doses well above the recommended dietary allowance.6
If the observed vitamin D dose-dependent loss of volumetric BMD seen in this trial represents a real effect, it might be related to the observed combination of an increased plasma marker of bone resorption (CTx) and suppression of PTH seen in the 10000-IU group. High-dose vitamin D without extra calcium supplementation has been associated with increased levels of the active vitamin D metabolite 1, 25(OH)2 vitamin D (calcitriol), and an increase in CTx.27 Calcitriol stimulates osteoclastogenesis and differentiation, either through action on osteoblasts28 or by direct action on osteoclast precursors.29 High-dose vitamin D may also suppress PTH by direct action on parathyroid cells or indirectly by enhancing intestinal calcium absorption. This might reduce PTH-mediated bone formation, which when combined with a vitamin D–mediated direct effect on osteoclast activity (as supported by the trend to higher CTx in the 10
000-IU group), could result in the dose-related accelerated decline in observed volumetric BMD. The observation of an increased trabecular volumetric BMD while cortical volumetric BMD declined may reflect this increase in resorption, leading to trabecularization of the endocortical surface of the cortex.
DXA findings in this study align with others showing nonsignificant changes in areal BMD at the total hip following high-dose vitamin D supplementation10,30,31,32 and no additional benefit for high compared with low-dose supplementation.10,31,32,33 These results are supported by a recent meta-analysis that did not support a public health recommendation for vitamin D supplementation for the prevention of falls, fractures, or beneficial effects on bone density.4
There is growing evidence that for bone, the benefit of vitamin D supplementation is only seen in the treatment of vitamin D deficiency.1,2 There is also evidence that very high intermittent (monthly or annual) doses of vitamin D may be harmful, with increased risk of falls or fracture34,35; but not all such studies report increased risk of falls or fractures.36 There was no effect on fall rates in this study and no evidence of a relationship between changes in volumetric BMD and fracture risk, which may reflect the healthy status of the population that was studied. However, if high-dose vitamin D does stimulate an increased rate of bone loss, this could have greater clinical significance in older individuals with osteoporosis.
Most of the prespecified safety outcomes occurred in all 3 vitamin D treatment groups. However, episodes of hypercalcemia and hypercalciuria were more common with increasing vitamin D dose, consistent with previous reports,37 and the risk of hypercalcemia and hypercalciuria is higher in individuals taking calcium supplementation,38 suggesting that supplemental calcium intake may be a significant driver of hypercalcemia in individuals taking vitamin D. The dose-dependent increase in incidence of hypercalciuria and mild hypercalcemia suggests that for individuals taking high-dose vitamin D, it may be prudent to assess calcium intake and evaluate biochemical measures of calcium metabolism. However, in the 400-IU group that was following the National Academy of Medicine–recommended dietary allowance of calcium and vitamin D3, 17% had hypercalciuria on at least 1 occasion over the study duration.
Limitations
This study has several limitations. First, degradation of 2 lots of the vitamin D solution administered to participants in the 10000-IU per day group between months 18 and 36 resulted in those participants receiving less than the designated dose. Despite this occurrence, dose-dependent changes were observed.
Second, participants with osteoporosis or with 25(OH)D levels of less than 30 nmol/L were excluded from this study. It is possible that participants with osteoporosis or low 25(OH)D levels would have responded differently to high-dose vitamin D supplementation, which would need to be explored following a different study design. The mean normal baseline 25(OH)D levels in this study were above the National Academy of Medicine–recommended levels considered adequate for bone health in 97.5% of the population (50 nmol/L), and are similar to the baseline levels in the VITAL (Vitamin D and Omega-3) trial.39
Third, this study did not include a placebo control group. The participants in the 400-IU group served as a reference group in the study design, receiving the National Academy of Medicine–recommended intake for adults younger than age 70 (accounting for dietary input). Affirming their appropriateness as a reference group, their serum 25(OH)D did not change throughout the study, and a dose-dependent effect on bone relative to this 400-IU group was shown.
Fourth, since the effect of high-dose vitamin D supplementation on HR-pQCT measurements of volumetric BMD were in the opposite direction of the hypothesized effect, it is not appropriate to interpret the findings as proof of skeletal harm. These unanticipated results should be regarded as hypothesis-generating for future studies.
Conclusions
Among healthy adults, treatment with vitamin D for 3 years at a dose of 4000 IU per day or 10000 IU per day, compared with 400 IU per day, resulted in statistically significant lower radial BMD; tibial BMD was significantly lower only with the daily dose of 10
000 IU. There were no significant differences in bone strength at either the radius or tibia. These findings do not support a benefit of high-dose vitamin D supplementation for bone health; further research would be needed to determine whether it is harmful.
Notes
Supplement 3.
eTable 1. Raw Data for Biochemistry
eTable 2. Raw Data for the Primary Outcome Variables
eTable 3. Raw Data for the Secondary Outcome Variables
eTable 4. Summary of the Statistical Models
eFigure 1. Change in Serum 25-Hydroxyvitamin D, Parthyroid Hormone, and C-Telopeptide of Type 1 Collagen During Three Years of Vitamin D Supplementation
eFigure 2. Change in Cortical and Trabecular Bone Mineral Density During Three Years of Vitamin D Supplementation
eFigure 3. Data Distribution for Cortical and Trabecular Bone Mineral Density Throughout the Three-Year Study
eFigure 4. Change in Trabecular Number and Cortical Porosity During Three Years of vitamin D Supplementation
efigure 5. Data Distribution for Trabecular Number and Cortical Porosity Throughout the Three-Year Study
eReferences.
References

Full text links
Read article at publisher's site: https://doi.org/10.1001/jama.2019.11889
Read article for free, from open access legal sources, via Unpaywall:
https://jamanetwork.com/journals/jama/articlepdf/2748796/jama_burt_2019_oi_190088.pdf
Citations & impact
Impact metrics
Article citations
Vitamin D-Do Diet Recommendations for Health Remain Strong?
Curr Osteoporos Rep, 22(6):523-535, 02 Oct 2024
Cited by: 0 articles | PMID: 39356464
Review
Association of white matter hyperintensities with cognitive decline and neurodegeneration.
Front Aging Neurosci, 16:1412735, 12 Sep 2024
Cited by: 0 articles | PMID: 39328245 | PMCID: PMC11425965
Changes in bone density and microarchitecture following treatment of Graves' disease and the effects of vitamin D supplementation. A randomized clinical trial.
Osteoporos Int, 12 Sep 2024
Cited by: 0 articles | PMID: 39264438
Consensus Statement on Vitamin D Status Assessment and Supplementation: Whys, Whens, and Hows.
Endocr Rev, 45(5):625-654, 01 Sep 2024
Cited by: 15 articles | PMID: 38676447 | PMCID: PMC11405507
Review Free full text in Europe PMC
Reversal of heavy arterial calcification in a rat model of chronic kidney disease using targeted ethylene diamine tetraacetic acid-loaded albumin nanoparticles.
Cardiovasc Diagn Ther, 14(4):489-508, 23 Aug 2024
Cited by: 0 articles | PMID: 39263487 | PMCID: PMC11384463
Go to all (125) article citations
Data
Data behind the article
This data has been text mined from the article, or deposited into data resources.
BioStudies: supplemental material and supporting data
Clinical Trials
- (1 citation) ClinicalTrials.gov - NCT01900860
Similar Articles
To arrive at the top five similar articles we use a word-weighted algorithm to compare words from the Title and Abstract of each citation.
Effect of Vitamin D Supplementation on Bone Mass in Infants With 25-Hydroxyvitamin D Concentrations Less Than 50 nmol/L: A Prespecified Secondary Analysis of a Randomized Clinical Trial.
JAMA Pediatr, 177(4):353-362, 01 Apr 2023
Cited by: 4 articles | PMID: 36780180 | PMCID: PMC9926359
Effect of different dosages of oral vitamin D supplementation on vitamin D status in healthy, breastfed infants: a randomized trial.
JAMA, 309(17):1785-1792, 01 May 2013
Cited by: 115 articles | PMID: 23632722
Effect of Higher vs Standard Dosage of Vitamin D3 Supplementation on Bone Strength and Infection in Healthy Infants: A Randomized Clinical Trial.
JAMA Pediatr, 172(7):646-654, 01 Jul 2018
Cited by: 37 articles | PMID: 29813149 | PMCID: PMC6137511
Effectiveness and safety of vitamin D in relation to bone health.
Evid Rep Technol Assess (Full Rep), (158):1-235, 01 Aug 2007
Cited by: 138 articles | PMID: 18088161
ReviewBooks & documents Free full text in Europe PMC