Abstract
Free full text

Dopamine D1 and NMDA Receptors Mediate Potentiation of Basolateral Amygdala-Evoked Firing of Nucleus Accumbens Neurons
Abstract
Interactions between the basolateral amygdala (BLA) and the nucleus accumbens (NAc) mediate reward-related processes that are modulated by mesoaccumbens dopamine (DA) transmission. The present in vivo electrophysiological study assessed: (1) changes in the firing probability of submaximal BLA-evoked single neuronal firing activity in the NAc after tetanic stimulation of the BLA, and (2) the functional roles of DA and NMDA receptors in these processes. Tetanic stimulation of the BLA potentiated BLA-evoked firing activity of NAc neurons for a short duration (~25 min). This short-term potentiation was associated with an increase in DA oxidation currents that was monitored with chronoamperometry. Systemic or iontophoretic application before BLA tetanus of the D1 receptor antagonist SCH23390, but not the D2 receptor antagonist sulpiride, abolished the potentiation of BLA-evoked NAc activity, whereas administration of SCH23390 3 min after tetanus had no effect. However, systemic administration of the NMDA antagonist 3-(2-carboxypiperazin-4-yl)-propyl-1-phosphonic acid (CPP), either before or after BLA tetanus, abolished the potentiation of BLA-evoked firing of NAc neurons. These data suggest that higher-frequency activity in BLA efferents can autoregulate their excitatory influence over neural activity of NAc neurons by facilitating the release of DA and activating both DA D1and NMDA receptors. This may represent a cellular mechanism that facilitates approach behaviors directed toward reward-related stimuli that are mediated by BLA–NAc circuitries.
The association of previously neutral stimuli with primary rewards to establish conditioned reinforcers is dependent on interactions between the basolateral amygdala (BLA) and the nucleus accumbens (NAc) (Cador et al., 1991;Everitt et al., 1991; Robbins and Everitt, 1992a, 1996; Baldwin et al., 2000). In addition, mesoaccumbens dopamine (DA) transmission has been shown to exert a powerful modulatory effect on behaviors mediated by BLA–NAc circuits (Robbins and Everitt, 1992a,b; Burns et al., 1993;Wolterink et al., 1993; Smith-Roe and Kelley, 2000). Contemporary theory regarding the role of mesoaccumbens DA in the modulation of reward-related learning has posited that DA transmission can exert a “gain-amplifying” effect over approach behavior that is mediated by the BLA (Robbins and Everitt, 1992b; 1996).
Glutamatergic projections from the BLA to the NAc (Kelley et al., 1982;Robinson and Beart, 1988), synapse in close apposition to DA axons on medium spiny neurons of the NAc (Johnson et al., 1994). Recent neurochemical studies have shown that tetanic stimulation of the BLA evokes DA efflux in the NAc via glutamate receptor-dependent mechanisms localized within the NAc (Floresco et al., 1998). In addition, electrophysiological studies have shown that application of either exogenous DA or synaptically released DA by stimulation of the ventral tegmental area can modulate excitatory responses of NAc neurons evoked by low-frequency stimulation of the BLA (Yim and Mogenson, 1982,1986). In light of these data, it is likely that a complex interaction between glutamatergic BLA inputs and mesoaccumbens DA inputs in the NAc mediate behaviors dependent on BLA–NAc circuitries.
Given the importance of BLA–NAc circuits in reward-related learning and its modulation by mesoaccumbens DA, it is surprising that few studies have examined plasticity of evoked activity in this pathway (Uno and Ozawa, 1991). Moreover, the role of glutamatergic and dopaminergic interactions in the modulation of the BLA–NAc pathway is poorly understood. Therefore, the present study used extracellular single-unit recordings from NAc neurons that receive input from the BLA to assess the relation between evoked efflux of DA in the NAc, measured by chronoamperometry, and post-tetanic potentiation of the BLA–NAc pathway. In addition, administration of selective DA and NMDA antagonists was used to assess the involvement of these receptors in this form of potentiation.
MATERIALS AND METHODS
Animals and surgical preparations. All experiments were approved by the University of British Columbia Animal Care Committee and were conducted in accordance with the standards of the Canadian Council on Animal Care. Forty-three male Long–Evans rats weighing 250–350 gm were anesthetized with urethane (1.5 gm/kg, i.p.) and mounted in a stereotaxic frame. Body temperature was maintained at 37°C with a temperature-controlled heating pad. Rats were implanted with concentric bipolar electrical stimulating electrodes in the BLA (flat skull, anteroposterior (AP), −3.2 mm; mediolateral (ML), +5.0 mm; dorsoventral (DV), −7.0 mm). In some rats, stearate-modified graphite paste electrochemical recording electrodes were implanted stereotaxically into the NAc, ipsilateral to the stimulating electrode (AP, +1.5; ML, −1.0 at a 15° angle; DV, −6.5 mm). In these preparations, an Ag–AgC1 reference and stainless steel auxiliary electrode combination was placed in contact with cortical tissue 4 mm posterior to bregma.
Extracellular recordings. Extracellular single-unit activity was recorded with filament-filled glass microelectrodes (outer diameter, 1.5 mm; World Precision Instruments, Sarasota, FL) pulled by a programmable horizontal electrode puller (P-87; Sutter Instruments, Novato, CA). The microelectrodes were filled with fast green (Sigma, St. Louis, MO) mixed in 0.5 msodium acetate and had an impedance of 5–10 MΩ. The electrode was targeted toward the medial shell of the NAc (AP, +1.7–0.7 mm; ML, 0.9–1.3 mm; DV, 5.0–7.0 mm) using a hydraulic microdrive (MHW-40; Narishige, Tokyo, Japan). Single-unit activity was amplified by an XCell-3 amplifier (Frederick Haer & Co. Inc., Brunswick, ME) and filtered (bandpass, 500–5000 Hz); individual action potentials from single units were isolated from background noise using a window discriminator and sampled on-line by a computer connected to a Data Translation DT 2821 A/d board interface. Sampling of the spike signals was performed at 10 kHz by a PC computer using IPEE software (Dr. Conrad Yim, CY Electronics, Toronto, Canada). Peristimulus time histograms (see Figs. Figs.2,2, ,4)4) were compiled from 100 stimulus sweeps and plotted with a bin width of 1 msec. For rats that had electrochemical recording electrodes implanted in the NAc, the glass microelectrode was driven vertically in the same anterior plane as the electrochemical recording electrode (AP, 1.5 mm at 0° angle) and was 0.9–1.3 mm lateral from midline and 5.0–7.0 mm ventral from cortex.
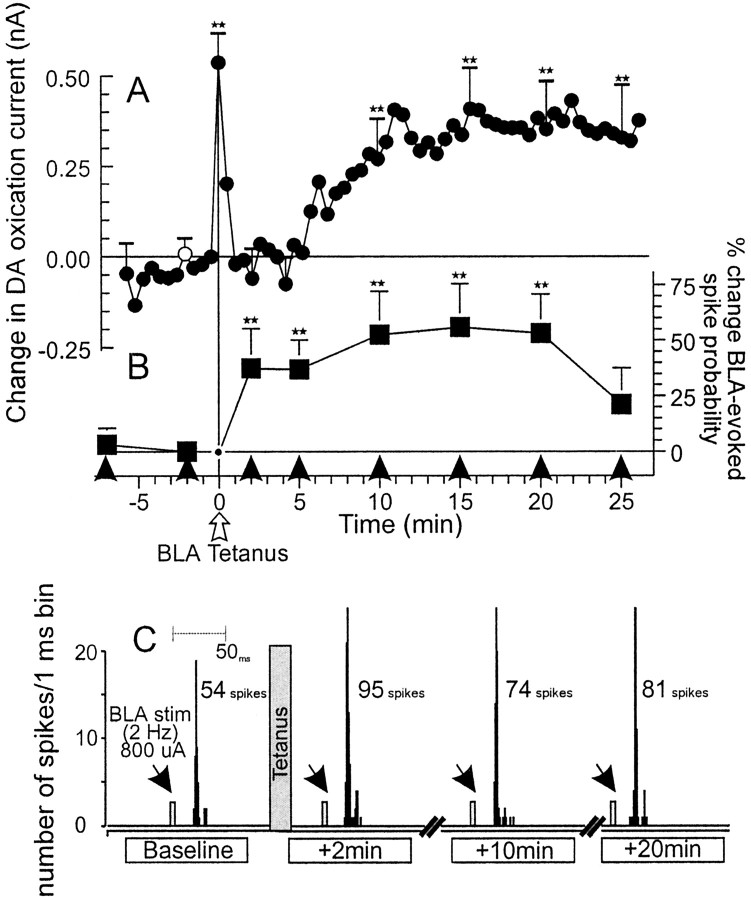
Tetanic stimulation of BLA afferents increases mesoaccumbens DA oxidation currents and enhances BLA-evoked spiking activity in NAc neurons. A, Mean changes in DA oxidation currents in the NAc recorded by chronoamperometry. ** denote significant difference from baseline (white circle) atp < 0.05. Error bars (+ SEM) are placed on those time points that were included in the data analysis. B, Mean percentage change (+SEM) in BLA-evoked spiking activity recorded from NAc neurons in the same animals from which the chronoamperometic recordings were obtained. Squares represent percentage change in BLA-evoked spiking probability normalized to the spike probability obtained 2 min before tetanus. Arrowheadsindicate time points at which trains of 2 Hz BLA stimulation were administered. ** denote significance versus baseline spike probability at p < 0.01. C, Peristimulus–time histograms showing the typical response from a NAc neuron 2 min before and 2, 10, and 20 min after BLA tetanus. This neuron displayed a baseline spiking probability of 0.54 (800 μA stimulation current). After BLA tetanus (gray bar), the spiking probability of the neuron was increased to nearly 1.0.Arrows represent time points when BLA stimulation was administered.
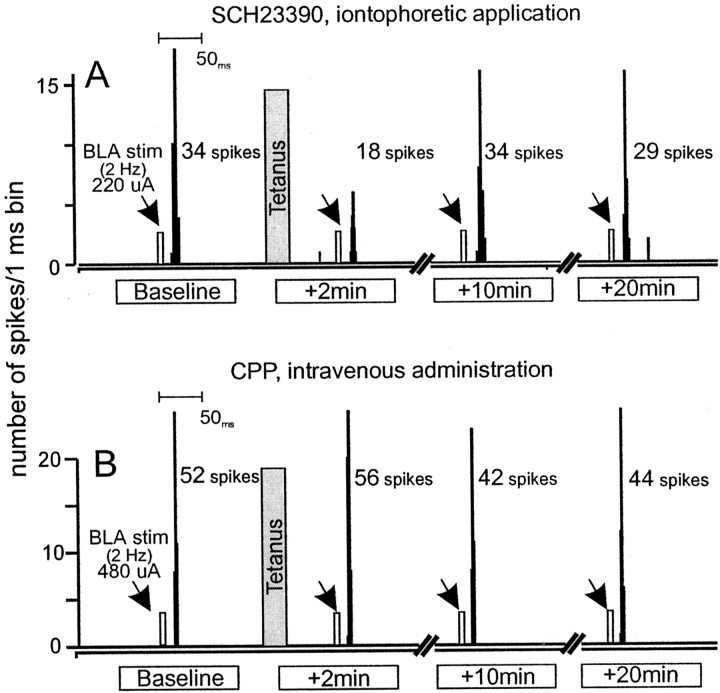
Individual data. A, Peristimulus–time histograms showing the typical response from a NAc neuron that was treated with SCH23390 iontophoretically (+40 nA) at time points 2 min before and 2, 10, and 20 min after BLA tetanus. This neuron displayed a baseline spiking probability of 0.34 (220 μA stimulation current). After BLA tetanus (gray bar), there was no potentiation of evoked-spiking activity.B, Peristimulus–time histograms showing the typical response from a NAc neuron recorded from an animal that had received intravenous administration of CPP (1.0 mg/kg) 10 min before tetanus. Histograms were taken from time points 2 min before and 2, 10, and 20 min after BLA tetanus. This neuron displayed a baseline spiking probability of 0.52 (480 μA stimulation current). After BLA tetanus (gray bar), there was no potentiation of evoked-spiking activity. For A and B,arrows represent time points when BLA stimulation was administered.
Electrochemical recordings. Repetitive chronoamperometric measurements selective for DA (Blaha and Phillips, 1996, and references therein) of oxidation current using an electrometer (Echempro, Vancouver, British Columbia, Canada) were made by applying a potential pulse from −0.15 V to +0.25 V versus Ag–AgCl to the recording electrode for 1 sec at 30 sec intervals and monitoring the oxidation current at the end of each 1 sec pulse. The timing of the potential pulse was set so that it would occur >300 msec after electrical stimulation of the BLA to ensure that the artifact produced by the pulse would not overlap with spikes evoked by BLA stimulation. Prestimulation baseline currents were normalized to zero current values with stimulated changes in the baseline signal presented as absolute changes in DA oxidation current (Floresco et al., 1998).
Stimulation protocol. During an initial cell searching procedure, stimuli were delivered to the BLA at 1 Hz while the microelectrode was advanced into the NAc. Cathodal monophasic square current pulses (0.2 msec duration) were delivered to the BLA through a concentric bipolar electrode (NE-100; Rhodes Medical Co.) connected to an Iso-Flex optically isolated stimulator (AMPI, Jerusalem, Israel) that received programmed pulses from a Master-8 pulse generator (AMPI). After isolating a NAc cell that responded to BLA stimulation, we adjusted the stimulation currents to approximately half-maximal stimulation intensity [i.e., ~50 action potentials were evoked in response to a train of 100 BLA stimulations delivered at 2 Hz (range 100–1800 μA; mean current, 1075 ± 75 μA; median current, 1200 μA)]. There were no differences in the mean level of stimulation currents used between treatment groups (F(5,49) = 1.53; p > 0.1).
Evoked spike probabilities were calculated by dividing the number of action potentials observed by the number of stimuli administered. Changes in spike probabilities were used as an index of the effect of tetanic stimulation on the magnitude of change in NAc neuronal activity produced by subsequent BLA stimulation. Single-pulse stimuli trains (2 Hz, 100 pulses for 50 sec) were delivered to the BLA every 3–5 min to sample the evoked spike probability. Once stable levels of evoked-spiking activity were obtained (<15% variability in spike probability over 10–15 min), one train of tetanic stimulation was administered to the BLA (200 pulses delivered at 20 Hz for 10 sec, current adjusted to near maximal intensity so that each stimulus evoked a spike). This tetanus parameter produces robust increases in mesoaccumbens DA efflux (Floresco et al., 1998) and is comparable with the activity of BLA neurons recorded from freely moving rats that have been presented with primary or conditioned rewards (e.g., 10–30 Hz) (Ono et al., 1995; Pratt and Mizumori, 1998). After tetanus, trains of 2 Hz stimulation (100 pulses) were administered at the same submaximal current intensity used before tetanus, at 2 min after tetanus, and then at 5 min intervals for another 25 min. No more than two tetani were administered per animal, and the delivery of a second tetanus was spaced by an interval of at least 3 hr.
Pharmacological manipulations. Drugs obtained from Research Biochemicals (Natick, MA) were administered via intravenous jugular catheters. No electrochemical recordings were taken during these pharmacological experiments. The D1 receptor antagonist SCH23390 (0.5 mg/kg) and NMDA receptor antagonist 3-(2-carboxypiperazin-4-yl)-propyl-1-phosphonic acid (CPP) (1.0 mg/kg) were dissolved in physiological saline. The D2 receptor antagonist sulpiride (5.0 mg/kg) was dissolved in a drop of NaOH and PBS. No more than two drug injections were given per animal, separated by at least 3.5 hr. Based on pharmacokinetic studies, this would have been a sufficient period of time to allow for >90% clearance of these drugs from plasma and brain (Segura et al., 1976; Patel et al., 1990; Hietala et al., 1992). There were no significant differences between cells in a particular drug treatment group regarding the effects between the first and second drug injections or between the first and second tetanus for control cells (all Fs < 1.6; NS). The doses of the DA antagonists were chosen from previous studies (White and Wang, 1986; Floresco et al., 2001).
The D1 antagonist SCH23390 was iontophoretically applied onto some NAc cells before tetanic stimulation of the BLA. In these experiments, three-barrel glass micropipettes were pulled from a vertical electrode puller (Narishige), and the tips were broken back to an average size of 5 μm. One barrel was filled with fast green in 0.5 m sodium acetate, the second barrel contained a 5 mm solution of SCH23390, pH 4.0, and the third barrel was filled with 1 m NaCl solution for automatic current balancing. SCH23390 was ejected using a Dagan 6400 iontophoretic current generator with an ejection current of +40 nA. A retaining current of −10 nA was used between injection periods.
Histology. After completion of each experiment, an iron deposit was made in the BLA stimulation site by passing DC current (100 μA for 10 sec) through the stimulating electrode. A dye deposit was made in the NAc recording site by ejecting fast green with a 20 μA anodal current for 20 min. The brain was removed and placed in 10% buffered formalin containing 0.1% potassium ferricyanide. After fixation, 50 μm sections were cut on a freezing microtome and stained for Nissl substance with cresyl violet. A Prussian blue spot resulting from a redox reaction of ferricyanide marked the stimulation site. Both the glass microelectrodes and electrochemical recording electrodes were verified to be located in the medial NAc or the border between the NAc shell and core (Fig.(Fig.11A). The stimulation electrodes were found to be located primarily in the caudal BLA (Fig.(Fig.11B).
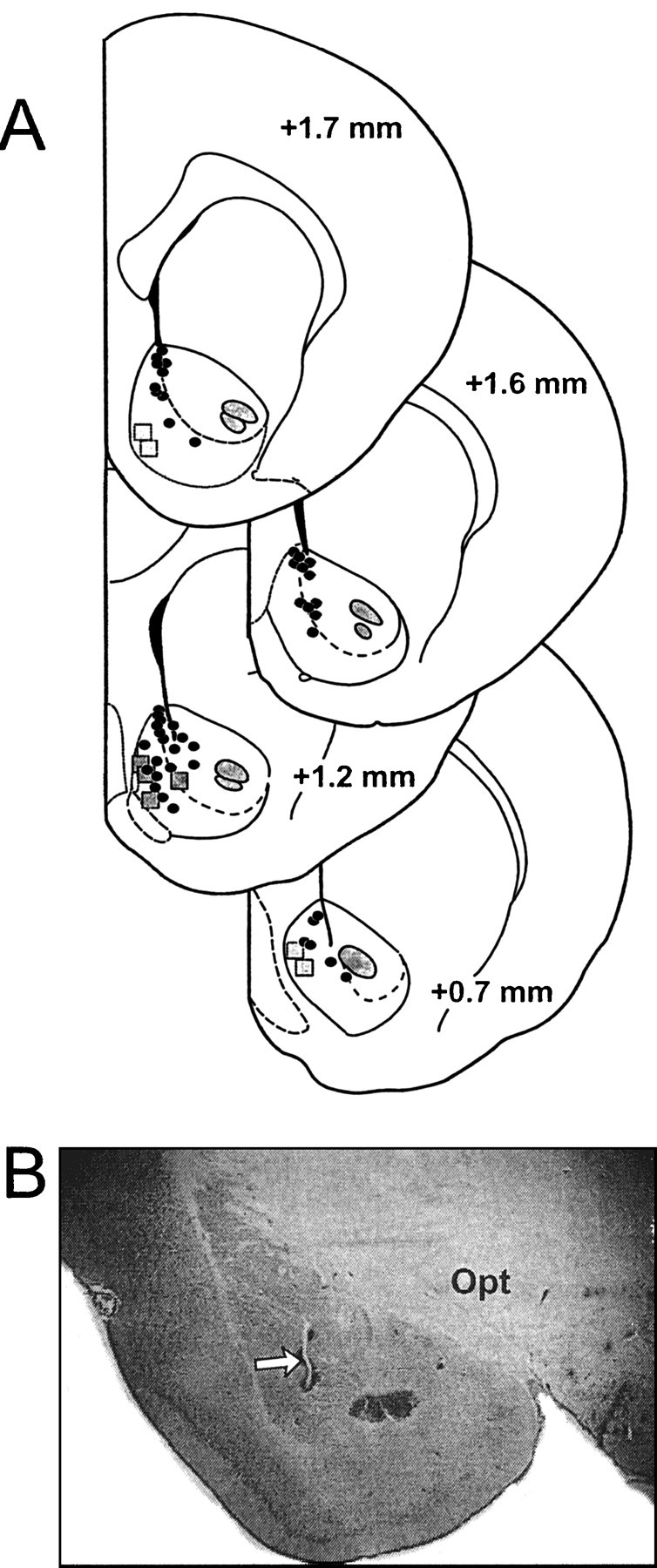
Histology. A, Schematic of coronal sections of the rat brain (Paxinos and Watson, 1997) showing representative placements of electrochemical electrodes (squares) and location of extracellular single-unit recording electrodes (circles) recorded from control rats and rats whose data are presented in Figure Figure33A–C.Numbers correspond to millimeters from bregma.B, Photograph of a representative placement of a stimulating electrode in the BLA. Arrow highlights the location of stimulating electrode placements. Opt, Optic tract.
Data analysis. In the electrochemical studies, the data were analyzed using a one-way, repeated measures ANOVA with time as a within-subjects factor. Only samples that were taken at tetanus and at time points at which the BLA was stimulated at 2 Hz were used in this analysis. Multiple comparisons were made versus the sample taken 2 min before tetanus and for data points at time 0 (tetanus) and at time points when the BLA was stimulated at 2 Hz. For the extracellular recording data, pretetanus baseline spike probabilities were normalized to the spike probability that was recorded 2 min before tetanus so that the change in spike probability at this time point would be 0. These data were then converted to percentage change in spike probability, using the formula:
Statistical comparisons were made with the spike probability recorded 7 min before tetanus. Multiple comparisons were made using Dunnett's test for repeated measures for both the electrochemical and electrophysiological comparisons.
RESULTS
Concurrent monitoring of mesoaccumbens DA efflux and electrophysiological recordings from NAc neurons
Extracellular single-unit recordings were obtained from 10 individual NAc neurons that received input from the BLA. These recordings were taken from eight rats that had electrochemical recording electrodes implanted into the ipsilateral NAc. Single-pulse stimulation of the BLA evoked excitatory responses in these NAc neurons with a latency range of 17–25 msec. After monitoring of a stable baseline firing probability of the NAc neurons for at least 10 min, tetanic stimulation of the BLA was presented. Tetanic stimulation of the BLA resulted in a transient increase in DA efflux that was time-locked to the presentation of the tetanus. This was followed promptly by a brief return to baseline, and then by a second, more prolonged increase in DA efflux that was significantly elevated 10–25 min after tetanus, as reported previously (Floresco et al., 1998) (F(8,40) = 2.96; p < 0.05; Dunnett's, p < 0.05) (Fig.(Fig.22A). In 9 of 10 (90%) NAc neurons that were recorded simultaneously with electrochemical measures, tetanic BLA stimulation also produced a robust short-term enhancement (lasting ≤25 min) in BLA-evoked spiking activity (F(6,54) = 2.4; p < 0.05; Dunnett's, p < 0.01) (Fig.(Fig.22B,C).
Pharmacological manipulations
To confirm that the potentiation of BLA-evoked spiking activity in NAc neurons was attributable to the increase in mesoaccumbens DA release after BLA tetanus, separate groups of rats received intravenous administration of selective D1 and D2 antagonists 10 min before BLA tetanus. There was no difference in the baseline spiking probability displayed by neurons in different drug treatment groups (F(5,49) = 1.3, NS; mean baseline spiking probability, 0.43 ± 0.02). The overall analysis of these data revealed a significant main effect of drug treatment (F(5,49) = 3.1; p < 0.05) and a significant effect of time (F(6,294) = 3.2; p < 0.005). Planned comparisons revealed that administration of the D1 receptor antagonist SCH23390 (0.5 mg/kg;n = 11, 8 rats) abolished the enhancement of BLA-evoked spiking activity in all cells tested (11 of 11 cells, 100%) and caused a significant (p < 0.05) but transient suppression in BLA-evoked activity that returned to baseline levels within 10 min (Fig.(Fig.33A).
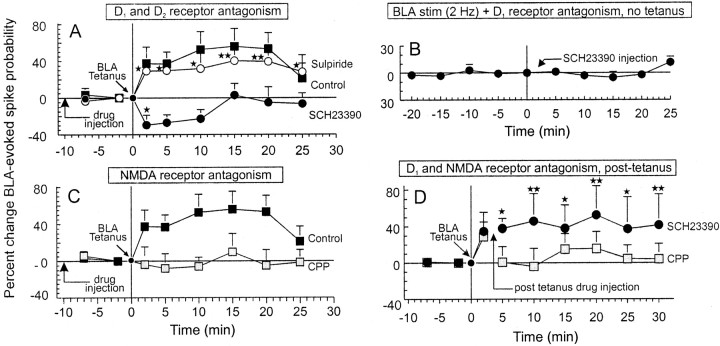
Potentiation of BLA-evoked spiking activity in NAc neurons is dependent on both D1 and NMDA receptors.Symbols represent mean percentage change (+SEM) in BLA-evoked spiking activity of NAc neurons. A, Change in BLA-evoked spiking activity under control conditions (black squares, same as Fig. Fig.22B), after treatment with the D1 receptor antagonist SCH23390 (0.5 mg/kg; black circles), and the D2 receptor antagonist sulpiride (5.0 mg/kg; white circles).Arrow indicates time point of drug injection.B, Change in BLA-evoked spiking activity recorded from NAc neurons after 25 min of 2 Hz stimulation in the absence of drug and for another 25 min after injection of SCH23390. Black circles represent time points when trains of 2 Hz stimulation were delivered to the BLA. In this experiment, no tetanus of the BLA was administered. Neither repeated trains of 2 Hz stimulation over 25 min nor injections of SCH23390 produced any significant change in evoked activity. C, Change in BLA-evoked spiking from control neurons (black squares, same as Fig.Fig.22B), after pretreatment with the NMDA receptor antagonist CPP (1.0 mg/kg; black squares).D, Change in BLA-evoked spiking activity after post-tetanus injection of SCH23390 (black circles) or CPP (gray squares). Arrowindicates time point when the drugs were administered (3 min after tetanus). * and ** denote significance from baseline spiking probabilities at p < 0.05 and 0.01, respectively.
In a separate group of cells (n = 4), SCH23390 was administered iontophoretically and continuously on the NAc neurons locally 5 min before BLA tetanus. In these cells, BLA tetani failed to induce any augmentation of BLA-evoked spiking activity (F(6,18) = 0.55, NS) (Fig.(Fig.44A). This finding is consistent with our observations after systemic administration of SCH23390.
In contrast to the effects of SCH23390, administration of the D2 receptor antagonist sulpiride (5.0 mg/kg;n = 11, 8 rats) had no significant effect, with 9 of 11 neurons (82%) displaying a robust increase in evoked spiking probability after tetanus (p < 0.01) (Fig.(Fig.33A). Thus, higher-frequency stimulation of BLA afferents to the NAc facilitates the release of DA and produces an increase in the excitability of NAc neurons in response to subsequent afferent input from the BLA that is in part dependent on activation of D1 receptors.
A separate group of neurons (n = 5) were activated with repeated trains of 2 Hz BLA stimulation over a 25 min period, but no tetanic stimulation was administered. After this period, an injection of SCH23390 (0.5 mg/kg) was administered, and the response of NAc neurons to 2 Hz BLA stimulation was monitored for another 25 min. Analysis of these data revealed no significant change in BLA-evoked spiking activity either before or after injection of SCH23390 (F(8,32) = 1.1; p > 0.1, NS) (Fig. (Fig.33B). Thus, repeated trains of 2 Hz stimulation do not cause any significant changes in BLA-evoked activity of NAc neurons. In addition, injection of SCH23390 in the absence of BLA tetanus also produces no change in BLA-evoked activity. This finding is similar to those observed in hippocampal afferents to the forebrain in which prolonged low-frequency stimulation had no long-lasting effects in evoked activity in either the prefrontal cortex or the NAc (Burette et al., 1997; Floresco et al., 2001). It is notable that this lack of effect was different from previous findings in the hippocampal slices in vitro in which extended lower frequency stimulation (e.g., 1 Hz, >10 min) can result in long-term depression (Mulkey and Malenka, 1992).
In a separate group of rats, administration of the NMDA receptor antagonist CPP (1.0 mg/kg; n = 9, 7 rats) also abolished the enhancement of evoked spiking activity of NAc neurons after BLA tetanus in eight of nine (89%) neurons tested (Figs.(Figs.33C, ,44B). From these data, it may be inferred that the augmentation of BLA-evoked spiking activity of NAc neurons requires a cooperative action of both D1and NMDA receptors. Blockade of either of these receptors is sufficient to disrupt the potentiation of NAc neuronal firing activity that normally follows tetanic stimulation of BLA afferents.
Post-tetanus blockade of D1 or NMDA receptors
To determine whether the initial transient increase in mesoaccumbens DA efflux or the prolonged post-BLA tetanus DA increase (Fig. (Fig.11A) was responsible for the increase in BLA-evoked spiking activity, a separate group of NAc cells was treated with SCH23390 intravenously 3 min after BLA tetanus, just before the secondary prolonged phase of DA efflux would have occurred. In six of seven neurons (86%, 7 rats), injection of SCH23390 (0.5 mg/kg, i.v.) after tetanus did not suppress the enhancement of BLA-evoked NAc spiking activity (Fig. (Fig.33D). This finding suggests that the initial transient increase in DA release that is coincidental with tetanic depolarization of NAc neurons by the glutamatergic BLA inputs is critical in inducing a longer lasting potentiation of BLA-evoked NAc activity.
In contrast to the effects of SCH23390, injection of the NMDA antagonist CPP after tetanus abolished or attenuated the potentiation (n = 6 of 7 cells, 86%) of cells tested (4 rats) (Fig.(Fig.33D). These data confirm that NMDA receptors are necessary for both the initial induction and subsequent expression of DA-mediated potentiation of BLA-evoked spiking activity in NAc neurons. In three NAc cells recorded from this post-tetanus group, the animals had received an initial post-tetanus injection of CPP 3–4 hr before the second injection. These cells exhibited an enhancement of BLA-evoked NAc spiking activity 2 min after tetanus (before injection of CPP) that was abolished after a post-tetanus administration of CPP. This confirms that 3–4 hr was a sufficient period of time for the effects of the initial injection of CPP to dissipate.
DISCUSSION
The present data demonstrate that higher-frequency stimulation of BLA inputs to the NAc can facilitate the release of mesoaccumbens DA, and this increase is correlated with an enhancement of firing of NAc neurons in response to subsequent BLA stimulation. The enhancement of BLA-evoked spiking activity is mediated by both DA D1 receptors and glutamate NMDA receptors, because administration of the D1 antagonist SCH23390 or the NMDA antagonist CPP abolished the potentiation of BLA-evoked spiking activity. In contrast, blockade of D2 receptors with sulpiride had no significant effect. Furthermore, the DA-mediated potentiation appears to be critically dependent on the initial transient increase of DA release relative to the arrival of higher-frequency inputs to the NAc, because post-tetanic administration of SCH23390 did not abolish the potentiation of evoked spiking activity. In contrast, NMDA receptor activation is essential for the induction and maintenance of the potentiation, because administration of CPP either before or after tetanic stimulation of the BLA blocks the potentiation of BLA-evoked activity.
There are a number of potential cellular mechanisms by which D1 receptor activation could facilitate BLA-evoked spiking activity of NAc neurons. For example, the inhibition of Na+/K+ATPase by D1 receptor activation (Betrorello et al., 1990; White and Hu, 1993) would lead to a general depolarization of NAc neurons, making them more responsive to BLA stimulation. D1 receptor stimulation can also lead to an activation of L-type Ca2+ channels (Hernández-López et al., 1997; Cépeda and Levine, 1998), and augmentation of NMDA receptor activity via phosphorylation of NMDA channels (Blank et al., 1997; Cépeda and Levine, 1998). Thus, activation of D1 receptors after DA release, in combination with tetanic depolarization of NAc neurons (both of which are achieved by stimulation of BLA afferents), could activate a number of different cellular mechanisms that would lead to a longer lasting increase in NAc neuronal excitability. Under these circumstances, NAc medium spiny neurons are more responsive to subsequent stimulation of glutamate inputs from the BLA, thereby increasing probability of cell firing.
Blockade of NMDA receptors, either before or after tetanic stimulation of the BLA, abolished the potentiation of BLA-evoked activity, suggesting that the enhancement of NMDA receptor activity is involved in both the induction and maintenance of the potentiated neuronal excitability in NAc neurons. This finding stands in contrast to studies of neural plasticity in the hippocampal slices in vitro, in which NMDA receptor activity is required for the induction but not the maintenance of long-term potentiation (Collingridge et al., 1983;Malenka and Nicoll, 1999). Thus, it is apparent that the cellular mechanisms that mediate the maintenance of potentiation of evoked field potentials in the hippocampus in vitro and those that regulate potentiation of BLA-evoked spiking activity of NAc neuronsin vivo differ with respect to the involvement of the NMDA receptor. Alternatively, this difference may be attributable to different dependent measures used in the present study relative to those studies conducted in the hippocampus in vitro (i.e., spiking probability versus postsynaptic field potentials recorded extracellularly or postsynaptic membrane potentials recorded intracellularly).
As noted above, tetanic stimulation of the BLA resulted in an initial increase in mesoaccumbens DA efflux, followed by a more prolonged increase in extracellular DA levels. Injections of SCH23390 before tetanus, which would have blocked the effects of both the initial transient and more prolonged increase, abolished the potentiation of BLA-evoked spiking activity. However, when SCH23390 was administered 3 min after tetanus, (which would have blocked the effects of the late-onset, prolonged increase in DA while leaving the effects of the transient increase intact), there was no block of this potentiation. The fact that the potentiation of BLA-evoked activity occurred after the initial increase in DA efflux suggests that a transient increase in DA release that is coincidental with tetanic depolarization is sufficient to induce a longer lasting potentiation of the excitability of NAc neurons to BLA inputs, and the subsequent release of DA does not to appear to play a role in this enhancement. However, we cannot rule out the possibility that basal levels of mesoaccumbens DA (and not the transient increase linked to BLA tetanus), in combination with higher frequency activity in the BLA–NAc pathway, may also be sufficient to cause potentiation of BLA-evoked activity in NAc neurons. In this context, we note that pulsatile application of DA, coincidental with tetanic stimulation of glutamatergic inputs, resulted in long-term potentiation of a corticostriatal pathway in vitro (Wickens et al., 1996). Furthermore, prolonged bath application of DA combined with tetanus resulted in long-term depression. This latter finding, together with the present data, suggests that DA exerts differential effects on glutamatergic inputs, depending on the timing of release.
With respect to dopaminergic modulation of the BLA–NAc pathway, activation of NAc DA receptors by synaptically evoked release of endogenous DA from the ventral tegmental area inputs suppresses low frequency (1 Hz) BLA-evoked firing of NAc neurons (Yim and Mogenson, 1982, 1986; Mogenson et al., 1993). However, stimulation of DA receptors in combination with coincidental activation of NAc neurons by higher-frequency BLA input to the NAc (as was achieved in the present study) augments subsequent BLA-evoked NAc activity. It is important to note that the modulation of mesoaccumbens DA release by BLA inputs to the NAc (Floresco et al., 1998) may provide an intrinsic auto-regulatory mechanism to ensure that DA is released when BLA inputs are active at a high frequency, which in turn enhances BLA inputs to the NAc.
The present data suggest that higher-frequency activity in afferents from the BLA to the NAc may autoregulate their influence over the neural activity of NAc neurons by facilitating the release of DA, which in turn, increases the response of NAc neurons to glutamatergic inputs from the BLA. These data are strikingly similar to other findings showing that hippocampal inputs to the forebrain can regulate mesolimbic DA release, which in turn, amplifies hippocampal influence on NAc neural activity. Tetanic stimulation of hippocampal afferents to the NAc also facilitates mesoaccumbens DA release (Blaha et al., 1997), and DA released by this mechanism can potentiate hippocampal-evoked firing of NAc neurons via a cooperative interaction between D1 and NMDA receptors (Floresco et al., 2001). Similarly, tetanic stimulation of the ventral subiculum of the hippocampus is associated with both an increase in DA efflux in the prefrontal cortex and the induction of long-term potentiation in the hippocampal–cortical pathway; this effect is abolished by either DA D1 or NMDA receptor blockade (Jay et al., 1995;Gurden et al., 2000). Thus, the modulation of mesotelencephalic DA release by higher-frequency activity in glutamatergic efferents, originating in the temporal lobe, may serve as an essential component of a cellular gating mechanism that ensures that a specific input to either NAc or the prefrontal cortex has preferential influence on neural activity.
Functional implications
The present data have important theoretical implications for reward-related processes that are mediated by BLA–NAc circuits. It is well established that the control over behavior by conditioned reinforcers and Pavlovian-to-instrumental transfers is mediated by interactions between the BLA and the NAc (Everitt et al., 1991,1999; Robbins and Everitt 1992a, 1996; Baldwin et al., 2000). In addition, BLA or NAc neurons recorded from awake rats displays increases in firing rates (10–30 Hz) during the presentation of either primary reward or conditioned reinforcers, or immediately before an approach response directed toward these stimuli (Lavoie and Mizumori, 1994; Ono et al., 1995; Peoples et al., 1998; Pratt and Mizumori, 1998). Of particular interest are the recent findings by Smith-Roe and Kelley (2000) showing that instrumental learning, which is mediated by both the BLA and the NAc, is critically dependent on coincidental activation of NMDA and DA D1 receptors in the NAc. From these data, it is reasonable to suggest that approach toward conditioned stimuli is mediated in part by increases in NAc neural activity that is driven by input from the BLA and these behaviors are facilitated by both DA D1 and NMDA receptors.
Recent studies investigating plasticity in limbic–ventral striatal pathways in vivo have revealed that potentiation of evoked activity in these circuits is relatively short lasting (<1 hr) (Mulder et al., 1997; Floresco et al., 2001). As such, it is unlikely that long-lasting changes in reward-related behaviors are mediated by changes in synaptic strength at the level of the NAc. Rather, longer lasting changes in neural activity in response to particular environmental stimuli that may be relevant to reward-related learning likely occur at the level of the BLA (Maren, 1999). In contrast, the short-term potentiation of BLA-evoked activity in NAc neurons may be part of a cellular mechanism that allows the BLA to have a preferred (but temporary) access to the motor systems via the NAc, facilitating distinct patterns of behavior. In addition, under certain circumstances, increased activity in the BLA–NAc pathway may work in concert with inputs from either the hippocampus or prefrontal cortex to facilitate the firing of NAc neurons driven by these other afferents (O'Donnell and Grace, 1995; Moore and Grace, 2000; Floresco et al., 2001). Taken together, the present data support our previous conjecture (Floresco et al., 1998) that increases in activity of BLA neurons in response to environmental stimuli that have gained incentive salience may serve a dual function: (1) to activate specific NAc output ensembles (Pennartz et al., 1994) that result in preparatory and approach behaviors directed toward reward-related stimuli, and (2) to augment DA efflux that, via activation of DA D1receptors, may strengthen the NMDA-mediated plasticity that occurs in BLA–NAc ensembles. This would ensure that subsequent approach behavior is directed toward the appropriate salient incentive stimuli in the environment (Robbins and Everitt, 1996; Kelley et al., 1997).
Footnotes
This work was supported by a grant from the Natural Science and Engineering Research Council of Canada to A.G.P. S.B.F. is a recipient of a Human Frontiers Science Organization Post-Doctoral Fellowship. We thank Amiel Rosenkranz and Dr. Holly Moore for helpful comments on this manuscript and Dr. Anthony West for his invaluable technical assistance.
Correspondence should be addressed to Dr. Stan B. Floresco, Department of Neuroscience, University of Pittsburgh, 446 Crawford Hall, Pittsburgh, PA 15260. E-mail:ude.ttip.snb.niarb@ocserolf.
REFERENCES
Articles from The Journal of Neuroscience are provided here courtesy of Society for Neuroscience
Full text links
Read article at publisher's site: https://doi.org/10.1523/jneurosci.21-16-06370.2001
Read article for free, from open access legal sources, via Unpaywall:
http://www.jneurosci.org/content/jneuro/21/16/6370.full.pdf
Free to read at www.jneurosci.org
http://www.jneurosci.org/cgi/content/abstract/21/16/6370
Free after 6 months at www.jneurosci.org
http://www.jneurosci.org/cgi/reprint/21/16/6370.pdf
Free after 6 months at www.jneurosci.org
http://www.jneurosci.org/cgi/content/full/21/16/6370
Citations & impact
Impact metrics
Citations of article over time
Article citations
The dose-dependent effect of the D2R agonist quinpirole microinjected into the ventral pallidum on information flow in the limbic system.
Prog Neuropsychopharmacol Biol Psychiatry, 134:111059, 18 Jun 2024
Cited by: 0 articles | PMID: 38901759
Ca2+-modulated photoactivatable imaging reveals neuron-astrocyte glutamatergic circuitries within the nucleus accumbens.
Nat Commun, 13(1):5272, 07 Sep 2022
Cited by: 6 articles | PMID: 36071061 | PMCID: PMC9452556
Contingent Amygdala Inputs Trigger Heterosynaptic LTP at Hippocampus-To-Accumbens Synapses.
J Neurosci, 42(34):6581-6592, 15 Jul 2022
Cited by: 6 articles | PMID: 35840324 | PMCID: PMC9410749
Cocaine induces input and cell-type-specific synaptic plasticity in ventral pallidum-projecting nucleus accumbens medium spiny neurons.
Neuropsychopharmacology, 47(8):1461-1472, 04 Feb 2022
Cited by: 7 articles | PMID: 35121830 | PMCID: PMC9205871
Involvement of Basolateral Amygdala Dopamine D1 Receptors in the Acquisition and Expression of Morphine-Induced Place Preference in Rats.
Adv Biomed Res, 11:8, 31 Jan 2022
Cited by: 2 articles | PMID: 35284350 | PMCID: PMC8906092
Go to all (91) article citations
Similar Articles
To arrive at the top five similar articles we use a word-weighted algorithm to compare words from the Title and Abstract of each citation.
Modulation of hippocampal and amygdalar-evoked activity of nucleus accumbens neurons by dopamine: cellular mechanisms of input selection.
J Neurosci, 21(8):2851-2860, 01 Apr 2001
Cited by: 159 articles | PMID: 11306637 | PMCID: PMC6762526
Heightened amygdala long-term potentiation in neurotensin receptor type-1 knockout mice.
Neuropsychopharmacology, 33(13):3135-3145, 19 Mar 2008
Cited by: 12 articles | PMID: 18354386
Basolateral amygdala modulation of the nucleus accumbens dopamine response to stress: role of the medial prefrontal cortex.
Eur J Neurosci, 17(6):1287-1295, 01 Mar 2003
Cited by: 42 articles | PMID: 12670317
Contribution of AMPA and NMDA receptors to excitatory responses in the inferior colliculus.
Hear Res, 168(1-2):35-42, 01 Jun 2002
Cited by: 27 articles | PMID: 12117507
Review