Abstract
Background
LRRK2 mutations are a common cause of dominantly inherited PD. Previous studies showed decreases in urine levels of didocohexaenoyl (22:6) bis(monoacylglycerol)phosphate in LRRK2-knockout mice and in non-human primates treated with LRRK2 kinase inhibitors. We hypothesized that urine levels of bis(monoacylglycerol)phosphate isoforms will be higher in individuals with a PD-causing gain-of-kinase function mutation, LRRK2 G2019S. The objective of this study was to investigate alterations in urinary phospholipids as biomarkers of LRRK2 mutations and Parkinson's disease status/phenotypes.Methods
Ultra-performance liquid chromatography-tandem mass spectrometry (UPLC-MS/MS) was used to assess 54 bioactive phospholipids in urine from the LRRK2 Cohort Consortium (n = 80). To confirm and extend the findings, urine from an independent LRRK2 cohort from Columbia University Irving Medical Center (n = 116) was used. Both cohorts were composed of LRRK2 G2019S carriers and non-carriers with and without PD.Results
In each cohort, 4 bis(monoacylglycerol)phosphate isoforms (di-18:1-bis[monoacylglycerol]phosphate, didocohexaenoyl [22:6] bis[monoacylglycerol] phosphate, 2,2'-di-22:6-bis[monoacylglycerol]phosphate, and 2,2'-di-18:1-bis[monoacylglycerol]phosphate) were significantly higher (2.5- to 4.3-fold) in G2019S carriers compared with non-carriers. Interestingly, 2,2'-di-18:1-bis(monoacylglycerol)phosphate levels were marginally higher in LRRK2 carriers with PD than in those without PD (P = 0.045). Moreover, increased 2,2' and total di-22:6-bis(monoacylglycerol)phosphate were associated with worse cognitive status assessed by the Montreal Cognitive Assessment (P = 0.0033 and 0.0144, respectively).Conclusions
The observed association of bis(monoacylglycerol)phosphate isoforms with LRRK2 G2019S mutation, PD status among G2019S carriers, and correlation with cognitive decline suggest the potential use of urinary bis(monoacylglycerol)phosphate isoforms as biomarkers for clinical trials of LRRK2-targeted therapies. © 2019 The Authors. Movement Disorders published by Wiley Periodicals, Inc. on behalf of International Parkinson and Movement Disorder Society.Free full text
Higher Urine bis(Monoacylglycerol)Phosphate Levels in LRRK2 G2019S Mutation Carriers: Implications for Therapeutic Development
Abstract
Background
LRRK2 mutations are a common cause of dominantly inherited PD. Previous studies showed decreases in urine levels of didocohexaenoyl (22:6) bis(monoacylglycerol)phosphate in LRRK2‐knockout mice and in non‐human primates treated with LRRK2 kinase inhibitors. We hypothesized that urine levels of bis(monoacylglycerol)phosphate isoforms will be higher in individuals with a PD‐causing gain‐of‐kinase function mutation, LRRK2 G2019S. The objective of this study was to investigate alterations in urinary phospholipids as biomarkers of LRRK2 mutations and Parkinson's disease status/phenotypes.
Methods
Ultra‐performance liquid chromatography–tandem mass spectrometry (UPLC‐MS/MS) was used to assess 54 bioactive phospholipids in urine from the LRRK2 Cohort Consortium (n = 80). To confirm and extend the findings, urine from an independent LRRK2 cohort from Columbia University Irving Medical Center (n = 116) was used. Both cohorts were composed of LRRK2 G2019S carriers and non‐carriers with and without PD.
Results
In each cohort, 4 bis(monoacylglycerol)phosphate isoforms (di‐18:1‐bis[monoacylglycerol]phosphate, didocohexaenoyl [22:6] bis[monoacylglycerol] phosphate, 2,2′‐di‐22:6‐bis[monoacylglycerol]phosphate, and 2,2′‐di‐18:1‐bis[monoacylglycerol]phosphate) were significantly higher (2.5‐ to 4.3‐fold) in G2019S carriers compared with non‐carriers. Interestingly, 2,2′‐di‐18:1‐bis(monoacylglycerol)phosphate levels were marginally higher in LRRK2 carriers with PD than in those without PD (P = 0.045). Moreover, increased 2,2′ and total di‐22:6‐bis(monoacylglycerol)phosphate were associated with worse cognitive status assessed by the Montreal Cognitive Assessment (P = 0.0033 and 0.0144, respectively).
Conclusions
The observed association of bis(monoacylglycerol)phosphate isoforms with LRRK2 G2019S mutation, PD status among G2019S carriers, and correlation with cognitive decline suggest the potential use of urinary bis(monoacylglycerol)phosphate isoforms as biomarkers for clinical trials of LRRK2‐targeted therapies. © 2019 The Authors. Movement Disorders published by Wiley Periodicals, Inc. on behalf of International Parkinson and Movement Disorder Society.
Mutations in the gene encoding leucine‐rich repeat kinase 2 (LRRK2) are among the most common genetic causes of Parkinson's disease (PD).1 Although the association between LRRK2 gene mutations and PD was initially reported in 2004,2 the mechanism by which these mutations cause PD is not entirely clear. However, several lines of evidence indicate that increased LRRK2 kinase activity is important in the pathogenesis of PD.3 Therefore, LRRK2 kinase inhibitors are highly pursued as a therapeutic approach for PD.3
Experience from failed therapeutic trials of PD and Alzheimer's disease highlights the need for biomarker‐informed patient enrichment strategies for successful clinical development of disease‐modifying therapies. For LRRK2 kinase inhibitors, 3 potential strategies may be considered. The first would be to assess the slowing of disease progression in LRRK2 pathogenic mutation carriers with PD (PD+LRRK2+). Although attractive, a major hurdle of this approach is access to a sufficient number of patients for the entirety of the drug approval path. Second is to study whether disease onset may be prevented in non‐manifesting carriers of LRRK2 pathogenic mutations without motor deficits of PD (PD‐LRRK2+). This approach could be medically most beneficial, but the length of the trial duration and regulatory path for drug approval present major challenges currently. Last, idiopathic PD without LRRK2 mutations (PD+LRRK2‐) offers an attractive treatment group to circumvent the challenges mentioned above. Scientifically, this group could also be rationalized on the basis of the potential role of LRRK2 in idiopathic PD pathogenesis4 (reviewed by Roosen and Cookson, 20165). However, idiopathic PD represents highly heterogeneous pathogenic mechanisms. Therefore, biomarkers that can help enrich the trial with those most likely to benefit from alterations in the metabolic pathways of LRRK2 are required. A reliable biomarker of LRRK2 activity would also be beneficial for confirming target engagement and monitoring treatment compliance of LRRK2 kinase inhibitors.
Several lines of evidence indicate that bis(monoacylglycerol)phosphate (BMP) isoforms are candidate biomarkers of LRRK2 activity. BMPs are localized within the inner membranes of late endosomes (multivesicular bodies) and lysosomes, where they contribute to the multivesicular/lamellar morphology of the endolysosomal network.6, 7 Recent studies have identified several GTPases as bona fide substrates of LRRK2 and in conjunction with other evidence have implicated a role of LRRK2 in endolysosomal vesicular trafficking and lysosomal functions.8 Didocosahexaenoyl(22:6)‐BMP (di‐22:6‐BMP), a specific species of BMP, is decreased in the urine of LRRK2‐knockout mice and in non‐human primates treated with chemically distinct LRRK2 kinase inhibitors (PFE‐360, MLi‐2, and GNE‐7915),9 directly implicating its utility as a biomarker of LRRK2 activity. However, changes in BMP levels have not been examined in humans with LRRK2 mutations.
The G2019S mutation in LRRK2 has been demonstrated to increase LRRK2 kinase activity in various model systems.3 Thus, we hypothesized that in contrast to the decreased levels of di‐22:6‐BMP in LRRK2‐knockout and non‐human primates treated with LRRK2 kinase inhibitors, G2019S mutation carriers will have elevated levels of urinary di‐22:6‐BMP and other BMP species. In addition, we aimed to evaluate whether PD status and disease severity indicated by the Unified Parkinson's Disease Rating Scale (UPDRS) and the Montreal Cognitive Assessment (MoCA) are associated with BMP levels in LRRK2 G2019S carriers and non‐carriers.
Methods
Clinical Cohorts
Urinary BMPs were measured in 2 independent cross‐sectional cohorts. Both studies were approved by local institutional review boards, and all participants signed informed consents. The first cohort included bio‐banked urine samples from the Michael J. Fox Foundation (MJFF) LRRK2 Cohort Consortium (LCC). Urine samples were analyzed from 80 participants who were frequency‐matched by sex, disease duration, and age at onset: 20 PD patients with LRRK2 G2019S mutation (PD+LRRK2+), 20 idiopathic PD without LRRK2 G2019S or other LRRK2 pathogenic mutations (PD+LRRK2‐), 20 non‐manifesting carriers of LRRK2 G2019S mutation (PD‐LRRK2+), and 20 healthy individuals without LRRK2 pathogenic mutations (PD‐LRRK2). Disease severity scales were not available for these participants. In this cohort, in addition to quantitative assessment of di‐22:6‐BMP levels, we conducted preliminary analysis of 54 distinct bioactive phospholipids from 3 classes: BMPs, cell membrane phospholipids (phosphotidylinositol [PI], phosphotidylethanolamine [PE], and phosphotidylcholine [PC]), and globotriaosylceramides (Gb3s; implicated in Fabry's disease).
To confirm and extend the findings from the LCC cohort, urine from a second cohort recruited at Columbia University Irving Medical Center (CUIMC) was tested. Participants in the CUIMC cohort donated urine under a MJFF‐funded LRRK2 biomarker project from March 2016 to April 2017. They underwent clinical evaluation for motor severity using the UPDRS part III (UPDRS‐III), and their cognitive functioning was assessed using the MoCA. Genotyping for LRRK2 G2019S and GBA mutations was conducted as previously described.10 Urine was analyzed from 25 PD patients with LRRK2 G2019S mutation (PD+LRRK2+), 40 idiopathic PD without LRRK2 pathogenic mutations (PD+LRRK2‐), 16 non‐manifesting carriers of LRRK2 G2019S mutation (PD‐LRRK2+), and 35 healthy individuals without LRRK2 pathogenic mutations (PD‐LRRK2‐). There was no participant overlap between the LCC and CUIMC cohorts. In this cohort, UPLC‐MS/MS analysis was focused only on di‐oleoyl‐BMP (di‐18:1‐BMP), di‐22:6‐BMP, and their 2,2′ isoforms.
Broad Profiling of Bioactive Phospholipids in LCC Urine
UPLC‐MS/MS analyses
A multiplexed UPLC‐MS/MS method was used to simultaneously quantitate (semiquantitate) 54 species of urinary BMPs, PC, PE, and PI, and Gb3s with various fatty acid chains (Supplementary Tables 1–3). The analyses were conducted by Nextcea, Inc. (Woburn, MA) as previously described using a SCIEX TripleTOF 6600 mass spectrometer equipped with an IonDrive Turbo V source (SCIEXm Framingham, MA).11 Standard curves were prepared from related standards using a class‐based approach (ie, di‐22:6‐BMP, di‐myristoyl‐PC [di‐14:0‐PC], di‐14:0‐PE, di‐octanoyl‐PI [di‐8:0‐PI], and Gb3 d18:1/16:0, respectively). Internal standards were used for each analyte reported. Injections were made using a Shimadzu Nexera XR UPLC system (Shimadzu Scientific Instruments, Japan). A SCIEX TripleTOF 6600 mass spectrometer was used for the detection of analytes. The instruments were controlled by AnalystTF 1.7 software.
Calibration and data processing
The intensities of the analytes and internal standards were determined by integration of extracted ion peak areas using AnalystTF 1.7 and MultiQuant 3.0 software. Calibration curves were prepared by plotting the peak area ratios for each analyte to internal standard versus concentration. The model for the calibration curve was linear with (1/x2) weighting. Measured concentrations of urine lipids (ng/mL) were divided by the concentration of urine creatinine and reported as ng/mg creatinine.
Measurement of Urinary 2,2′‐di‐22:6‐BMP, Total di‐22:6‐BMP, 2,2′‐di‐18:1‐BMP, and Total di‐18:1‐BMP
BMPs can exist in 3 geometrical isoforms (2,2′‐, 2,3′‐, and 3,3′‐BMP), which may influence their functional properties.12, 13 Targeted high resolution UPLC‐MS/MS was used to accurately quantitate total di‐22:6‐BMP (the sum of its 3 isoforms) and its distinct 2,2′‐isoform (2,2′‐di‐22:6‐BMP). Total di‐18:1‐BMP and 2,2′‐di‐18:1‐BMP were measured as well. Quantitation was performed by Nextcea, Inc. (Woburn, MA) using authentic di‐22:6‐BMP and di‐18:1‐BMP reference standards. Di‐14:0‐BMP was employed as an internal standard. Urinary BMPs were extracted by liquid‐liquid extraction and measured by UPLC‐MS/MS as described above.
Creatinine measurement
Concentrations of urinary creatinine were measured by a colorimetric assay (method of Jaffé) with Parameter Creatinine Assay test reagents (R&D Systems, Minneapolis, MN) using a BioTek ELx800 absorbance microplate reader with Gen5 Microplate Reader and Imager Software 2.09 (Fisher Scientific, Hampton, NH).
Statistical Analyses
We used descriptive statistics to report findings from both LRRK2 cohorts. In both cohorts we used chi‐square for categorical variables and the Kruskal‐Wallis test for continuous variables to compare the 4 groups (PD+LRRK2+, PD+LRRK2‐, PD‐LRRK2+, and PD‐LRRK2‐). In the LCC cohort, we present the concentration of 54 analytes in supplementary files. For both cohorts, we used Kruskal‐Wallis to compare creatinine‐normalized BMP levels across the groups. Given that BMP levels in each of the groups were comparable between the 2 cohorts, we combined the data from both cohorts and compared the BMP analytes across the 4 groups in the merged database.
Last, in the CUIMC cohort in which UPDRS, MoCA, and l‐dopa‐equivalent daily dose (LEDD) were available, we tested the association between disease severity markers (predictors) and BMP isoforms (outcome) in linear regression models among PD patients. We first ran unadjusted models and then adjusted for covariates. When testing for the association between cognitive performance (by MoCA), we adjusted for age, sex, disease duration, education (years), and LRRK2 mutation status. When we tested the association between motor functioning (UPDRS‐III) and BMPs, we adjusted for age, sex, duration of disease, LEDD, and LRRK2 mutation status.
Results
Analyses of Biospecimens From the LCC Donors
The demographics and LRRK2 genotype status of LCC participants are described in Table Table1.1. As shown, the 4 groups were balanced for all demographic variables. The broad profiling of LCC cohort urine for 54 distinct analytes is reported in Supplemental Tables 1–3. These data showed that all BMP species measured and PI levels (but not Gb3 isoforms) were higher in LRRK2 G2019S mutation carriers independent of PD status and sex. A correlational analyses indicated that total di‐18:1‐BMP and total di‐22:6‐BMP most strongly discriminated the LRRK2 mutation carriers from non‐carriers (data not shown). Hence, all subsequent studies focused on analyzing total and 2,2′ isoforms of di‐18:1 BMP and di‐22:6 BMP. Creatinine‐normalized BMP isoforms were significantly elevated in LRRK2 G2019S mutation carriers independent of PD status and sex. BMP levels in urine of idiopathic PD patients (PD+LRRK2‐) did not differ from healthy controls (PD‐LRRK2‐). Note that urinary creatinine levels were consistent across the 4 groups. As a result, normalization of phospholipids to creatinine had no effect on the overall BMP concentration differences.
Table 1
Demographics and urine BMP concentrations among LCC participants
PD+LRRK2+ (n = 20) | PD+LRRK2‐ (n = 20) | PD‐LRRK2+ (n = 20) | PD‐LRRK2‐ (n = 20) | P | |
---|---|---|---|---|---|
Age, mean (SD) | 69.5 (9.9) | 70.7 (9.0) | 68 (12.4) | 71 (11.3) | 0.7622 |
Age at onset, mean (SD) | 58.2 (9.8) | 58.8 (11.3) | n/a | n/a | 0.9245b |
Disease duration | 11.4 (5.9) | 11.9 (6.2) | n/a | n/a | 0.6643b |
Sex (female/male) | 10/10 | 10/10 | 10/10 | 10/10 | 1 |
Total di‐18:1‐BMP, median (range) | 5.84 (1.50–28.97) | 2.80 (0.69–19.01) | 5.49 (1.05–24.98) | 4.79 (1.43–15.14) | 0.0084a |
Total di‐22:6‐BMP, median (range) | 35.25 (9.37–380.92) | 11.28 (2.06–100.01) | 33.80 (6.13–190.25) | 11.5 (3.97–67.20) | <0.0001a |
2,2′‐di‐18:1‐BMP, median (range) | 3.95 (1.20–23.42) | 2.21 (0.53–14.84) | 3.68 (0.770–20.12) | 3.27 (0.54–8.92) | 0.0407a |
2,2′‐di‐22:6 BMP, median (range) | 23.04 (6.81–302.84) | 7.21 (1.24–72.02) | 20.19 (3.29–142.91) | 6.11 (1.10–42.26) | <0.0001a |
Creatinine, median (range) | 1.10 (0.12–3.14) | 1.10 (0.07–3.19) | 1.07 (0.29–2.08) | 0.91 (0.36–2.58) | 0.7658 |
BMP values shown are median (range) of creatinine‐normalized isoforms (ng/mg creatinine). The P values were calculated using the Kruskal‐Wallis test for continuous variables (all variables other than sex, age of onset, and disease duration).
Analyses of Biospecimens From the CUIMC Participants
The demographics, disease characteristics, and BMP concentrations of the CUIMC participants are described in Table Table2.2. Similar to the LCC cohort, BMP isoforms were significantly elevated in urine from LRRK2 G2019S mutation carriers, and there were no significant differences in BMP levels in LRRK2 non‐carriers with or without PD. Twenty‐two participants in this cohort also carried a GBA1 mutation, including 4 who carried both a GBA mutation and the LRRK2 G2019S mutation.
Table 2
Demographics and urine BMP concentrations among CUIMC participants
PD+LRRK2+ (n = 25) | PD+LRRK2‐ (n = 40) | PD‐LRRK2+ (n = 16) | PD‐LRRK2‐ (n = 35) | P | |
---|---|---|---|---|---|
Age, mean (SD) | 69.4 (8.5) | 65.1 (9.3) | 56.8 (12.7) | 67.4 (10.4) | 0.0053 |
Age at onset, mean (SD) | 56.9 (11.3) | 57.9 (10.9) | n/a | n/a | 0.4298b |
Disease duration | 11 (1–26) | 7 (0–18) | n/a | n/a | 0.0026b |
Sex (% female) | 10 (40%) | 16 (40%) | 8 (50%) | 17 (48.6%) | 0.8127 |
UPDRS‐III, mean (SD) | 22.1 (10.3) | 16.9 (9.6) | 0.81 (1.04) | 1.08 (1.5) | <0.0001a |
MoCA, mean (SD) | 26.5 (4.7) | 26.9 (1.6) | 28.7 (1.1) | 27.5 (2.1) | 0.0080a |
LEDD | 547 (313.3) | 415 (375.7) | n/a | n/a | 0.097b |
Total di‐18:1‐BMP, median (range) | 5.81 (0–30.8) | 2.26 (0–18.5) | 3.43 (1.03–11.49) | 2.30 (0–8.5) | 0.0007a |
Total di‐22:6‐BMP, median (range) | 38.9 (9.36–134.5) | 10.5 (1.13–39.1) | 30.3 (5.16–61.9) | 8.29 (0–33.7) | <0.0001a |
2,2′‐di‐18:1‐BMP, median (range) | 4.56 (0–21.3) | 1.36 (0–12.69) | 2.36 (0.75–.31) | 1.15 (0–6.84) | <0.0001a |
2,2′‐di‐22:6 BMP, median (range) | 31.3 (6.73–106.42) | 5.72 (0.59–25.5) | 20.84 (4.35–35.62) | 5.29 (0–25.8) | <0.0001a |
Creatinine, median (range) | 0.87 (0.34) | 0.89 (0.59) | 0.72 (0.44) | 0.68 (0.56) | 0.0984 |
BMP values shown are median (range) of creatinine‐normalized isoforms (ng/mg creatinine). The P values were calculated using the Kruskal‐Wallis test for continuous variables (all variables other than sex, age at onset, and disease duration).
Analyses of BMP Isoforms in the Combined Cohorts
Table Table33 and Figure Figure1(A‐D)1(A‐D) demonstrate BMP analytes by LRRK2 genotype and PD status in the combined cohorts. Interestingly, the normalized levels of di‐18:1‐BMP, di‐22:6‐BMP, and their 2,2′ isoforms were ~20%–30% higher in the LRRK2 G2019S mutation carriers with PD when compared with those without PD, although only the 2,2′‐di‐18:1‐BMP isoform reached marginal statistical significance (P = 0.0459).
Table 3
Median BMP analyte levels in the combined cohort
PD+LRRK2+ (n = 45) | PD+LRRK2‐ (n = 60) | PD‐LRRK2+ (n = 36) | PD‐LRRK2‐ (n = 55) | P | P b | |
---|---|---|---|---|---|---|
Total di‐18:1‐BMP | 5.81 (0–30.85) | 2.31 (0–19.1) | 4.91 (1.03–24.9) | 1.63 (0–8) | <0.0001a | 0.1061 |
Total di‐22:6‐BMP | 37.4 (9.36–380.92) | 10.5 (1.13–100.01) | 31.5 (5.16–190.3) | 9.23 (0–67) | 0.0004a | 0.1802 |
2,2′‐di‐18:1‐BMP | 4.25 (0–23.42) | 1.39 (0–14.84) | 3.15 (0.75–20.12) | 1.62 (0–8.92) | <0.0001a | 0.0459a |
2,2′‐di‐22:6‐BMP | 26.2 (6.73–302.8) | 6.15 (0.59–72.02) | 20.5 (3.29–142.9) | 5.38 (0–42.26) | <0.0001a | 0.0871 |
BMP values shown are median (range) of creatinine‐normalized isoforms (ng/mg creatinine). The P values were calculated using the Kruskal‐Wallis test.
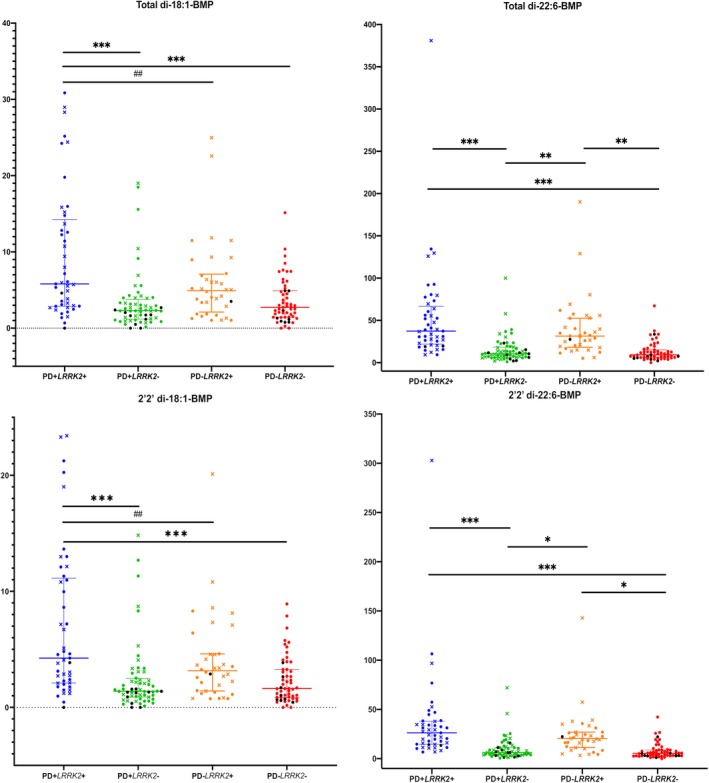
Levels of BMP isoforms in the combined LCC and CUIMC data sets. (A–D) Data for a specific BMP isoform measured in the 4 groups indicated on the X axis. Circular symbols indicate the measurement was from the CUIMC cohort, and X symbols indicate the measurement was from the LCC cohort. Any symbol in black is a GBA mutation/variant carrier. Wilcoxon rank sum test was used for pairwise comparisons to identify groups differing from each other. *P<
0.05, **P
<
0.005, ***P
<
0.0001; ##P
<
0.01.
BMP and PD Phenotypes
Last, in the CUIMC cohort, we tested the association between creatinine‐normalized urine BMP levels and PD severity scales, UPDRS, and MoCA either unadjusted (model 1) or adjusted (model 2). MoCA score was negatively associated with creatinine‐normalized 2,2′ and total di‐22:6‐BMP isoforms, in both models (Table (Table4).4). For example, for every point increase in the MoCA score the total di‐22:6‐BMP decreases by 1.76 (adjusting for age, sex, disease duration, education years, and LRRK2 mutation status).
Table 4
Assessing MoCA and UPDRS as predictors of BMP levels in PD patients (n = 65)
Model 1 | Model 2 | |||
---|---|---|---|---|
Beta (SE) | P | Beta (SE) | P | |
MoCA | ||||
Normalized total di‐18:1‐BMP | 0.185 (0.26) | 0.4875 | 0.34 (0.27) | 0.2226 |
Normalized total di‐22:6‐BMP | −2.48 (0.98) | 0.0144a | −1.76 (0.86) | 0.0461a |
Normalized 2,2′‐di‐18:1‐BMP | 0.121 (0.19) | 0.5246 | 0.26 (0.19) | 0.1900 |
Normalized 2,2′‐di‐22:6‐BMP | −2.14 (0.70) | 0.0033a | −1.60 (0.61) | 0.0104 |
UPDRS‐III | ||||
Normalized total di‐18:1‐BMP | 0.14 (0.08) | 0.0878 | 0.07 (0.09) | 0.4416 |
Normalized total di‐22:6‐BMP | 0.40 (0.32) | 0.2129 | −0.13 (0.27) | 0.6396 |
Normalized 2,2′‐di‐18:1‐BMP | 0.09 (0.06) | 0.1079 | 0.04 (0.06) | 0.5265 |
Normalized 2,2′‐di‐22:6‐BMP | 0.29 (0.23) | 0.2222 | −0.12 (0.20) | 0.5424 |
Model 1, unadjusted; model 2, adjusted for covariates as indicated below.
MoCA, adjusted for age, sex, disease duration, education years, and LRRK2 status.
UPDRS‐III, adjusted for age, sex, disease duration, LEDD, and LRRK2 status.
Discussion
In the current study we demonstrated higher urine BMP isoform concentrations in carriers of LRRK2 G2019S mutation than in non‐carriers, independent of PD status and sex in 2 independent cohorts. Furthermore, in the combined cohort analyses, a small but statistically significant increase in 2,2′‐di‐18:1 BMP isoform was seen in PD+LRRK2+ when compared with PD‐LRRK2+. Finally, among PD patients, higher di‐22:6‐BMP (total levels and 2,2′ isoform levels) were associated with worse cognitive performance on the MoCA suggesting that BMP isoforms may be biomarkers of pathophysiology of PD. These data indicate the potential of urinary BMP isoforms as biomarkers of LRRK2 biology, PD status, and symptoms.
BMP is a structural isomer of phosphatidylglycerol found in most tissues and in different cell types. BMP is negatively charged at the acidic pH of lysosomes, and these charges are central to its role in the degradation of lipids and membranes in the lysosome by facilitating the adhesion of the soluble positively charged hydrolases (eg, glucocerebrosidase) and activator proteins (saposins) at the interface of the lysosomal inner membranes.6 Alterations in BMP levels have been linked to lysosomal dysfunction. Both di‐18:1‐BMP and di‐22:6‐BMP are elevated in patients with Niemann‐Pick disease,11, 14 a lysosomal disorder caused by diminished acid sphingomyelinase levels. Interestingly, variants in the gene encoding sphingomyelinase are also risk factors for PD.15
In this study, urinary di‐18:1‐BMP and di‐22:6‐BMP and their 2,2′ isoforms were elevated in LRRK2 G2019S mutation carriers (with and without PD), compared with non‐carriers. In contrast, mice with germline deletion of LRRK2 and cynomolgus monkeys treated with 3 distinct LRRK2 kinase inhibitors show decreases in urinary di‐22:6‐BMP levels.9 Moreover, urinary di‐22:6‐BMP levels were restored to normal concentrations on withdrawal from treatment with a LRRK2 kinase inhibitor.9 Because the G2019S mutation increases LRRK2 kinase activity 2‐3X,3 our data could suggest that the elevation in urinary di‐22:6‐BMP levels may reflect the kinase activity of LRRK2. However, the animal studies cited above also showed lysosomal alterations in both rodents and non‐human primates. Furthermore, elevated BMP levels in Niemann‐Pick C patients indicate that changes in BMP levels may not be specific to LRRK2 activity, but may also represent lysosomal dysfunction. Therefore, additional studies are required to determine whether urinary BMP levels reflect LRRK2 kinase activity and/or endolysosomal deficits. For example, studying alterations in BMP levels in GBA mutation carriers may provide critical insights into upstream mechanisms of regulation of urinary BMP levels in humans.
What is a possible molecular mechanism underlying the observed increases in urine from LRRK2 G2019S carriers? Recent studies have demonstrated that a subset of Rab GTPases is phosphorylated by LRRK2 kinase activity.8 Rab GTPases modulate the maturation/formation of MVBs, vesicular trafficking, and exocytosis of MVB‐derived vesicles (exosomes) at the plasma membrane.16 A consequence of higher kinase activity of G2019S LRRK2 will be increased phosphorylation of its Rab GTPase substrates and thereby their accumulation in membranes as shown by Steger et al.8 We propose that this shift in membrane‐bound Rabs may affect the biogenesis, motility, or extracellular release of exosomes, resulting in increased concentrations of BMPs in urine. One candidate LRRK2 Rab GTPase substrate contributing to the observed increases in urinary BMP is Rab35, because it has been shown to regulate the endocytic/recycling pathway and secretion.17
As LRRK2 kinase inhibitors move forward into the clinic, better strategies are needed to enrich clinical trials with those most likely to benefit because of elevated LRRK2 activity and evaluate pharmacodynamic effects of the drug. One obvious patient population is those carrying kinase‐activating mutations of LRRK2, such as G2019S. However, in light of recent data indicating that LRRK2 may also play a role in the pathogenesis of iPD,4 a biomarker to identify iPD patients with increased LRRK2 kinase activity is required to enrich clinical trials with the patients most likely to respond to LRRK2 inhibitors (ie, those with higher LRRK2 kinase activity). Our data indicate the potential utility of urinary di‐22:6‐BMP and di‐18:1‐BMP as non‐invasive, quantitative, and relatively facile measures to monitor LRRK2 kinase activity. A preliminary but intriguing observation of the present study was that BMP levels were higher in LRRK2 mutation carriers with PD compared with those without PD and that among PD patients higher di‐22:6‐BMP levels predicted worse cognitive performance, even after adjustment for covariates. These data raise the question of whether urine BMP levels may also offer a marker of disease state and progression. Of note, LRRK2 carriers without PD were not screened for non‐motor symptoms or for dopamine deficiency on dopamine transporter (DAT) scans. It is critical to assess whether urinary BMP levels are associated with preclinical signs of PD such as abnormal DaT scans or non‐motor symptoms (eg, hyposmia). Studies are underway to monitor BMP isoform levels in LRRK2‐PD and iPD patients at baseline and longitudinally in the deeply phenotyped cohort of Parkinson's Progression Markers Initiative to directly assess the possibility of urinary BMP as a state and/or progression marker of PD.
Urinary BMP levels also appear to be a viable pharmacodynamic biomarker of LRRK2 kinase inhibitors. Indeed, the non‐human primate studies discussed above9 support this contention because these studies demonstrated decreases in urinary di‐22:6‐BMP levels following treatment with 3 distinct LRRK2 kinase inhibitors and its restoration to normal levels following treatment cessation.9 At this point, the tissue source of BMP in the urine remains unclear. BMP‐enriched exosomes may be secreted from a variety of cells and tissue types in which LRRK2 is natively expressed. Future preclinical studies assessing urinary BMP levels in combination with markers of brain LRRK2 kinase inhibition (eg, pSer1292‐LRRK2, p‐Rabs) and detailed pharmacokinetics in plasma, brain, and cerebrospinal fluid are needed to determine whether urinary BMP levels may be extrapolated to brain LRRK2 inhibition. It is noteworthy that urine contains brain‐derived biomarkers such as neurofilament proteins and brain‐specific Rab GTPases, possibly because of the excretion of brain‐generated exosomes in the urine. Thus, the possibility that BMP levels in the urine reflect altered endolysosomal function in the brain also needs to be further investigated.
In conclusion, using 2 independent cohorts and quantitative mass spectrometric assays we have provided robust evidence that specific BMP species in urine are associated with G2019S mutations in the LRRK2 gene. The biology of BMP suggests that the alterations in urine BMP levels may indicate deficits in endolysosomal function. An exciting preliminary finding is that BMP levels are also state markers because LRRK2 carriers with PD had higher BMP levels than those without PD. Moreover, increased total di‐18:1‐BMP and total di‐22:6‐BMP were associated with worse cognitive performance by MoCA. Thus, the results described here open avenues for future studies to establish the utility of urinary BMP as a biomarker to monitor disease progression and as a pharmacodynamic biomarker to demonstrate LRRK2 kinase modulation by drug candidates.
Author Roles
1) Research project: A. Conception, B. Organization, C. Execution;
2) Statistical Analysis: A. Design, B. Execution, C. Review and Critique;
3) Manuscript: A. Writing of the first draft, B. Review and Critique.
Roy N. Alcalay: 1A, 1B, 1C, 2C, 3A.
Frank Hsieh: 1B, 1C, 2C, 3A.
Elizabeth Tengstrand: 1C, 2C, 3B.
Shalini Padmanabhan: 1B, 3A, 3B.
Marco Baptista: 1B, 3A, 3B.
Caitlin Kehoe: 1B, 3B.
Sushma Narayan: 1B, 3B.
Amelia K. Boehme: 2A, 2B, 3B.
Kalpana Merchant: 1A, 1B, 2C, 3A.
Full financial disclosure for the previous 12 months
Roy N. Alcalay: Received research funding from the NIH, Parkinson's Foundation, and the Michael J. Fox Foundation. Consultation fees from ResTORbio, Genzyme/Sanofi, Roche.
Frank Hsieh: employee of Nextcea.
Elizabeth Tengstrand: employee of Nextcea.
Shalini Padmanabhan: employee of te Michael J. Fox Foundation.
Marco Baptista: employee of the Michael J. Fox Foundation.
Caitlin Kehoe: nothing to disclose.
Sushma Narayan: nothing to disclose.
Amelia K. Boehme: nothing to disclose.
Kalpana Merchant: received consulting fees from Lysosomal Therapeutics, Sinopia Biosciences, Michael J. Fox Foundation and angel investors and shares from Origami Therapeutics, Sinopia Biosciences. Paid for contractual engagement in the capacity of chief scientific officer at Vincere Biosciences, Inc. Received research funding from the Michael J. Fox Foundation and honoraria from the Wellcome Trust as a member of the interview panel. The data disclosed here constitute no conflict of interest.
Supporting information
Supplement Table 1: Broad profiling of bis(monoacylglycerol)phosphate (BMPs, ng/mg creatinine) in LCC Urine
Supplement Table 2: Broad profiling of Phospholipids in LCC Urine
Supplement Table 3: Preliminary analyses of Globotriaosylceramides (Gb3s) in LCC urine
Notes
Relevant conflicts of interest/financial disclosures: Dr. Hsieh and Ms. Tengstrand are employed by Nextcea, Inc., which holds patent rights to the di‐22:6‐BMP and 2,2′‐di‐22:6‐BMP biomarkers for neurological diseases involving lysosomal dysfunction (US 8,313,949, Japan 5,702,363, and Europe EP2419742).
Funding agencies: This research was funded by the Michael J. Fox Foundation.
Contributor Information
Roy N. Alcalay, Email: ude.aibmuloc.cmuc@4012anr.
Kalpana Merchant, Email: [email protected].
References
Full text links
Read article at publisher's site: https://doi.org/10.1002/mds.27818
Read article for free, from open access legal sources, via Unpaywall:
https://www.ncbi.nlm.nih.gov/pmc/articles/PMC6981003
Citations & impact
Impact metrics
Citations of article over time
Alternative metrics
Article citations
Biomarkers of Parkinson's Disease: From Basic Research to Clinical Practice.
Aging Dis, 15(4):1813-1830, 01 Aug 2024
Cited by: 5 articles | PMID: 37815899 | PMCID: PMC11272192
Review Free full text in Europe PMC
Navigating the metabolic maze: anomalies in fatty acid and cholesterol processes in Alzheimer's astrocytes.
Alzheimers Res Ther, 16(1):63, 23 Mar 2024
Cited by: 1 article | PMID: 38521950 | PMCID: PMC10960454
Review Free full text in Europe PMC
The Impact of 90 Parkinson's Disease-Risk Single Nucleotide Polymorphisms on Urinary Bis(monoacylglycerol)phosphate Levels in the Prodromal and PD Cohorts.
Int J Mol Sci, 25(4):2286, 14 Feb 2024
Cited by: 0 articles | PMID: 38396963 | PMCID: PMC10889274
A potential patient stratification biomarker for Parkinson´s disease based on LRRK2 kinase-mediated centrosomal alterations in peripheral blood-derived cells.
NPJ Parkinsons Dis, 10(1):12, 08 Jan 2024
Cited by: 2 articles | PMID: 38191886 | PMCID: PMC10774440
Lipids as Emerging Biomarkers in Neurodegenerative Diseases.
Int J Mol Sci, 25(1):131, 21 Dec 2023
Cited by: 3 articles | PMID: 38203300 | PMCID: PMC10778656
Review Free full text in Europe PMC
Go to all (34) article citations
Data
Data behind the article
This data has been text mined from the article, or deposited into data resources.
BioStudies: supplemental material and supporting data
Similar Articles
To arrive at the top five similar articles we use a word-weighted algorithm to compare words from the Title and Abstract of each citation.
Differential Phospho-Signatures in Blood Cells Identify LRRK2 G2019S Carriers in Parkinson's Disease.
Mov Disord, 37(5):1004-1015, 20 Jan 2022
Cited by: 6 articles | PMID: 35049090 | PMCID: PMC9306798
Motor and non-motor features of Parkinson's disease in LRRK2 G2019S carriers versus matched controls.
J Neurol Sci, 388:203-207, 17 Mar 2018
Cited by: 6 articles | PMID: 29627023 | PMCID: PMC5908224
Exhaustion of mitochondrial and autophagic reserve may contribute to the development of LRRK2 G2019S -Parkinson's disease.
J Transl Med, 16(1):160, 08 Jun 2018
Cited by: 18 articles | PMID: 29884186 | PMCID: PMC5994110
Clinical Features of LRRK2 Carriers with Parkinson's Disease.
Adv Neurobiol, 14:31-48, 01 Jan 2017
Cited by: 31 articles | PMID: 28353277
Review