Abstract
Free full text

PAI-1 Regulation of TGF-β1–induced Alveolar Type II Cell Senescence, SASP Secretion, and SASP-mediated Activation of Alveolar Macrophages
Associated Data
Abstract
Senescence of alveolar type II (ATII) cells, progenitors of the alveolar epithelium, is a pathological feature and contributes importantly to the pathogenesis of idiopathic pulmonary fibrosis. Despite recognition of the importance of ATII cell senescence in idiopathic pulmonary fibrosis pathogenesis, how ATII cell senescence is regulated and how senescent ATII cells contribute to lung fibrogenesis remain unclear. In this study, we show that TGF-β1 (transforming growth factor-β1), a most ubiquitous and potent profibrotic cytokine, induces plasminogen activator inhibitor-1 (PAI-1), a cell senescence and fibrosis mediator, and p16 as well as senescence, but not apoptosis, in primary mouse ATII cells. We also found that senescent ATII cells secrete various cytokines and chemokines, including IL-4 and IL-13, which stimulate the expression of genes associated with a profibrotic phenotype in alveolar macrophages. Similar responses were also observed in TGF-β1–treated rat ATII (L2) and rat macrophage NR8383 cells. Deletion of PAI-1 or inhibition of PAI-1 activity with a small molecule PAI-1 inhibitor, however, blocks TGF-β1–induced senescence as well as a senescence-associated secretory phenotype in ATII and L2 cells and, consequently, the stimulatory effects of the conditioned medium from senescent ATII/L2 cells on macrophages. Moreover, we show that silencing p16 ameliorates PAI-1 protein–induced ATII cell senescence and secretion of profibrotic mediators. Our data suggest that PAI-1 mediates TGF-β1–induced ATII cell senescence and secretion of profibrotic mediators through inducing p16, and they also suggest that senescent ATII cells contribute to lung fibrogenesis in part by activating alveolar macrophages through secreting profibrotic and proinflammatory mediators.
Alveolar type II (ATII) cells are progenitors of the alveolar epithelium and play an essential role in maintaining alveolar structure and function (1–3). There is overwhelming evidence showing that ATII cells undergo senescence in idiopathic pulmonary fibrosis (IPF), an aging-related progressive fatal lung disorder, and in experimental lung fibrosis models (4–9). Despite increasing appreciation of an important role of ATII cell senescence in the pathogenesis of fibrotic lung diseases (10–13), little is known about how ATII senescence is regulated in fibrotic lung and how senescent cells contribute to the pathogenesis of lung fibrosis.
PAI-1, a physiological inhibitor of tissue-type and urokinase-type plasminogen activators, has multiple functions and is involved in the regulation of cell adhesion, migration, proliferation, and apoptosis. Studies at our laboratory and others have shown that PAI-1 plays a pivotal role in the development of lung fibrosis, although the underlying mechanisms are still not entirely understood (14–18). PAI-1 has long been used as a cell senescence marker. Emerging evidence suggests that PAI-1 is not merely a marker but also a mediator of cell senescence (19–24). In a recent study, on the one hand, we showed that PAI-1 was highly induced in ATII cells in IPF and in a bleomycin-induced lung fibrosis model; ablation of PAI-1 in ATII cells in PAI-1 conditional knockout mice, on the other hand, almost abolished bleomycin-induced ATII cell senescence and lung fibrosis (18). Our data suggest that increased PAI-1 may underlie ATII cell senescence in fibrotic lung and that senescent ATII cells contribute importantly to lung fibrogenesis. Nonetheless, whether TGF-β1 (transforming growth factor-β1), a most ubiquitous and potent profibrogenic cytokine, induces ATII cell senescence and whether PAI-1 mediates the TGF-β1 effect are unknown.
Senescent ATII cells not only lose normal physiological functions and reparative capacity but also secrete an array of bioactive molecules, collectively called the “senescence-associated secretory phenotype” (SASP). These bioactive molecules can modulate the function of secreting cells (autocrine functions) as well as adjacent cells (paracrine function). Alveolar macrophages (AMs) are the major type of cells residing in alveoli under unchallenged conditions and function as the first line of defense against inhaled infectious agents and environmental pollutants. Macrophages can be activated by various cytokines, chemokines, and growth factors as well as other biological agents. Macrophages activated by IFN-γ and/or LPS are historically called M1 or classically activated macrophages, whereas macrophages activated by T-helper type 2 (Th2) cytokines IL-4 and/or IL-13 are often referred to as M2 or alternatively activated macrophages (25–28). It is believed in general that M1 macrophages participate in the eradication of bacterial and viral infection, whereas M2 macrophages play a critical role in host defense against parasite infection, tumor progression, and tissue remodeling (26, 27, 29). Whether ATII SASP is involved in AM activation, M1 and/or M2, in fibrotic lung has not been reported. Moreover, whether ATII PAI-1 modulates ATII SASP components and therefore AM activation is also unknown.
In this study, we addressed two issues: 1) whether TGF-β1, the most potent and ubiquitous profibrogenic cytokine, induces ATII cell senescence and the role of ATII PAI-1 in the process; and 2) whether ATII SASP promotes profibrotic polarization of AMs and the effect of ATII PAI-1 on ATII SASP secretion as well as macrophage activation. Both primary cells and cell lines were used to address these issues. The findings reported in this study suggest that PAI-1 mediates TGF-β1–induced ATII cell senescence and SASP secretion through inducing p16 and that ATII SASP promotes a profibrotic phenotype of AMs.
Methods
Isolation of Mouse Lung ATII Cells and AMs
Murine ATII epithelial cells were isolated from adult mice (6–12 wk old) according to a protocol we have described previously (18). Briefly, mouse lungs were digested with protease solution (300 U/ml collagenase type I, 4 U/ml elastase, 5 U/ml dispase, and 100 μg/ml DNase I in Hanks’ balanced salt solution) at 37°C for 25 minutes and then with 0.1% trypsin-EDTA and 100 μg/ml deoxyribonuclease I at 37°C for 20 minutes. After tissue dissociation, single-cell suspensions were prepared by passing the lung digestions through a 40-μm mesh size cell strainer. Red blood cells were lysed, and then macrophages and lymphocytes were removed by incubation with biotinylated rat antimouse CD45 and rat antimouse CD16/32 (BD Biosciences). The cells were then cultured with Dulbecco’s modified Eagle medium/Nutrient Mixture F-12 (DMEM/F-12) medium containing 10% FBS in 100-mm culture dishes at 37°C overnight to remove fibroblasts. The suspended cells were harvested for further culture and treatment. Immunostaining with anti-SPC (anti–surfactant protein C) antibody confirms that greater than 90% of isolated cells are ATII cells (18). AMs were isolated from mice by BAL and cultured with RPMI medium containing 10% FBS, 2 mM l-glutamine, 100 IU/ml penicillin, and 100 μg/ml streptomycin. The purity of isolated cells was assessed by flow cytometric analysis after immunostaining with CD64 antibody (CD64-phycoerythrin [PE]-Vio770) or REA isotope control antibody (REA-control-PE-Vio770; BD Biosciences) according to the manufacturer’s protocol. The results showed that more than 90% of isolated cells were macrophages (Figure E1 in the data supplement). All procedures involving animals were approved by the institutional animal care and use committees at the University of Alabama at Birmingham (UAB) and conducted at the UAB animal facilities under specific pathogen–free conditions.
Cell Culture and Treatment
Primary ATII cells were cultured with DMEM/F-12 medium containing 10% FBS, 100 U/ml penicillin, and 100 μg/ml streptomycin at 5% CO2 and 37°C. Primary rat lung epithelial (L2) cells (American Type Culture Collection) stably transduced with nontarget shRNA or PAI-1 shRNA (18) were cultured with Ham’s F-12 medium containing 10% FBS and antibiotics. ATII cells and L2 cells were treated with 2 ng/ml of TGF-β1 in serum-free medium for 48 hours and then cultured in TGF-β1–free and serum-free medium for an additional 24 hours. Cells were then harvested for analysis of senescence markers, and the conditioned medium (CM) was collected, mixed with RPMI medium (1:1), and used for the treatment of AMs or NR8383 cells, a rat macrophage cell line. The treatment conditions were selected on the basis of our preliminary studies, which showed that the secretion of senescent markers IL-6 and IGFBP3 (insulin-like growth factor–binding protein 3) was not increased until 48 hours after TGF-β1 treatment, whereas the activity of caspase 3/7, an apoptosis marker, increased after 72 hours of TGF-β1 treatment (Figure E2).
Determination of Activity of Senescence-associated β-Galactosidase in ATII and L2 Cells
The activity of senescence-associated β-galactosidase (SA-β-gal) was determined using 5-bromo-4-chloro-3-indolyl β-d-galactoside (X-gal), following a protocol described previously (18). Briefly, cells were fixed in 2% formaldehyde plus 0.2% glutaraldehyde in PBS at room temperature for 10 minutes, rinsed with PBS, and then incubated overnight in SA-β-gal staining solution containing 1 mg/ml X-gal. SA-β-gal–positive cells (blue color) were counted under a microscope, and the results were expressed as the percentage of total cells.
Western Blot Analysis
Cells were lysed in radioimmunoprecipitation assay buffer containing protease inhibitor cocktail (P8340; Sigma-Aldrich) and phosphatase inhibitor cocktail (P5726; Sigma-Aldrich) and centrifuged at 13,000×
g at 4°C for 10 minutes. The protein concentrations were determined by bicinchoninic acid assay, and Western blot analysis was conducted with 50 μg of proteins from each sample as we described previously (16, 17). The membranes were probed with the following antibodies at 4°C overnight: PAI-1 (ASMPAI-GF, ASRPAI-GF; Molecular Innovation), p16 (10883-1-AP; Proteintech), p53 (SC-6243; Santa Cruz Biotechnology), pRB (phosphorylated retinoblastoma protein, CN 8180; Cell Signaling Technology), Bax (CN 2772S; Cell Signaling Technology), cleaved caspase 3 (CN 9661X; Cell Signaling Technology), GAPDH (G9545; Sigma-Aldrich), and β-actin (A5441; Sigma-Aldrich). The protein bands were visualized using enhanced chemiluminescence detection reagent (Chemiluminescent ECL Substrate [WBKLS0500 Millipore Sigma]) and an Odyssey infrared imager (model 2800 S/N OFC-0172; LI-COR Biosciences); the results were normalized by β-actin or GAPDH band intensity.
ELISAs of Cytokines, Chemokines, and Growth Factors in CM
ELISAs were performed to determine the amounts of cytokines, chemokines, and growth factors in the CM from ATII/L2 cells using ELISA kits from R&D Systems, according to the manufacturer’s protocols.
Measurement of Caspase 3/7 Activity in CM
The activity of caspase 3/7 in CM was assessed using an assay kit from Promega (Caspase-Glo 3/7 Assay) according to the manufacturer’s protocol.
Quantitative Real-Time PCR Analysis of mRNAs
Total RNA was isolated from macrophages using TRIzol reagent. The purities and concentrations of the isolated RNA were assessed using a NanoDrop spectrophotometer. cDNA was synthesized using a high-capacity cDNA kit (Invitrogen), and quantitative real-time PCRs (qRT-PCRs) were performed using RT-PCR primers (Life Technologies) as listed in Table E1 and the LightCycler 480 System (Roche Life Science) as described previously (30, 31). Triplicates were analyzed for each of the samples, and average numbers were used for calculation. The comparative threshold cycle method, also known as the Ct method, was used to calculate relative mRNA concentrations according to the equations described previously (30).
siRNA Transfection
Freshly isolated mouse ATII cells were seeded at a density of 2×
105 cells per well in 6-well culture plates with DMEM/F-12 containing 10% serum and antibiotics. Cells were transfected with 25 nM control (nontargeting) siRNA or mouse p16 siRNA (Dharmacon) using Lipofectamine RNAiMAX reagent (catalog no. 13778030; Life Technologies), following the manufacturer’s protocol. After 48-hour culture, the medium was replaced, and cells were treated with active PAI-1 (PAI-A; Molecular Innovation) at a concentration of 1 μg/ml for 48 hours.
Statistics
Data are expressed as mean±
SD. We performed t tests for two-group comparisons, and one-way ANOVA was conducted for four-group comparisons. Statistical significance was determined post hoc by Tukey’s test.
Results
TGF-β1 Activated p16-pRb Pathway and Induced Senescence and Secretion of Profibrotic and Proinflammatory Mediators in Primary Mouse ATII Cells
TGF-β1 is a most ubiquitous and potent profibrogenic mediator. Whether TGF-β1 induces ATII cell senescence has not been reported yet. We show, in these experiments, that treatment of primary mouse ATII cells with 2 ng/ml of TGF-β1 increased the activity of SA-β-gal (Figures 1A and 1B) as well as the protein concentrations of PAI-1 and p16 in these cells (Figures 1C and 1D). This was associated with a decrease in pRb (Figures 1C and 1D). Under the treatment conditions, however, TGF-β1 had no significant effect on the expression or activity of caspase 3/7, an apoptosis marker (Figures 1C–1E). ELISA results further show that TGF-β1 treatment increased the secretion of IL-6, IGFBP3, and PAI-1, three senescence markers (Figures 1F–1H). Importantly, TGF-β1 treatment increases the secretion of IL-4 and IL-13, two Th2 cell cytokines that induce alternative activation of macrophages (32), as well as PDGF-BB (platelet-derived growth factor), a profibrogenic mediator (Figures 1I–1K). The amount of PAI-1 in the CM (ng/ml) was 10- to 100-fold higher than other SASP components measured, which were in the picograms per milliliter range (Figures 1F–1K). Together, these results suggest that TGF-β1, under the treatment conditions, induces ATII cell senescence but not apoptosis and that senescent ATII cells secrete large amounts of PAI-1 as well as other profibrotic mediators.
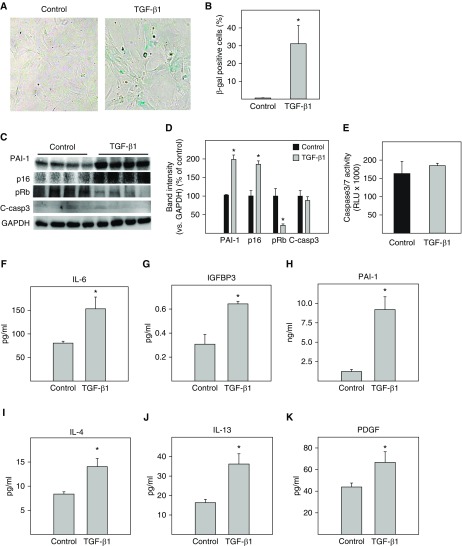
TGF-β1 (transforming growth factor-β1) activated the p16-pRb pathway and induced senescence as well as secretion of profibrotic mediators in primary mouse alveolar type II (ATII) cells. Freshly isolated mouse ATII cells were treated with 2 ng/ml of TGF-β1 in serum-free medium for 48 hours and then incubated with TGF-β1–free and serum-free medium for an additional 24 hours. (A and B) Senescence-associated β-galactosidase (SA-β-gal) activity was revealed by 5-bromo-4-chloro-3-indolyl β-d-galactoside (X-gal) staining. (C and D) Western analyses of the proteins of interest in isolated ATII cells. Representative Western blots (C). Quantification of the band intensities, normalized by GAPDH (D). (E) Caspase 3/7 activity in the conditioned medium (CM) was measured with a kit from Promega. (F–K) The amounts of different cytokines, chemokines, and growth factors in the CM were assessed by ELISA. *Significantly different from untreated ATII cells (P<
0.05; n
=
3–6). C-casp3
=
cleaved caspase 3; IGFBP3
=
insulin-like growth factor–binding protein 3; PAI-1 = plasminogen activator inhibitor-1; PDGF
=
platelet-derived growth factor; pRB
=
phosphorylated retinoblastoma protein; RLU
=
relative luminescence units.
Deletion of PAI-1 Blocked TGF-β1–induced ATII Cell Senescence and Secretion of Profibrotic Mediators
Our previous studies showed that PAI-1 mediated bleomycin-induced ATII cell senescence through activating p53-p21-pRb pathways (18). To determine whether PAI-1 is also involved in TGF-β1–induced ATII cell senescence and the potential underlying mechanism, ATII cells isolated from PAI-1–deficient (PAI-1−/−) and wild-type (PAI-1+/+) mice were treated with TGF-β1. The results show that deletion of PAI-1 in ATII cells abolished TGF-β1–stimulated activity of SA-β-gal (Figures 2A and 2B) as well as accumulation of p16 (Figures 2D and 2F). TGF-β1 treatment had no significant effect on the activity of caspase 3/7 (Figure 2C) or the expression of p53, Bax, and cleaved caspase 3 protein (Figures 2D and 2G–2I), although deletion of PAI-1 reduced the basal level of caspase 3/7 activity and Bax expression (Figures 2C, 2D, and 2G–2I). Our data also show that, associated with the suppression of ATII cell senescence, deletion of ATII PAI-1 abolished TGF-β1–stimulated secretion of IL-6, IL-4, IL-13, and PDGF (Figure 3). These data further suggest that TGF-β1, under the treatment conditions, induces ATII cell senescence but not apoptosis and that TGF-β1 promotes ATII cell senescence through induction of PAI-1 and thus activation of the p16-pRb, but not p53, cell cycle repression pathway.
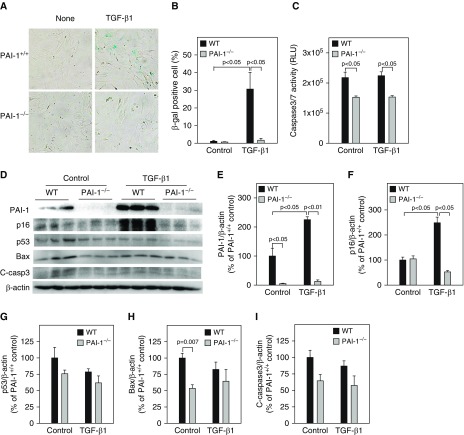
Deletion of PAI-1 blocked TGF-β1–induced ATII cell senescence. ATII cells isolated from wild-type (WT) and PAI-1–knockout (PAI-1−/−) mice were treated with TGF-β1 as described in Figure 1. (A and B) SA-β-gal activity was revealed by X-gal staining. (C) Caspase 3/7 activity in the CM was measured with a kit from Promega. (D–I) Western blot analyses of the proteins of interest in ATII cells. (D) Representative Western blots. (E–I) Quantification of the band intensities, normalized by β-actin (n=
3–4).
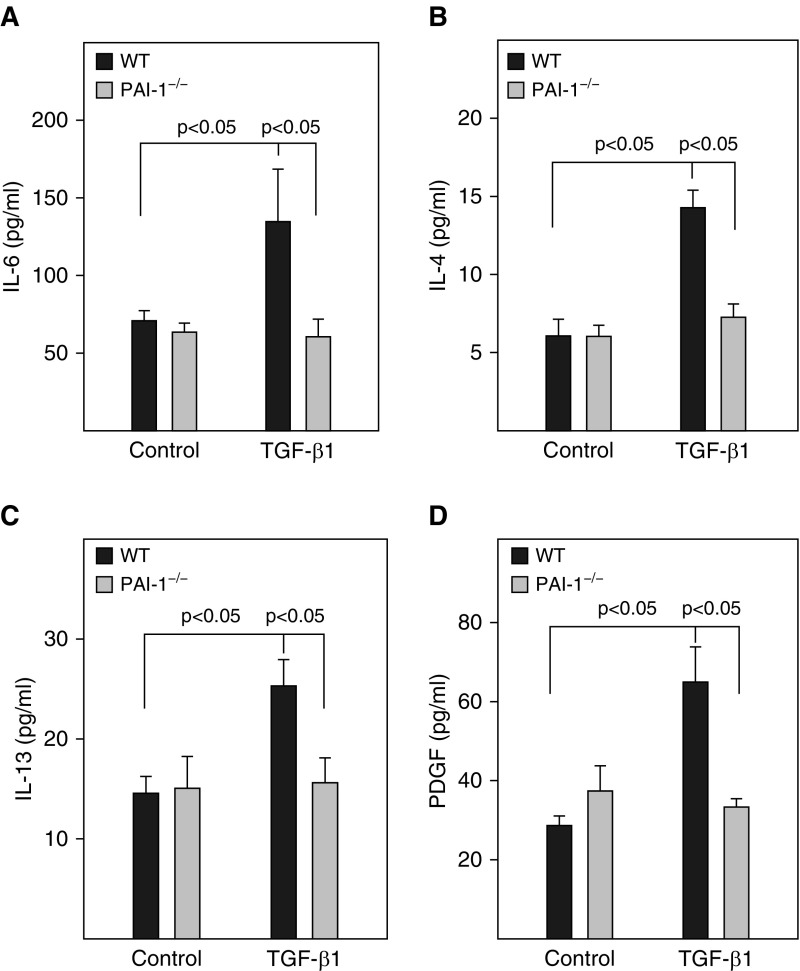
Deletion of PAI-1 in ATII cells blocked TGF-β1–induced secretion of profibrotic mediators. ATII cells isolated from WT and PAI-1−/− mice were treated with TGF-β1, and the CM was collected at Day 3 after TGF-β1 treatment as described in Figure 1. (A–D) The amounts of IL-4, IL-6, IL-13, and PDGF in the CM were measured by ELISA (n=
3–6).
Deletion of PAI-1 in ATII Cells Blunted the Stimulatory Effect of CM from Senescent ATII Cells on AM Gene Expression
To determine whether ATII SASP modulates AM gene expression and the effect of ATII PAI-1, we treated primary AMs with the CM from TGF-β1–stimulated senescent, not apoptotic, ATII cells and analyzed gene expression in AMs by qRT-PCR. The results show that incubation of primary AMs with the CM from senescent ATII cells induced an expression profile of selected genes (Figure 4) that resembles the gene expression profile of AMs in lungs of mice with experimental fibrosis (33). These include found in inflammatory zone 1 (Fizz1, also known as Retnla) and Ym1 (also known as chitinase-like 3), two macrophage alternative activation markers; STAT6 (signal transducer and activator of transcription 6), a transcription factor mediating IL-4– and IL-13–induced macrophage alternative activation; and inducible nitric oxide synthase (iNOS), TNF-α, IL-4, IL-6, IL-13, and TGF-β1 (Figure 4). Importantly, the stimulatory effects of ATII cell SASP on AM gene expression were completely or partially lost when AMs were cultured with the CM from TGF-β1–treated PAI-1−/− ATII cells (Figure 4).
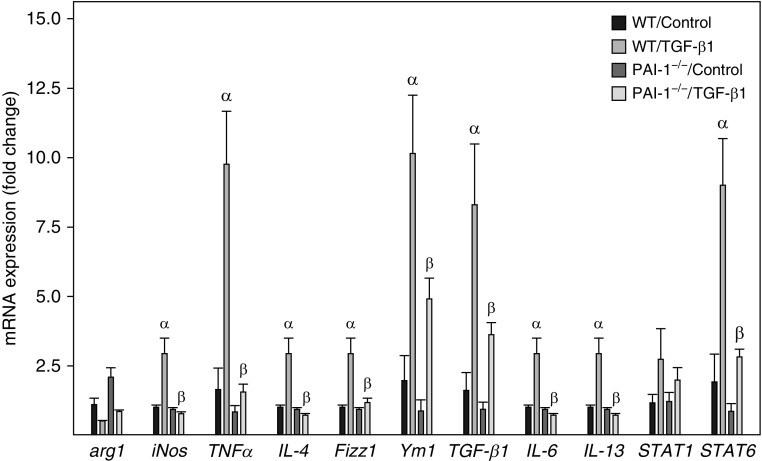
Deletion of PAI-1 in ATII cells blunted the stimulatory effect of the CM from senescent ATII cells on alveolar macrophage gene expression. Alveolar macrophages isolated from lungs of WT mice were incubated with the CM from ATII cells isolated from WT or PAI-1–deficient (PAI-1−/−) mice and treated with saline or TGF-β1 for 24 hours. The amounts of mRNAs were assessed by quantitative real-time PCR as described in the Methods section, and the results are expressed as fold changes relative to the control. α=
significantly different from AMs incubated with the CM from saline-treated WT ATII cells; AM
=
alveolar macrophage; arg1
=
arginase 1; β
=
significantly different from AMs incubated with the CM from TGF-β1–treated WT ATII cells (P
<
0.05; n
=
3–4); Fizz1
=
found in inflammatory zone 1; iNos
=
inducible nitric oxide synthase; STAT
=
signal transducer and activator of transcription; Ym1
=
chitinase-like 3.
Inhibition of PAI-1 Activity Protected Rat Lung ATII Cells from TGF-β1–induced Senescence and Suppressed the Secretion of Profibrotic Mediators
Our previous studies showed that administration of TM5275, a small molecule PAI-1 inhibitor, ameliorated lung fibrosis induced by Ad-TGF-β1223/225, an adenovirus expressing constitutively active TGF-β1 (16). To determine whether treatment with TM5275 blocks TGF-β1–induced ATII cell senescence, rat lung ATII (L2) cells were treated with 2 ng/ml TGF-β1 in the presence or absence of TM5275. The results show that treatment of L2 cells with TGF-β1 significantly increased the number of SA-β-gal–positive cells (Figures 5A and 5B) but not the activity of caspase 3/7 (Figure 5C). Western blot analysis further showed that treatment with TGF-β1 increased the expression of PAI-1 and p16, which was associated with a reduction of Rb phosphorylation (Figures 5D–5G), but had no significant effect on the expression of cleaved caspase 3 or Bax, two apoptosis markers (Figures 5D, 5H, and 5I). Treatment with TM5275, however, almost completely blocked TGF-β1’s effects on SA-β-gal activity, p16, and Rb phosphorylation (Figures 5A–5F). There was no significant effect of TM5275 on the expression of cleaved caspase 3 or Bax, with or without TGF-β1 (Figures 5D, 5H, and 5I). Our data also show that TGF-β1 treatment stimulated the secretion of two senescence markers, IGFBP3 and IL-6, as well as IL-4, IL-13, and PDGF from L2 cells (Figures 5E and and6A).6A). Importantly, our data show that treatment with TM5275 almost completely blocked TGF-β1–induced secretion of senescent markers as well as IL-4, IL-13, and PDGF (Figures 5E and 6 A). These data further suggest that PAI-1 mediates TGF-β1–induced ATII cell senescence as well as secretion of profibrogenic mediators, probably through inducing p16. Our data also suggest that TM5275, with or without TGF-β1, does not induce L2 cell apoptosis under the treatment conditions.
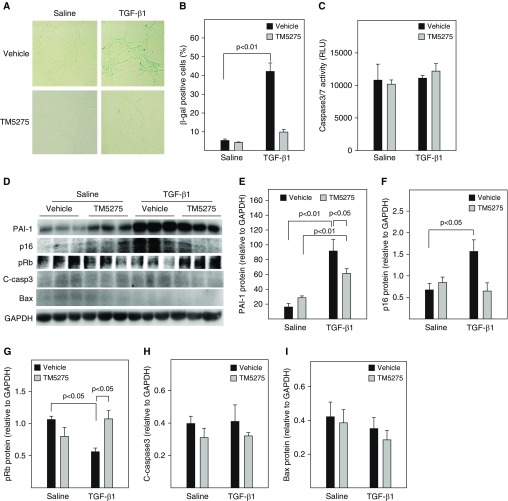
Inhibition of PAI-1 activity protected rat alveolar epithelial (L2) cells from TGF-β1–induced senescence. L2 cells were treated with 2 ng/ml of TGF-β1 in serum-free medium containing vehicle or 25 μM TM5275 for 48 hours and then cultured in TGF-β1–free and TM5275-free medium for an additional 24 hours. (A and B) SA-β-gal activity was revealed by X-gal staining. (C) Caspase 3/7 activity in the CM measured with a kit from Promega. (D–I) Western blot analyses of the proteins of interest in L2 cells. (D) Representative Western blots. (E–I) Quantification of the band intensities, normalized by GAPDH (n=
3–4).
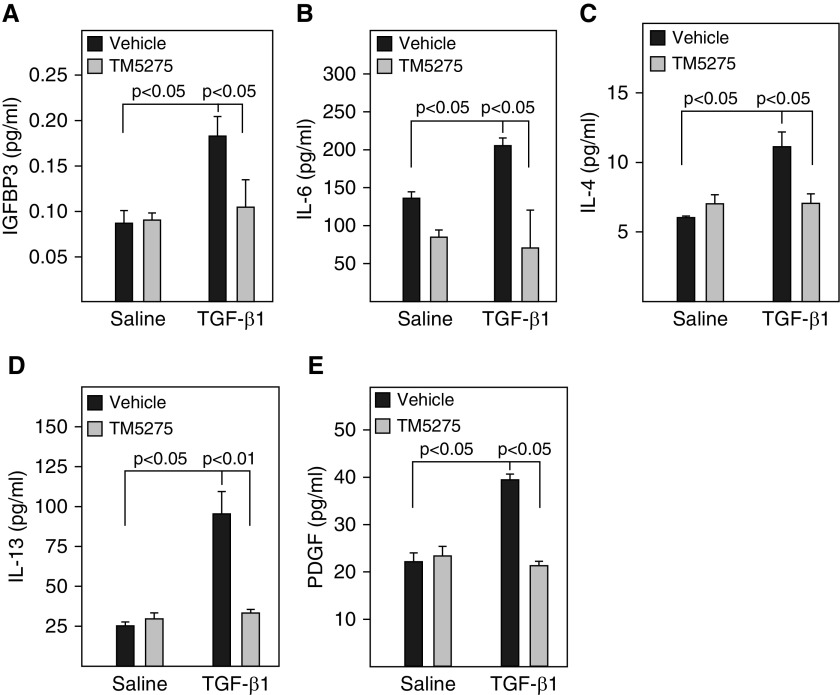
Inhibition of PAI-1 activity blocked TGF-β1–induced secretion of profibrotic mediators from L2 cells. L2 cells were treated with TGF-β1 in the presence or absence of TM5275 as described in Figure 5. (A–E) The amount of senescence-associated secretory phenotype (SASP) components in the CM were assessed by ELISA (n=
3–4).
Inhibition of PAI-1 Activity Attenuated the Stimulatory Effect of L2 Cell SASP on Macrophage Gene Expression
To determine whether TM5275 altered L2 cell SASP and therefore the responses of macrophages, rat macrophage NR8383 cells were cultured with the CM from L2 cells that had been treated with TGF-β1 in the presence or absence of TM5275. The results show that incubation of NR8383 cells with the CM from L2 cells treated with TGF-β1 alone increased the expression of arginase 1, Fizz1, and Ym1, three M2 macrophage markers; iNOS and TNF-α, two M1 macrophage markers; and TGF-β1. However, it had no significant effect on the expression of STAT1 or STAT6 (Figure 7). The CM from L2 cells that had been treated with TGF-β1 in the presence of TM5275, however, had significantly reduced stimulatory effects on NR8383 cells (Figure 7). These data further suggest that PAI-1 modulates SASP-stimulated macrophage responses.
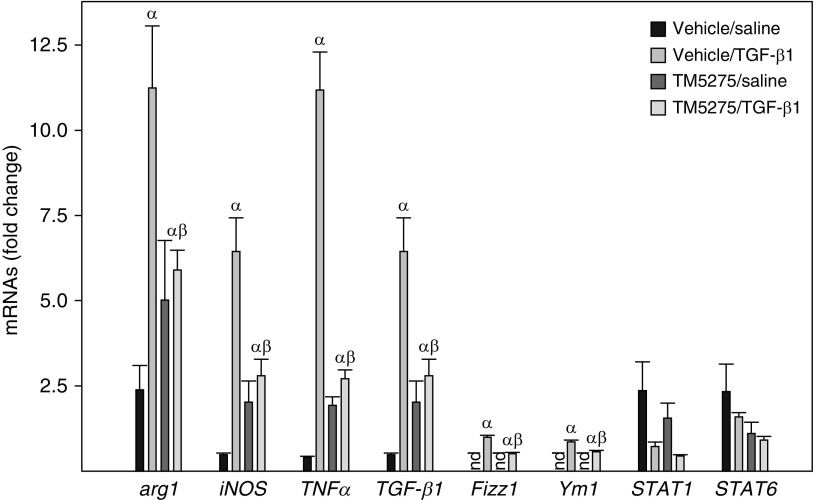
Inhibition of PAI-1 activity attenuated the stimulatory effect of L2 cell SASP on macrophage gene expression. Rat macrophage NR8383 cells were incubated with the CM from L2 cells treated with saline or TGF-β1 in the presence or absence of TM5275 as described in Figure 5 for 24 hours. The expression levels of mRNAs were assessed by quantitative real-time PCR as described in the Methods section, and the results were expressed as fold changes relative to NR8383 cells incubated with the CM from saline- and solvent-treated L2 cells (n=
3–4). P
<
0.05. α
=
significantly different from NR8383 cells incubated with the CM from saline- and solvent-treated L2 cells; β
=
significantly different from NR8383 cells incubated with the CM from TGF-β1– plus solvent-treated L2 cells; nd
=
not detected.
Silencing p16 Attenuated PAI-1 Protein–induced ATII Cell Senescence and Secretion of Profibrotic Mediators
To explore the potential mechanism whereby PAI-1 mediates TGF-β1–induced ATII cell senescence, p16 expression was silenced in these cells with p16 siRNA. The results show that, on the one hand, treatment of primary ATII cells with active PAI-1 protein significantly increased the number of SA-β-gal–positive cells as well as p16 expression. Silencing p16, on the other hand, completely blocked PAI-1 protein–induced SA-β-gal activity and restored Rb phosphorylation, although it had no significant effect on PAI-1 protein expression (Figures 8A–8F). Silencing p16 also blocked PAI-1 protein–induced secretion of IL-4, IL-6, IL-13, and PDGF (Figures 8G–8J). These data suggest that PAI-1 mediates TGF-β1–induced ATII cell senescence and secretion of profibrotic mediators through inducing p16.
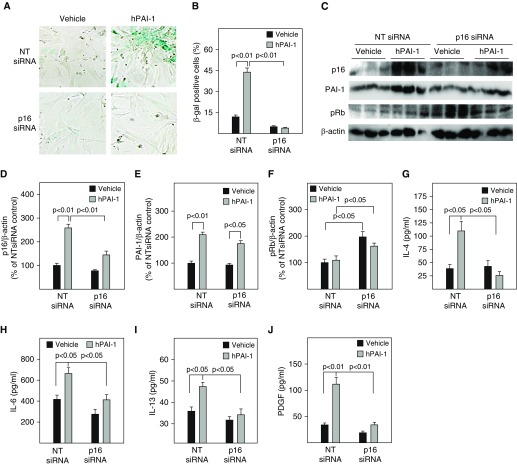
Silencing p16 attenuated PAI-1 protein–induced ATII cell senescence and secretion of profibrotic mediators. Freshly isolated ATII cells were transfected with nontarget (NT) or p16 siRNA and then treated with 1 μg/ml of active PAI-1 protein as described in the Methods section. (A and B) SA-β-gal activity was revealed by X-gal staining. (C–F) Western analyses of p16, PAI-1, and pRb. (F–J) The amounts of cytokines, chemokines, and growth factors in the CM were assessed by ELISA (n=
3–6).
Discussion
IPF is an aging-related progressive fatal lung disorder with no known etiology and very limited therapeutic options. One of the pathological features of IPF lung is ATII cell senescence (4–9). Although increasing evidence indicates important roles of ATII cell senescence in lung fibrogenesis (10–12, 18), how ATII senescence is regulated in fibrotic lung and how senescent ATII cells contribute to lung fibrogenesis remain poorly understood. In this study, we showed that TGF-β1, a most ubiquitous and potent profibrogenic cytokine, induced ATII cell senescence and that senescent ATII cells secreted an array of profibrotic and proinflammatory mediators, which activated AMs. We also found that deletion ATII PAI-1 or inhibition of PAI-1 activity almost completely blocked TGF-β1–induced ATII cell senescence, the secretion of profibrotic mediators, and the stimulatory effects of ATII SASP on macrophages. Because PAI-1 expression is increased in ATII cells in IPF (10, 18), our results suggest that increased PAI-1 may underlie ATII cell senescence in IPF lung and that senescent ATII cells promote lung fibrosis in part by activating macrophages through secreting profibrotic mediators.
Previous studies from our laboratory and others have shown that PAI-1 is not only a marker but also a key mediator of cell senescence (18–24). Although the mechanisms underlying PAI-1 promotion of cell senescence remain elusive, several hypotheses have been proposed, including inhibition of proteolytic activity and thus degradation of a senescence mediator, IGFBP3, and suppression of cyclin D1 and thus Rb phosphorylation (20–22, 24, 34). In a recent study, on the one hand, we showed that PAI-1 expression was increased in ATII cells in IPF lung and in a bleomycin-induced lung fibrosis model; ablation of PAI-1 specifically in ATII cells in PAI-1 conditional knockout mice, on the other hand, attenuated bleomycin-induced ATII cell senescence and lung fibrosis in mice (18). Mechanistically, we found that ablation of ATII PAI-1 attenuated bleomycin-induced ATII cell senescence through downregulation of p53, a master regulator of cell proliferation and death, because PAI-1 induced p53 in ATII cells; deletion or silencing PAI-1 reduced bleomycin-stimulated increase in p53 protein, whereas silencing p53 abolished PAI-1 protein–induced L2 cell senescence (18). In this study, we further showed that TGF-β1 induced ATII cell senescence, which was associated with induction of p16 but not p53. Importantly, we found that deletion of PAI-1 or inhibition of PAI-1 activity almost completely abolished TGF-β1–induced p16 expression and ATII cell senescence, whereas silencing p16 abrogated PAI-1 protein–induced ATII cell senescence. Our data suggest that PAI-1 mediated TGF-β1–induced ATII cell senescence by inducing p16, although the molecular mechanism underlying PAI-1 induction of p16 is currently unknown. Because PAI-1, p53, and p16 are all upregulated in ATII cells in IPF lung (10, 18, 35), our results suggest that PAI-1 plays a pivotal role in ATII cell senescence in fibrotic lung through activating different cell cycle repression pathways, including p53 and p16, upon stimulation by different stimuli.
Despite the recognition of the important role of ATII cell senescence in IPF pathogenesis, how ATII cell senescence is linked to lung fibrosis is not well understood. Besides loss of normal function and reparative capacity, senescent cells secrete an array of bioactive molecules, including profibrotic and proinflammatory cytokines, chemokines, and proteases, collectively called the “senescence-associated secretory phenotype.” These bioactive molecules can modulate the function of secreting cells as well as adjacent cells. Nonetheless, whether senescent ATII cells secrete profibrotic mediators, whether ATII PAI-1 modulates the secretion profile, and whether ATII SASP promotes a profibrotic phenotype in AMs are unknown. In this study, we showed that senescent ATII cells secreted various proinflammatory and profibrotic mediators, including IL-4 and IL-13, two Th2 cell cytokines involved in M2 activation of macrophages. We also showed that ATII PAI-1 promoted the secretion of profibrotic mediators as deletion of PAI-1 or inhibition of PAI-1 activity abolished TGF-β1-induced secretion of IL-4, IL-13, and PDGF in ATII and L2 cells. These data suggest that senescent ATII cells may facilitate lung fibrosis by secreting profibrotic mediators. PAI-1, on the other hand, promotes lung fibrosis at least in part by inducing ATII cell senescence and the secretion of profibrotic mediators.
ATII cells secrete both profibrotic and senescent mediators upon TGF-β1 stimulation. Although we did not do a systematic study to dissect the time courses of the secretions of senescent versus profibrotic mediators, our preliminary studies showed that the secretions of IL-6 and IGFBP3, two senescence markers, and IL-13, a profibrotic cytokine, were not increased until 48 hours of TGF-β1 treatment (Figure E2). Moreover, we found that the activity of caspase 3/7, an apoptosis marker, was increased after 72 hours of TGF-β1 treatment (Figure E2). These data suggest that the secretion of senescence and profibrotic mediators follows a similar time course and that there is a small window between cell senescence and apoptosis. It has been well documented that ATII cells will be transformed into type I cells when they are cultured for more than 5 to 6 days. This restricted our time-course study to a maximum of 3 days because cell isolation and experiment setup take 2 days. It should be stressed that the time courses may be different with different stimuli. Nonetheless, more studies are needed to address these issues, which are beyond the scope of the present study.
AMs are the first line of defense in the airways and play a critical role in lung injury and fibrosis. AMs are activated by diverse stimuli, including infectious agents and air pollutants. Whether ATII SASP modulates macrophage activation has not been reported. In this study, we show for the first time that SASP from TGF-β1–induced senescent ATII cells stimulated the expression of many genes in AMs, including M1 macrophage markers iNOS, TNF-α, and IL-6 as well as M2 macrophage markers Fizz1, Ym1, STAT6, arginase 1, and TGF-β1. Deletion of PAI-1 in ATII cells or inhibition of PAI-1 activity, however, attenuated the stimulatory effects of ATII SASP on macrophage gene expression. Together, the data suggest that ATII PAI-1 modulates SASP secretion and thus ATII SASP-mediated activation of AMs. It should be stressed that, although M1 macrophages are believed to be involved mainly in the inflammatory response, whereas M2 macrophages play an important role in tissue remodeling and fibrogenesis (29, 33, 36–39), numerous studies have shown that macrophages in fibrotic lungs express both proinflammatory and profibrotic phenotypes and that M1 macrophages are also important in pulmonary fibrogenesis (33, 40–42). Importantly, the gene expression profile of AMs that we describe resembles that demonstrated by macrophages in lungs of mice with experimental fibrosis (33). In summary, our data suggest that senescent ATII cells promote lung fibrosis in part through secreting bioactive mediators, which, in turn, activate AMs, and this process is regulated by ATII PAI-1.
Aging is a major risk factor for many chronic diseases, including IPF, and increased senescent cell burden is believed to contribute importantly to the aging process and the pathogenesis of aging-related diseases. A new class of drugs called “senolytics” has been used recently in preclinical and clinical studies to specifically remove senescent cells for the treatment of aging-related diseases (10–13, 43, 44). Several studies have shown that targeting senescent ATII cells may be an attractive therapeutic option for the treatment of IPF, although this is premature (10–13). PAI-1 expression increases with age, and in many aging-related diseases, including IPF (17, 18, 45–49), PAI-1 plays a critical role in lung fibrogenesis (14, 15–18). Most important, PAI-1 is not only a validated marker but also a critical mediator of cell senescence (18–24). Therefore, targeting PAI-1 may be an effective therapeutic option for the treatment of IPF as well as other aging-related diseases. Our previous studies have shown that inhibition of PAI-1 activity with a small molecule PAI-1 inhibitor, TM5275, attenuated TGF-β1–induced lung fibrosis in mice (16) and reduced bleomycin-induced ATII cell senescence (18). In this study, we further show that inhibition of PAI-1 activity with TM5275 blocked TGF-β1–induced ATII cell senescence, the secretion of SASP, and ATII SASP-induced macrophage activation. These data support the notion that small molecule PAI-1 inhibitors may have therapeutic potential for the treatment of IPF. More studies are needed to further explore the therapeutic potential of these small molecule PAI-1 inhibitors for the treatment of aging-related diseases, including IPF.
In summary, we showed in this study that TGF-β1–induced senescent ATII cells secrete various profibrotic mediators through a PAI-1-p16–dependent mechanism. ATII SASP further induces a profibrotic phenotype in AMs. These results suggest that PAI-1 promotes lung fibrosis at least in part by inducing ATII cell senescence and thus secretion of profibrotic mediators as well as, consequently, activation of AMs.
Footnotes
Supported by National Institutes of Health grants HL088141; and R56HL131054 (R.-M.L.) and HL114470 (V.J.T.).
Author Contributions: T.R. and R.-M.L. designed the study. T.R., C.J., and R.-M.L. performed the experiments. T.R., C.J., G.L., and R.-M.L. analyzed the data. G.L., T.M., V.A., and V.J.T. edited the manuscript and contributed intellectually to the study. T.R. and R.M.L. wrote the manuscript.
This article has a related editorial.
This article has a data supplement, which is accessible from this issue’s table of contents at www.atsjournals.org.
Originally Published in Press as DOI: 10.1165/rcmb.2019-0071OC on September 12, 2019
Author disclosures are available with the text of this article at www.atsjournals.org.
References
Articles from American Journal of Respiratory Cell and Molecular Biology are provided here courtesy of American Thoracic Society
Full text links
Read article at publisher's site: https://doi.org/10.1165/rcmb.2019-0071oc
Read article for free, from open access legal sources, via Unpaywall:
https://www.ncbi.nlm.nih.gov/pmc/articles/PMC7055702
Citations & impact
Impact metrics
Citations of article over time
Alternative metrics
Article citations
A signaling pathway map of plasminogen activator inhibitor-1 (PAI-1/SERPINE-1): a review of an innovative frontier in molecular aging and cellular senescence.
Cell Commun Signal, 22(1):544, 14 Nov 2024
Cited by: 0 articles | PMID: 39543686 | PMCID: PMC11566301
Review Free full text in Europe PMC
Aging of alveolar type 2 cells induced by <i>Lonp1</i> deficiency exacerbates pulmonary fibrosis.
Biomol Biomed, 24(5):1258-1272, 06 Sep 2024
Cited by: 0 articles | PMID: 38625722 | PMCID: PMC11378998
Cellular senescence and SASP in tumor progression and therapeutic opportunities.
Mol Cancer, 23(1):181, 31 Aug 2024
Cited by: 1 article | PMID: 39217404 | PMCID: PMC11365203
Review Free full text in Europe PMC
The Regulation of Fatty Acid Synthase by Exosomal miR-143-5p and miR-342-5p in Idiopathic Pulmonary Fibrosis.
Am J Respir Cell Mol Biol, 70(4):259-282, 01 Apr 2024
Cited by: 3 articles | PMID: 38117249
NR2F2 alleviates pulmonary fibrosis by inhibition of epithelial cell senescence.
Respir Res, 25(1):154, 02 Apr 2024
Cited by: 3 articles | PMID: 38566093 | PMCID: PMC10985909
Go to all (63) article citations
Data
Data behind the article
This data has been text mined from the article, or deposited into data resources.
BioStudies: supplemental material and supporting data
Lay summaries
Plain language description
Similar Articles
To arrive at the top five similar articles we use a word-weighted algorithm to compare words from the Title and Abstract of each citation.
Serpine 1 induces alveolar type II cell senescence through activating p53-p21-Rb pathway in fibrotic lung disease.
Aging Cell, 16(5):1114-1124, 19 Jul 2017
Cited by: 100 articles | PMID: 28722352 | PMCID: PMC5595683
PAI-1 Regulation of p53 Expression and Senescence in Type II Alveolar Epithelial Cells.
Cells, 12(15):2008, 05 Aug 2023
Cited by: 2 articles | PMID: 37566086 | PMCID: PMC10417428
Senescence of alveolar epithelial cells impacts initiation and chronic phases of murine fibrosing interstitial lung disease.
Front Immunol, 13:935114, 18 Aug 2022
Cited by: 13 articles | PMID: 36059455 | PMCID: PMC9434111
Angiotensin-TGF-beta 1 crosstalk in human idiopathic pulmonary fibrosis: autocrine mechanisms in myofibroblasts and macrophages.
Curr Pharm Des, 13(12):1247-1256, 01 Jan 2007
Cited by: 94 articles | PMID: 17504233
Review
Funding
Funders who supported this work.
NHLBI NIH HHS (4)
Grant ID: P01 HL114470
Grant ID: R01 HL088141
Grant ID: R56 HL131054
Grant ID: R35 HL135830
NIEHS NIH HHS (2)
Grant ID: R01 ES029981
Grant ID: P42 ES027723