Abstract
Objectives
To improve our understanding of the global epidemiology of common respiratory viruses by analysing their contemporaneous incidence at multiple sites.Methods
2010-2015 incidence data for influenza A (IAV), influenza B (IBV), respiratory syncytial (RSV) and parainfluenza (PIV) virus infections were collected from 18 sites (14 countries), consisting of local (n = 6), regional (n = 9) and national (n = 3) laboratories using molecular diagnostic methods. Each site submitted monthly virus incidence data, together with details of their patient populations tested and diagnostic assays used.Results
For the Northern Hemisphere temperate countries, the IAV, IBV and RSV incidence peaks were 2-6 months out of phase with those in the Southern Hemisphere, with IAV having a sharp out-of-phase difference at 6 months, whereas IBV and RSV showed more variable out-of-phase differences of 2-6 months. The tropical sites Singapore and Kuala Lumpur showed fluctuating incidence of these viruses throughout the year, whereas subtropical sites such as Hong Kong, Brisbane and Sydney showed distinctive biannual peaks for IAV but not for RSV and PIV.Conclusions
There was a notable pattern of synchrony of IAV, IBV and RSV incidence peaks globally, and within countries with multiple sampling sites (Canada, UK, Australia), despite significant distances between these sites.Free full text

Comparative global epidemiology of influenza, respiratory syncytial and parainfluenza viruses, 2010–2015
Abstract
Objectives
To improve our understanding of the global epidemiology of common respiratory viruses by analysing their contemporaneous incidence at multiple sites.
Methods
2010–2015 incidence data for influenza A (IAV), influenza B (IBV), respiratory syncytial (RSV) and parainfluenza (PIV) virus infections were collected from 18 sites (14 countries), consisting of local (n = 6), regional (n = 9) and national (n = 3) laboratories using molecular diagnostic methods. Each site submitted monthly virus incidence data, together with details of their patient populations tested and diagnostic assays used.
Results
For the Northern Hemisphere temperate countries, the IAV, IBV and RSV incidence peaks were 2–6 months out of phase with those in the Southern Hemisphere, with IAV having a sharp out-of-phase difference at 6 months, whereas IBV and RSV showed more variable out-of-phase differences of 2–6 months. The tropical sites Singapore and Kuala Lumpur showed fluctuating incidence of these viruses throughout the year, whereas subtropical sites such as Hong Kong, Brisbane and Sydney showed distinctive biannual peaks for IAV but not for RSV and PIV.
Conclusions
There was a notable pattern of synchrony of IAV, IBV and RSV incidence peaks globally, and within countries with multiple sampling sites (Canada, UK, Australia), despite significant distances between these sites.
Introduction
Respiratory infections cause significant morbidity and mortality in both children and adults.1, 2, 3, 4, 5 The healthcare impact from community wide outbreaks with complications in risk groups add up to the globally recognized high burden of disease, which has formed the basis for the global influenza surveillance and prevention program.6, 7 Therefore, there is a need to improve our understanding of the epidemiology and trends of common respiratory virus infections to inform the timing and resourcing of various interventions, as well as to prioritise research and development funding for enhancing such measures.
A global network of collaborators with shared clinical, public health, and epidemiological research interests in respiratory viruses was recently established – the International Network for the Sequencing of Respiratory Viruses (INSPIRE).8 This long-term INSPIRE project aims to share viral incidence and other related data, obtained through routine diagnostic testing, in a cross-sectional, contemporaneous manner to reveal and compare real-life (i.e. outside of prospective, planned studies) global trends in viral epidemiology and feed into potential healthcare burden estimates.
Despite multiple single-site or national surveillance data for influenza and/or RSV being available either via publication or online, e.g. from Argentina,9 Brazil,10 Canada,11 UK,12 The Netherlands,13 Finland,14 Bulgaria,15 Russia,16 South Africa,17 Rwanda,18 Madagascar,19 Cambodia,20 Thailand,21 Malaysia,22 Singapore,23 The Philippines,24 Indonesia,25 Hong Kong,26 Mongolia,27 Japan,28 Australia,29 and New Zealand,30 there are still relatively few global-scale studies that compare the contemporaneous (i.e. over the same time period) incidence patterns of common respiratory viruses across different countries – and nearly all of these used mostly publicly available surveillance data extracted from national surveillance websites, or published studies.31, 32, 33, 34, 35
However, it is clear from the literature that international collaborations are increasing, in an effort to pool and compare such contemporaneous epidemiological data, to improve our understanding of how these respiratory viruses are behaving – not just within our local or national populations, but simultaneously across the world. Although national surveillance data are invaluable to reveal how these viruses are behaving and moving through populations, and also capture the incidence of viral infections in the community to some extent, the bulk of that population's healthcare burden may be more accurately represented by the routine diagnostic testing data obtained from patients who were sufficiently ill to be admitted to hospital.
To explore this further, we report on the compilation and analysis of mostly routinely collected, diagnostic data for influenza A (IAV), influenza B (IBV), respiratory syncytial (RSV) and parainfluenza (PIV) virus, to provide a contemporaneous, cross-sectional comparison of the epidemiology and seasonal trends of these common respiratory viruses from 18 sites (14 countries).
Methods
INSPIRE (International Network for the Sequencing of Respiratory Viruses) network
The INSPIRE network was primarily achieved through personal contacts with known collaborators with a common interest in respiratory viruses, who then disseminated invitations to other collaborators. This was done mostly by email, but also face-to-face at conferences where potential collaborators were attending, and is currently facilitated by the network website (www.global-inspire.org).
Network construction was partially aided by most collaborators who already had an interest in influenza, particularly laboratories in existing influenza surveillance networks, with the U.S. Centers for Disease Control and Prevention (CDC) and/or the World Health Organization (WHO).
Epidemiological data
Each INSPIRE network site submitted monthly incidence numbers (i.e. laboratory-confirmed positives and the number of samples tested) for IAV, IBV, RSV and PIV types 1–4 viruses, for the period 2010–2015 (6 years, inclusive). In addition, each site provided information on their patient population tested with their diagnostic assays used, as well as any relevant clinical testing protocols (Table 1 ).
Table 1
Contributing collaborating sites, with details of their routine diagnostic assays and patient populations.
Geographical site | Diagnostic assay used | Respiratory virus targets (denominator population) | Years covered by data (only 2011–2015 data sets presented) | Catchment population (estimate) | Patient age groups (i.e. adult or children, or both) | Testing criteria (i.e. only when symptomatic +/− surveillance) | Patient origin (i.e. inpatient or outpatient, or both) |
---|---|---|---|---|---|---|---|
North America | |||||||
Vancouver, Canada (regional) | In-house, Luminex xTAG | IAV, IBV, RSV, PIV1-4, HRV/EV, HAdV, HCoV, HMPV, and HBoV (n = 45,598) | 2011–01 to 2015–12; RSV, PIV | Vancouver and surrounding BC ~ 4.6 million | Mostly adults | Yes | Mainly in-patients, outbreaks and occasional outpatients |
Edmonton, Canada (regional) | In-house, Luminex xTAG | IAV, IBV, RSV, PIV1-4, HRV/EV, HAdV, HCoV, HMPV, and HBoV (n = 117,206) | 2010–01 to 2015–12 | Edmonton and Northern Alberta ~ 2 million | Adults and children | Yes | Mostly inpatients |
Halifax, Canada (regional) | Seeplex RV15 | IAV, IBV, RSV, PIV1-4, HRV/EV, HAdV, HCoV, HMPV, and HBoV (n = 12,133) | 2010–01 to 2015–12 | Halifax, Nova Scotia ~ 930,000 | Mainly adults but some children | Yes | Mainly in patient and outbreak related specimens. Very few out patients were tested |
Europe | |||||||
Leicester, UK (regional) | In-house (+PHE assay) | IAV, IBV, RSV, PIV 1–4 (n = 13,673) | 2010–01 to 2015–09 | Leicester and surroundings, UK ~ 330,000 | Adults and children | Yes | Mostly inpatients |
Cambridge, UK (regional) | In-house validated single-/multiplex PCR assays | IAV, IBV, RSV, PIV 1–4 (n = 36,228) | 2010–01 to 2015–12 | Cambridge and surroundings ~ 200,000 | Adults and children | Yes | Mostly inpatients |
Turku, Finland (regional) | Seeplex RV12 (2010–2012; Anyplex RV16 (2013–2015) | IAV, IBV, RSV A and B, PIV1-3, PIV4 (2013–2015), RV, HCoV (OC43, 229E, NL63), HMPV, HAdV, HBoV (2013–2015), EV (2013–2015) | 2010–01 to 2015–12 | Turku and Southwest Finland ~ 450,000 | Adults and children | Yes | Mainly in-patients – few outpatients |
Rotterdam, The Netherlands (local) | In-house validated single-/multiplex PCR assays | IAV, IBV, RSV, PIV1-4, HMPV, HCoV (excl. HKU1), HRV, HBoV HAdV (n = 8424) | 2010–01 to 2015–10 | Rotterdam, Netherlands ~ 600,000 | Adults and children | Mostly, though occasionally for outbreaks also | Mixture of in- and out-patients |
Africa | |||||||
Rwanda (national) | Fast Track FTD21 | IAV, IBV, RSV, PIV1-4, HAdV, HMPV, HRV, HCoV, HBoV, EV, HPEV (n = 1397) | 2012–02 to 2013–09 | Rwanda ~ 11 million | Adults and children | Yes | Mainly in-patients – any outpatients – pending |
Madagascar (national) | Fast Track FTD21 | IAV, IBV, RSV, PIV1-4, HAdV, HMPV, HRV, HCoV, HBoV, EV, HPEV (n = 6483) | 2011–01 to 2015–12 | Madagascar (mostly Antananarivo ~ 700,000) | Adults and children | Yes | Mainly outpatients |
Middle East | |||||||
Lebanon (local) | PCR and DFA | IAV, IBV, RSV (n = 473) | 2013–07 to 2015–12 | Beirut ~ 1.4 million | Mostly children, some adults | Yes | Mainly outpatients |
East/ Southeast Asia | |||||||
Mongolia (national) | WHO influenza and Fast Track FTD21 | IAV, IBV, RSV, PIV1-4, HAdV, HMPV, HRV, HCoV, HBoV, EV, HPEV (n = 20,420) | 2010–11 to 2015–12, without 2015–02 | Mongolia ~ 3 million | Adults and children | Yes | Combined in-patients/out-patients |
Singapore (local) | In-house, Luminex NxTAG | IAV, IBV, RSV, PIV1-4, HAdV, HMPV, HRV/EV, HCoV, HBoV (n = 1787) | 2012–04 to 2015–12; without 2012–10 | Singapore (National University Hospital) ~ 1.5 million | Adults and children | Yes | Mainly in-patients |
Kuala Lumpur, Malaysia (local) | Luminex xTAG | IAV, IBV, RSV, PIV1-4, HRV/EV, HAdV, HCoV, HMPV, and HBoV (n = 3935) | 2012–02 to 2014–05 | Kuala Lumpur ~ 7 million | Mainly adults, some children | Yes | Outpatients |
Hong Kong, China (local) | IFA | IAV, IBV, RSV, PIV1-3, HAdV (n = 69,161) | 2010–01 to 2015–12 | Shatin district ~ 0.6 million | Adults and children | Yes | Mainly in-patients |
Sendai, Japan (local) | viral culture + IFA | IAV, IBV, RSV, PIV1-3, HAdV (n = 7896) | 2011–01 to 2015–10 | Sendai ~ 1.3 million | Mostly children, but mixed with adults | Yes | Mostly out-patients, but mixed with in-patients |
Australasia | |||||||
Brisbane, Australia (regional) | In-house validated single-/multiplex PCR assays | IAV, IBV, RSV, PIV1-3, HAdV, HMPV (n = 160,601) | 2011–01 to 2015–12 | Queensland ~ 4.7 million | Adults and children | Yes | Mainly in-patients, outpatients – some ED also |
Sydney, Australia (regional) | In-house validated single-/multiplex PCR assays | IAV, IBV, RSV, HRV, EV, PIV1-3, HAdV, HMPV (n = 482,877) | 2010–01 to 2015–12 | New South Wales ~ 7.7 million | Adults and children | Yes | Combined in-patients/out-patients |
Christchurch, New Zealand (NZ) (regional) | Fast Track FTD 21 | IAV, IBV, RSV, PIV1-4, HAdV, HMPV, HRV, HCoV, HBoV, EV, HPEV (n = 22,871) | 2010–01 to 2015–12 | Christchurch (1 acute admitting hospital) ~ 49,500 | Adults and children | Diagnostic or surveillance, all symptomatic. | Mainly in-patients – also outpatients and community surveillance activity |
Abbreviations: PCR – polymerase chain reaction; IFA – immunofluorescence (antigen) assay; NRL – National Reference Laboratory; NUH – National University Hospital (Singapore); PWH/CUHK/NT – Prince of Wales Hospital/The Chinese University of Hong Kong/ New Territories; IAV – influenza A virus; IBV – influenza B virus; RSV – respiratory syncytial virus; PIV1-4 – parainfluenza viruses types 1–4; HAdV – human adenovirus; HMPV – human metapneumovirus; HRV – human rhinovirus; HCoV – human coronavirus; HBoV – human bocavirus; EV – enterovirus; HPEV – human parechovirus; HRV/EV – indicates that the assay cannot distinguish between human rhinovirus and enterovirus.
The incidence data were plotted as a heat map to aid the visualisation of any seasonal trends for each virus, across all sites, during 2010–2015. By combining and stratifying plots of the data in various ways, e.g. by individual viruses, Northern vs. Southern Hemisphere, temperate vs. tropical regions, various trends in this global data were explored.
Periodicity and peak analysis
The seasonality of IAV, IBV, RSV and mainly PIV3 (due to low incidence and/or limited capability to screen for other PIV types) was examined for the periodicity and annual timing of their incidence peaks. A continuous wavelet transform was applied to the incidence time series, as has been previously used for various infectious disease studies.10, 36 This wavelet method decomposes the underlying time series process to the wavelet form that is a function of frequency.
The wavelet transform was conducted using package WaveletComp in R program,37 and the Morlet wavelet was adopted as previously described.38 To account for the truncated effect on the beginning and end of the time series, we shaded the area in the plot when such effect occurs. Observations are log-transformed and normalized (by subtracting the mean then dividing by the standard error for each log-transformed time series) to reduce skewing.
Since the onset or transmission of an infectious disease is temporally auto-correlated, the analysis of available incidence data may lead to a high auto-correlative power that may be inferred instead of the actual periodicity.
We assessed the significance by comparing the wavelet power of the disease incidence time series with the mean power spectrum of the random series simulated from the autoregression AR(1) process which shares the same autocorrelation.
We first calculated the wavelet power for an observed time series (e.g. X), then we simulated random series from the AR(1) process that share the same auto-correlation with X. The power of X is then compared with the power of these random series (the power of these random series form the “mean power spectrum”) to assess the significance by using the pre-defined function in WaveletComp in R.
Here, we define a significant biannual seasonality if a significantly high power signal occurs every six months, or when any two frequencies adding up to 12 months shows a significantly high power signal. Annual seasonality is therefore defined if there is a significantly higher power signal only every 12 months. The results are given in Figure S1.
For those localities showing significant biannual and annual seasonality, the timing of their disease peaks was investigated by the gravity method. Briefly, the centre of gravity (i.e. mean month) of the monthly distribution of incidence was computed by the circular statistics in the R package “circular”. Finally, 1000 bootstrap samples were conducted to obtain the 95% confidence interval (CI) of the centre of gravity.10, 39
Results
Viral incidence data
Eighteen sites representing 14 countries provided epidemiological data for influenza, RSV and parainfluenza viruses. Sites were a mixture of local (n = 6), regional (n = 9) or national centres (n = 3) with advanced (molecular) diagnostic capacity. Many of these collaborating laboratories were already routinely testing for respiratory viruses as part of their diagnostic service at local hospital or national reference laboratory level (Table 1). Each site provided monthly incidence data (expressed as a percentage, i.e. the number of positives divided by the total number of patients tested) for IAV, IBV, RSV, and PIV covering 2010–2015 (Fig. 1 ).
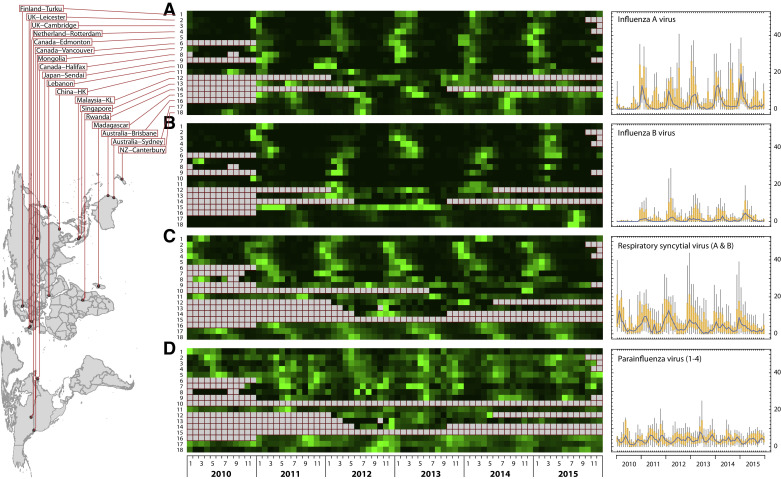
The incidence of the four respiratory viruses in the eighteen surveillance sites. Panels (A)–(D) show the monthly incidence of (A) influenza A virus, (B) influenza B virus, (C) respiratory syncytial virus, and (D) parainfluenza viruses from January 2010 to December 2015. The left-hand column displays the order of the geographical origin of the data for each of the four heat maps in the central column. The final right-hand column indicates the individual viruses for which the monthly incidence is displayed at each of these geographical sites. The maximum value of the colour scale is set to the highest incidence value (against which the other data for that site is normalised) at each surveillance site after excluding any outliers. The grey boxes indicate that no data was available from that site during that period. Some sites were only able to provide data for only some of these viruses for a limited period (usually within the period of a specifically funded study).
The average combined incidences of the four viruses (IAV, IBV, RSV and PIV) ranged from 13% to 39% across the 18 study sites, without significant difference (Wilcoxon signed rank test, p = 0.41) between the temperate and subtropical/tropical regions. IAV and RSV were the predominant viruses causing the infections, followed by PIV and IBV (Fig. 1 right column; and Fig. 2 , showing the relative incidences of the four viruses stratified at each location). It was also noted that PIV incidences were higher (p < 0.05) at the RSV-predominated sites than those at the IAV-predominated sites.
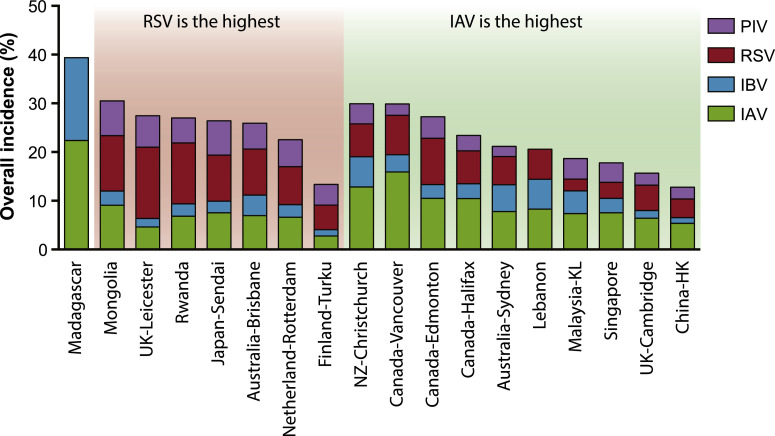
A comparison of the overall incidence of the four respiratory RNA viruses in the tested samples. IAV – influenza A virus, IBV – influenza B virus, RSV – respiratory syncytial virus, PIV parainfluenza virus. Site abbreviations: NZ – New Zealand, UK – United Kingdom, KL – Kuala Lumpur. The white/grey squares indicate whether the samples are mostly children (white) or adult (grey) patients, or both.
Distinctive peaks in the autumn/winter months were readily observed for IAV, IBV and RSV on the global plots. These were more pronounced in the individual Northern Hemisphere temperate sites than those in the Southern Hemisphere temperate sites, though there was less data available overall from the Southern Hemispheric locations (only three sites). The seasonality of the individual PIV subtypes (1–4) varied within each site's population, though the PIV3 often showed a more distinct seasonality than the other PIV subtypes at most Northern and Southern hemisphere sites. Tropical Singapore and subtropical Hong Kong showed a typically fluctuating incidence of all these viruses throughout the year, though the peaks in the Hong Kong data were slightly more distinctive for IAV.
Relatively few laboratories submitted influenza A/H1 and A/H3 subtyping data. When combined as influenza A, the Northern and Southern incidence peaks are approximately 6 months apart in January and July, respectively. Though less clear-cut, the influenza B peaks show a similar pattern.
Seasonality patterns
The heat map in Fig. 1 revealed that most temperate countries have annual patterns, whereas the sub-tropical and tropical regions had more year-round activity with some seasonal peaks, though these are not necessarily consistent, year-to-year. The most clearly defined seasonality can be seen for IAV and RSV, followed by IBV and PIV3. Wavelet and gravity analyses were used to detect the significant seasonality and estimate the peak time of annual and biannual seasonality, respectively (Figure S1, Fig. 3 ).
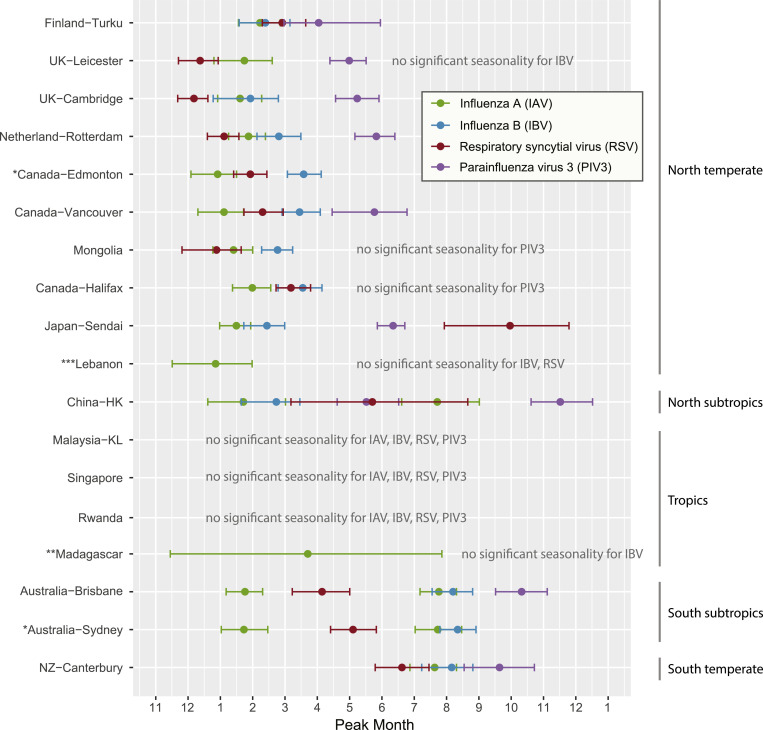
Month of peak incidence for the four RNA respiratory viruses. IAV – influenza A virus, IBV – influenza B virus, RSV – respiratory syncytial virus, PIV3 - parainfluenza type 3 virus. Peak incidence months showing significant annual and biannual seasonality (determined by wavelet analysis) were estimated via a circular statistics method. Study sites are ordered by their latitudes. *no PIV3-specific data. only IAV and IBV data available.
Most northern and southern temperate sites showed significant single annual seasonality for IAV (n = 11/11), followed by RSV (n = 10/11), IBV (n = 9/11) and PIV3 (n = 7/9) (Figure S1). Among most of these sites with seasonality, IAV, IBV and RSV peaked during December to March (northern temperate) and June to August (southern temperate), with the exception that the RSV in Sendai (Japan) peaked in September (CI: August to November), which was apparently later than the RSV peaking in other sites in the year (Fig. 3). PIV3 peaked during April to June (northern temperate) and September (southern temperate).
The four topical sites (Kuala Lumpur, Singapore, Rwanda and Madagascar) did not show significant annual and biannual seasonality for the four viruses, except that Madagascar showed an annual peak estimate in March with a wide confidence interval (November to July) (Fig. 3).
All three subtropical sites (Hong Kong, Brisbane and Sydney) showed significant seasonality, and particularly with biannual IAV peaks in January and July, respectively (Fig. 3). Notably, Hong Kong also showed biannual PIV3 peaks in May (CI: April to June) and November (CI: October to December). Other seasonality observations were of single annual cycles, including RSV's, that tended to peak between the biannual IAV peaks (i.e. April to May).
Synchrony of epidemics over geographic space
Next, we studied the level of synchrony of occurrence for the four viruses (defined as the degree to which the timing of the peak incidence of each of these viruses, coincide across the different populations and countries). This showed that in temperate regions IAV, IBV and RSV seasonal peaks were most synchronized, in which most of their peaks occurred in the same or next month of the others; whereas in the three subtropical cities (Hong Kong, Brisbane and Sydney), the RSV seasonal peaks were separated from the IAV peaks for two or more months (Fig. 3). It is also noted that IBV peaks mostly followed the IAV winter peaks in both the temperate and subtropical cities, and did not appear in the summer epidemic time in the subtropics.
Another aspect of synchrony can be seen in the data from three countries (Canada, UK and Australia) which had more than one intra-country sampling point populations, and from two countries (Singapore and Kuala Lumpur, Malaysia) which were sufficiently close, geographically, to compare virus seasonality. Plotting these data reveals that the incidence peaks of these respiratory viruses from each of the intra-country sampling sites for Canada, UK and Australia are virtually coincident, regardless of the inter-city distances between the individual cities (Fig. 4 ). However, it can be seen that the trends for Singapore and Kuala Lumpur, two sites with no obvious seasonality, were surprisingly quite different, despite their geographical proximity (less than 300 km apart). This finding will require further investigation in future studies.
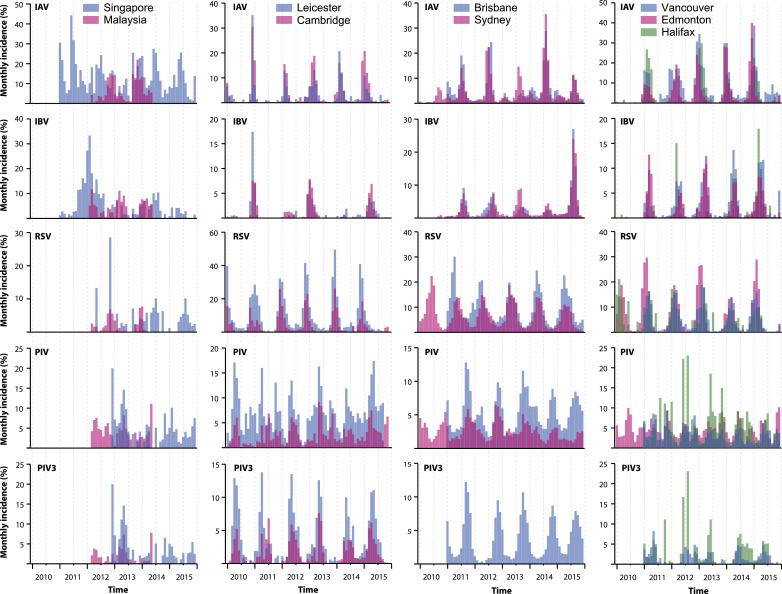
Patterns of viral incidence between geographically closely related cities within the same counties. Note that the incidence peaks for influenza A (IAV), influenza B (IBV), respiratory syncytial virus (RSV) and (where available) parainfluenza virus 3 (PIV3) are more or less coincident for Leicester-Cambridge, Brisbane-Sydney, and Vancouver-Edmonton-Halifax, despite the variable and large distances between these cities within the same country. The coincidence is less obvious when all subtypes of PIV are grouped and compared. In contrast, note that there is much less synchrony between the incidence peaks of these viruses in the Singaporean and Malaysian populations. * Sydney and Edmonton do not have PIV3 specific data.
Discussion
The establishment of the INSPIRE global network has allowed the pooling of basic incidence data for respiratory viruses from routine patient testing. This cross-sectional data from 18 sites globally from 2010–2015 has given some useful quantitative data on the contemporaneous pattern of the incidence.
Currently, although the full INSPIRE network does include multiple members in Africa and those with collaborators in South America,8 this current study does lack large data sets from these continents. This is mainly due to a lack of funding for routine screening for non-influenza respiratory viruses. Conversely, some countries (Canada, UK, Australia) may appear to be over-represented where there are multiple sampling sites within that country. However, two of these countries cover large geographical areas (Canada, Australia), and the availability of multiple intra-country sampling sites has revealed other aspects about the seasonality of these common respiratory viruses.
It is clear that our approach, using mostly routine diagnostic test data, has some disadvantages, as it is not based on systematic sentinel surveillance, unlike the routine influenza surveillance in many countries. However, our findings, based mostly on routinely collected diagnostic data for influenza and RSV, are similar to those reported by other global studies using mainly national surveillance data,31, 32, 33, 34 i.e. that there are annual seasonal peaks of influenza and RSV in the colder months in the Northern and Southern hemispheres, with more year-round activity in the subtropical/tropical regions. The influenza and RSV seasons generally last 3–4 months, with both viruses showing some year-to-year variation in the timing of their peak incidence (Fig. 1).
We do recognize that patterns of incidence are potentially impacted by the population and age groups targeted. Nevertheless, despite the variation in the clinical testing and laboratory assay protocols, the high level of comparability of incidence data from our INSPIRE sites to established, publicly available respiratory virus surveillance sites, such as WHO's FluNet,40 confirms the utility of this diagnostic data for revealing important epidemiological patterns in these common respiratory viruses (Figure S2).
From our epidemiologic data, several interesting findings have emerged. The first of these is a notable pattern of synchrony of the peaks of IAV, IBV and RSV across global sites with distinct and discernible patterns of seasonality. Whilst the recurring, predictable seasonality of IAV, IBV and RSV has been reported by single centre or country, or even several countries, this study has shown that this phenomenon appears to be occurring simultaneously in multiple countries sharing the same Northern or Southern hemisphere, which cannot be demonstrated with a series of individual, single-site studies, or multiple-site studies confined to the Northern or Southern hemisphere alone.
Secondly, it can be seen that more than one-third (7/18 of the sampling sites) showed RSV to have the highest incidence among the four viruses, demonstrating it as a major global health burden. This is well recognised in hospitalised paediatric patient populations,2 where it is the commonest cause of bronchiolitis. It has also been shown that RSV is also an important cause of hospitalization and death in some adults.41 This further supports the WHO's recent BraVe initiative in tackling some of the non-influenza respiratory viruses (NIRVs).7 Whilst we acknowledge that the nature and pattern of this data will be affected by the specific populations fitting the individual testing criteria at each contributing site, the widely recognised universality of RSV as a major cause of pediatric morbidity makes such testing criteria likely similar at each site, such that the patterns of RSV incidence obtained from these INSPIRE network laboratories are very likely globally generalizable and real.
In addition, in this data set, PIV, a related paramyxovirus, appears to be associated with this high RSV incidence, more strongly than with IAV (Fig. 2), which might be explained by the tendency of RSV and PIV to infect younger patients, compared to IAV,42 particularly where childhood influenza vaccination programs are in place.43 However, due to the unavailability of patient age data in most of the datasets from each site, further studies will explore this link in greater detail.
Thirdly, there is a well-defined synchrony for IAV, IBV and RSV incidences across the countries within the INSPIRE network, as well as from multiple intra-country sites where such data is available (Canada, UK, Australia) (Fig. 4). In contrast, the marked difference between the virus incidence data between populations of Singapore and Kuala Lumpur is conspicuous, despite them being relatively close geographically. The reasons for this unexpected finding are unclear and require further investigation. This will help us to understand further the factors contributing to seasonality of these respiratory viruses in tropical regions.
Although some of this incidence data may have been submitted to other publicly available website (such as WHO's FluNet), this study utilises the raw data available from the participating laboratories, rather than extracting the data from published articles. The ability to communicate directly with the laboratories within the network offers many additional benefits, including the availability of additional metadata to enhance the analysis and the interpretation of any results obtained.
Conclusions
The main differences between this study and previously published, mostly single-site studies, is that it allows a direct contemporaneous comparison of the incidence of the same viruses across the globe, which enables the detection of unusual trends in seasonality that may need further investigation – such as the intra-country synchrony of incidence seen in Canada and Australia, and the contrasting seasonality between the geographically much closer neighbouring cities of Kuala Lumpur and Singapore.
Therefore, we propose that a regular timely update of such global, contemporaneous, incidence data, from surveillance or diagnostic data sources, will be a valuable indicator of changes in the overall global respiratory virus epidemiology. This is not otherwise possible with single-site studies or more limited international data sets. Such incidence data can be used to estimate and compare the potential local healthcare burden across each of these sites.
Conflicts of interest
None of the authors have any competing interests/Conflicts of interest to declare.
Funding
ST was supported by the Children's Hospital Foundation Sakzewski Translational Research grant (no. 10416). TL was supported by Theme-based Research Scheme (T11-705/14-N) from University Grants Committee of the HKSAR.
Conference presentation
This paper was presented, in part, as a poster (APCCMI-IICC18-1109). 17th Asia-Pacific Congress of Clinical Microbiology and Infection cum 8th International Infection Control Conference, Hong Kong. 30 AUG - 2 SEP 2018: https://www.apccmi-iicc2018.hk/articles.html
Footnotes
Supplementary material associated with this article can be found, in the online version, at 10.1016/j.jinf.2019.07.008.
References
Full text links
Read article at publisher's site: https://doi.org/10.1016/j.jinf.2019.07.008
Read article for free, from open access legal sources, via Unpaywall:
http://www.journalofinfection.com/article/S016344531930218X/pdf
Citations & impact
Impact metrics
Article citations
Multiplexed detection of respiratory pathogens using a portable device combining a CREM strategy.
Chem Sci, 08 Oct 2024
Cited by: 0 articles | PMID: 39421201 | PMCID: PMC11480825
Burden of Disease Due to Respiratory Syncytial Virus in Adults in Five Middle-Income Countries.
Infect Dis Rep, 16(4):750-762, 15 Aug 2024
Cited by: 0 articles | PMID: 39195008 | PMCID: PMC11354146
Estimating the incidence of COVID-19, influenza and respiratory syncytial virus infection in three regions of Queensland, Australia, winter 2022: findings from a novel longitudinal testing-based sentinel surveillance programme.
BMJ Open, 14(4):e081793, 22 Apr 2024
Cited by: 0 articles | PMID: 38653507 | PMCID: PMC11043701
Seasonal extreme temperatures and short-term fine particulate matter increases pediatric respiratory healthcare encounters in a sparsely populated region of the intermountain western United States.
Environ Health, 23(1):40, 15 Apr 2024
Cited by: 1 article | PMID: 38622704 | PMCID: PMC11017546
Expert consensus on the diagnosis, treatment, and prevention of respiratory syncytial virus infections in children.
World J Pediatr, 20(1):11-25, 08 Dec 2023
Cited by: 4 articles | PMID: 38064012 | PMCID: PMC10828005
Review Free full text in Europe PMC
Go to all (39) article citations
Data
Data behind the article
This data has been text mined from the article, or deposited into data resources.
BioStudies: supplemental material and supporting data
Similar Articles
To arrive at the top five similar articles we use a word-weighted algorithm to compare words from the Title and Abstract of each citation.
Global patterns in monthly activity of influenza virus, respiratory syncytial virus, parainfluenza virus, and metapneumovirus: a systematic analysis.
Lancet Glob Health, 7(8):e1031-e1045, 01 Aug 2019
Cited by: 192 articles | PMID: 31303294
Review
Seasonality of distinct respiratory viruses in a tropical city: implications for prophylaxis.
Trop Med Int Health, 26(6):672-679, 18 Apr 2021
Cited by: 2 articles | PMID: 33666303
Comparative evaluation of real-time PCR and conventional RT-PCR during a 2 year surveillance for influenza and respiratory syncytial virus among children with acute respiratory infections in Kolkata, India, reveals a distinct seasonality of infection.
J Med Microbiol, 58(pt 12):1616-1622, 27 Aug 2009
Cited by: 39 articles | PMID: 19713363
Community respiratory virus infections following lung transplantation.
Transpl Infect Dis, 3(3):138-148, 01 Sep 2001
Cited by: 38 articles | PMID: 11493396
Review