Abstract
Free full text

Pulmonary Angiopathy in Severe COVID-19: Physiologic, Imaging, and Hematologic Observations
Abstract
Rationale: Clinical and epidemiologic data in coronavirus disease (COVID-19) have accrued rapidly since the outbreak, but few address the underlying pathophysiology.
Objectives: To ascertain the physiologic, hematologic, and imaging basis of lung injury in severe COVID-19 pneumonia.
Methods: Clinical, physiologic, and laboratory data were collated. Radiologic (computed tomography (CT) pulmonary angiography [n=
39] and dual-energy CT [DECT, n
=
20]) studies were evaluated: observers quantified CT patterns (including the extent of abnormal lung and the presence and extent of dilated peripheral vessels) and perfusion defects on DECT. Coagulation status was assessed using thromboelastography.
Measurements and Results: In 39 consecutive patients (male:female, 32:7; mean age, 53±
10 yr [range, 29–79 yr]; Black and minority ethnic, n
=
25 [64%]), there was a significant vascular perfusion abnormality and increased physiologic dead space (dynamic compliance, 33.7
±
14.7 ml/cm H2O; Murray lung injury score, 3.14
±
0.53; mean ventilatory ratios, 2.6
±
0.8) with evidence of hypercoagulability and fibrinolytic “shutdown”. The mean CT extent (±SD) of normally aerated lung, ground-glass opacification, and dense parenchymal opacification were 23.5
±
16.7%, 36.3
±
24.7%, and 42.7
±
27.1%, respectively. Dilated peripheral vessels were present in 21/33 (63.6%) patients with at least two assessable lobes (including 10/21 [47.6%] with no evidence of acute pulmonary emboli). Perfusion defects on DECT (assessable in 18/20 [90%]) were present in all patients (wedge-shaped, n
=
3; mottled, n
=
9; mixed pattern, n
=
6).
Conclusions: Physiologic, hematologic, and imaging data show not only the presence of a hypercoagulable phenotype in severe COVID-19 pneumonia but also markedly impaired pulmonary perfusion likely caused by pulmonary angiopathy and thrombosis.
The global spread of coronavirus disease (COVID-19), caused by severe acute respiratory syndrome coronavirus 2 (SARS-CoV-2), was classified a pandemic by the World Health Organization in March 2020. Respiratory failure is the dominant cause of death, and current management of COVID-19 centers on supportive care. However, it has been stated that respiratory physiology in COVID-19 differs from “conventional” acute respiratory distress syndrome (ARDS) (1). Emerging evidence suggests that aberrant and exuberant activation of inflammatory and coagulation cascades (termed “immunothrombosis”) in COVID-19 pneumonia together with elevated levels of IL-6, D-dimer, lactate dehydrogenase (LDH), and ferritin on admission are linked with higher mortality (2–5).
The other major issue in COVID-19 is the fundamental role of endothelial injury and disrupted vasoregulation (6). Indeed, the latter has been proposed as a key component in early COVID-19–related ARDS (7). Accordingly, we examined a cohort of mechanically ventilated patients with severe COVID-19 pneumonia abnormalities focusing on 1) physiologic data, 2) findings on computed tomographic pulmonary angiography (CTPA), 3) lung perfusion as demonstrated by dual-energy computed tomography (DECT) pulmonary blood volume “maps,” and 4) hematologic tests evidence of hypercoagulability and impaired fibrinolysis.
Methods
Study Design and Participants
This retrospective, observational study describes 39 consecutive mechanically ventilated patients with confirmed SARS-CoV-2 infection, admitted through a specialist severe respiratory failure and extracorporeal membrane oxygenation (ECMO) service. Patients were transferred from referral sites to our institution between March 17 and April 10, 2020. Eligible patients 1) were laboratory-confirmed positive for COVID-19, 2) were mechanically ventilated or on ECMO with active COVID-19–induced respiratory failure, and 3) had undergone CTPA including, where feasible, DECT. All patients were transferred either for the possible requirement of ECMO support or for further management after having already been commenced on ECMO at the referring center.
The study was approved by the Research Ethics Committee (reference number: 20/EE/0160). All patients lacked capacity, and the need for individual informed consent was waived for retrospective analysis of data collected prospectively for routine care, with no breach of privacy or anonymity.
Data Collection
Demographic, clinical, laboratory, and treatment data were extracted from electronic medical records and a point-of-care testing database. Respiratory physiology measurements are presented as on admission and shortly before CT-scan imaging. All clinical data were reviewed and collated by investigating physicians (B.V.P. and D.J.A.).
Laboratory Procedures
SARS-CoV-2 infection was confirmed on real-time PCR assay. Routine blood examinations included 1) complete blood count, 2) coagulation profile, 3) serum biochemical tests (including renal and liver function, LDH, and electrolytes), 4) myocardial enzymes, and 5) serum ferritin. Overall coagulation state and fibrinolysis were assessed using thromboelastography (TEG 6; Haemonetics) (see online supplement for interpretation). The frequency of investigations and further tests were determined by the treating physicians.
CT Image Acquisition and Interpretation
Full details regarding image acquisition protocols plus the quantification of morphologic CT patterns and perfusion defects on DECT images are provided in the online supplement. All patients were scanned in a dedicated COVID-19 CT suite using a second-generation dual-source CT system (Somatom Definition Flash; Siemens Healthineers). The methodology for CT interpretation was designed as an observational study, investigating a novel disease. Two observers (A.D. and S.R.D., both thoracic radiologists of 14 and 24 years’ experience, respectively), reviewed all available CT studies by consensus, blinded to clinical data (see online supplement). In brief, the overall extent of abnormal lung (to the nearest 5%) and the percentage of aerated, ground-glass opacification, and dense parenchymal opacification (denoting consolidated and/or atelectatic lung), up to total of 100%, as a component of abnormal lung were visually quantified. Additionally, the presence of peripheral dilated (branching and tortuous) vessels in the lung not obscured by dense parenchymal opacification was recorded. The incidence of venous thrombosis was confirmed by review of reports of lower and upper limb compression ultrasound and/or CT venography where available. In 20 of 39 (51%) cases, CTPA studies were acquired using the DECT technique; in those, 18/20 had assessable lobes (see online supplement). Pulmonary blood volume images acquired using DECT were reviewed by two thoracic radiologists (C.R. and S.P.G.P.; 14 and 30 years’ experience, respectively) (see online supplement) and evaluated for the presence/absence of perfusion defects and, when present, categorized as 1) wedge-shaped, 2) mottled, or 3) mixed based on appearances described in chronic thromboembolic disease.
Statistical Analysis
Descriptive statistics were used to summarize the data; results are reported as medians and interquartile ranges or means and SD, as appropriate. Categorical variables were summarized as counts and percentages. Normality for continuous variables was tested with the D’Agostino and Pearson normality test. Two-tailed t test, Mann-Whitney U test, or Kruskal-Wallis test with Dunn’s multicomparison was used to compare differences between groups where appropriate. Correlations were performed using Spearman correlation coefficient and nonlinear least squares regression fitting. A two-sided P<
0.05 was considered statistically significant. Statistical analyses were performed using GraphPad Prism v8.4 (GraphPad Software).
Results
Baseline Demographic and Clinical Characteristics of Patients with COVID-19
During the study period, 39 mechanically ventilated patients (male, 79.5%; mean age, 53±
10 yr; Black and minority ethnic, 64%) were admitted to our institution, of whom 20 (51%) also received ECMO support. The demographic and clinical characteristics are shown in Table 1. The mean duration of symptoms before hospital admission was 8.2
±
3.5 days, and the mean time between hospital admission and endotracheal intubation was 1.3
±
2.0 days. Comorbid chronic medical conditions and/or a body mass index >30 kg/m2 were present in 80% of patients. Of the 20 patients on ECMO, 3 were placed onto ECMO at our center, having already been transferred, whereas 17 were transferred from referring ICUs on mobile ECMO. The mean number of days of mechanical ventilation at the referring center, prior to transfer, was 3.2
±
3.1 days (range, 0–13 d). From admission, all patients received at least prophylactic dose of low-molecular-weight heparin or unfractionated heparin for patients on ECMO or renal replacement therapy.
Table 1.
Clinical and Laboratory Characterization
Demographics and Clinical Characteristics | Number (%), Median (Range), or Mean (±SD) |
---|---|
Age | 52.5 (29–79) |
Sex, M | 32 (82) |
Sex, F | 7 (18) |
White | 14 (36) |
Black and minority ethnic | 25 (64) |
BMI, kg/m2 | 31.3 (±6.1) |
BMI![]() ![]() | 22 (57) |
Diabetes mellitus | 8 (21) |
Hypertension | 15 (39) |
Asthma | 3 (8) |
Hyperlipidemia | 2 (5) |
Physiologic characteristics (on admission) | |
![]() | 114.9 (±74.2) |
![]() | 63.6 (±20.6) |
![]() | 11.7 (±2.2) |
![]() | 33.7 (±14.7) |
![]() | 12.3 (±2.4) |
![]() | 3.14 (±0.53) |
![]() | 2.6 (±0.8) |
![]() | 8.0 (±2.5) |
![]() | 18.7 (±5.0) |
![]() | 3.4 (±1.9) |
Laboratory tests on admission (normal values) | |
![]() | 10.6 (±4.4) |
![]() | 9.3 (±4.3) |
![]() | 0.76 (±0.4) |
![]() | 172 (±141) |
![]() | 305 (±101) |
![]() | 987 (552–1,425) |
![]() | 996 (773–1,270) |
![]() | 272 (±77) |
![]() | 6.6 (±1.9) |
![]() | 70.6 (±23.7) |
![]() | 38.8 (±13.1) |
![]() | 14.1 (±2.1) |
![]() | 6,440 (±10,434) |
![]() | 143 (±262) |
![]() | 186 (±274) |
Definition of abbreviations: APACHE=
The Acute Physiology and Chronic Health Evaluation; APTT
=
activated partial thromboplastin time; BMI
=
body mass index; CRP
=
C-reactive protein; ECMO
=
extracorporeal membrane oxygenation; PT
=
prothrombin time.
Physiologic and Laboratory Findings
The clinical and laboratory findings in all patients on admission are given in Table 1. Lymphocytopenia was a common finding with significantly elevated markers of organ damage and inflammation including creatinine, troponin, LDH, ferritin, and C-reactive protein. ARDS severity on admission was graded as moderate to severe: PaO2/FiO2=
114.9
±
74.2 with dynamic respiratory system compliance (Crs)
=
33.7
±
14.7 ml/cm H2O, and Murray lung injury score
=
3.14
±
0.53. Dynamic Crs was correlated with PaO2/FiO2 (r
=
0.49; P
=
0.0017). Increased positive end-expiratory pressure (PEEP) was associated with worse PaO2/FiO2 (r
=
−0.38; P
=
0.02) and a higher ventilatory ratio (an indicator of ventilatory insufficiency and increased physiological dead space) (r
=
0.49; P
=
0.0048); furthermore, there was a strong negative correlation between PaO2/FiO2 and ventilatory ratio (r
=
−0.65; P
<
0.0001) independent of dynamic Crs (Figure 1).
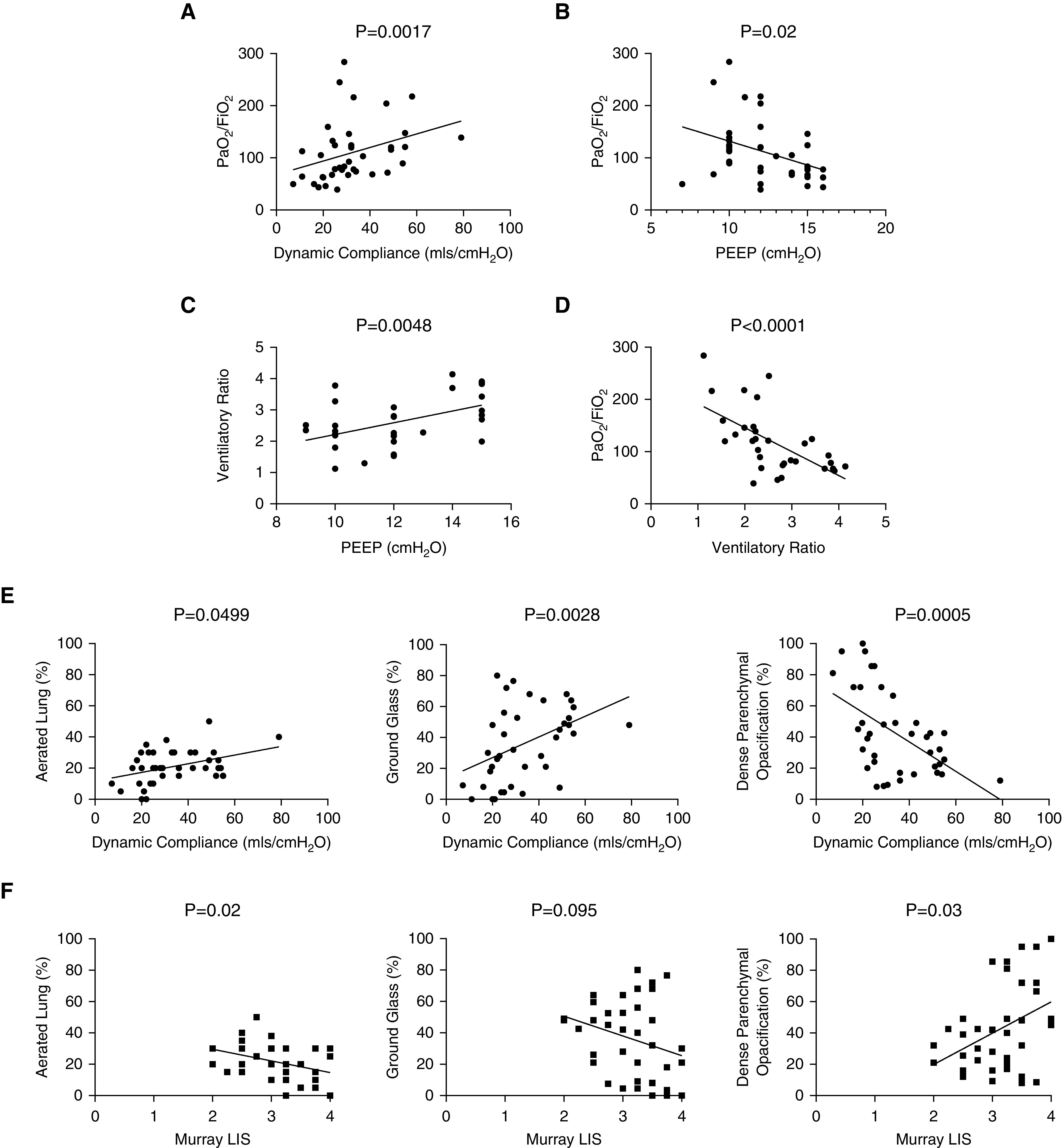
Physiologic correlations (on admission) between (A) PaO2/FiO2 and dynamic respiratory system compliance (N=
39; r
=
0.485; P
=
0.0017); (B) PaO2/FiO2and positive end-expiratory pressure (PEEP) (N
=
38; r
=
−0.377; P
=
0.02); (C) ventilatory ratio (VR) and PEEP (N
=
32; r
=
0.486; P
=
0.0048); (D) PaO2/FiO2 and VR (N
=
38; r
=
−0.649; P
<
0.0001). (E) Associations between computed tomography (CT) features and dynamic compliance (on day of CT scan) showing positive correlations with percentage aeration (N
=
39; r
=
−0.316; P
=
0.499) and percentage ground-glass opacification (N
=
39; r
=
−0.466; P
=
0.0028) and negative correlations with dense parenchymal opacification (N
=
39; r
=
−0.362; P
<
0.0001). (F) Associations between CT features and Murray lung injury score showing negative correlations with percentage aeration (N
=
39; r
=
−0.365; P
=
0.022) and percentage ground-glass opacification (N
=
39; r
=
−0.271; P
=
0.095) and positive correlations with dense parenchymal opacification (N
=
39; r
=
0.349; P
=
0.03). LIS
=
lung injury score.
CTPA and DECT Findings
CTPA was performed in 39 patients, and, for reasons of technical limitation (i.e., body mass index ≥35 or an inability to arm-raise), 20/39 (51%) underwent DECT. Eighteen of 39 (46%) patients were on ECMO at the time of CT; two patients were subsequently placed on ECMO and data were analyzed at the time of CT scan. The median interval between intubation and CT was 6 days (range, 0–18 d).
The mean CT extent of aeration, ground-glass opacification (%GGO), and dense parenchymal opacification (%DPO) were 21.1±
10.8%, 36.3
±
24.7%, and 42.7
±
27.1%, respectively (Table 1). Crs (on day of CT imaging) was positively correlated with extent of normally aerated lung (P
=
0.0499) and %GGO (P
=
0.0028) but negatively with the extent of %DPO (P
=
0.0005) (Figure 1). There were no correlations between CT findings and PaO2/FiO2 or ventilatory ratio.
There was a negative correlation in percentage aeration (P=
0.02) and positive correlation in %DPO (P
=
0.03) with increasing severity of injury as assessed using the Murray lung injury score. There was tendency to reduction in %GGO with increasing with worsening injury (Figure 1).
Vascular Perfusion Abnormalities on CTPA and DECT Findings
Dilated (tortuous and branching) peripheral vessels (Figure 2) were present on CT in 21 out of 33 (63.6%) patients (Table 2) who had at least two “assessable” lobes (i.e., those with <50% obscuration by dense parenchymal opacification—see also online supplement); dilated peripheral vessels were seen in 10/21 (47.6%) without pulmonary embolism (PE). Arterial filling defects on CTPA, consistent with acute PE, were present in 15/39 (38%) patients. The prevalence of dilated peripheral vessels increased significantly with increasing duration of hospitalization and length of ventilation (Figure 2).
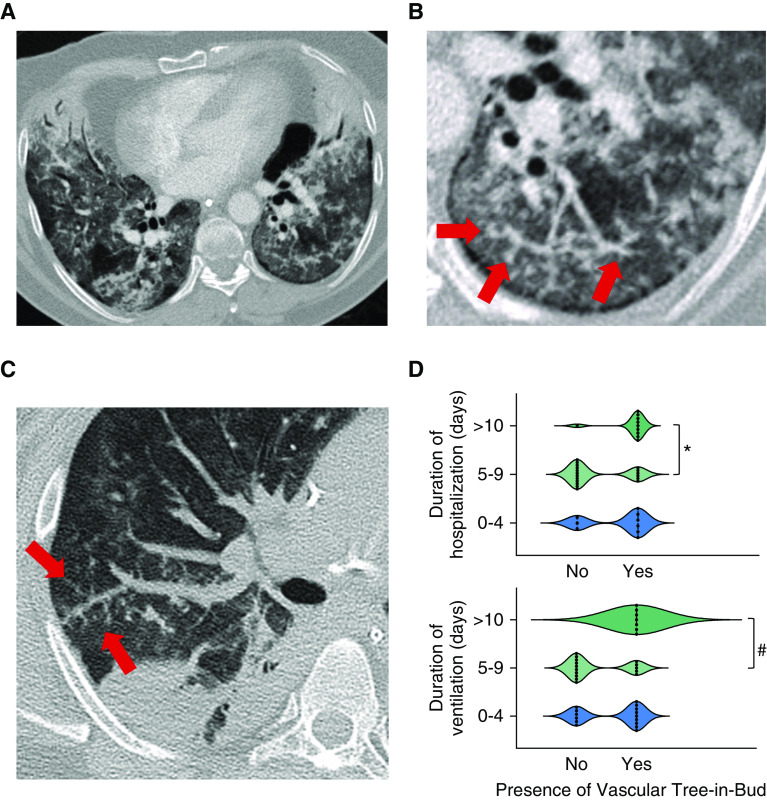
The computed tomography “vascular tree-in-bud pattern” in two patients with severe coronavirus disease (COVID-19) pneumonia. (A) A 52-year-old male patient scanned on day 1 following intubation. There is bilateral ground-glass opacification and patchy consolidation. Dilated branching and tortuous vessels are present in the left lower lobe representing the vascular tree-in-bud pattern. (B) Targeted, enlarged image of the left lower lobe in the same patient and from the same image slice showing bizarre dilated subsegmental vessels in greater detail (arrows). (C) Targeted image of the right lung in a second patient again showing striking dilatation of vessels in the right upper lobe and a vascular tree-in-bud pattern (arrows). (D) Relationship between the prevalence of the vascular tree-in-bud pattern and duration of hospitalization (*P=
0.013 with Kruskal-Wallis; Dunn’s multicomparison P
=
0.0127: group “5–9” vs. “>10”) and ventilation (#P
=
0.0142 with Kruskal-Wallis; Dunn’s multicomparison P
=
0.0112: group “5–9” vs. “>10”).
Table 2.
Computed Tomography Abnormalities in 39 Mechanically Ventilated Patients with Severe COVID-19 Pneumonia
Computed Tomography Findings | All (N![]() ![]() | |
---|---|---|
Aerated lung, % | 23.5 (±16.7) | |
Ground-glass opacity, % | 36.3 (±24.7) | |
Dense parenchymal opacification, % | 42.7 (±27.1) |
Number of Patients | Proportion (%) | |
---|---|---|
Pulmonary embolism | 15 | 15/39 (38.5) |
Dilated peripheral vessels | 21 | 21/33* (63.6) |
![]() | 14 | 14/21 (66.7) |
![]() | 7 | 7/21(33.3) |
![]() | 0 | 0/21 (0) |
Dilated peripheral vessels without PE | 10 | 10/21 (47.6) |
Perfusion defect present | 18 | 18/18† (100) |
![]() | 14 | 14/18 (77.8) |
![]() | 4 | 4/18 (22.2) |
![]() | 0 | 0/18 |
Perfusion defects without PE | 8 | 8/18 (44.4) |
DECT perfusion defect morphology | ||
![]() | 3 | 3/18 (16.7) |
![]() | 9 | 9/18 (50) |
![]() | 6 | 6/18 (33.3) |
Deep venous thrombosis | 4 | 4/22‡ (18.2) |
Definition of abbreviations: COVID-19=
coronavirus disease; DECT
=
dual-energy computed tomography; PE
=
pulmonary embolism.
Lung parenchyma was considered assessable on DECT in 18/20 patients. Perfusion defects (Figure 3) were present in 18/18 (100%) patients, with a median extent of 46.3% (range, 20.0–77.5%) (Figure 3). Perfusion defects were classed as wedge-shaped (Videos E1A and E1B in the online supplement) in three patients, mottled (Videos E2A and E2B) in nine, and mixed (i.e., both wedge-shaped and mottled patterns coexisted; Videos E3A and E3Bb) in six (Figure 3). A healthy control DECT (Figure 3 and Video E4) shows a homogeneous color map indicating normal iodine distribution and perfusion.
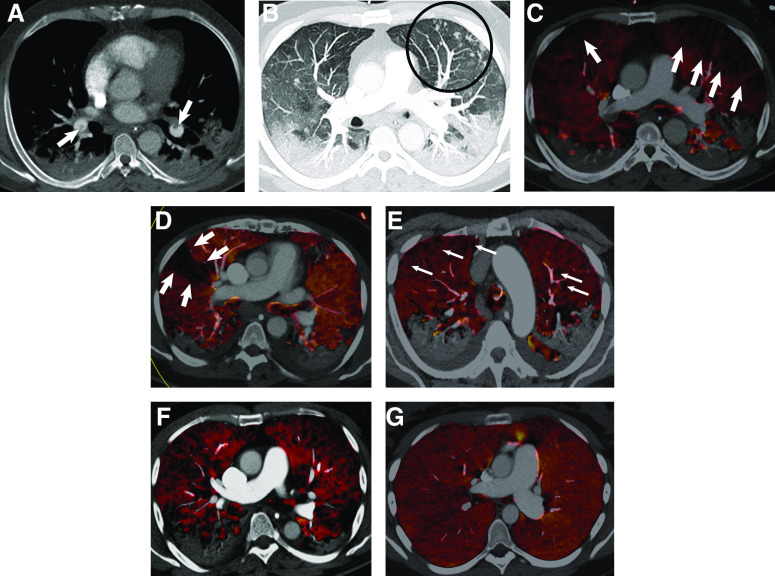
(A–C) Computed tomography (CT) pulmonary angiography and dual-energy CT (DECT) perfusion in a 47-year-old male patient with coronavirus disease (COVID-19) pneumonia, day 9 after intubation. (A) Soft-tissue reconstruction showing filling defects in lower lobe pulmonary arteries (thick arrows). (B) Maximal-intensity-projection CT images showing vascular tree-in-bud pattern (circled) in the left upper lobe anterolaterally and (C) corresponding DECT perfused blood volume color map showing widespread perfusion defects (thick arrows). (D–F) Representative DECT perfused blood volume color maps in three patients showing wedge-shaped (thick arrows) (D), mottled (thin arrows) (E), and mixed (F) perfusion defects. (G) Axial perfused blood volume images of the lungs in a 32-year-old female patient without COVID-19 or pulmonary embolism demonstrates a homogeneous color map indicating normal iodine distribution.
Coagulation Abnormalities
D-dimer, platelet count, fibrinogen, prothrombin time, activated partial thromboplastin time, and antithrombin levels on admission are shown in Table 1. TEG data from the citrated kaolin heparinase channel showed hypercoagulability characterized by raised maximal amplitude (MA) and absent fibrinolysis (Figure 4A); the MA represents the maximal clot strength as determined by platelet number and function (of note, 80% of MA contribution is from platelets and the other 20% from fibrinogen). Twenty-one of 39 (54%) patients had MA above the normal reference range (52–69 mm) with an overall median MA of 69.2 mm (range, 52–75 mm) despite being on at least prophylactic dose of low-molecular-weight heparin or unfractionated heparin with factor Xa levels of 0.2–0.3 IU/ml. There were significantly raised functional fibrinogen levels in the citrated functional fibrinogen trace suggesting a strong contribution of platelets and fibrin to clot strength. Twenty-nine of 39 patients (74%) had functional fibrinogen levels higher than the normal reference range (15–32 mm) with an overall median of 56 mm (range, 23–69 mm). All patients showed absent fibrinolysis as evident by LY30 of 0% across the whole cohort, whereas normal fibrinolysis activation shows some evidence of activation by 30 minutes (Figure 4B).
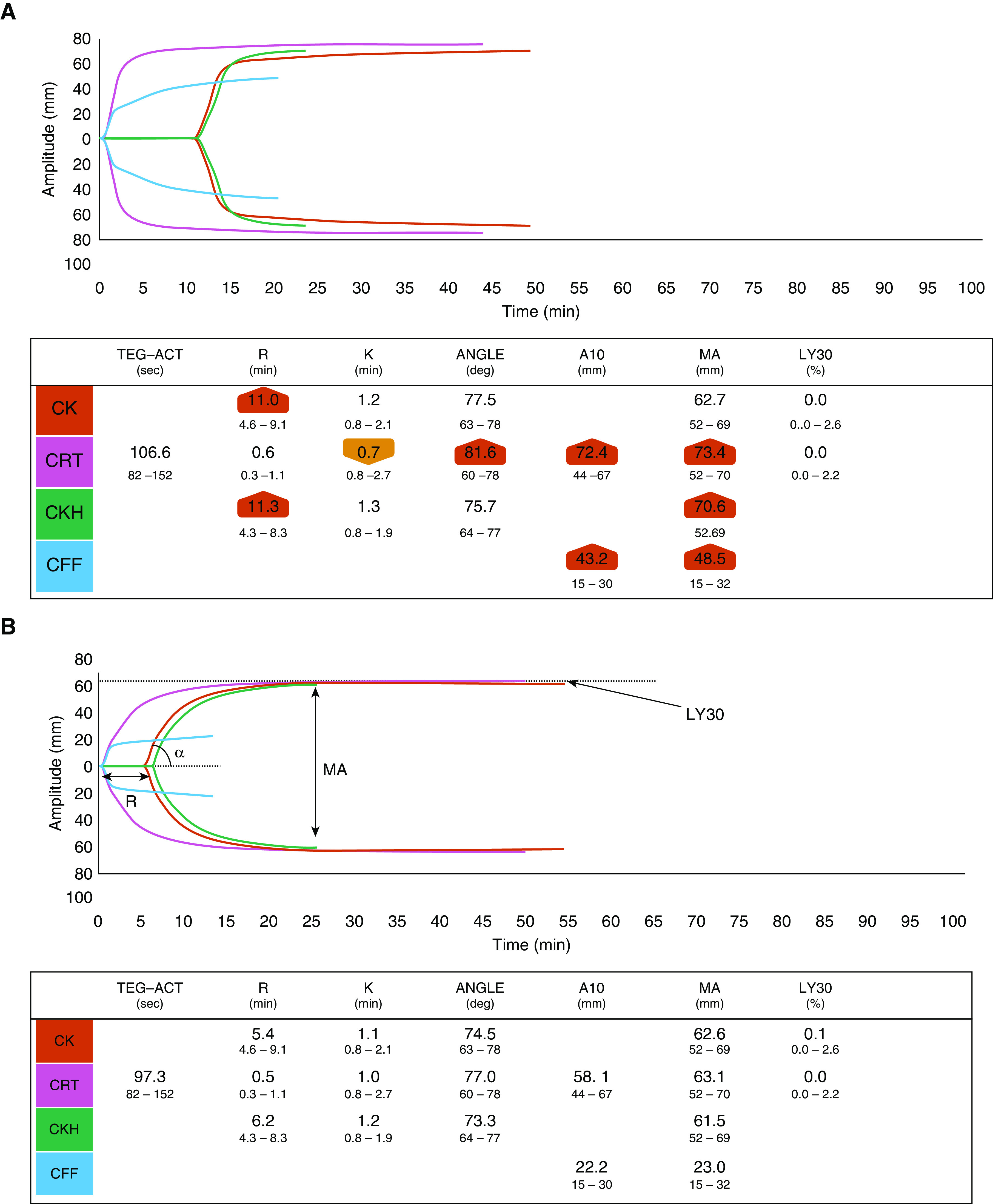
Representative thromboelastography (TEG) tracings of a (A) ventilated patient with coronavirus disease (COVID-19) and (B) a control healthy volunteer. The patient TEG shows universal hypercoagulability, with higher α-angle and maximal amplitude (MA), and absent fibrinolysis at 30 minutes (LY30=
0%). The most frequently used parameters in TEG include reaction time, which reflects the time of latency from start of test to initial fibrin formation, which is prolonged if the patient is on an anticoagulant or has a coagulation factor deficiency. Heparinase TEG eliminates the effect of heparin. The α-angle measures the speed at which fibrin buildup and cross-linking takes place and hence assesses the rate of clot formation but also provides information on fibrin formation and cross-linking. The MA is a measure of the ultimate strength of the fibrin clot, that is, the overall stability of the clot. This is dependent on platelets (80%) and fibrin (20%) interactions. Fibrinolysis activation (the percentage lysis at 30 min after MA) is evident in the control TEG with the red trace beginning to decrease in amplitude. In contrast, the COVID-19 red trace continues to show a slow increase in amplitude. A10
=
amplitude 10 minutes after the time blood starts to clot; ACT
=
activated clotting time; CFF
=
citrated blood sample activated by the functional fibrinogen test; CK
=
citrated blood sample activated with kaolin; CKH
=
citrated blood sample activated with kaolin and heparinase; CRT
=
citrated blood sample activated with RapidTEG; K
=
coagulation time (min); LY30
=
percentage lysis at 30 minutes after MA; R
=
reaction time.
Discussion
Mechanically ventilated patients with severe COVID-19 pneumonia present with hypercapnic respiratory failure (8) and a relatively preserved respiratory system compliance initially (9), reflecting increased pulmonary dead space and a predominant defect in pulmonary perfusion. To the best of our knowledge, the current study is the first to systematically evaluate the combined physiologic, hematologic, and morphologic abnormalities in patients with COVID-19 pneumonia. Our observations point to an increased physiologic dead space, hypercoagulability (with absent fibrinolysis), and imaging signs of major vascular involvement. Accordingly, and in light of emerging pathologic evidence of major vascular involvement (6, 10, 11), we suggest that our results support the presence of a widespread pulmonary angiopathy in severe COVID-19 pneumonia.
Interest in the physiology of COVID-19–induced ARDS stemmed from the proposal by Gattinoni and colleagues of two broad (but almost certainly overlapping) phenotypes: “type L,” with high lung compliance and limited ground-glass opacification on CT and responsive to lower PEEP, and “type H,” in which compliance is low and disease more extensive on CT, potentially benefitting from higher PEEP (1). This apparently simple dichotomy has attractions but has been questioned: Bos and colleagues found no relationship between compliance and qualitative CT measurements, suggesting that these phenotypes may not be mutually exclusive (12). However, our data also confirm considerable overlap in phenotypes, with all patients showing a significant (>50% of lung volume) combination of ground-glass and diffuse parenchymal opacification, presumably related to the progression of disease. The latter was confirmed with patients showing a higher Murray lung injury score showing lower percentage aeration/ground-glass opacification but greater dense parenchymal opacification.
The ventilatory ratio—a simple bedside index of physiological dead space fraction in patients with ARDS (13)—was associated with worsening hypoxemia, in line with other studies of COVID-19–related ARDS (14). Moreover, the association between higher PEEP (i.e., lower PaO2/FiO2) and worsening hypoxemia and greater physiological dead space fraction (i.e., higher ventilatory ratio) all point to disproportionate vascular dysregulation compounded by redirection of perfusion away from overdistended oxygenated alveoli, without the benefits of additional lung recruitment, in line with recent studies of the variable efficacy of lung recruitment in COVID-19–induced ARDS (15, 16).
Two crucial observations from our study shed light on the possible pathophysiological explanation for this increased physiological dead space: first, the frequent presence of dilated, branching, and/or tortuous vessels in the peripheral lung, and second, perfusion defects on DECT. We believe that the dilatation of peripheral vessels is an extension of the enlarged vessels reported by others (17–19). Albarello and colleagues commented on enlarged tubular vessels in two patients (17). In a larger study, Caruso and coworkers found enlarged subsegmental vessels in 89% of 158 patients (19). The peripheral location and branching nature of the dilated vessels in our study bears a striking resemblance to the CT findings in rare patients with pulmonary tumor thrombotic microangiopathy (20): in the first report of its kind, the authors described the pathologic and CT features of a 48-year-old patient with progressive breathlessness. On thin-section CT, there were bilateral longitudinal branching opacities, the so-called “vascular tree-in-bud” pattern. Cardiac catheterization confirmed severe pulmonary hypertension, and at postmortem, most small arteries and arterioles were occluded by fibrocellular intimal hyperplasia and clumps of tumor cells were seen in some arterioles. The logical explanation, presumably, is that the small arterioles—normally not resolved on CT—were enlarged and thus rendered visible (20). It has been previously suggested that vessel enlargement in COVID-19 pneumonia might be a marker of increased blood flow (21). However, we do not subscribe to this explanation but instead suspect that the vascular tree-in-bud pattern in COVID-19 likely is a manifestation of pulmonary thrombotic angiopathy. Support for this comes from postmortem data (11, 22). In one of these studies, features of diffuse alveolar damage were present in all cases, but COVID-19 lungs were distinguished by the presence of widespread microthromboses and striking new vessel growth; the latter was termed “intussusceptive angiogenesis” and linked with increasing hospitalization (11). Intriguingly, albeit in a small number, we also found a linkage between the vascular tree-in-bud pattern and duration of both hospitalization and ventilation before CT. Thus, it is tempting to speculate that the vascular tree-in-bud pattern in COVID-19 pneumonia may be an important CT marker of immunothrombosis and angiogenesis.
The DECT scanning technique, although lacking a routine role in the imaging of lung disease, has certainly attracted attention as an alternative means of assessing pulmonary perfusion in acute and chronic thromboembolic disease (23–25). In the specific context of chronic thromboembolic disease, there is high concordance between findings on DECT and traditional ventilation-perfusion imaging (24, 25). Indeed, patterns of perfusion abnormality appear to differ significantly between chronic thromboembolic disease and patients with pulmonary arterial hypertension (24). The striking finding of abnormal pulmonary perfusion on DECT has not, to the best of our knowledge, been fully explored in COVID-19 pneumonia (21). Abnormalities of perfusion were seen in all patients with assessable lobes, irrespective of whether this was in dependent or nondependent areas of the lung. This finding in patients with the most severe COVID-19–related respiratory failure suggests that vascular dysregulation is not, as has been proposed, restricted to those with the early or so-called “type L” phenotype (7). The finding of abnormal perfusion, although supporting the hypothesis of major vascular involvement, was certainly unexpected in the context of ARDS where, given the inflammatory pathology, one might have expected regions of overperfused lung on DECT (26).
Given the preferential respiratory route of entry and significant expression of ACEII in vascular endothelium (27), it might be speculated that SARS-CoV-2 might, through direct endothelial infection (facilitated by the ACEII receptor uptake), lead to significant pulmonary microvascular endothelial injury with associated viremia (6). Interestingly, SARS-CoV-1 has been shown to induce pulmonary cellular necrosis (28), and the pathobiological processes leading to immunothrombosis (endothelial injury, vascular inflammation, and thrombotic microangiopathy) might then serve to explain our observation of dilated peripheral vessels and perfusion defects on imaging. Furthermore, the vascular injury and so-called “cytokine storm” of COVID-19, with its release of eicosanoids (e.g., arachidonic acid), could stimulate platelet aggregation, simultaneous with a release of adenine nucleotides from activated platelets promoting thrombus formation, further amplifying the platelet response (29, 30). Indeed, pulmonary vascular inflammation, in particular, leukocyte–platelet interactions, is associated with the development of ARDS from non-COVID etiologies (31).
The issue of deep venous thrombosis (DVT) and acute PE in COVID-19 pneumonia merits brief consideration. Approximately 90% of symptomatic PEs are known to originate from thrombi located in lower limbs (32, 33). Moreover, in line with other viral pneumonias (34, 35), PE is reported in patients with COVID-19 (36, 37) and, admittedly, was present on CT in 15 of 39 patients in our study. Thus, it might conceivably be argued that the dilated peripheral vessels in our patients were simply being rendered visible on CT by embolic material from upper- or lower-limb DVT. However, against this, DVT was recorded in only 4 of 22 patients undergoing lower-limb compression ultrasound or CT venography, and only 1 patient with PE had DVT. Furthermore, it is noteworthy that dilatation of peripheral vessels with embolic material has, as far as we are aware, never been documented in patients with acute or chronic PE. Although accepting fully that DVT occurs commonly in critically ill patients (and that these may result in PE), we suggest that in COVID-19 there may be dual pathologies at play, namely, acute PE from DVT and a widespread pulmonary angiopathy.
We regard the hematological tests in our patients as important and complementary, serving to confirm hypercoagulability (as evidenced by raised MA, functional fibrinogen levels), and absent fibrinolysis given the LY30 of 0% in all patients. The term fibrinolysis “shutdown” refers to the acute impairment of fibrinolysis (38). Impaired fibrinolysis is regarded as a predictor of first and recurrent PE (39). Although use of TEG outside cardiac surgery and liver disease is limited, there is emerging evidence from several studies for a role for viscoelastic tests in assessing the bleeding and thrombotic risk in critically ill patients with infection/inflammation (40). Furthermore, hypercoagulability as evidence by TEG has been shown to be predictive of thrombosis in patients with trauma and in the general population (41).
There are clear limitations to our study, not least the small sample size, the retrospective observational nature, the absence of matched (non–COVID-19) controls, the lack of a validation cohort, and, given the tertiary nature of our practice, the focus on patients with severe COVID-19–induced respiratory failure. The physiology does not directly prove pulmonary angiopathy, but we believe such relatively simple bedside physiological assessments may enable further diagnostic evaluation of vascular abnormalities and pulmonary angiopathy in severe COVID-19. Future studies comparing a matched control group of patients with non–COVID-19–related ARDS are clearly necessary to ascertain whether the imaging findings we report herein are truly related to SARS-CoV-2. However, in light of current evidence of a significant vascular component to COVID-19 (7, 11), we propose that our observations not only provide important noninvasive evidence of pulmonary vascular involvement through imaging but also add to the understanding of clinicophysiological phenotypes and pathobiology of COVID-19 pneumonia/ARDS.
In summary, our observations on CTPA and DECT, coupled with the physiologic and hematologic features, point to major pulmonary vascular involvement in severe COVID-19 pneumonia. Further validation of these imaging markers is clearly warranted, but in light of the emerging pathologic evidence of angiopathy, we believe our findings have implications for further pathobiologic and therapeutic studies.
Footnotes
A list of members of the Severe Acute Respiratory Failure Service and the Departments of Anesthesia and Critical Care is provided in the online supplement.
Author Contributions: Study concepts and design: B.V.P., D.J.A., C.A.R., S.P.G.P., A.D., and S.R.D. Literature review: B.V.P., D.J.A., C.A.R., S.P.G.P., A.D., and S.R.D. Clinical data collection: all authors. Manuscript preparation/editing: B.V.P., D.J.A., C.A.R., S.P.G.P., A.D., and S.R.D. Data analysis: B.V.P., D.J.A., C.A.R., S.P.G.P., A.D., and S.R.D. Statistical analysis: B.V.P., C.A.R., and A.D. Final manuscript review: all authors.
This article has a related editorial.
This article has an online supplement, which is accessible from this issue’s table of contents at www.atsjournals.org.
Originally Published in Press as DOI: 10.1164/rccm.202004-1412OC on July 15, 2020
Author disclosures are available with the text of this article at www.atsjournals.org.
References
Articles from American Journal of Respiratory and Critical Care Medicine are provided here courtesy of American Thoracic Society
Citations & impact
Impact metrics
Citations of article over time
Alternative metrics
Smart citations by scite.ai
Explore citation contexts and check if this article has been
supported or disputed.
https://scite.ai/reports/10.1164/rccm.202004-1412oc
Article citations
A pooled analysis of the incidence and mortality risk of atrial fibrillation in patients with COVID-19.
PeerJ, 12:e18330, 16 Oct 2024
Cited by: 0 articles | PMID: 39430559 | PMCID: PMC11490229
Review Free full text in Europe PMC
Lung imaging methods: indications, strengths and limitations.
Breathe (Sheff), 20(3):230127, 01 Oct 2024
Cited by: 0 articles | PMID: 39360028 | PMCID: PMC11444493
Review Free full text in Europe PMC
Physiologic Effects of Extracorporeal Membrane Oxygenation in Patients with Severe Acute Respiratory Distress Syndrome.
Am J Respir Crit Care Med, 210(5):629-638, 01 Sep 2024
Cited by: 1 article | PMID: 38526489 | PMCID: PMC11389568
Respiratory mechanics characteristics at the time of barotrauma presentation in patients with critical COVID-19 infection.
Crit Care Sci, 36:e20240248en, 30 Aug 2024
Cited by: 0 articles | PMID: 39230074 | PMCID: PMC11463995
Contribution of intrapulmonary shunt to the pathogenesis of profound hypoxaemia in viral infection: a mechanistic discussion with an illustrative case.
J Intensive Care Soc, 25(4):427-431, 06 Aug 2024
Cited by: 0 articles | PMID: 39524067 | PMCID: PMC11549710
Go to all (196) article citations
Data
Data behind the article
This data has been text mined from the article, or deposited into data resources.
BioStudies: supplemental material and supporting data
Similar Articles
To arrive at the top five similar articles we use a word-weighted algorithm to compare words from the Title and Abstract of each citation.
Clinical Features and Chest CT Manifestations of Coronavirus Disease 2019 (COVID-19) in a Single-Center Study in Shanghai, China.
AJR Am J Roentgenol, 215(1):121-126, 14 Mar 2020
Cited by: 150 articles | PMID: 32174128
Imaging and clinical features of patients with 2019 novel coronavirus SARS-CoV-2.
Eur J Nucl Med Mol Imaging, 47(5):1275-1280, 28 Feb 2020
Cited by: 421 articles | PMID: 32107577 | PMCID: PMC7080117
Pulmonary circulation abnormalities in post-acute COVID-19 syndrome: dual-energy CT angiographic findings in 79 patients.
Eur Radiol, 33(7):4700-4712, 25 Apr 2023
Cited by: 7 articles | PMID: 37145145 | PMCID: PMC10129318
Chest CT in COVID-19 pneumonia: A review of current knowledge.
Diagn Interv Imaging, 101(7-8):431-437, 11 Jun 2020
Cited by: 57 articles | PMID: 32571748 | PMCID: PMC7287482
Review Free full text in Europe PMC