Abstract
Free full text

Neuropilin-1 is a host factor for SARS-CoV-2 infection
Abstract
SARS-CoV-2, the causative agent of COVID-19, uses the viral Spike (S) protein for host cell attachment and entry. The host protease furin cleaves the full-length precursor S glycoprotein into two associated polypeptides: S1 and S2. Cleavage of S generates a polybasic Arg-Arg-Ala-Arg C-terminal sequence on S1, which conforms to a C-end rule (CendR) motif that binds to cell surface Neuropilin-1 (NRP1) and Neuropilin-2 (NRP2) receptors. Here, using X-ray crystallography and biochemical approaches we show that the S1 CendR motif directly bound NRP1. Blocking this interaction using RNAi or selective inhibitors reduced SARS-CoV-2 entry and infectivity in cell culture. NRP1 thus serves as a host factor for SARS-CoV-2 infection and may potentially provide a therapeutic target for COVID-19.
SARS-CoV-2 is the coronavirus responsible for the current COVID-19 pandemic (1,2). A striking difference between the S protein of SARS-CoV-2 and SARS-CoV is the presence, in the former, of a polybasic sequence motif, RRAR, at the S1/S2 boundary. It provides a cleavage site for a host proprotein convertase, furin (3–5) (Fig. S1A). The resulting two proteins, S1 and S2, remain non-covalently associated, with the serine protease TMPRSS2 further priming S2 (6). Furin-mediated processing increases infectivity and affects the tropism of SARS-CoV-2, while furin inhibition diminishes SARS-CoV-2 entry, and deletion of the polybasic site in the S protein reduces syncytia formation in cell culture (3–5,7).
The C-terminus of the S1 protein generated by furin cleavage has an amino acid sequence (682RRAR685), that conforms to a [R/K]XX[R/K] motif, termed the ‘C-end rule’ (CendR) (Fig. S1B) (8). CendR peptides bind to Neuropilin-1 (NRP1) and NRP2, transmembrane receptors that regulate pleiotropic biological processes, including axon guidance, angiogenesis and vascular permeability (8–10). To explore the possibility that the SARS-CoV-2 S1 protein may associate with neuropilins we generated a GFP-tagged S1 construct (GFP-S1) (Fig. S1C). When expressed in HEK293T cells engineered to express the SARS-CoV-2 receptor ACE2, GFP-S1 immunoprecipitated endogenous NRP1 and ACE2 (Fig. 1A). We transiently co-expressed NRP1-mCherry and either GFP-S1 or GFP-S1 ΔRRAR (a deletion of the terminal 682RRAR685 residues) in HEK293T cells. NRP1 immunoprecipitated the S1 protein, and deletion of the CendR motif reduced this association (Fig. 1B). Comparable binding was also observed with mCherry-NRP2, a receptor with high homology to NRP1 (Fig. S1D, S1E). In both cases, residual binding was observed with the ΔRRAR mutant indicating an additional CendR-independent association between neuropilins and the S1 protein.
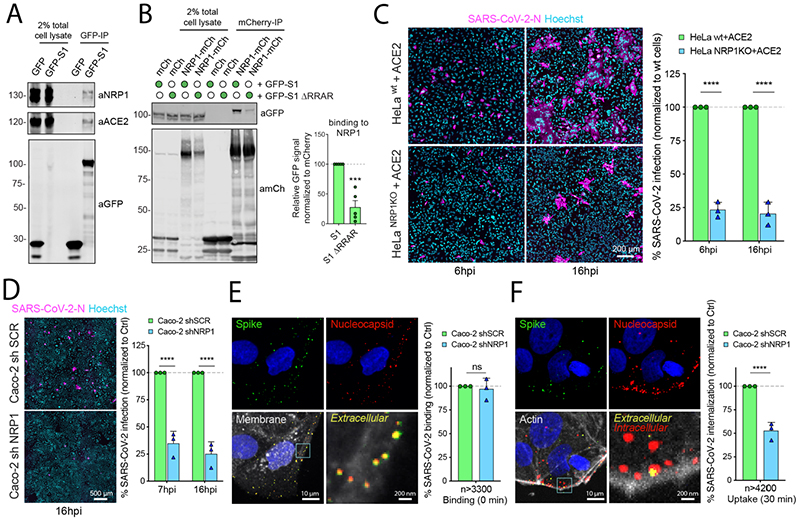
(A) HEK293T cells transduced to express ACE2 were transfected to express GFP or GFP-tagged S1 and lysed after 24h. The lysates were subjected to GFP-nanotrap and the immune-isolates were blotted for ACE2 and NRP1 (N=3). (B) HEK293T cells were co-transfected to express GFP-tagged S1 or GFP-S1 ΔRRAR and mCherry or mCherry-tagged NRP1 and subjected to GFP-nanotrap (N=5). Two-tailed unpaired t-test; P= 0.0002. (C) HeLawt+ACE2 and HeLaNRP1 KO+ACE2 cells were infected with SARS-CoV-2. Cells were fixed at 6 or 16 hpi and stained for N protein (magenta) and Hoechst (cyan), and virus infectivity was quantified (N=3). Two-tailed unpaired t-test; P=0.00002 and 0.00088. Scale bar=200 μm. (D) Caco-2 cells expressing shRNA against NRP1 or a non-targeting control (SCR) were infected with SARS-CoV-2 and fixed at 7 or 16 hpi. The cells were stained for N protein (magenta) and Hoechst (cyan), and infectivity was quantified (N=3). Two-tailed unpaired t-test; P=0.0005 and 0.00032. Scale bar=500 μm. (E) Caco-2 shSCR or shNRP1 cells were inoculated with MOI=50 of SARS-CoV-2 and incubated in the cold for 60 min, and fixed. A two-step antibody staining procedure was performed using anti-S and -N Abs to distinguish external (green) and total (red) virus particles, and the binding of particles per cell was quantified for over 3300 particles per condition (N=3). Two-tailed unpaired t-test; P=0.6859. (F) Caco-2 shSCR or shNRP1 cells were bound with SARS-CoV-2 as in (E), followed by incubation at 37 °C for 30 min. The cells were fixed and stained as in (E). Viral uptake was quantified for over 4200 particles per condition (N=3). Two-tailed unpaired t-test; P=0.00079. Scale bars for (E) and (F) = 10 μm and 200 nm (zoom panels). The square regions were zoomed in.
The bars, error bars, circles and triangles represent the mean, SEM (B) and SD (C-F), individual data points, respectively. *P< 0.05, **P< 0.01, ***P< 0.001, ****P< 0.0001.
To probe the functional relevance of this interaction, we generated HeLa wild type and NRP1 knock out (KO) cell lines stably expressing ACE2, designated as HeLawt+ACE2 and HeLaNRP1KO+ACE2 respectively (the level of ACE2 expression was comparable between these lines) (Fig. S1F). Using a clinical isolate SARS-CoV-2 (SARS-CoV-2/human/Liverpool/REMRQ001/2020), we performed viral infection assays and fixed the cells at 6 and 16 hours post infection (hpi). SARS-CoV-2 infection was reduced in HeLaNRP1KO+ACE2 relative to HeLawt+ACE2 (Fig. 1C). HeLa cells lacking ACE2 expression were not infected (Fig. S1G). In Caco-2 cells, a human colon adenocarcinoma cell line endogenously expressing ACE2 and widely used in COVID-19 studies, the suppression of NRP1 expression by shRNA greatly reduced SARS-CoV-2 infection at both 7 and 16 hpi respectively, whereas that of vesicular stomatitis virus (VSV) pseudotyped with VSV-G was unaffected (Fig. 1D, S1H, S2A). To determine if NRP1 was required for early virus infection, we established a sequential staining procedure using antibodies against SARS-CoV-2 S and N proteins to distinguish extracellular and intracellular viral particles (Fig. S2B). While NRP1 depletion did not affect SARS-CoV-2 binding to the Caco-2 cell surface (Fig. 1E), virus uptake was halved in NRP1-depleted cells compared to control cells after 30 minutes of internalization (Fig. 1F). NRP1 therefore enhances SARS-CoV-2 entry and infection.
We also observed that SARS-CoV-2-infected HeLawt+ACE2 cells displayed a multi-nucleated syncytia cell pattern, as reported by others (Fig. 1C) (5). Using an image analysis algorithm and supervised machine learning (Fig. S2C-F) (11), we quantified syncytia of infected HeLawt+ACE2 and HeLaNRP1KO+ACE2 cells. At 16 hpi, the majority of HeLawt+ACE2 cells formed syncytia, while in HeLaNRP1KO+ACE2 cells this phenotype was reduced (Fig. S2G). When infected with a SARS-CoV-2 isolate lacking the furin cleavage site (SARS-CoV-2 ΔS1/S2) (Fig. S1A) the differences in infection and syncytia formation were less pronounced (Fig. S2H, S2I). However, a significant decrease in infection of HeLaNRP1KO+ACE2 was still observed at 16 hpi, indicating that NRP1 may additionally influence infection through a CendR-independent mechanism (Fig. S2H).
The extracellular regions of NRP1 and NRP2 are composed of two CUB domains (a1 and a2), two coagulation factor domains (b1 and b2), and a MAM domain (9). Of these, the b1 domain contains the specific binding site for CendR peptides (Fig. S3A) (12). Accordingly, the mCherry-b1 domain of NRP1 immunoprecipitated GFP-S1, and a shortened GFP-S1 construct spanning residues 493-685 (Fig. S1C, S3B). Isothermal titration calorimetry (ITC) established that the b1 domain of NRP1 directly bound a synthetic S1 CendR peptide (679NSPRRAR685) with an affinity of 20.3 μM at pH 7.5, that was enhanced to 13.0 μM at pH 5.5 (Fig. 2A). Binding was not observed to a S1 CendR peptide in which the C-terminal arginine was mutated to alanine (679NSPRRAA685) (Fig. 2A). We co-crystallized the NRP1 b1 domain in complex with S1 CendR peptide (Fig. 2B). The resolved 2.35 Å structure revealed 4 molecules of b1 with electron density of the S1 CendR peptide clearly visible in the asymmetric unit (Fig. S3C). S1 CendR peptide binding displayed remarkable similarity to the previously solved structure of NRP1 b1 domain in complex with its endogenous ligand VEGF-A164 (Fig. 2B, S3D) (12). The key residues responsible for contacting the C-terminal R685 of the CendR peptide - Y297, W301, T316, D320, S346, T349 and Y353 - are almost identical between the two structures (Fig. 2B, S3D). The R682 and R685 sidechains together engage NRP1 via stacked cation-π interactions with NRP1 side chains of Y297 and Y353. By projecting these findings onto the structure of the NRP1 ectodomain, the b1 CendR binding pocket appears to be freely accessible to the S1 CendR peptide (Fig. S3E) (13).
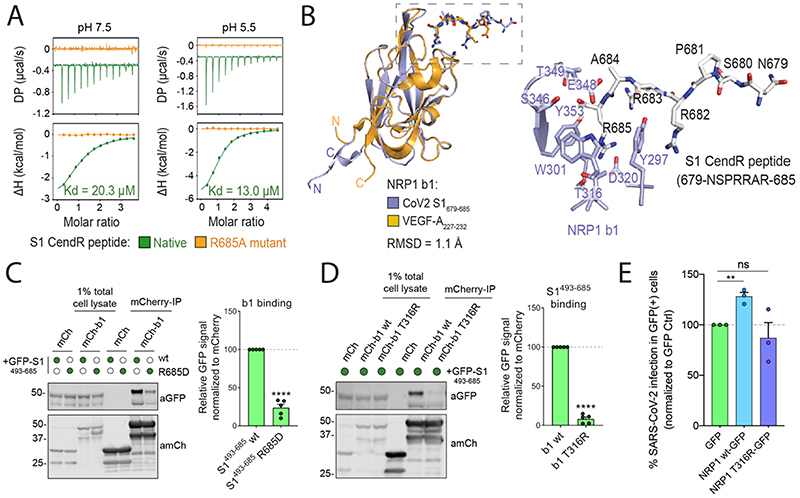
(A) Binding of NRP1 b1 with native (green line) and mutant (orange line) form of S1 CendR peptide (corresponding to residues 679-685) by ITC at two different pH conditions (N=3). All ITC graphs represents the integrated and normalized data fit with 1-to-1 ratio binding. (B) Left: NRP1 b1 – S1 CendR peptide complex superposed with NRP1 b1 – VEGF-A fusion complex (PDB ID: 4DEQ). Bound peptides are shown in stick representation. RMSD = root mean square deviation. Right: Enlarged view highlighting the binding of S1 CendR peptide b1. Key binding residues on b1 are shown in stick representation. (C). HEK293T cells were co-transfected with combinations of GFP-tagged S1493-685 and S1493-685 R685D, and mCherry or mCherry-NRP1 b1, and subjected to mCherry-nanotrap (N=5). Two-tailed unpaired t-test; P <0.0001. (D). HEK293T cells were co-transfected with combinations of GFP-tagged S1493-685 and mCherry, mCherry-NRP1 b1 or mCherry-NRP1 b1 T316R mutant and subjected to mCherry-nanotrap (N=5). Two-tailed unpaired t-test; P <0.0001. (E) HeLaNRP1KO + ACE2 cells transfected with GFP, NRP1 wt-GFP or NRP1 T316R-GFP constructs were infected 24 h later with SARS-CoV-2. At 16 hpi the cells were fixed and stained for SARS-CoV-2-N, and viral infection quantified in the GFP-positive subpopulation of cells (N=3). The percentage of infection was normalized to that of GFP-transfected cells. Two-tailed unpaired t-test; p = 0.002. The bars, error bars and circles represent the mean, SEM (C-D) and SD (E), individual data points, respectively. *P< 0.05, **P< 0.01, ***P< 0.001, ****P< 0.0001.
Site-directed mutagenesis of the S1 R685 residue to aspartic acid drastically reduced GFP-S1493-685 immunoprecipitation by mCherry-b1, confirming the critical role of the C-terminal arginine (Fig. 2C). Mutagenesis of the T316 residue within the mCherry-b1 domain of NRP1 to arginine also reduced association with GFP-S1493-685, consistent with its inhibitory impact on VEGF-A164 binding (12) (Fig. 2D). Accordingly, incubation of mCherry-b1 with VSV particles pseudotyped with trimeric S resulted in immunoprecipitation of processed forms of S1, which was dependent on the T316 residue (Fig. S3F). Next, we transiently expressed either GFP, full length NRP1 wt-GFP or full length NRP1-GFP harboring the T316R mutation in HeLaNRP1KO+ACE2 cells. GFP expression and ACE2 expression levels were comparable and both constructs retained similar cell surface localization (Fig. S3G, S3H). SARS-CoV-2 infection was significantly enhanced in cells expressing NRP1 wt-GFP compared to GFP control, whereas it was not enhanced in cells expressing the T316R mutant (Fig. 2E). Thus, the SARS-CoV-2 S1 CendR and NRP1 interaction promotes infection.
To establish the functional relevance of the S1 CendR-NRP1 interaction, we screened monoclonal antibodies (mAb#1, mAb#2, mAb#3) raised against the NRP1 b1b2 ectodomain.
All three bound to the NRP1 b1b2 domain, displayed staining by immunofluorescence in NRP1-expressing PPC-1 (human primary prostate cancer) cells but not in M21 (human melanoma) cells that do not express NRP1 (Fig. S4A) (8), and stained the extracellular domain of NRP1-GFP expressed in cells (Fig. S4B). Of these antibodies, mAb#3, and to a lesser extent mAb#1, bound to the CendR-binding pocket with high specificity, as defined by reduced ability to bind to a b1b2 mutant that targets residues (S346, E348, T349) at the opening of the binding pocket (Fig. 3A) (12). Incubation of Caco-2 cells with mAbs#1 and 3, reduced SARS-CoV-2 infection compared to a control mAb targeting avian influenza A virus (H11N3) hemagglutinin (Fig. 3B). Consistent with this, mAb#3 inhibited binding of GFP-S1493-685 and mCherry-b1 (Fig. 3C). As a comparison, Caco-2 and Calu-3 cells were incubated with soluble ACE2, which inhibited SARS-CoV-2 infection in both cases (Fig. S4C).
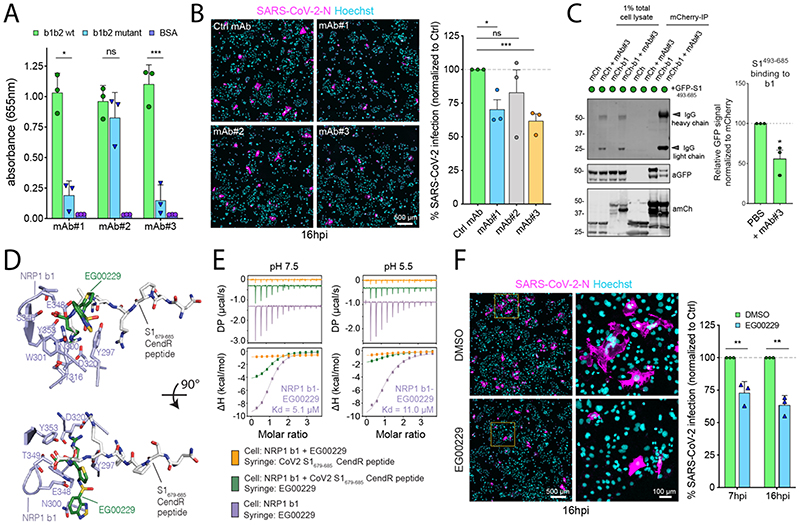
(A) ELISA of anti-NRP1 monoclonal antibodies (mAb#1, mAb#2, mAb#3) at 3 μg/mL using plates coated with NRP1 b1b2 wild type, b1b2 mutant (S346A, E348A, T349A) or BSA, used as control (N=3). Binding is represented as arbitrary units of absorbance at 655 nm. Two-tailed unpaired t-test; P = 0.0207, 0.2430, 0.0007. (B) Cells were pre-treated with 100 μg/mL of anti-H11N3 (Ctrl) mAb, mAb#1, 2 or 3 for 1 h prior to infection with SARS-CoV-2. Cells were fixed at 16 hpi and stained for N protein (magenta) and Hoechst (cyan) (N=3). Two-tailed unpaired t-test; P=0.015, 0.36, 0.0003. Scale bar=500 μm. (C) HEK293T cells were co-transfected with combinations of mCherry or mCherry-b1 and GFP-tagged S1493-685 and subjected to mCherry-nanotrap with or without co-incubation with mAb#3 (N=3). Two-tailed unpaired t-test; P = 0.0143. (D) NRP1 b1 – S1 CendR peptide complex superimposed with NRP1 b1 – EG00229 inhibitor complex (PDB ID:3I97). Key binding residues on b1, bound peptides and EG00229 are shown in stick representation. (E) ITC analysis of EG00229 binding to b1 domain of NRP1 at two different pH conditions. Pre-incubation with EG00229 blocks S1 CendR peptide binding (orange line), and the CendR peptide can reduce binding of EG00229 (green line). (N=3). All ITC graphs represents the integrated and normalized data fit with 1-to-1 ratio binding. (F). Cells were pre-treated with 100 μM of EG00229 or DMSO prior to infection with SARS-CoV-2. Cells were fixed at 7 and 16 hpi and stained for N protein (magenta) and Hoechst (cyan) (N=3). The square region was zoomed in. Scale bars=500 μm and 100 μm (zoom panel). Two-tailed unpaired t-test; P = 0.0059 and 0.0013. The bars, error bars, circles and triangles represent the mean, SEM (C) and SD (A, B, F) and individual data points, respectively. *P< 0.05, **P< 0.01, ***P< 0.001, ****P< 0.0001.
Next, we turned to the small molecule EG00229, a selective NRP1 antagonist that binds the b1 CendR binding pocket and inhibits VEGF-A binding (Fig. 3D) (14). ITC established that EG00229 bound to the NRP1 b1 domain with a Kd of 5.1 and 11.0 μM at pH 7.5 and 5.5 respectively (Fig. 3E). EG00229 inhibited the direct binding between b1 and the S1 CendR peptide, and the immunoprecipitation of GFP-S1493-685 by mCherry-b1 (Fig. 3E and S4D). Finally, incubation of Caco-2 cells with EG00229 reduced the efficiency of SARS-CoV-2 infection at 7 and 16 hpi (Fig. 3F). Thus, the SARS-CoV-2 interaction with NRP1 can be targeted to reduce viral infectivity in relevant human cell lines (Fig. S5).
Cell entry of SARS-CoV-2 depends on priming by host cell proteases (5,6,15). Our data indicate that a component of SARS-CoV-2 S protein binding to cell surface neuropilins occurs via the S1 CendR motif generated by the furin cleavage of S1/S2. While not affecting cell surface attachment, this interaction promotes entry and infection by SARS-CoV-2 in physiologically relevant cell lines widely used in the study of COVID-19. The molecular basis for the effect is unclear, but neuropilins are known to mediate the internalization of CendR ligands through an endocytic process resembling macropinocytosis, (8,16,17). Interestingly, gene expression analysis has revealed an up-regulation of NRP1 and NRP2 in lung tissue from COVID-19 patients (18). A SARS-CoV-2 virus with a natural deletion of the S1/S2 furin cleavage site demonstrated attenuated pathogenicity in hamster models (19). NRP1 binding to the CendR peptide in S1 is thus likely to play a role in the increased infectivity of SARS-CoV-2 compared with SARS-CoV. The ability to target this specific interaction may provide a route for COVID-19 therapies.
Acknowledgments
We thank the Bristol Synthetic Biology Centre and the Advanced Computing Research Centre for provision of HPC (Bluegem), and the University of Bristol Wolfson Bioimaging Facility. We thank the University of Queensland Remote Operation Crystallisation and X-ray facility (UQ-ROCX) and the staff for their support with the crystallization experiments, and the staff of the Australian Synchrotron for assistance with X-ray diffraction data collection.
Funding
JLD was supported by a Wellcome Trust studentship from the Dynamic Molecular Cell Biology Ph.D. programme (203959/Z/16/Z), CAP was supported by Beca Fundación Ramón Areces Estudios Postdoctorales en el Extranjero and MKW was supported by a MRC grant (MR/R020566/1) awarded to ADD. This project has received funding from the MRC (MR/P018807/1), Wellcome Trust (104568/Z/14/2), Lister Institute of Preventive Medicine, and Elizabeth Blackwell Institute for Health Research Rapid Response Call (COVID-19) awarded to PJC, the European Research Council under the European Union’s Horizon 2020 research and innovation programme (No 856581 - CHUbVi), and from MRC-AMED (MR/T028769/1) awarded to YY, the Swiss National Science Foundation and Kanton Zurich awarded to UFG. BMC is supported by an Australian National Health and Medical Research Council (NHMRC) Senior Research Fellowship (APP1136021) and Project Grant (APP1156493), and the United States Food and Drug Administration grant number HHSF223201510104C ‘Ebola Virus Disease: correlates of protection, determinants of outcome and clinical management’ amended to incorporate urgent COVID-19 studies awarded to JAH, ADD and DAM. RH and PH acknowledge support from the LENDULET-BIOMAG Grant (2018-342), from H2020-discovAIR (874656), and from Chan Zuckerberg Initiative, Seed Networks for the HCA-DVP. TT was supported by the European Regional Development Fund (Project No. 2014-2020.4.01.15-0012), by European Research Council grant GLIOGUIDE and Estonian Research Council (grants PRG230 and EAG79, to T.T.).
Footnotes
Author Contributions
JLD, BS, AH, PJC and YY conceived the study. JLD, BS, KK and YY performed most of the experiments. KK, MKW, DAM and ADD performed all work with infectious SARS-CoV-2 supervised by ADD. MKW and ADD isolated SARS-CoV-2 strains used for the work. KC, CAP, MB, LSG, UFG, KK, RBS, DKS, JAH and TT did experimental work and/or provided essential reagents. RH and PH performed image analysis. BS, ADD, BMC, PJC and YY supervised the research. JLD, BS, ADD, PJC and YY wrote the manuscript and made the figures. All authors read and approved the final manuscript.Competing Interests
T. Teesalu is an inventor of patents on CendR peptides and shareholder of Cend Therapeutics Inc., a company that holds a license for the CendR peptides and is developing the peptides for cancer therapy. J. Hiscox is a member of the Department of Health, New and Emerging Respiratory Virus Threats Advisory Group (NERVTAG) and the Department of Health, Testing Advisory Group. U. Greber is a consultant to F. Hoffmann-La Roche Ltd, Switzerland. All other authors declare no competing interests.
Data and Materials Availability
All data are available in the manuscript or the supplementary material.
References
Full text links
Read article at publisher's site: https://doi.org/10.1126/science.abd3072
Read article for free, from open access legal sources, via Unpaywall:
https://www.science.org/cms/asset/b7734c5b-6668-41ee-a2a4-c252ba76fc5b/pap.pdf
Citations & impact
Impact metrics
Citations of article over time
Alternative metrics
Article citations
Facilitating and restraining virus infection using cell-attachable soluble viral receptors.
Proc Natl Acad Sci U S A, 121(45):e2414583121, 31 Oct 2024
Cited by: 0 articles | PMID: 39480852 | PMCID: PMC11551432
Gene expression profiles of precursor cells identify compounds that reduce NRP1 surface expression in macrophages: Implication for drug repositioning for COVID-19.
Front Cardiovasc Med, 11:1438396, 24 Oct 2024
Cited by: 0 articles | PMID: 39512370 | PMCID: PMC11541348
Cardiovascular and kidney diseases are positively associated with neuroinflammation and reduced brain-derived neurotrophic factor in patients with severe COVID-19.
Brain Behav Immun Health, 41:100855, 19 Sep 2024
Cited by: 0 articles | PMID: 39391797 | PMCID: PMC11466569
Structural basis for receptor-binding domain mobility of the spike in SARS-CoV-2 BA.2.86 and JN.1.
Nat Commun, 15(1):8574, 07 Oct 2024
Cited by: 0 articles | PMID: 39375326 | PMCID: PMC11458767
HLA-G neo-expression modifies genetic programs governing tumor cell lines.
Cancer Immunol Immunother, 73(12):247, 03 Oct 2024
Cited by: 0 articles | PMID: 39358558 | PMCID: PMC11447172
Go to all (792) article citations
Data
Data behind the article
This data has been text mined from the article, or deposited into data resources.
BioStudies: supplemental material and supporting data
Protein structures in PDBe (2)
-
(1 citation)
PDBe - 4DEQView structure
-
(1 citation)
PDBe - 3I97View structure
Protocols & materials
Ximbio (3)
Similar Articles
To arrive at the top five similar articles we use a word-weighted algorithm to compare words from the Title and Abstract of each citation.
Neuropilin-1 facilitates SARS-CoV-2 cell entry and infectivity.
Science, 370(6518):856-860, 20 Oct 2020
Cited by: 1210 articles | PMID: 33082293 | PMCID: PMC7857391
Optimized Pseudotyping Conditions for the SARS-COV-2 Spike Glycoprotein.
J Virol, 94(21):e01062-20, 14 Oct 2020
Cited by: 86 articles | PMID: 32788194 | PMCID: PMC7565639
Broad and Differential Animal Angiotensin-Converting Enzyme 2 Receptor Usage by SARS-CoV-2.
J Virol, 94(18):e00940-20, 31 Aug 2020
Cited by: 113 articles | PMID: 32661139 | PMCID: PMC7459545
ACE2: Evidence of role as entry receptor for SARS-CoV-2 and implications in comorbidities.
Elife, 9:e61390, 09 Nov 2020
Cited by: 208 articles | PMID: 33164751 | PMCID: PMC7652413
Review Free full text in Europe PMC
Funding
Funders who supported this work.
Beca Fundación Ramón Areces Estudios Postdoctorales en el Extranjero
Biotechnology and Biological Sciences Research Council (2)
Grant ID: BB/M02542X/1
BrisSynBio: Bristol Centre for Synthetic Biology
Prof Derek Neil Woolfson, University of Bristol
Grant ID: BB/L01386X/1
Estonian Research Council (1)
Grant ID: PRG230 and EAG79
European Regional Development Fund (1)
Grant ID: 2014-2020.4.01.15-0012
European Research Council (2)
Grant ID: No 856581 - CHUbVi
Grant ID: GLIOGUIDE
H2020-discovAIR (1)
Grant ID: 874656
LENDULET-BIOMAG (1)
Grant ID: 2018-342
MRC-AMED (1)
Grant ID: MR/T028769/1
Medical Research Council (4)
The production and application of SARS-CoV-2 reverse genetic systems to facilitate vaccine development and biosafe drug discovery platforms.
Professor Andrew Davidson, University of Bristol
Grant ID: MR/V027506/1
Defining the role of retromer-like in endolysosomal cargo sorting in health and disease.
Professor Peter Cullen, University of Bristol
Grant ID: MR/P018807/1
Analysis of flavivirus infection on the cellular lipidome - implications for virus particle production and replication.
Professor Andrew Davidson, University of Bristol
Grant ID: MR/R020566/1
MRC AMED - The HDAC6/USP7 axis in enveloped RNA viral infection
Professor Anne Ridley, University of Bristol
Grant ID: MR/T028769/1
Swiss National Science Foundation
U.S. Food and Drug Administration (1)
Grant ID: HHSF223201510104C
Wellcome Trust (4)
Grant ID: 104568/Z/14/Z
University of Bristol - Dynamic Molecular Cell Biology
Mr James Daly, University of Bristol
Grant ID: 203959
University of Bristol - Dynamic Molecular Cell Biology
Mr James Daly, University of Bristol
Grant ID: 203959/Z/16/Z
Defining the role of retromer in endosomal sorting in health and disease.
Professor Peter Cullen, University of Bristol
Grant ID: 104568
Wellcome Trust Centre for Mitochondrial Research (1)
Grant ID: 104568/Z/14/2